Flavonoids are polyphenolic compounds present in vegetables, tea, red wine and fruits and are widely consumed as part of a habitual diet(Reference Manach, Scalbert and Morand1). Flavonoids that are present in citrus fruits possess diverse beneficial effects, including anti-oxidative and anti-inflammatory properties(Reference Testai and Calderone2,Reference Yi, Ma and Ren3) . Several gastrointestinal diseases, such as inflammatory bowel disease and celiac disease, have been associated with increased levels of oxidative stress and inflammatory mediators, contributing to intestinal barrier disruption(Reference Tian, Wang and Zhang4,Reference Turner5,Reference Ferretti, Bacchetti and Masciangelo6) . Certain pro-inflammatory cytokines such as TNF-α and IFN-γ are described to increase intestinal permeability(Reference Xu, Elamin and Elizalde7,Reference Smyth, Phan and Wang8) . An increased intestinal permeability may result in translocation of luminal content, further triggering the inflammatory response, thereby contributing to disease onset and progression. Via their anti-oxidative and anti-inflammatory properties, citrus flavonoids may beneficially affect intestinal barrier function and intestinal health. Hesperidin and naringin are two important flavonoids present in citrus fruits that are largely resistant to hydrolysation in the stomach and small intestine. Once reaching the colon, they are extensively metabolised by the gut microbiota. Here, hesperidin and naringin are first converted into their aglycones hesperetin and naringenin and subsequently into various smaller phenolics(Reference Pereira-Caro, Borges and Ky9,Reference Brett, Hollands and Needs10,Reference Nielsen, Chee and Poulsen11) . A recent study using an in vitro digestion model of the gastrointestinal tract showed that after digestion of hesperidin, the phenolic metabolites found at the highest concentrations were dihydrocaffeic acid, isoferulic acid, 4-hydroxyphenylacetic acid and dihydroferulic acid. These metabolites were mainly present in the compartments representing the proximal parts of the colon(Reference Van Rymenant, Salden and Voorspoels12).
Beneficial effects of citrus flavonoids as well as their aglycones on intestinal health have been observed in various studies employing rodent colitis models(Reference Azuma, Shigeshiro and Kodama13,Reference Shigeshiro, Tanabe and Suzuki14,Reference Xu, Yang and Li15,Reference Crespo, Galvez and Cruz16) . Citrus flavonoids at dosages ranging from 10 mg/kg to 3 g/kg resulted in decreased colonic permeability, inflammation, oxidative stress and disease activity index scores(Reference Azuma, Shigeshiro and Kodama13,Reference Shigeshiro, Tanabe and Suzuki14,Reference Xu, Yang and Li15,Reference Crespo, Galvez and Cruz16) . Furthermore, in vitro studies using intestinal cell lines have shown beneficial effects of citrus flavonoid aglycones or phenolic metabolites on either intestinal barrier function(Reference Noda, Tanabe and Suzuki17,Reference Noda, Tanabe and Suzuki18,Reference Bergmann, Rogoll and Scheppach19,Reference He, Liu and Xu20) or inflammation(Reference Larrosa, Luceri and Vivoli21), indicating that both the parent compounds that are ingested and the (phenolic) metabolites formed are able to exert beneficial effects. However, as these in vitro models did not include the crosstalk between epithelial cells and immune cells, assessed only one or a few metabolites and/or did not include the parent compounds, the effects of the metabolites on immune-mediated barrier disruption and their relative contribution compared with the parent compounds remain unclear.
Therefore, the aim of this study was to assess the potential beneficial effects of hesperidin, naringin and several of the main metabolites formed by bacterial metabolism in the intestine on immune-mediated intestinal barrier disruption, using an in vitro co-culture model of intestinal inflammation and barrier dysfunction.
Methods
Test compounds
The citrus flavonoid extract used in this study (MicrobiomeX®, containing >85 % hesperidin and >5 % naringin) and hesperidin (Cordiart®) were kindly provided by BioActor BV. Hesperetin, naringin, naringenin, dihydrocaffeic acid, 4-hydroxyphenylacetic acid, ferulic acid, hydrocinnamic acid, phloretic acid and phloroglucinol were purchased at Sigma-Aldrich. Isoferulic acid and dihydroferulic acid were purchased at TCI Europe. All compounds were administered at a concentration of 100 µM based on previous in vitro studies(Reference Noda, Tanabe and Suzuki17,Reference Noda, Tanabe and Suzuki18) and because it is anticipated that both the parent compounds and metabolites can reach concentration in this order of magnitude in the intestinal lumen after oral consumption of citrus flavonoids(Reference Van Rymenant, Salden and Voorspoels12,Reference Noda, Tanabe and Suzuki17,Reference Gao, Xu and Krul22) . Furthermore, to investigate whether similar effects can be expected at lower dosages, several experiments were also performed at a dose of 50 µM for the following compounds: hesperidin, naringin, hesperetin, naringenin, isoferulic acid and 4-hydroxyphenylacetic acid. These compounds were selected as they showed the most promising effects and represent different stages of intestinal metabolism (i.e. parent compounds, aglycones and phenolic metabolites). All test compounds were first dissolved in dimethylsulfoxide (DMSO; Applichem) and diluted in complete Dulbecco’s Modified Eagle Medium (composition as described below) resulting in a final concentration of 0·1 % DMSO.
