It is commonly held that dietary fibre fermentation in monogastric animals, including humans, occurs mainly in the large intestine with little fermentation occurring in the foregut (stomach and small intestine). This, however, may not be the case(Reference Montoya, Rutherfurd and Moughan1–Reference Montoya, de Hass and Moughan6). A combined in vivo/in vitro ileal fermentation assay has been developed using the growing pig as an animal model for the adult human(Reference Montoya, de Hass and Moughan6). The assay is based on collecting ileal digesta as this part of the small intestine has a high population of microbes and is associated with a longer digesta retention time when compared with other foregut regions. In the assay, ileal digesta are collected for the preparation of an inoculum, which is used to ferment in vitro the substrate collected from the lower jejunum. The combined in vivo/in vitro fermentation assay has been applied to estimate the amount of organic matter (OM) fermented for a human-type diet in the ileum and caecum(Reference Hoogeveen, Moughan and de Haas7). There appears to be considerable ileal fermentation for a typical human diet in the growing pig, with ileal-fermented OM potentially being 1·5-fold greater than caecal-fermented OM. This work underlines the possible importance of ileal fermentation in the pig, but little is known about the ileal fermentation of different types of fibre substrates.
Dietary fibres have different monomeric units with specific links (e.g. α-1,4-linked glucose for resistant starch, β-2,1-linked fructose for fructo-oligosaccharide, FOS). These structural differences can affect their rate and extent of fermentation by the gastrointestinal (GIT) microbial population(Reference Stewart, Timm and Slavin8–Reference Barry, Hoebler and Macfarlane10). For instance, the fermentability over 24 h with a pooled human faecal inoculum did not change for cellulose, but it increased linearly for sugar beet fibre and quadratically for apple pectin(Reference Barry, Hoebler and Macfarlane10). Recently, it has been reported that one-third of purified citrus pectin was fermented in vitro with an ileal inoculum from growing pigs(Reference Montoya, de Hass and Moughan6). Due to structural differences in fibre types, it is hypothesised that ileal fermentation may differ among fibre substrates leading to a different production of organic acids. Thus, different energy contributions are expected across fibre substrates during ileal fermentation.
Differences in the rate and extent of fermentation of dietary fibres can also be influenced by the composition of the microbial population(Reference Chen, Long and Zhang11). For example, the production of acetic, propionic and butyric acids differed after in vitro fermentation of FOS, sorghum arabinoxylan and maize arabinoxylan with Prevotella spp. and Bacteroides spp. predominant faecal bacteria(Reference Chen, Long and Zhang11). Considering the differences in microbial populations between the ileum and large intestine(Reference Zoetendal, Raes and van den Bogert12,Reference Crespo-Piazuelo, Estellé and Revilla13) , the ileal fermentation of dietary fibre sources may differ from caecal fermentation. However, there are no reported studies that have determined both ileal and caecal fermentation across a range of fibre substrates.
In this study, the ileal fermentation of several fibre substrates was characterised in terms of OM fermentability and organic acid production, using an optimised in vitro ileal fermentation assay(Reference Montoya, de Hass and Moughan6) and compared with an optimised in vitro caecal fermentation assay(Reference Coles, Moughan and Awati14). For ileal and caecal inocula, ileal and caecal digesta, respectively, were collected from growing pigs fed a human-type diet. The growing pig was used as an animal model for in vitro ileal fermentability in the adult human due to similarities between species in foregut digestion(Reference Miller and Ullrey15,Reference Moughan, Cranwell, Darragh, Souffrant and Hagemeister16) . Similar viable counts of lactobacilli, streptococci and coliforms have been found in the ileal digesta of humans and pigs(Reference Graham and Åman17). Purified fibre sources commonly found in foods, and mucin (fermentable material found in GIT endogenous secretions) were used as substrates. The pig was not used as a human model for caecal fermentation due to the known anatomical differences between the species in this GIT location. The quantitative significance of ileal fermentation in the pig was determined by comparing the in vitro ileal and caecal fermentation.
Experimental methods
Animals and dietary treatment
Ethics approval for the animal trial was obtained from the Animal Ethics Committee, Massey University, Palmerston North, New Zealand. Five entire male pigs of 9 weeks of age (Hampshire × (Landrace × Large white) and 23 (sd 1·6) kg body weight) were housed individually in metabolism pens (1·5 m × 0·5 m) in a room maintained at 24 (sd 2·4)°C with a 10 h light–14 h dark cycle. The pigs were fed a high-fibre human-type diet for 15 d (Table 1). The diet was formulated to meet the nutrient requirements of the growing pig(18) as described in detail elsewhere(Reference Montoya, de Hass and Moughan6), with ingredients commonly used in Western diets.
