Introduction
Small fruit and vegetable growers use polyethylene plastic mulch films (PE) for weed management, increased soil temperature, water retention and increased crop yields (Tofanelli and Wortman, Reference Tofanelli and Wortman2020). While valuable, PE must be removed from the field after use and most is landfilled or burned (Kasirajan and Ngouajio, Reference Kasirajan and Ngouajio2012). Even after removal, up to 11% (or 8.4 kg ha−1) of PE is left behind in the field (He et al., Reference He, Wang, Li and Malhi2018). The PE fragments left in the soil can persist for decades (Chamas et al., Reference Chamas, Moon, Zheng, Qiu, Tabassum, Jang, Abu-Omar, Scott and Suh2020) releasing toxins, adsorbing pollutants, and contaminating water or other organisms (Feuilloley et al., Reference Feuilloley, César, Benguigui, Grohens, Pillin, Bewa, Lefaux and Jamal2005; Ashton et al., Reference Ashton, Holmes and Turner2010; Wang et al., Reference Wang, Luo, Teng, Ma, Christie and Li2013; Briassoulis et al., Reference Briassoulis, Babou, Hiskakis and Kyrikou2015).
Biodegradable and biobased mulch films and fabrics (BDMs) are potentially sustainable alternatives to PE because they can provide similar agronomic benefits and be left in the soil to decompose after their useful life (Tofanelli and Wortman, Reference Tofanelli and Wortman2020). Commercially available BDMs include bioplastic films manufactured from starch and polyester polymer blends and paper mulch membranes. Many other experimental BDM products are in development including composite biofabrics manufactured from polylactic acid and agricultural residues (Samuelson et al., Reference Samuelson, Reid, Drijber, Jeske, Blanco-Canqui, Mamo, Kadoma and Wortman2022). Despite their promise, on-farm adoption of BDMs in lieu of PE has been limited. In a survey of Tennessee, Texas and Washington growers, premature deterioration of BDMs during the growing season and slow degradation after soil incorporation were identified as barriers to adoption (Goldberger et al., Reference Goldberger, Jones, Miles, Wallace and Inglis2015). Consistent with these grower concerns, recent studies have documented inconsistent durability of bioplastic and paper BDMs and the persistence of biofabric BDM residues in soil (Wortman et al., Reference Wortman, Kadoma and Crandall2015; Dharmalingam et al., Reference Dharmalingam, Hayes, Wadsworth and Dunlap2016; Thompson et al., Reference Thompson, Samuelson, Kadoma, Soto-Cantu, Drijber and Wortman2019).
As BDMs decompose or persist in soil, they have the potential to influence soil chemical and physical properties. Any changes to soil properties that negatively affect soil fertility and productivity could further contribute to BDM hesitancy among growers. The C/N ratio of BDMs is greater than 300 and there may be potential for soil N immobilization during BDM biodegradation (Thompson et al., Reference Thompson, Samuelson, Kadoma, Soto-Cantu, Drijber and Wortman2019). Nitrogen immobilization occurs in high C/N soil environments where microbial communities scavenge and store nitrogen within their cells, which renders it temporarily unavailable for crop uptake (Mooshammer et al., Reference Mooshammer, Wanek, Hämmerle, Fuchslueger, Hofhansl, Knoltsch, Schnecker, Takriti, Watzka, Wild, Keiblinger, Zechmeister-Boltenstern and Richter2014). This effect is commonly observed following soil incorporation of straw residues and cover crops, but less is known about how the decomposition of C-rich BDMs will influence soil nitrate availability (Reichel et al., Reference Reichel, Wei, Islam, Schmid, Wissel, Schröder, Schloter and Brüggemann2018).
Soil additions of C-rich amendments including wood chips and straw can increase soil organic matter (SOM), but the effects of soil-incorporated BDMs are unknown (Powlson et al., Reference Powlson, Riche, Coleman, Glendining and Whitmore2008; Li et al., Reference Li, Schneider, Morreale, Xie, Li and Li2018). Depending on BDM type, row spacing, and deterioration during the growing season, the mass of BDMs added to soil likely ranges from 50 (e.g., bioplastic films) to 2000 kg ha−1 (e.g., biofabric and paper membranes). For comparison, manure and compost amendments typically range from 1000 to 50,000 kg ha−1 (Wortman et al., Reference Wortman, Holmes, Miernicki, Knoche and Pittelkow2017) and cover crop biomass incorporated in soil often ranges from 2000 to 14,000 kg ha−1 (Osipitan et al., Reference Osipitan, Dille, Assefa, Radicetti, Ayeni and Knezevic2019). While BDM mass contributions are considerably lower than other amendments, potential effects on SOM are difficult to predict given the higher C/N ratio of BDMs (Thompson et al., Reference Thompson, Samuelson, Kadoma, Soto-Cantu, Drijber and Wortman2019).