Cell cultures
Caco-2 cells were derived from the American Type Culture Collection and were maintained in Dulbecco’s Modified Eagle Medium high glucose (Cat no. D5796, Sigma-Aldrich), supplemented with 10 % fetal bovine serum (cat no. 26140079, Invitrogen), 1 % Antibiotic-Antimycotic (cat no. 15240062, Invitrogen) and 1 % non-essential amino acids (cat no. 11140035, Invitrogen) at 37 °C in a 5 % CO2 atmosphere.
THP-1-Blue™ NF-κB cells (InvivoGen) were kindly provided by ProDigest BVBA. These cells were maintained in Roswell Park Memorial Institute medium 1640 (cat no. R8758, Sigma-Aldrich) supplemented with 10 % fetal bovine serum (Invitrogen), 1 % Antibiotic-Antimycotic (Invitrogen) and 1 % sodium pyruvate (cat no. 11360070, Invitrogen). THP-1 cells are human monocytic leukaemia cells that differentiate into macrophage-like cells after treatment with phorbol 12-myristate 13-acetate (PMA)(Reference Takashiba, Van Dyke and Amar23). THP-1-Blue cells include an NF-κB-inducible secreted embryonic alkaline phosphatase (SEAP) reporter construct, enabling monitoring of NF-κB activity by use of the colorimetric QUANTI-BlueTM enzyme assay (cat code rep-qb1, InvivoGen).
Assessment of cytotoxicity
The cytotoxic effect of the test compounds was assessed by a fluorometric lactate dehydrogenase assay (CytoTox-ONETM Homogeneous Membrane Integrity Assay, Promega) according to the manufacturer’s protocol. Caco-2 cells in 96-well plates (Greiner CELLSTAR) were incubated with the test compounds for 24 h, after which lactate dehydrogenase release into the culture medium was determined as described previously(Reference Xu, Elamin and Elizalde7). Cytotoxicity of the test compounds was expressed as percentage of the values obtained for the 100 % cell lysis control by Triton® X-100.
Caco-2/THP-1 co-culture experiments
Co-culture experiments were performed as described previously by Possemiers et al. (Reference Possemiers, Pinheiro and Verhelst24). Briefly, Caco-2 cells (passage 30–36) were seeded into 24-well Transwell inserts (cat no. 3470, Corning) at a density of 80·000 cells per insert and cultured for 14 d until a functional monolayer with a transepithelial electrical resistance (TEER) of > 330 Ω•cm2 was formed(Reference Satsu, Ishimoto and Nakano25), before being placed in co-culture. Two days preceding the co-culture experiment, the THP-1 cells were seeded in 24-well plates at a density of 500·000 cells/well in the presence of 100 ng/mL PMA (cat no. P8139, Sigma-Aldrich) prepared in Roswell Park Memorial Institute 1640 (Sigma-Aldrich). PMA-stimulated THP-1 cells produce considerable amounts of inflammatory mediators, which when placed in co-culture with Caco-2 cells, can contribute to a disruption of the cell monolayer(Reference Satsu, Ishimoto and Nakano25). After 2 d of PMA treatment, the THP1-cells were washed with PBS, placed in Caco-2 culture medium and the inserts containing the Caco-2 monolayer were placed on top of the THP-1 cells (Fig. 1). Subsequently, the test products were added apically to the Transwell inserts. Cells were also treated with culture medium containing 0·1 % DMSO as vehicle control, this condition will hereafter be referred to as the medium + DMSO control. After a treatment period of 24 h, the THP-1 cells were incubated with 500 ng/ml ultrapure lipopolysaccharide (LPS) from E. coli (cat code tlrl-peklps, InvivoGen) in the basolateral compartment for an additional 6 h. Binding of LPS to toll-like receptor 4 results in NF-kB activation and production of cytokines(Reference Sharif, Bolshakov and Raines26). After the 6-h co-incubation period, the basolateral medium was collected and stored at -80 °C.