Table 1. Ingredient and determined nutrient composition of the diet
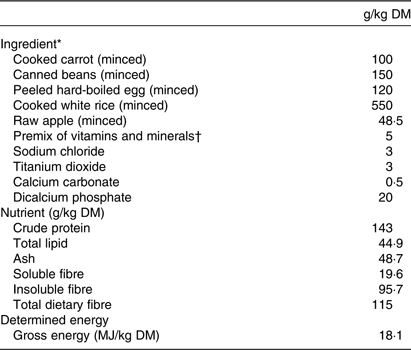
* The chemical composition of the ingredients to formulate the diet was obtained from the US Department of Agriculture National Nutrient Database (https://ndb.nal.usda.gov/).
† Vitamin and mineral premixes were obtained from Vitec Nutrition Ltd and supplied (per kg of diet as-fed): Mn, 45 mg; Zn, 80 mg; Cu, 25 mg; Co, 0·5 mg; Se, 0·3 mg; Fe, 100 mg; iodine, 1·0 mg; choline, 100 mg; all-trans retinylacetate, 3·0 mg; cholecalciferol, 0·05 mg; α-tocopherol, 50 mg; menadione, 2·0 mg; thiamin, 1·0 mg; riboflavin, 3·0 mg; nicotinic acid, 15 mg; pantothenic acid, 20 mg; pyridoxine, 2·0 mg; cyanocobalamin, 0·01 mg; folic acid, 0·5 mg; biotin, 0·1 mg.
Substrates for in vitro fermentation
Different purified substrates (arabinogalactan (AG), cellulose, FOS, inulin, mucin, citrus pectin and resistant starch) were used for both the in vitro ileal and caecal fermentations. Cellulose, pectin, AG (a model hemicellulose) and resistant starch were selected as they represent important dietary fibre components of a human diet. FOS and inulin were selected to determine if structural differences (i.e. chain length) affect OM fermentability and the production of organic acids. Mucin was selected as it is one of the major contributors to SCFA produced in the hindgut(Reference Montoya, Rutherfurd and Moughan19). All substrates, except for resistant starch, were purchased from Sigma. Resistant starch (native high-amylose maize starch) was obtained from Megazyme.
Experimental design
In vivo assay
Pigs received the human-type diet for 14 d (100 g DM/kg metabolic body weight (body weight0·75) per d), with a gradual adaptation from a commercial to the human-type diet during the first three experimental days. Two equal meals were given per day (08.00 and 16.00 hours) with ad libitum access to water. The daily ration was adjusted after 1 week. Pigs were monitored at least twice a day, and cages and toys were cleaned after each meal. On day 15, pigs were fed half their daily ration in a single meal and, at 5 h postprandially, they were anaesthetised and euthanised(Reference Montoya, de Hass and Moughan6). The small intestine and caecum were ligated with clamps and immediately removed. Digesta from the last one-third of the small intestine (ileum) and caecum for each individual pig for in vitro fermentation were collected in plastic bags containing CO2, prior to being stored in insulated containers at 37°C. Aliquots of the digesta were also collected in Eppendorf tubes and stored at –80°C for microbial analysis.
In vitro fermentation assays
In vitro ileal(Reference Montoya, de Hass and Moughan6) and caecal(Reference Coles, Moughan and Awati14) fermentation assays optimised for different parameters such as incubation time, amount of digesta and pH were used in this study. Therefore, parameters differed between in vitro ileal and caecal fermentation assays. Digesta were pooled to prepare the ileal and caecal inocula(Reference Aguirre, Ramiro-Garcia and Koenen20).
