Introduction
Historically, the management of weeds was a time and labor-intensive process (Gianessi Reference Gianessi2013; Timmons Reference Timmons2005). Records dating back to the 16th century indicate that farmers had begun to recognize the negative impacts that weeds had on subsequent crop yields and spent large amounts of time removing these weeds through mechanical tactics (Timmons Reference Timmons2005; Zimdahl Reference Zimdahl and Zimdahl2018). The introduction of selective herbicides in the 1940s and nonselective herbicides in the 1950s enhanced crop productivity through improved weed control. However, understanding herbicide–crop selectivity still challenged weed management and limited effective options to control a wide spectrum of weeds in various cropping systems (Burnside Reference Burnside1992; Duke Reference Duke2005; Kudsk and Streibig Reference Kudsk and Streibig2003; Timmons Reference Timmons2005). When agronomic crop varieties with genes conferring tolerance to glyphosate were released in 1996 for soybean [Glycine max (L.) Merr.], and then for field corn (Zea mays L.) and cotton in 1997, this introduced the opportunity to utilize a broad-spectrum, nonselective herbicide for in-season weed control (Duke and Powles Reference Duke and Powles2008; Dill Reference Dill2005; Reddy and Norsworthy Reference Reddy, Norsworthy and Nandula2010). The effectiveness of glyphosate in controlling numerous weeds during the growing season within resistant crops rapidly increased its popularity with growers, leading to heavy reliance on the herbicide, and subsequent movement away from the use of multiple herbicidal mechanisms of action and other mechanical or cultural management practices (Green Reference Green2011, Reference Green2014; Sosnoskie and Culpepper Reference Sosnoskie and Culpepper2014; Vencill et al. Reference Vencill, Nichols, Webster, Soteres, Mallory-Smith, Burgos, Johnson and McClelland2012).
Due to the large number of individual plants treated with glyphosate, the sizeable spatial distribution of glyphosate use, and the lack of diversity in weed management programs, the identification of glyphosate-resistant weeds was documented as early as 5 yr after the introduction of resistant crops (Green Reference Green2011; Owen Reference Owen2008; VanGessel Reference VanGessel2001; Vila-Aiub et al. Reference Vila-Aiub, Vidal, Balbi, Gundel, Trucco and Ghersa2008). The postemergence-only glyphosate system, which utilized only one chemistry, was adopted by cotton, field corn, and soybean growers across the country and quickly selected for rare resistant weed biotypes (Norsworthy et al. Reference Norsworthy, Griffith, Scott, Smith and Oliver2008). This included Palmer amaranth, which contained gene mutations conferring resistance to the herbicide (Culpepper et al. Reference Culpepper, Grey, Vencill, Kichler, Webster, Brown, York, David and Hannah2006, Reference Culpepper, Whitaker, MacRae and York2008; Steckel et al. Reference Steckel, Main, Ellis and Mueller2008). As more instances of glyphosate-resistant weed species were documented throughout the country, academic and industry agricultural scientists promoted a return to applying a diverse selection of weed management tactics to control these glyphosate-resistant weeds while preventing their spread (Green Reference Green2014; Gustafson Reference Gustafson2008; Sosnoskie and Culpepper Reference Sosnoskie and Culpepper2014).