Fig. 1. Schematic overview of the co-culture model with Caco-2 cells and THP-1-cells. Caco-2 cells cultured in Transwell inserts for 14 d were placed in co-culture with PMA-stimulated THP-1-BlueTM NF-κB cells. After 24 h of incubation, THP-1 cells were incubated with 500 ng/ml LPS in the basolateral compartment for an additional 6 h.
Measurement of barrier function
The epithelial integrity of the Caco-2 cell monolayers was assessed by measurement of TEER, fluorescein isothiocyanate–dextran 4 kDa (FITC-D4) permeation and gene expression of different barrier-related genes. TEER of the Caco-2 monolayers was measured at baseline, after 24 h co-incubation with the test compounds and after another 6 h of co-culture with the THP-1 cells (i.e. t = 30 h). TEER was measured using the EVOM2 Epithelial Voltohmmeter (World Precision Instruments). Monolayer resistance of treated wells was calculated by subtracting the TEER of empty inserts and multiplying these with the surface area of the filter membrane. Data are reported as TEER percentage of baseline values. FITC-D4 (1 mg/ml; Sigma-Aldrich) was added apically to the Caco-2 cells at t = 30 h and incubated for 1 h at 37 °C, after which the basolateral medium was collected and stored at −20 °C for further analysis. FITC-D4 concentrations were measured by fluorimetry using a SpectraMax M2 microplate reader (Molecular Devices) at excitation and emission wavelengths of 485 nm and 530 nm, respectively. Gene expression of barrier-related genes (i.e. zonula occludens-1, occludin, claudin-2, claudin-3, claudin-4, E-cadherin and myosin light chain kinase) was evaluated for the following six compounds: hesperidin, naringin, hesperetin, naringenin, isoferulic acid and 4-hydroxyphenylacetic acid at t = 30 h. RNA extraction, reverse transcription and qPCR were performed as described previously(Reference Xu, Elamin and Elizalde7). Briefly, RNA was extracted using the Qiagen RNeasy Mini kit (QIAGEN). After cells were lysed by adding lysis buffer to the monolayers, RNA was isolated and purified with on-column DNase digestion. All qPCR were performed with the iQ SYBR® Green Supermix (Bio-Rad Laboratories) on a thermal cycler (Bio-Rad Laboratories). The qPCR conditions used were 10 min at 95 °C, followed by 40 amplification cycles of 10 s at 95 °C and 40 s at 60 °C. Primer sequences used are listed in online Supplementary Table S1. Expressions of target genes were normalised to 18S ribosomal RNA (18S RNA) as reference gene as described previously(Reference Wilms, Jonkers and Savelkoul27).
Measurement of NF-κB activity and cytokine secretion
After stimulation with LPS, NF-κB activity of THP-1 cells was measured by use of the QUANTI-BlueTM enzyme assay (InvivoGen) according to the manufacturer’s instructions. In brief, 20 μl of the basolateral medium was incubated for 15 min with 200 μl QUANTI-Blue at 37 °C. Optical density was measured at 630 nm using the Synergy HT microplate reader (BioTek Instruments). Secreted cytokine levels were quantified in basolateral medium using a cytometric bead array flex set assay (BD Biosciences) followed by measurement with a flow cytometer (BD FACS Canto II™), as described previously(Reference Oth, Habets and Germeraad28). Levels of the pro-inflammatory cytokines IL-8, TNF-α, IL-6 and IL-1β were measured, with respective detection limits of 156·25, 39, 39 and 78 pg/ml. Data are expressed as percentage of the vehicle control to correct for limited interassay variation.
Statistical analysis
Data are presented as mean values with their standard error of the mean of three independent experiments, with three technical replicates. In the incidental case that one of the three replicates deviated more than 30 % from the median, this value was considered a technical outlier and removed from the data set. The final data were obtained from at least two independent experiments, each conducted at least in duplicate. For all cytokines, values below the detection limit were imputed with the corresponding detection limit. Statistical analyses were performed with an independent samples t-test using IBM SPSS Statistics version 25 (IBM Statistics) based on the individual values of each replicate. Statistical significance was set at P < 0·05. For the TEER, gene expression and cytokine results, P-values were corrected for multiple comparisons using the false discovery rate of Benjamini–Hochberg.
Results
Intestinal barrier function
As citrus flavonoids have anti-inflammatory potential, a Caco-2/THP-1 co-culture model was used(Reference Possemiers, Pinheiro and Verhelst24) to assess whether these compounds may be able to exert anti-inflammatory effects and thereby attenuate cytokine-induced barrier disruption. Prior to examining these effects, the cytotoxicity of all test compounds was evaluated by measurement of lactate dehydrogenase release from cells. These results showed that there were no significant differences in percentage cytotoxicity of the test compounds at the chosen working concentration of 100 µM as compared with the medium + DMSO control (all ≤ 10·7 % and P ≤ 0·18).