In vitro ileal fermentation assay
The inoculum was prepared under a constant flow of CO2 to maintain anaerobic conditions. Equal amounts of fresh ileal digesta from each pig were weighed. The pooled ileal digesta sample (220 g) was then mixed with 1 litre of a sterilised anaerobic 0·1 m phosphate buffer solution (plus 4·1 mm l-cysteine, pH 6·5 at 37°C), homogenised and filtered through four sterilised layers of cheesecloth. The inoculum was stirred constantly. A quantity of 5 ml of the inoculum was then added to a McCartney bottle containing 5 ml phosphate buffer either alone (blank) or containing 0·1 g substrate. Six bottles per blank (initial and final) or per substrate were used. Each bottle was flushed with CO2, capped and incubated at 37°C for 2 h(Reference Montoya, de Hass and Moughan6), with the exception of the initial blank that was not incubated. Initial blank bottles and the bottles after fermentation were cooled down on ice. Half of the bottles (blanks and substrates) were placed in an autoclave (121°C for 20 min) before determination of OM to allow calculation of OM fermentability. The other half of the bottles was thoroughly mixed, and an aliquot (1 ml) was collected in an Eppendorf tube. The Eppendorf tubes were centrifuged (14 000 g for 15 min at 4°C), and the supernatant (0·5 ml) was collected and stored at –20°C for subsequent determination of the organic acids.
In vitro caecal fermentation assay
In pigs, terminal ileal digesta are released into the caecum where fermentation with the caecal microbiota occurs. Thus, in this study, caecal digesta instead of faeces were used to prepare the caecal inoculum. The in vitro caecal fermentation assay was the same as that for the in vitro ileal fermentation assay, with the exception that the inoculum was prepared from a pooled 330 g sample of caecal digesta and the fermentation lasted for 24 h(Reference Coles, Moughan and Awati14). Two substrates (FOS and inulin) were also fermented in vitro with the caecal inoculum for 2 h.
Chemical and microbial analyses
The diet and substrates were analysed in duplicate for DM, ash and OM (DM minus ash)(21). The diet was analysed for starch (Kit AA/AMG; Megazyme), crude protein (N × 6·25; using an elemental analyser LECO), ether extract (using a Soxhlet apparatus and petroleum ether extraction), gross energy (LECO AC-350 Automatic Calorimeter), soluble and insoluble dietary fibres(Reference Prosky, Asp and Schweizer22). DM, ash and OM contents were also determined on the material remaining after the in vitro fermentation.
Organic acids (formic, acetic, propionic, butyric, isobutyric, valeric, isovaleric and lactic acids) were determined in in vitro fermentation samples using GC(Reference Richardson, Calder and Stewart23).
The ileal and caecal digesta were analysed for total bacteria using quantitative PCR and bacterial community composition using Illumina MiSeq 16S rRNA gene sequencing(Reference Blatchford, Bentley-Hewitt and Stoklosinski24).
Predicted diversity and number of glycoside hydrolases
The ileal and caecal bacterial diversity at the genus level was used to predict the number of sequences for each glycoside hydrolase family reported to have carbohydrate-active enzymes(Reference Lombard, Golaconda Ramulu and Drula25). The carbohydrate-active enzymes data set presents the activities for each family (e.g. β-glucosidase (EC 3.2.1.21), β-galactosidase (EC 3.2.1.23) and β-d-fucosidase (EC 3.2.1.38) for glycoside hydrolase family 1) for each bacterial strain.
The observations for bacterial relative abundance, total bacteria and the predicted number of glycoside hydrolases for each genus were combined to predict the diversity and number of enzymes.
Calculations
The in vitro ileal and caecal OM fermentability values and the amounts of organic acids were calculated as follows, following Montoya et al. (Reference Montoya, Rutherfurd and Moughan26):

where OMb and OMa is the OM (mg) of the substrate either before or after in vitro fermentation, respectively. OMblank initial and OMblank final are the OM in the blank bottle prior (initial) and after (final) in vitro fermentation, respectively. The amounts of organic acids produced during in vitro fermentation (mmol) and their energy contents (0·25, 0·87, 1·53, 2·18, 2·18, 2·84, 2·84 and 1·36 kJ/mmol for formic, acetic, propionic, isobutyric, butyric, isovaleric, valeric and lactic acids, respectively) were used to determine the total energy (kJ) produced from the organic acids.
The predicted number of ileal and caecal glycoside hydrolases for each family was derived considering the absolute number of bacterial 16S rRNA gene copies (quantitative PCR), bacterial community composition (Illumina) and number of glycoside hydrolases (in silico) as follows:


where glycoside hydrolasefamily i is the number of sequences in each glycoside hydrolase family for the genus j.
Statistical analysis
Based on the known means and variances for the in vitro ileal fermentation of pectin and cellulose (30 and 3·7 (sd 1·13) %), two in vitro replicates were required to detect a statistical difference between treatments with a power >80 % at a two-tail 5 % significance. In this study, three technical replicates were used.