Integrated weed management (IWM) is an approach to weed control that centers around diversity of weed management practices (Buhler Reference Buhler2002; Harker and O’Donovan Reference Harker and O’Donovan2013; Liebman and Gallandt Reference Liebman, Gallandt and Jackson1997). A successful and sustainable IWM program includes utilizing multiple chemical, cultural, or mechanical methods to control weeds, with the combination of these tactics preventing excessive selection for resistance from being placed on any one management practice (Harker and O’Donovan Reference Harker and O’Donovan2013; Owen et al. Reference Owen, Beckie, Leeson, Norsworthy and Steckel2014; Swanton and Weise Reference Swanton and Weise1991). As a function of individual plant biology, characteristics of genes conferring resistance, and the number of weedy plants acted on by a control measure, selection for resistance depends not only on the product applied but also on how the biology of the individual weed species reacts to the herbicidal action (Heap and Duke Reference Heap and Duke2017; Hand et al. Reference Hand, Randell, Nichols, Steckel, Basinger and Culpepper2021; Swanton et al. Reference Swanton, Mahoney, Chandler and Gulden2008). The biological characteristics of Palmer amaranth, including its rapid growth, continuous emergence, intense rooting structure, and resilient response to varying environmental conditions, lend themselves to positively responding to selection for resistance imposed on it by herbicides through rapid plant recovery in periods of injury and stress (Culpepper et al. Reference Culpepper, Webster, Sosnoskie, York and Nandula2010; Ehleringer Reference Ehleringer, Chabot and Mooney1985; Horak and Loughin Reference Horak and Loughin2000; Ward et al. Reference Ward, Webster and Steckel2013). Paired with its recorded ability to have developed a six-way resistance to multiple herbicidal mechanisms of action to date, Palmer amaranth must not only be managed throughout the growing season to minimize potential yield losses but must also be managed effectively to ensure that the longevity of herbicide utility remains intact (Morgan et al. Reference Morgan, Baumann and Chandler2001; Shyam et al. Reference Shayam, Borgato, Peterson, Dille and Jugulam2021; Whitaker et al. Reference Whitaker, York, Jordan, Culpepper and Sosnoskie2011). Cotton varieties with genes conferring tolerance to postemergence applications of glyphosate, glufosinate, and dicamba or 2,4-D are currently commercially available and provide growers with additional options for in-season weed control. With over 75% of cotton in the United States and 99% of Georgia cotton planted to these varieties during 2020, it is clear that these varieties provide significant weed management benefits while also performing agronomically (USDA 2020). However, overuse of these products has already led to emerging resistance concerns in Palmer amaranth throughout the midsouthern United States (Heap Reference Heap2023). Without diversification in management tactics, resistance, particularly in Palmer amaranth, to postemergence-applied herbicides will continue to become widespread and challenge the economic viability and sustainability of cotton and other agronomic cropping systems.
Research has thoroughly documented the benefits that IWM tactics, such as utilizing cover crops and at-plant residual herbicides, have in reducing the selection for resistance placed on individual herbicides. For example, within the first 2 wk of planting cotton, a terminated, high-biomass cereal rye (Secale cereale L.) or wheat (Triticum aestivum L.) cover crop prevented Palmer amaranth establishment, as the mulch barrier prevented emergence and limited sunlight penetration to the soil surface—hampering germination (Palhano et al. Reference Palhano, Norsworthy and Barber2017). To achieve greater than 75% control of glyphosate-resistant Palmer amaranth in Tennessee, preemergence herbicides were utilized in combination with cereal and legume cover crop mixtures where reduced weed emergence lessened the probability of selecting for herbicide resistance to postemergence-applied herbicides (Wiggins et al. Reference Wiggins, Hayes and Steckel2016). Due to rapid growth rates and highly competitive growth characteristics, any Palmer amaranth remaining in the field following crop emergence must be managed with effective postemergence herbicide applications to minimize subsequent impacts to cotton yield (MacRae et al. Reference MacRae, Webster, Sosnoskie, Culpepper and Kichler2013; Morgan et al. Reference Morgan, Baumann and Chandler2001). Previous research conducted by Hand et al. (Reference Hand, Randell, Nichols, Steckel, Basinger and Culpepper2021) investigated the value of combining multiple IWM tactics that are practical for the grower to reduce Palmer amaranth selection for resistance in a dicamba-based cotton production system. In their work, including a cover crop, preemergence-applied herbicides, and a layby directed application with sequential postemergence dicamba applications, created a complete herbicide program that effectively reduced Palmer amaranth exposure and subsequent selection for resistance on dicamba postemergence by 98%. Like that experiment, the present research aimed to develop a 2,4-D-based cotton production system that was sustainable and practical for grower adoption and focused on an integrated approach to delaying selection for herbicide resistance to postemergence-applied herbicides. Thus, the objectives of this research were to quantify the reduction in Palmer amaranth selection for resistance to 2,4-D applied postemergence in a cotton system as influenced by utilizing combinations of cover crops, preemergence residual herbicides, timely postemergence applications, and postemergence herbicide mixtures.