In this co-culture model, PMA-induced differentiation of the THP-1 cells resulted in a decrease in TEER of approximately 30 % (ranging from 23·4 to 35·4 %) compared with baseline (set at 100 %), indicating that Caco-2 monolayer integrity was compromised after 24 h of co-culture. Apical treatment with the citrus flavonoids, their aglycones and their metabolites at concentrations of 100 µM or 50 µM did not attenuate this decrease in TEER values (Fig. 2(a); online Supplementary Fig. S1a). After incubation for an additional 6 h in the presence of LPS, no further differences in TEER values were observed (decrease of 23·5–37·7 % compared with baseline). Treatment with the citrus flavonoid test compounds did not affect barrier function after this additional incubation period at both dosages (Fig. 2(b); online Supplementary Fig. S1b). Paracellular permeability of the monolayers was also evaluated by FITC-D4 permeation after incubation with citrus flavonoids at concentrations of 100 and 50 µM. In line with the TEER results, treatment with the test compounds did not affect FITC-D4 permeation (online Supplementary Fig. S2a and S2b). Furthermore, no significant effects on expression levels of tight junction or adherens junction-related genes were observed after treatment with citrus flavonoids and their key metabolites (Fig. 3).

Fig. 2. The effects of citrus flavonoids, their aglycones and their metabolites on transepithelial electrical resistance (TEER) of Caco-2 monolayers after 24 h (a) and after 30 h (b) of co-culture with PMA-simulated THP-1 cells. At baseline, Caco-2 cells cultured in Transwell inserts for 14 d were placed in co-culture with PMA-stimulated THP-1-BlueTM NF-κB cells. Caco-2 cells were then incubated with either the test compounds (100 µM) or culture medium containing 0·1 % DMSO as vehicle control (medium + DMSO control) added to the apical compartment. After 24 h of incubation, THP-1 cells were incubated with 500 ng/ml LPS in the basolateral compartment for an additional 6 h. TEER was measured at baseline, after 24 h and after 30 h of incubation. TEER values are expressed as mean percentage from the baseline value ± sem from at least two independent experiments in duplicate. No significant differences were observed between the test compounds and the medium + DMSO control. , control;
, compound.

Fig. 3. The effects of citrus flavonoids, their aglycones and their metabolites on expression levels of tight junction or adherens junction-related genes of Caco-2 monolayers in co-culture with PMA-simulated THP-1 cells. Caco-2 cells cultured in Transwell inserts for 14 d were placed in co-culture with PMA-simulated THP-1-BlueTM NF-κB cells. Caco-2 cells were then incubated with either culture medium containing 0·1 % DMSO (medium + DMSO control) or test compounds (100 µM) added to the apical compartment. RNA was extracted after 30 h of incubation. Expressions of target genes were normalised to 18S ribosomal RNA (18S RNA) as reference gene. Values are represented as mean values with their standard error of the mean. No significant differences were observed between the test compounds and the medium + DMSO control. A: ZO-1; B: occludin; C: claudin-2; D: claudin-3, E: claudin-4; F: E-cadherin; G: MLCK. ZO-1, zonula occldudens-1; MLCK, myosin light chain kinase. , control;
, compound.
NF-κB activity and cytokine secretion
The anti-inflammatory potential of the citrus flavonoids and their metabolites was evaluated by measuring NF-κB activity and cytokine secretion in the co-culture model after the 24-h treated THP-1 cells were incubated for 6 more hours with LPS. NF-κB activity was determined by assessment of NF-κB-inducible SEAP levels secreted into the basolateral medium. Incubation with LPS resulted in a significant increase in NF-κB-inducible SEAP levels secreted by the THP-1 cells (online Supplementary Fig. S3). The increase in NF-κB activity was significantly inhibited by all test compounds after treatment at a concentration of 100 µM except for phloroglucinol (Fig. 4; all P ≤ 0·03) and this effect seemed to be most pronounced for the phenolic metabolites isoferulic acid, 4-hydroxyphenylacetic acid and dihydroferulic acid. Except for hesperetin (P < 0·001), no significant effects of the citrus flavonoid test compounds on NF-κB activity were observed at a lower concentration of 50 µM (online Supplementary Fig. S4).