The statistical analyses were performed using the Mixed Model procedure of SAS (SAS/STAT version 9.4). A two-way ANOVA model was used to determine the effects of inoculum (ileal v. caecal), substrate (AG, cellulose, FOS, inulin, mucin, pectin and starch) and their interaction on OM fermentability, organic acid production and energy from organic acids. When the interaction effect was not statistically significant, it was removed from the final model.
The model diagnostics for each response variable were tested using the ODS Graphics procedure and the Repeated statement of SAS. The Repeated statement in the Mixed Model procedure allowed testing for the homogeneity of variance by fitting models with the Restricted Maximum Likelihood method and comparing them using the log-likelihood ratio test. The selected model for all response variables had similar Studentised residuals (i.e. equal variances) across treatments. When the F-value of the model was significant (P ≤ 0·05), the means were compared using Least Significant Difference. Correlations were determined between OM fermentability and total energy from organic acids (n 21) for each GIT location using the Correlation procedure of SAS.
Results
All pigs remained healthy throughout the study and once adapted to their diet consumed all of their daily ration.
The microbial population of the ileal digesta at the genus level mainly comprised Streptococcus (67 %), while the caecal digesta mainly comprised Streptococcus (15 %), Ruminococcaceae (15 %), Ruminococcus (15 %), Clostridiales (11 %) and Prevotella (9 %) (online Supplementary Fig. S1).
There was an interaction (P < 0·001; Fig. 1) between the inoculum and substrate for in vitro OM fermentability. During in vitro ileal fermentation, pectin had the highest fermentability (26 %, P ≤ 0·05) followed by AG, FOS and resistant starch, while cellulose, inulin and mucin had the lowest fermentability. In contrast, during in vitro caecal fermentation, FOS, inulin, mucin, pectin and resistant starch had the highest (P ≤ 0·05) fermentation, while AG and cellulose had the lowest fermentation. The fermentation of FOS, inulin and mucin was higher (17, 26 and 24 % units, respectively; P ≤ 0·05) during in vitro caecal fermentation than during in vitro ileal fermentation, whereas the fermentation of AG, cellulose, pectin and starch was similar (P > 0·05) for in vitro ileal and caecal fermentations.

Fig. 1. In vitro ileal and caecal organic matter (OM) fermentability. The P values for inoculum, substrate and their interaction were P < 0·001, P < 0·001 and P < 0·001, respectively. Values are means with their standard errors (n 3). Means in a gastrointestinal tract location (ileum or caecal) without a common letter differ (P ≤ 0·05). * Means for the caecal data differ from the ileal counterpart (P ≤ 0·05). AG, arabinogalactan; FOS, fructo-oligosaccharide; RS, resistant starch. Ileal and caecal digesta were collected from pigs fed a high-fibre human-type diet.
To investigate the effect of incubation time during in vitro caecal fermentation, FOS and inulin were fermented in vitro with the caecal inoculum for both 2 and 24 h. The extent of in vitro caecal OM fermentability during 2 h was 16 and 14 % for FOS and inulin, respectively.
There was an interaction (P ≤ 0·05; Table 2) between inoculum and substrate for the in vitro production of acetic, propionic, butyric, isovaleric and lactic acids. For acetic acid, FOS, inulin and mucin in general had the highest (P ≤ 0·05) in vitro ileal and caecal productions, though mucin had one of the lowest in vitro caecal acetic acid productions (P ≤ 0·05). For propionic, butyric and isovaleric acids, there were no differences (P > 0·05) between substrates during in vitro ileal fermentation. However, during in vitro caecal fermentation, there were large differences (P ≤ 0·05) between substrates. For instance, the in vitro caecal production of propionic acid was 3·3-fold higher for FOS when compared with cellulose. For lactic acid, resistant starch had the highest (P ≤ 0·05) in vitro ileal and caecal productions, while FOS (ileal fermentation) and pectin (caecal fermentation) had the lowest. In general, the in vitro caecal production of acetic, propionic and butyric acids was higher (P ≤ 0·05) than the in vitro ileal counterpart. In contrast, the in vitro caecal production of isovaleric and lactic acids was in general either similar (P > 0·05) or lower (P ≤ 0·05) than the in vitro ileal counterpart.