Materials and Methods
Study Establishment and Experimental Design
A field experiment was conducted three times during 2020 and 2021 at the University of Georgia Ponder Research Farm in Ty Ty, GA (long: −83.656917, lat: 31.507704; elev. 109 m). Soil at the farm was a Tifton loamy sand with 85% to 87% sand, 9% to 10% silt, and 4% to 5% clay; organic matter content was 0.65%, cation exchange capacity was 4.1, and pH ranged from 6.0 to 6.2. The experimental design was a split-plot, consisting of two production systems as the whole plot and six herbicide systems as the sub-plot. Four replications were included in each experimental run.
The two production system options included (i) conservation tillage using strip tillage practices and a rolled-rye cover crop, and (ii) conventional tillage where extensive land preparation was performed prior to planting the crop. Each fall prior to planting cotton, a disc (Case IH, Racine, WI) with mounted rolling baskets was used to remove plant debris and prepare the study site. Cereal rye (cultivar ‘Wrens Abruzzi’) was established at a rate of 100 kg ha–1 in the appropriate plots using a grain drill (Great Plains Manufacturing, Salina, KS). At least 2 wk prior to planting cotton, the cereal rye was terminated with glyphosate at 1.12 kg ae ha–1 and rolled using a roller crimper (I & J Manufacturing, Gordonville, PA). At the time of termination, rye was 2 m in height. At planting, the dry-weight biomass of the cover crop ranged from 4,970 to 8,830 kg ha–1 across site-years. In the conventional system, following a spring disking, the study site was ripped and bedded (KMC Manufacturing Co., Tifton, GA), and just prior to planting, each plant bed was roto-tilled (Maletti Macchine Agricole, Modena, Italy) flat. This ensured an optimum planting surface while removing all weeds and residual plant material from the soil surface. Cotton (variety PHY500 W3FE) was planted in both the conventional and conservation tillage system simultaneously using a strip-till implement (Kelly Manufacturing Co., Tifton, GA), including ripper shanks to penetrate the hardpan, with a Monosem vacuum planter (Monosem, Largeasse, France) attached to the back. This tillage-planting combination implement reduces planting error by lining up the tillage strip and planting row. Cotton was planted using the hill-drop method, with two seeds planted every 23 cm, at a depth of 1.2 cm, and on a 91-cm row spacing; individual plots were four rows by 9 m in length. To meet crop growth needs, all irrigation, fertilization, insect, and disease management requirements were met following university recommendations for the region (Hand Reference Hand2022). Overhead irrigation was utilized to supplement rainfall for irrigation throughout the season.
The five herbicide systems included the following: (i) acetochlor (840 g ai ha–1) plus fomesafen (210 g ai ha–1) applied preemergence followed by (fb) three timely sequential postemergence applications of 2,4-D (1,065 g ae ha–1) plus glyphosate (1,260 g ae ha–1), (ii) acetochlor plus fomesafen preemergence fb three delayed sequential postemergence applications of 2,4-D plus glyphosate, (iii) three timely postemergence applications of 2,4-D plus glyphosate, (iv) three delayed sequential postemergence applications of 2,4-D plus glyphosate, and (v) no herbicide treatment. Timely postemergence applications were initiated when Palmer amaranth reached 8 to 13 cm in height, which occurred 30 d after planting (DAP) with a preemergence and 15 DAP without the preemergence. Delayed postemergence applications were applied when Palmer amaranth reached 20 to 25 cm occurring 35 DAP with a preemergence and 21 DAP without the preemergence. Sequential postemergence treatments after the initial postemergence application were applied at approximately 15-d intervals. All herbicides were applied in water using a CO2-pressurized backpack sprayer and boom equipped with TTI 110015 nozzles for preemergence applications and AIXR 11002 nozzles for postemergence applications (TeeJet ® Technologies, Glendale Heights, IL), set to deliver 140 L ha–1 at 165 kPa. Residual preemergence herbicides were applied at planting and activated with 0.6 cm of overhead irrigation within 48 h of application.