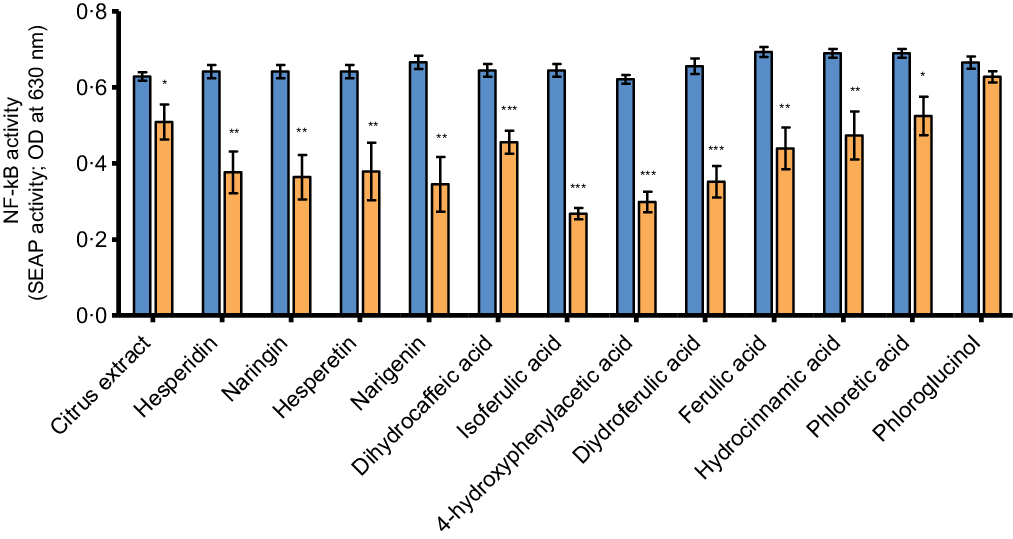
Fig. 4. The effects of citrus flavonoids, their aglycones and their metabolites on basolateral secretion of NF-κB-inducible SEAP after LPS stimulation by PMA-simulated THP-1 cells in co-culture with Caco-2 cells. At baseline, Caco-2 cells were placed in co-culture with PMA-stimulated THP-1-BlueTM NF-κB cells. Caco-2 cells were then incubated with either the test compounds (100 µM) or culture medium containing 0·1 % DMSO as vehicle control (medium + DMSO control) added to the apical compartment. After 24 h of incubation, THP-1 cells were incubated with 500 ng/ml LPS in the basolateral compartment for an additional 6 h. Levels of NF-κB-inducible SEAP were measured in basolateral medium after 30 h of incubation. Values are represented as mean values with their standard error of the mean from at least two independent experiments in duplicate. * represents significant difference from medium + DMSO control (*: P < 0·05; **: P < 0·01; ***: P < 0·001). , control + LPS;
, compound + LPS.
Cytokine production was determined by measuring the levels of the pro-inflammatory cytokines IL-8, TNF-α, IL-6 and IL-1β in the basolateral medium. Stimulation with LPS during the last 6 h of incubation resulted in significantly increased levels of these cytokines as compared with the control condition (cells treated with culture medium alone) (online Supplementary Fig. s5). Production of IL-8 was significantly inhibited by hesperidin, naringin, hesperetin, naringenin, isoferulic acid, 4-hydroxyphenylacetic acid, ferulic acid and hydrocinnamic acid (Fig. 5(a), all P ≤ 0·02). TNF-α levels were also significantly inhibited for all the aforementioned compounds and also for dihydroferulic acid (Fig. 5(b), all P ≤ 0·008). For IL-6, a similar pattern of inhibitory activity of the different test compounds could be observed, which was significant for isoferulic acid, dihydroferulic acid, ferulic acid and hydrocinnamic acid (Fig. 5(c), all P ≤ 0·01) and showed a trend towards significance for 4-hydroxyphenylacetic acid (P = 0·05). Although no IL-6 levels are depicted for hesperidin, naringin, hesperetin and naringenin, as the majority of the values were below the detection limit, the results do support the previous findings indicating that these compounds are able to inhibit the production of pro-inflammatory cytokines.

Fig. 5. The effects of citrus flavonoids, their aglycones and their metabolites on basolateral secretion of IL-8 (a), TNF-α (b), IL-6 (c) and IL-1β (d) after LPS stimulation by PMA-simulated THP-1 cells in co-culture with Caco-2 cells. At baseline, Caco-2 cells were placed in co-culture with PMA-stimulated THP-1-BlueTM NF-κB cells. Caco-2 cells were then incubated with either the test compounds (100 µM) or culture medium containing 0·1 % DMSO as vehicle control (medium + DMSO control) added to the apical compartment. After 24 h of incubation, THP-1 cells were incubated with 500 ng/ml LPS in the basolateral compartment for an additional 6 h. Cytokine levels were measured in basolateral medium after 30 h of incubation. Values are represented as mean values with their standard error of the mean from at least two independent experiments in duplicate. Absolute levels of IL-8:116–806 ng/ml; absolute levels of TNF-α: 39–959 pg/ml; absolute levels of IL-6:39–361 pg/ml; absolute levels of IL-1β: 78–1182 pg/ml. * represents significant difference from medium + DMSO control (*: P < 0·05; **: P < 0·01; ***: P < 0·001; #: P = 0·05). , control + LPS;
, compound + LPS.