Table 2. Organic acid production during in vitro ileal and caecal fermentation of different purified substrates. Ileal and caecal digesta were collected from pigs fed a high-fibre human-type diet
(Mean values with their standard errors)
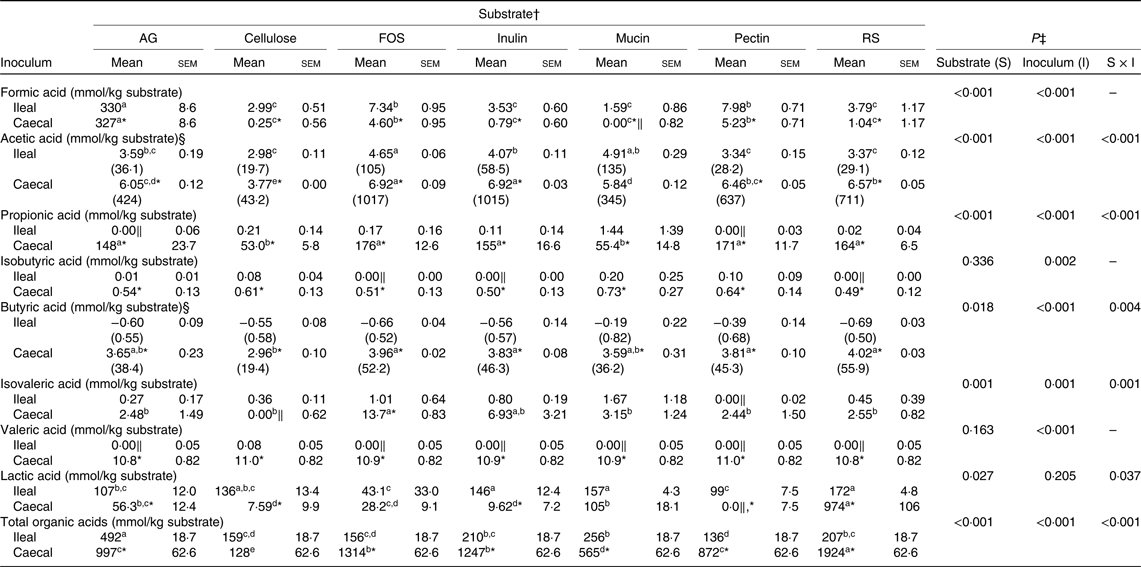
AG, arabinogalactan; FOS, fructo-oligosaccharide; RS, resistant starch.
* Means for the caecal inoculum data differ from the ileal counterpart (P ≤ 0·05).
† Values are means and standard error of the means, n 3 for each substrate and inoculum combination. Different Repeated statement for each organic acid were required to have similar Studentised residuals as described in the Statistical analysis section (e.g. for total organic acids, the best Repeated statement had a common sem for each inoculum, while for lactic acid had a standard error for each individual substrate by inoculum combination). Means within each inoculum (ileal or caecal) without a common letter differ (P ≤ 0·05).
‡ For formic, isobutyric and valeric acid production, there was no significant (P > 0·05) interaction between substrate and inoculum. Therefore, the interaction term was removed from the model.
§ A natural logarithm transformation of the raw data was required to achieve the model assumptions of normality and homoscedasticity. The values in parentheses represent the mean for each response variable after back transformation.
‖ Negative values were found. They are explained by the artifact of correcting a small number for the material found in the blanks. In these instances, values are assumed to be zero (i.e. the organic acid was not produced).
There was no interaction (P > 0·05) between inoculum and substrate for the in vitro production of formic, isobutyric and valeric acids (Table 2). The in vitro fermentation of AG rendered a very high (P < 0·001) production of formic acid (>50-fold compared with other substrates), followed by pectin and FOS. The production of formic acid was 5 % higher for in vitro ileal fermentation compared with in vitro caecal fermentation (P < 0·001). In contrast, the production of isobutyric and valeric acids was 10·3- and 8·4-fold higher for in vitro caecal fermentation in comparison with in vitro ileal fermentation (P ≤ 0·05), with no difference between substrates for their in vitro productions (P > 0·05).
The calculated gross energy of the organic acids produced during in vitro fermentation reflected the interaction between the inoculum and the substrate for OM fermentability (P < 0·001; Fig. 2). During in vitro ileal fermentation (2 h), AG, inulin, mucin and resistant starch had higher (P ≤ 0·05) energy productions (energy content of organic acid production) than FOS and pectin, while during in vitro caecal fermentation (24 h), resistant starch had the highest (P ≤ 0·05) gross energy production followed by FOS and inulin, while cellulose and mucin had the lowest. For all substrates, apart from cellulose, more energy (P ≤ 0·05) from organic acids was obtained during in vitro caecal fermentation than in vitro ileal fermentation. The gross energy production for in vitro ileal fermentation represented between 11 % (FOS and starch) and 100 % (cellulose) of the energy produced during in vitro caecal fermentation.