Data Collection
Crop assessments included subjective visible injury, Palmer amaranth control estimates, cotton height measurements, Palmer amaranth density counts, and cotton yield. Visible crop injury was estimated before the first postemergence application and 7 and 14 d following each postemergence application. Injury, which included stunting, necrosis, and necrotic lesions, was evaluated on a scale of 0 (no crop injury) to 100 (plant death). As injury never exceeded 10%, it is not reported herein. Cotton plant height measurements were obtained to quantify the influence of early-season weed competition on cotton growth. Cotton heights were collected weekly from 20 plants in each plot (10 plants from rows 2 and 3 from each four-row plot). At the end of the season, seed cotton yield was collected from the center two rows in each plot using a commercial spindle picker modified for small-plot harvesting.
Palmer amaranth assessments for visual control and density counts occurred 1 d before each postemergence application and bi-weekly after the final postemergence application until harvest. Visual control estimates were evaluated on a scale of 0 (no control) to 100 (complete control). When determining density, plants were counted and defined as newly emerged plants or plants surviving the previous postemergence 2,4-D application (when applicable). This process calculated the total number of Palmer amaranth plants treated with 2,4-D and the total number of plants exposed to 2,4-D two or more times. At each data collection event, the entire plot was counted. As results from both visual estimates of control and density counts were highly correlated, only density counts will be discussed.
Statistical Analysis
To determine the impact that tillage option and herbicide system had on reducing Palmer amaranth selection for resistance from 2,4-D, response variables were assessed for normality and subjected to an ANOVA using the GLIMMIX procedure in SAS Enterprise Guide 8.3 (SAS Institute, Cary, NC). Both tillage option and herbicide system were treated as fixed effects, whereas year and replication (nested within year) were treated as random effects. Interactions between treatments and site-years were evaluated and determined to be nonsignificant; therefore, all data has been combined over site-years for analysis. All data were assessed for normality and homogeneity of variance by evaluating residual plots and using Shapiro-Wilks and Bartlett’s tests, respectively in SAS. To improve these parameters, Palmer amaranth counts from all assessment timings were square-root transformed, but all means are presented in their back-transformed values for discussion. When significant differences between treatments was observed, the Tukey-Kramer least square means test was used to separate means at an alpha level of 0.05. For seed cotton yield, contrast comparisons were performed by designating the LSESTIMATE option in the GLIMMIX procedure in SAS. The probability for each contrast is provided in parentheses following the reference (Onofri et al. Reference Onofri, Carbonell, Piepho, Mortimer and Cousens2009).
Results and Discussion
Palmer Amaranth Density
In the conventional systems where no herbicide was applied, over 272,000 Palmer amaranth plants ha–1 were recorded at time of the first postemergence (Table 1). When examining the main effects of a rye cover crop, where it was terminated and rolled to create a dense mat, Palmer amaranth emergence was reduced 72% (Table 1). The benefits of weed suppression from cover crops are well documented in the literature, including their ability to create a dense biomass mat, block sunlight from the soil surface, and in some cases, prevent weed emergence through allelopathic root exudates (Palhano et al. Reference Palhano, Norsworthy and Barber2017; Price et al. Reference Price, Monks, Culpepper, Duzy, Kelton, Marshall, Steckel, Sosnoskie and Nichols2016; Price and Norsworthy Reference Price and Norsworthy2013; Webster et al. Reference Webster, Simmons, Culpepper, Grey, Bridges and Scully2016). Although the cover crop was valuable in reducing the number of Palmer amaranth being exposed to the first postemergence application, the addition of a preemergence residual herbicide mixture further reduced the number of emerged plants by >99%, regardless of tillage system (Table 1). Research conducted on farms in Alabama, Georgia, South Carolina, and Tennessee demonstrated that utilizing cover crop residues for weed suppression are most beneficial when combined with additional integrated practices (Price et al Reference Price, Monks, Culpepper, Duzy, Kelton, Marshall, Steckel, Sosnoskie and Nichols2016). Bunchek et al. (Reference Bunchek, Wallace, Curran, Mortensen, VanGessel and Scott2020) noted that when used in combination with a cover crop, preemergence herbicides significantly reduced the quantity of smooth pigweed (Amaranthus hybridus L.) populations to be exposed to postemergence herbicide applications compared with use of cover crops alone. Likewise, these results followed similar trends but also identified that approximately 196,000 fewer Palmer amaranth plants ha–1 were exposed to selection for resistance by the residual preemergence herbicide when including a cover crop (Table 1). Both the cover crop (72%) and the preemergence residual herbicide (99%) were extremely effective in reducing the selection for resistance on 2,4-D applied at the first postemergence application.