In contrast, levels of IL-1β were increased when compared with the medium + DMSO control, being significant for hesperidin, naringin, hesperetin, naringenin, dihydrocaffeic acid, isoferulic acid, 4-hydroxyphenylacetic acid, dihydroferulic acid, ferulic acid and hydrocinnamic acid (Fig. 5(d), all P ≤ 0·02).
Discussion
In this in vitro study, we assessed the potential beneficial effects of the citrus flavonoids hesperidin and naringin, their aglycone forms and several of their phenolic metabolites on immune-mediated barrier disruption. Incubation with the citrus flavonoids and their metabolites did not induce changes in TEER, FITC-D4 permeation or expression of barrier-related genes for any of the compounds tested. However, LPS-induced NF-κB activity and production of the cytokines IL-8, TNF-α and IL-6 were inhibited by most compounds, whereas levels of IL-1β were found to be increased.
Chronic intestinal inflammation is characterised among others by an increased production of pro-inflammatory cytokines, which are important mediators that can disrupt the intestinal barrier via modulation of expression and functioning of tight junction proteins(Reference Turner5). Intestinal barrier disruption, associated with local or systemic inflammation, has been observed in several gastrointestinal and metabolic diseases, where it can play a role in the onset and progression of disease(Reference Vancamelbeke and Vermeire29). Recently, we found plasma of Crohn’s disease patients to induce epithelial barrier disruption in 3D Caco-2 cysts(Reference Xu, Elamin and Elizalde7).
In the present study, an in vitro co-culture system was used, mimicking immune-mediated barrier disruption by combining the epithelial Caco-2 cell line with activated macrophages(Reference Satsu, Ishimoto and Nakano25). Treatment with citrus flavonoids, their aglycone forms and their phenolic metabolites did not attenuate the decrease in barrier integrity that was observed after 24 h of co-culture. In contrast to our findings, two previous studies using Caco-2 cells did show positive effects after treatment with the aglycones hesperetin and naringenin, as shown by increased expression of several tight junction proteins and a 5–15 % increase in TEER(Reference Noda, Tanabe and Suzuki17,Reference Noda, Tanabe and Suzuki18) . The aforementioned studies focused on improving barrier function in the absence of a stressor, while the present study was performed in order to assess whether citrus flavonoid treatment can attenuate an inflammation-induced disruption of barrier function, as was suggested based on animal studies. Using chemically induced mice models of colitis, it was shown that oral administration with naringenin or hesperetin was able to (partly) suppress dextran sulfate sodium-induced barrier impairment, as measured by FITC-D4 and expression of tight junction proteins(Reference Azuma, Shigeshiro and Kodama13,Reference Shigeshiro, Tanabe and Suzuki14) . In these mice studies, the citrus flavonoid aglycones were given at a dose of 3 g/kg per d. This dose was estimated to correspond to a daily dose of approximately 2 g in humans(Reference Azuma, Shigeshiro and Kodama13), while more physiological dosages of approximately 50–500 mg, which are also typically used in human intervention studies, are expected to result in luminal concentrations similar to the concentration of 100 µM that was used in the present study(Reference Van Rymenant, Salden and Voorspoels12,Reference Noda, Tanabe and Suzuki17,Reference Gao, Xu and Krul22) .
Despite the lack of an effect on barrier disruption after treatment with the different test compounds, we wanted to evaluate whether they could have an effect on markers of immune function. All test compounds, except for phloroglucinol, were found to significantly attenuate the NF-kB activity induced by LPS after treatment at a concentration of 100 µM. The LPS-induced secretion of IL-8, TNF-α and IL-6 was also reduced by most of the tested compounds. Overall, these anti-inflammatory effects seemed most pronounced for hesperidin, naringin, hesperetin, naringenin and the phenolics isoferulic acid and 4-hydroxyphenylacetic acid. Previous in vitro studies with various experimental setups corroborate our findings by providing evidence that citrus flavonoids and their aglycone forms exert anti-inflammatory effects, partly by inhibiting the activation of the NF-κB signalling pathway(Reference Liu, Wang and Fan30,Reference Hamalainen, Nieminen and Vuorela31,Reference Yang, Chen and Senthil Kumar32,Reference Nazari, Ghorbani and Hekmat-Doost33) . Furthermore, although these effects have not been studied extensively for all phenolic metabolites that were tested here, previous studies confirm that several of these compounds possess anti-inflammatory properties(Reference Larrosa, Luceri and Vivoli21,Reference Greten, Arkan and Bollrath34,Reference Dilshara, Lee and Jayasooriya35,Reference Nile, Ko and Kim36,Reference Lampiasi and Montana37,Reference Koshiguchi, Komazaki and Hirai38,Reference Navarrete, Alarcon and Palomo39,Reference Lampiasi and Montana40) .