Fig. 2. Calculated energy from the total amounts of organic acids recovered during in vitro ileal and caecal fermentation. The P values for inoculum, substrate and their interaction were P < 0·001, P < 0·001 and P < 0·001, respectively. Values are means with their standard errors, n 3. Means in a gastrointestinal tract location (ileum or caecal) without a common letter differ (P < 0·05). * Means for the caecal data differ from the ileal counterpart (P < 0·05). AG, arabinogalactan; FOS, fructo-oligosaccharide; RS, resistant starch. Ileal and caecal digesta were collected from pigs fed a high-fibre human-type diet.
Discussion
The study aimed to characterise the in vitro ileal fermentability of several purified substrates in the growing pig, selected as an animal model for ileal fermentation in the adult human. The quantitative significance of in vitro ileal fermentation for the pig was gauged by comparison with pig in vitro caecal fermentation.
For five out of seven of the substrates investigated, in vitro ileal OM fermentability exceeded 5 %, demonstrating the potential importance of ileal fermentation. As more total OM enters the ileum compared with the caecum, the amount of fermented OM could be similar or even higher in the ileum compared with the caecum as reported elsewhere for pigs fed a human-type diet (66 % more fermented OM in the ileum compared with the caecum)(Reference Hoogeveen, Moughan and de Haas7). Although this may be the case for some substrates, the generally higher production of organic acids in the caecal fermentation observed here demonstrates the importance of hindgut fermentation.
Although optimised in vitro ileal and caecal fermentation assays were used in this study(Reference Montoya, de Hass and Moughan6,Reference Coles, Moughan and Awati14) , these methodologies do have limitations. For instance, they use fixed parameters (e.g. fermentation time) that in the animal may vary for different diets and substrates. The use of a pooled inoculum does not provide for animal to animal variation but does allow characterising the fermentability of different substrates. The use of purified substrates in the fermentation also assumes that the substrates are not fermented to some degree in anterior GIT regions. For example, in the present work, we used intact pectin for the in vitro caecal fermentation, but 26 % of pectin was fermented in vitro in the ileum. Anterior fermentation may modify the structure of the substrates, thus affecting their fermentability. Although the present results should be regarded as indicative only, the methodologies do assist in developing an understanding of the fermentation of substrates in parts of the GIT. Based on our results, the ileum appears to be a site of substantial bacterial fermentation in the growing pig.
The large difference in OM fermentability seen among substrates during in vitro ileal fermentation may be explained by several factors such as: (i) a low bacterial phylogenetic diversity in the ileum and therefore a low diversity and number of enzymes able to cleave substrate bonds. For instance, and based on the ileal and caecal bacterial populations (online Supplementary Table S1), the predicted number of glycoside hydrolase families present in the ileum and caecum was sixty-five and seventy-three, respectively, for the pig fed a human-type diet (online Supplementary Table S2). It is important however to highlight that different glycoside hydrolase families could have the same activities (e.g. lysozyme, EC 3.2.1.17, could be expressed in families 18, 19, 23, 24, 25 and 73), although it is unknown if they may have the same capacity to hydrolyse glycoside bonds; (ii) structural differences in the substrates. For instance, the main difference between FOS and inulin is their chain length (on average five and twenty-five degrees of polymerisation, respectively(Reference Rossi, Corradini and Amaretti27)). This difference may have affected their in vitro ileal OM fermentability. Previous studies have shown that short fructans disappeared earlier than long fructans when fermented with either individual Bifidobacterium strains or a faecal human inoculum(Reference Stewart, Timm and Slavin8,Reference Rossi, Corradini and Amaretti27) ; (iii) monomeric composition of the substrate and specificity of the microbial glycoside hydrolases. For instance, pectin that had a greater in vitro ileal fermentation than the other substrates (e.g. inulin) has a greater diversity of monomeric units (galacturonic acids (65 %), xylogalacturonan, apiogalacturonan, rhamnogalacturonan I and II, arabinose, galactose, rhamnose, xylose and fucose(Reference Mohnen28)) v. inulin (β(2,1) bonds of fructose with a terminal α(1→2) linked d-glucose). Based on the monomeric unit composition, the predicted number of glycoside hydrolases available to break down pectin is 4·7-fold higher than for inulin; (iv) microbial preferences towards substrates, which could be explained by differences in other microbial enzymes (e.g. glycosyltransferases) and carbohydrate-binding modules(Reference Boraston, Bolam and Gilbert29). For example, after 24 h of in vitro fermentation with faecal inocula, specific bacteria were attached only to insoluble wheat bran (e.g. Roseburia faecis), high amylose starch (e.g. Ruminococcus bromii) and a commercial porcine gastric mucin (e.g. Bifidobacterium bifidum). In contrast, other species attached to all substrates (e.g. Eubacterium rectale and Bifidobacterium longum)(Reference Leitch, Walker and Duncan30).