Table 1. Palmer amaranth emergence recorded 1 d prior to postemergence (POST) applications, as influenced by the interaction of tillage and preemergence (PRE) herbicides, and averaged across three site-years in TyTy, GA during 2020 and 2021

a For conservation tillage systems, a combination strip tillage and vacuum planter implement was utilized to plant cotton into a rolled-rye cover crop; conventional tillage systems utilized extensive tillage and land preparation prior to planting cotton.
b Preemergence herbicide treatment applied immediately after seeding, and included acetochlor (840 g ai ha–1) plus fomesafen (210 g ai ha–1).
c Means followed by the same letter are not significantly different according to Tukey-Kramer honest significant difference (P ≤ 0.05).
Just prior to the second postemergence application, nearly 300,000 Palmer amaranth plants ha–1 were present in the conventional tillage system that did not include a preemergence (Table 2). With the addition of a cover crop, this number was reduced to 80,750 plant ha–1. At this point in the season, the first postemergence herbicide mixture of 2,4-D plus glyphosate had been applied, either as a timely or delayed application. Although the timely total-postemergence applications reduced the number of Palmer amaranth present in each tillage system 88% to 94%, it was less effective than making the timely postemergence application following preemergence treatments (99.99%). Delayed applications when Palmer amaranth is larger than 15 cm is not advised (Anonymous 2022) but occurs on many farms due to rapid growth, equipment or labor constraints, unfavorable weather, or any combination of the aforementioned factors beyond the control of the herbicide applicator. Simulating this scenario in our research confirmed both the value of including a residual preemergence herbicide when applications are delayed, and that delayed postemergence applications will likely allow more plants to survive the postemergence treatment. For example, in the conventional system, there were 49,980 plants ha–1 being treated with the second postemergence application in the delayed total-postemergence system, compared with 17,550 plants ha–1 in the comparable timely total-postemergence system. For programs with delayed postemergence applications following a preemergence application, only 90 to 440 plants ha–1 were observed at this time.
Table 2. Palmer amaranth emergence recorded 1 d prior to postemergence 2 (POST 2) applications, as influenced by the interaction of tillage and herbicide treatment, and averaged across three site-years in TyTy, GA during 2020 and 2021

a For conservation tillage systems, a combination strip tillage and vacuum planter implement was utilized to plant cotton into a rolled-rye cover crop; conventional tillage systems utilized extensive tillage and land preparation prior to planting cotton into a bare-ground site, where no cover crop was present.
b Preemergence herbicide treatment applied immediately after seeding, and included acetochlor (840 g ai ha–1) plus fomesafen (210 g ai ha–1). Postemergence herbicides included applications of 2,4-D (1,065 g ae ha–1) plus glyphosate (1,260 g ae ha–1) applied timely to Palmer amaranth 8 to 13 cm in height or delayed to Palmer amaranth 20 to 25 cm in height.
c Means followed by the same letter are not significantly different according to Tukey-Kramer honest significant difference (P ≤ 0.05).
Considering only Palmer amaranth plants that have survived the initial postemergence and remain to be treated with a second postemergence, the greatest density of surviving plants was observed in postemergence-only systems (Table 3). In the conservation tillage system, there were 2,710 times more plants ha–1 in the timely system where no preemergence was applied, compared with the same timely program utilizing a residual preemergence herbicide mixture. In this system, when the postemergence application was delayed, the difference was even greater at 9,105 times. In the conventional system, the value of the preemergence was less, but still impactful, ranging from 152 to 367 times fewer plants ha–1 by including the preemergence. The value of the cover crop was maximized in the total postemergence delayed system where 2.4 times more plants ha–1 were observed in the conventional system.
Table 3. Palmer amaranth survival following postemergence 1 (POST 1), recorded 1 d prior to POST 2 applications, as influenced by the interaction of tillage and herbicide treatment, and averaged across three site years in TyTy, GA during 2020 and 2021

a For conservation tillage systems, a combination strip tillage and vacuum planter implement was utilized to plant cotton into a rolled-rye cover crop; conventional tillage systems utilized extensive tillage and land preparation prior to planting cotton.
b Preemergence herbicide treatment applied immediately after seeding, and included acetochlor (840 g ai ha–1) plus fomesafen (210 g ai ha–1). Postemergence herbicides included applications of 2,4-D (1,065 g ae ha–1) plus glyphosate (1,260 g ae ha–1) applied timely to Palmer amaranth 8 to 13 cm in height or delayed to Palmer amaranth 20 to 25 cm in height.
c Means followed by the same letter are not significantly different according to Tukey-Kramer honest significant difference (P ≤ 0.05).