In the present study, however, citrus flavonoid treatment resulted in increased levels of the cytokine IL-1β. These results were consistent among the various test compounds and unexpected, as activated NF-κB induces the transcription of cytokine genes, including IL-1β. Although the increased IL-1β levels in combination with an inhibition of NF-κB activity seem contradictory, a possible explanation for these findings might be an increased pro-IL-1β processing. In a study by Greten et al.(Reference Greten, Arkan and Bollrath34), LPS treatment of IKKβ-deficient macrophages resulted in increased IL-1β secretion, while the expression of pro-IL-1β was decreased, suggesting that the increased IL-1β levels might be due to an increase in pro-IL-1β processing that was already present in the cell. Another possible explanation may be that because the NF-κB activity was not completely inhibited by the test compounds, in contrast to the reduction in IL-8, TNF-α and IL-6 secretion, some cytokines including IL-1β may still have been up-regulated. How our findings relate to the in vivo situation is however not clear. For example, a study using a mouse model of colonic inflammation showed reduced IL-1β levels together with a decrease in other inflammation markers such as TNF-α and nuclear NF-kB translocation after oral administration of bergamot juice, although this could also have been the effect of other compounds present in the juice(Reference Impellizzeri, Bruschetta and Di Paola41).
As citrus flavonoids are metabolised by the intestinal microbiota, it is also important to take the bioactivity of the large number of different metabolites that are formed and their relative contribution into account. The observed effects on NF-κB activity and cytokine production showed some variation between the different compounds that were tested. Phloroglucinol did not significantly affect NF-κB activity or cytokine production, while the citrus extract, dihydrocaffeic acid and phloretic acid significantly inhibited NF-κB activity but not the production of all cytokines. Although it is not entirely clear why the effects on cytokine production are not in line with the NF-κB activity results for these compounds, it should be noted that a slight (non-significant) inhibition could be observed in most cases. Overall, the parent compounds, aglycone forms and the phenolic metabolites isoferulic acid and 4-hydroxyphenylacetic acid showed the most potent (anti-)inflammatory effects of which the order of magnitude was similar. This shows that the parent compounds and several of the metabolites can exert comparable effects. It should be noted however that in vivo, the exact concentrations in the lumen after oral ingestion of citrus flavonoids will most likely be different for each compound and it is possible that not all compounds are able to reach a luminal concentration of 100 µM. Furthermore, the anti-inflammatory effects found after treatment with a dose of 100 µM citrus flavonoids were not observed at a lower dose of 50 µM.
Although there is some variation in literature about the specific metabolites that are formed during intestinal metabolism of citrus flavonoids and their concentrations, evidence suggests that the highest concentrations of the aglycone forms and smaller metabolites (including isoferulic acid and 4-hydroxyphenylacetic acid) can be found in the more proximal parts of the colon, while the parent compounds are present at the highest concentrations in the small intestine(Reference Stevens, Rymenant and Grootaert42). Based on the results of the present study, it is therefore highly likely that citrus flavonoid ingestion will result in anti-inflammatory effects in the small intestine as well as the proximal colon and that especially in the colon effects in vivo will be more pronounced than in vitro as they are the result of a combination of the effects of the various compounds present in the intestinal lumen. Furthermore, similar to the in vivo situation, the parent compounds are very poorly transported across the epithelium while the aglycones and several phenolics can be converted into phase II metabolites by Caco-2 cells and are also transported to the basolateral compartment(Reference Van Rymenant, Salden and Voorspoels12,Reference Brand, van der Wel and Rein43,Reference Kobayashi and Konishi44,Reference Kobayashi, Tanabe and Sugiyama45) . As the anti-inflammatory effects of the parent compounds and the most potent metabolites are of the same order of magnitude, this is likely the result of a combination of luminal and basolateral effects. Future research assessing the absorption, metabolism and transport of these compounds by intestinal epithelial cells may contribute to further insight in the underlying mechanisms.