In this study, fermentation was also investigated by determining the production of organic acids. Acetic, formic and lactic acids were found in quantifiable amounts for in vitro ileal fermentation. However, in vitro ileal productions of the other organic acids were low. Considering that organic acids produced in the ileum are absorbed (metabolised) in the ileum(Reference Hoogeveen, Moughan and de Haas7), it could be expected that they have similar beneficial effects both locally and systemically (e.g. intestinal tissue proliferation, GIT motility) to those absorbed in the large intestine(Reference Kamath, Phillips and Zinsmeister31,Reference Roy, Kien and Bouthillier32) . The relatively high production of acetic, formic and lactic acids may be explained by the high relative abundance of the Streptococcus genus (67 % of the total 16S rRNA gene copies) in the ileal digesta(Reference Neijssel, Snoep and Teixeira de Mattos33,Reference Louis, Hold and Flint34) . For instance, streptococci can rapidly ferment available carbohydrates to lactate. This is achieved through efficient uptake and conversion of sugars using the relatively simple and energy efficient phosphotransferase systems(Reference Zoetendal, Raes and van den Bogert12). Ileal digesta did not host members of the Bacteroidetes phylum (online Supplementary Fig. S2). Bacteria in the Bacteroidetes phylum possess a range of carbohydrate enzymes (e.g. α- and β-glucosidases) that allow them to utilise substrates at a competitive advantage over other members of the gut microbial consortium, resulting in the production of acetate, succinate and especially propionate(Reference Sakamoto and Benno35). The lack of butyric acid production during in vitro ileal fermentation can also be attributed to the absence of bacterial families including Lachnospiraceae and Ruminococcaceae, which are key butyrate producers(Reference Louis, Hold and Flint34).
During in vitro ileal fermentation, the production of formic, acetic and lactic acids differed between substrates. For instance, FOS had the highest production of acetic acid, but the lowest production of lactic acid, while AG had the lowest production of acetic acid, but the highest production of formic acid. These differences could be explained by the same factors described above (e.g. chemical compositions of the fibre sources). Pectin, which had the highest in vitro OM fermentability, did not have the highest production of the analysed organic acids. Other intermediate microbial metabolites (e.g. pyruvic acid) not analysed in this study could have been produced during pectin fermentation in greater amounts before being converted to endpoint organic acids later in the fermentation (e.g. pyruvic acid can be metabolised to lactic acid)(Reference Louis, Scott and Duncan36).
In this study, pigs fed a human-type diet were used as an animal model for the adult human due to their similarities in foregut digestion. The ileal fermentation observed here may also be important for the adult human. However, more work is needed to validate the pig as a model for ileal fermentation and to elucidate the physiological significance of ileal fermentation in humans. The results reported here give encouragement for this work to be undertaken.
The differences in OM fermentability between the substrates observed for in vitro ileal fermentation were reduced during in vitro caecal fermentation. This could be ascribed to some of the factors discussed above such as a greater amount and diversity of caecal microbial enzymes. Some caecal bacteria may have different pathways to produce enzymes, which are able to degrade both dietary and non-dietary materials(Reference Sonnenburg, Xu and Leip37,Reference Sonnenburg, Zheng and Joglekar38) , which explains why mucin was also fermented during in vitro caecal fermentation. It may also be related to the longer time of fermentation. Important differences in the in vitro caecal production of formic, acetic, propionic, butyric, isovaleric and lactic acids were observed. In general, FOS had the greatest production of acetic, propionic, butyric and valeric acids, while cellulose the lowest. The low production of these organic acids for cellulose may be related to its low in vitro caecal fermentability due to the absence of cellulose-digesting enzymes in many of the GIT metagenome members, while the high value for FOS may be explained by several factors such as its high in vitro caecal fermentability and a lower number of pathways to produce organic acids as reviewed elsewhere(Reference Sarbini and Rastall39).