To understand selection for resistance on an herbicide or its mode of action, it is critical to quantify the total number of plants within the population that are being exposed during the cropping season, including those plants treated once or multiple times. When calculating together each plant treated with 2,4-D in this research, immense selection for resistance occurred from postemergence-only applications. In conventional tillage systems, between 281,690 and 331,570 Palmer amaranth ha–1 were exposed to 2,4-D. Adding a cover crop significantly reduced exposure by 71% to 72%, with the greatest reduction in exposure at 99% occurring by including the preemergence residual herbicide regardless of tillage systems (Table 4).
Table 4. Total Palmer amaranth plants exposed to 2,4-D throughout the season as influenced by the interaction of tillage and herbicide treatment, and averaged across three site-years in TyTy, GA during 2020 and 2021 a
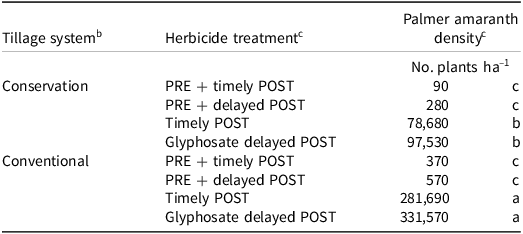
a Abbreviations: POST, postemergence; PRE, preemergence.
b For conservation tillage systems, a combination strip tillage and vacuum planter implement was utilized to plant cotton into a rolled -rye cover crop; conventional tillage systems utilized extensive tillage and land preparation before planting cotton.
c Preemergence herbicide treatment applied immediately after seeding, and included acetochlor (840 g ai ha–1) plus fomesafen (210 g ai ha–1). Postemergence herbicides included sequential applications of 2,4-D (1,065 g ae ha–1) plus glyphosate (1,260 g ae ha–1) applied timely to Palmer amaranth 8 to 13 cm in height or delayed to Palmer amaranth 20 to 25 cm in height.
c Means followed by the same letter are not significantly different according to Tukey-Kramer honest significant difference (P ≤ 0.05).
Focusing exclusively on plants treated multiple times with 2,4-D further allows a better interpretation of the potential for resistance evolution. In this work, the benefit of including the preemergence residual mixture was evident, with only 4 to 20 plants ha–1 being treated at least twice in the conservation tillage system; in the conventional system, that number ranged from 100 to 170 plants ha–1 (Table 5). These values were drastically less than those observed in the total postemergence delayed programs for the conventional (61,560 plants ha–1) or conservation (23,780 plants ha–1) system. Timely programs, although not as effective as including the preemergence mixture, were extremely impactful, with 6,580 plants ha–1 in the conservation and 16,640 plants ha–1 in the conventional system being treated at least twice.
Table 5. Total Palmer amaranth plants exposed to 2,4-D two or more times throughout the season as influenced by the interaction of tillage and herbicide treatment, and averaged across three site-years in TyTy, GA during 2020 and 2021 a

a Abbreviations: POST, postemergence; PRE, preemergence.
b For conservation tillage systems, a combination strip tillage and vacuum planter implement was utilized to plant cotton into a rolled-rye cover crop; conventional tillage systems utilized extensive tillage and land preparation prior to planting cotton.
c Preemergence herbicide treatment applied immediately after seeding, and included acetochlor (840 g ai ha–1) plus fomesafen (210 g ai ha–1). Postemergence herbicides included sequential applications of 2,4-D (1,065 g ae ha–1) plus glyphosate (1,260 g ae ha–1) applied timely to Palmer amaranth 8 to 13 cm in height or delayed to Palmer amaranth 20 to 25 cm in height.
c Means followed by the same letter are not significantly different according to Tukey-Kramer honest significant difference (P ≤ 0.05).