In the present study, we used a validated co-culture system of Caco-2/THP-1 cells as model for intestinal inflammation and cytokine-induced barrier disruption(Reference Possemiers, Pinheiro and Verhelst24,Reference Satsu, Ishimoto and Nakano25) . THP-1 cells in this model are treated with PMA to induce differentiation into activated macrophages, as was supported by the secretion of inflammatory markers, such as NF-κB-inducible SEAP, IL-8 and IL-1β. At the same time, a significant decrease in TEER values of the Caco-2 monolayer was observed, indicating that the barrier was disrupted. In order to further induce an inflammatory environment, the activated macrophages were stimulated with LPS after 24 h of co-culture, resulting in an increase in NF-kB activity and (enhanced) secretion of the pro-inflammatory cytokines IL-8, IL-1β, TNF-α and IL-6. Based on data from previous studies(Reference Kampfer, Urban and Gioria46,Reference Eckmann, Jung and Schürer-Maly47) , it is likely that the inflammatory markers measured basolaterally before and after LPS stimulation were secreted by the activated macrophages, without additional effects of the Caco-2 cells. This increased production of pro-inflammatory mediators was not associated with further disruption of the epithelial barrier, possibly because the effect of some of the cytokines was not concentration dependent and/or the maximal decrease in TEER already occurred as a result of basal cytokine release by the activated macrophages(Reference Al-Sadi and Ma48). Treatment with the citrus flavonoids and their metabolites significantly inhibited NF-kB activity and the production of IL-8, TNF-α and IL-6, but this effect did not result in an improved barrier function. For some compounds, the effects on LPS-induced and basal cytokine production may not be the same(Reference Possemiers, Pinheiro and Verhelst24,Reference Lampiasi and Montana40) and the citrus flavonoid compounds may therefore have only reduced the release of cytokine secretion stimulated by LPS without affecting basal levels. However, we cannot exclude that the lack of an effect on TEER, FITC-D4 permeation or the expression of barrier-related genes after treatment with the test compounds may be due the increased levels of IL-1β found. IL-1β can lead to barrier disruption, as shown by multiple in vitro studies using Caco-2 monolayers(Reference Al-Sadi and Ma48,Reference Al-Sadi, Ye and Said49,Reference Al-Sadi, Guo and Ye50) .
The validated co-culture model(Reference Possemiers, Pinheiro and Verhelst24,Reference Satsu, Ishimoto and Nakano25) that was used provides a better reflection of the in vivo situation than a mono-culture, as it includes crosstalk between immune cells and epithelial cells. However, many components of the in vivo situation are not represented in this model. For instance, this model does not include other immune cells nor the intestinal microbial population, which varies between subjects and is affected by, for example, disease status, medication use and dietary intake(Reference Lozupone, Stombaugh and Gordon51). The microbiota composition in particular might be important, as we know that bacteria can convert the citrus flavonoids into bioactive metabolites(Reference Pereira-Caro, Borges and Ky9,Reference Brett, Hollands and Needs10,Reference Nielsen, Chee and Poulsen11) and emerging evidence also shows that citrus flavonoids may induce alterations in the microbiota composition and activity(Reference Brasili, Hassimotto and Del Chierico52,Reference Lima, Cecatti and Fidelix53) . Therefore, the present in vitro findings warrant further research and validation in human subjects.
In conclusion, we have demonstrated that treatment with citrus flavonoid compounds did result in a significant inhibition of NF-κB activity and production of several inflammatory cytokines for both the citrus flavonoids and several of their metabolites, but did not protect against an immune-mediated barrier disruption. Overall, these results suggest that citrus flavonoids may decrease intestinal inflammation via reduction of NF-κB activity and that the parent compounds and their metabolites formed during intestinal metabolism are able to exert comparable effects.
Acknowledgements
We thank Lisa van de Ven and Monique van Erp for technical assistance.
This work was in part funded by BioActor B.V., that is, by contributing to the consumable costs and salary of Y. S. BioActor B.V. had no further role in the design, analysis or writing of this article.
Y. S.: conceptualisation, methodology, investigation, formal analysis, writing – original draft. T. D. B.: investigation. I. P.: conceptualisation, methodology, resources, writing – review and editing. M. E.: investigation. A. M.: conceptualisation, methodology, writing – review and editing, supervision. D. J.: conceptualisation, methodology, writing – review and editing, supervision.
Y. S. is an employee of BioActor B.V. D. J. is involved in research projects as part of private public partnership grants (i.e. TKI/Well on Wheat project and CCC NWO Carbokinetics) and in H2020 DISCOvERIE nr. 848228. A. M. has received a ZON MW, The Netherlands Organization for Health Research and Development, health care efficiency grant to evaluate efficacy of peppermint oil in IBS; has received an unrestricted research grant from Will Pharma SA and received research funding from Allergan and Grünenthal on IBS topics; has given scientific advice to Bayer (topic: IBS), to Kyowa Kirin (topic: constipation) and to Takeda (topic: gastroparesis); has received funding from Pentax Europe GmBH and received funding from the Dutch Cancer Society related to endoscopy and to colorectal polyps. T. D. B., I. P. and M. E. report no conflict of interest.
Supplementary material
For supplementary materials referred to in this article, please visit https://doi.org/10.1017/S0007114521004797