To demonstrate the quantitative significance of ileal fermentation in the pig itself, in vitro ileal fermentation was compared with in vitro caecal fermentation. The considerably greater in vitro caecal OM fermentability of FOS, inulin and mucin compared with their in vitro ileal counterparts in the pig could be attributed to both the longer fermentation time and greater amounts of glycoside hydrolases. For instance, in this study, the extent of caecal OM fermentability during 2 h of in vitro caecal fermentation for FOS (16 %) and inulin (14 %) was lower than that for their 24 h counterparts (33 and 32 %, respectively). This is supported by the rate of SCFA production reported elsewhere for FOS and inulin(Reference Stewart, Timm and Slavin8). For FOS, the in vitro ileal OM fermentability was comparable to the in vitro caecal OM fermentability for 2 h. In contrast for inulin, the in vitro ileal OM fermentability was still lower. This lower fermentability could be related to a lower number of inulin degrading enzymes in the ileal digesta compared with caecal digesta of the pigs fed the human-type diet. In this study, the predicted number of endo-inulinase (EC 3.2.1.7) and exo-inulinase (EC 3.2.1.80) enzymes was 2-fold higher in the caecal digesta compared with ileal digesta. Similarly, the predicted number of inulin lyases ((DFA-I-forming) (EC 4.2.2.17) and (DFA-III-forming) (EC 4.2.2.18)) in caecal digesta was 9-fold greater. For the remaining substrates (AG, cellulose, pectin and starch), there was no difference in their in vitro ileal and caecal OM fermentability. Despite similarities in OM fermentability between the ileum and caecum for AG, pectin and resistant starch, the total organic acid production and energy derived from the organic acids were higher during in vitro caecal fermentation for all substrates. A correlation (r 0·52; P ≤ 0·05; n 21) was found between OM fermentability and organic acid energy production for in vitro caecal fermentation, but not for in vitro ileal production. The lack of a correlation for in vitro ileal fermentation could be attributed to the different microbial composition. Different microbial populations may have different energy requirements and different efficiencies of fermentation(Reference Pirt and Hinshelwood40–Reference Maitra and Dill42). Based on energy and OM disappearance in the hindgut of pigs fed diets containing different levels of fibre, and on energy lost as methane and heat, assuming that they are lost in the hindgut(Reference Jørgensen, Zhao and Eggum43), 8·7 kJ of energy is expected to be produced per gram of fermented ileal digesta. This value is similar to 8·3 kJ/g dietary fibre fermented suggested by the European Commission(44). In this study, the energy derived from organic acids ranged between 0·6 and 11 kJ/g substrate fermented (in vitro) in the ileum (except for cellulose), and between 2·5 and 12·8 kJ/g substrate fermented (in vitro) in the caecum. This suggests that important amounts of energy from the microbial fermentation of fibre substrates can be produced in the ileum. Further, in some instances, organic acids other than the ones determined in this study may have contributed to the energy arising from fermentation.
In conclusion, the extent of ileal fermentation of both dietary and non-dietary substrates appears to be quantitatively significant in the growing pig adapted to a human-type diet, and such ileal fermentation may also be important in the adult human. The extent of ileal fermentation, however, depends upon the substrate. This could be attributed to factors such as structural differences of the substrates and available glycoside microbial hydrolases. The energy from fibre fermentation in the ileum is estimated to be between 0·6 and 11 kJ/g substrate fermented. Some substrates appear to be fermented to the same extent as in the caecum.
Acknowledgements
The authors acknowledge the help of Edward de Haas and Megane Cognée during the study.
This study was funded by the Centre of Research Excellence Fund from the Tertiary Education Commission and the New Zealand Ministry of Education.
C. A. M. and P. J. M. were responsible for planning the study. P. B. was responsible for the microbial analysis. C. A. M. was responsible for conducting the experiments, did the statistical analysis and prepared the first draft of the manuscript which was revised by P. J. M. and P. B. All authors read and approved the final manuscript.
The authors declare that there are no conflicts of interest.
Supplementary material
For supplementary materials referred to in this article, please visit https://doi.org/10.1017/S0007114520003542