Cotton Height
The influence of the herbicide system on cotton height was significant, whereas the tillage system or the interaction between the two was not. When assessed 59 DAP, cotton heights were reduced by 5% to 22% in postemergence-only herbicide systems, compared with herbicide systems that included preemergence applications, while not differing from the nontreated control. Previous research by Morgan et al. (Reference Morgan, Baumann and Chandler2001) reported the effects of early-season Palmer amaranth competition on cotton canopy volume. Therefore, the reduction in crop growth observed in this research was likely due to competition from large densities of Palmer amaranth present, beginning early in the season, from postemergence-only herbicide systems.
Cotton Yield
Across site-years, seed cotton yield was significantly influenced by herbicide treatment but not by tillage system. Often, when irrigation is available in cotton, differences are not observed when comparing conventional and conservation tillage production practices. When pooled over tillage system, seed cotton yield was 2,370 kg ha–1 and maximized when preemergence herbicides were followed by timely postemergence applications (data not reported). When removing the preemergence (P < 0.0001) or delaying the postemergence application in this system (P < 0.0001), cotton yields were 5% to 16% lower when contrasting between application timings. Yields in the total postemergence-delayed program were the lowest, ranging from 2,000 to 2,080 kg ha–1, likely a result of extended early-season weed competition.
The importance of developing and implementing dynamic and diverse weed management programs in a timely fashion is highlighted by the results of this experiment. Using a cover crop, residual preemergence herbicides, and timely postemergence applications all reduced the selection for resistance placed on the postemergence herbicide application. When considering minimizing the number of Palmer amaranth treated with a postemergence application of 2,4-D in a cotton system, the preemergence herbicide mixture was the most effective option fb the cover crop fb making timely postemergence applications. However, the most effective approach is to utilize each of these tactics in the same growing season. In this study, where the cover crop, preemergence residual herbicide mixture, and timely postemergence applications were implemented, only 90 plants ha–1 (approximately 0.03% of the population) were treated with 2,4-D postemergence, and only 20 plants ha–1 (approximately 0.0006% of the population) were treated with 2,4-D more than once. Furthermore, the use of high-residue cover crops or preemergence herbicides extends the period in which Palmer amaranth can be effectively treated with postemergence herbicides due to reduced growth rates, as also observed by both Wiggins et al. (Reference Wiggins, McClure, Hayes and Steckel2015) and Montgomery et al. (Reference Montgomery, McClure, Hayes, Walker, Senseman and Steckel2018).
Practical Implications
Reducing selection for resistance placed upon herbicides, especially those applied postemergence, is critical to ensure prolonged product utility in cotton. Although varieties with resistance to an increasing number of postemergence-applied herbicides continue to be developed, the large spatial use of the respective herbicides has allowed for rapid selection of resistant weed biotypes, especially in Palmer amaranth. An integrated approach of multiple chemical, cultural, and mechanical control tactics must be utilized during the growing season to control weeds, to prevent excessive selection for resistance from being placed on any one management practice.
Previous research has thoroughly documented the benefits of adopting an IWM system to reduce weed for resistance and subsequent selection during the growing season. However, for grower adoption to occur, these systems must be economically sustainable and practical to implement. This research provided an opportunity to quantify Palmer amaranth selection for resistance within a 2,4-D-based cotton production system as influenced by including a cover crop, preemergence residual herbicides, and timely postemergence applications.
When considering the goal of minimizing the number of Palmer amaranth plants treated with a postemergence application of 2,4-D in a cotton system, the preemergence residual herbicide mixture was the most effective option, reducing Palmer amaranth exposure to 2,4-D once or more than once by over 99% fb the cover crop reducing exposure to 2,4-D once by over 72% and more than once by over 60% fb making timely postemergence applications, which reduced the number of Palmer amaranth plants being exposed to multiple applications of 2,4-D by a factor of 3.7. Although each of these methods successfully reduced exposure to the postemergence herbicide, the most effective approach was to utilize each of these tactics in the same growing season. When not including a preemergence residual herbicide or delaying postemergence applications, crop height, and yield reductions were observed as a result of early-season weed competition.
Acknowledgments
The authors wish to thank Tim Richards for his technical assistance with this research.
Funding
This research was funded by the Georgia Cotton Commission.
Competing Interests
The authors declare none.