Changes to soil microbial communities after incorporation of BDMs could indirectly contribute to changes in SOM and physical properties. After soil incorporation, some BDMs, including those containing polylactic acid polymers, can increase the abundance of soil fungi (Bandopadhyay et al., Reference Bandopadhyay, Martin-Closas, Pelacho and DeBruyn2018; Janczak et al., Reference Janczak, Hrynkiewicz, Znajewska and Dąbrowska2018). One study found that the recovered mass of a polylactic acid BDM increased 160% over the course of 20 months due in part to the accumulation of mycelia (Ghimire et al., Reference Ghimire, Saxton, Wszelaki, Moore and Miles2017). Increases in soil fungi, often associated with the addition of organic amendments, have been shown to improve soil aggregate stability (Bossuyt et al., Reference Bossuyt, Denef, Six, Frey, Merckx and Paustian2001). Management practices that promote aggregate stability are particularly relevant in vegetable systems because intensive tillage that is typical for seedbed preparation and weed management reduces the abundance of C-rich macroaggregates (Six et al., Reference Six, Paustian, Elliott and Combrink2000). If BDM incorporation in soil influences SOM and aggregate stability, it is also likely to influence related physical properties including soil sorptivity (initial water infiltration), aggregate tensile strength, and others (Murphy and Murphy, Reference Murphy and Murphy2015).
While polylactic acid BDMs may contribute to improved soil structure, there is some evidence that bioplastic BDMs could behave like PE films in soil and degrade soil structure until fully decomposed. Repeated incorporation of BDMs in the soil can increase the abundance of microplastics (Yu et al., Reference Yu, Griffin-LaHue, Miles, Hayes and Flury2021), but it is not clear if degrading BDM fragments and microplastics affect soil physical properties in the same way that PE microplastics do (de Souza Machado et al., Reference de Souza Machado, Lau, Till, Kloas, Lehmann, Becker and Rillig2018). Long-term accumulation of PE in soil has been shown to reduce soil organic C (Liu et al., Reference Liu, Huang, Hu, Qin, Zheng, Wang, Wang, Xu, Guo, Hu and Xu2021), and Jiang et al. (Reference Jiang, Liu, Wang, Zhou and Xin2017) reported decreased soil water content, bulk density, and saturated hydraulic conductivity, and increased porosity when PE residues were present in the soil. However, Qi et al. (Reference Qi, Beriot, Gort, Huerta Lwanga, Gooren, Yang and Geissen2020a) found that residues of PE and bioplastic BDM had no effect on aggregate stability. Gao et al. (Reference Gao, Xie and Yang2021) found that BDM residues [from a polylactic acid/polybutylene-adipate-co-terephthalate film] increased SOM in the first year after soil incorporation, but had no effect on aggregate stability, bulk density, porosity, EC or pH; however, PE residues increased bulk density and reduced porosity.
Changes in soil properties reported by Gao et al. (Reference Gao, Xie and Yang2021) influenced subsequent crop yield. The BDM residues in soil from a previous potato (Solanum tuberosum) crop (51.6 kg ha−1 at time of planting) did not affect subsequent rice (Oryza sativa) crop yield, but 30.0 kg ha−1 PE residue reduced rice yield compared to the no residue control. Similarly, Hu et al. (Reference Hu, Li, Gonçalves, Shi, Tian and Chen2020) found that PE residues greater than 300 kg ha−1 negatively impacted maize (Zea mays) root and shoot growth under field conditions, partly due to reduced water use efficiency. However, a pot study conducted by Qi et al. (Reference Qi, Yang, Pelaez, Huerta Lwanga, Beriot, Gertsen, Garbeva and Geissen2018) demonstrated that residues of a starch-based BDM reduced wheat (Triticum aestivum) growth, particularly in the first two months of growth, and the negative effects of PE were less pronounced compared to BDM. Variable effects of mulch residues on soil properties and crop productivity suggest additional research is needed to better understand the importance of mulch composition and mass, local conditions, management and subsequent crops.
The objective of this study was to evaluate the effects of two different types of BDM residue and compost on soil chemical and physical properties and vegetable crop yield across two diverse environments. Generally, we hypothesized that differences in BDM composition and residue management (incorporation in soil compared to removal) would drive differences in soil nitrogen dynamics, soil physical properties, and crop yields in the two years following the initial use of the mulch.
Materials and methods
Experimental locations and design
We conducted experiments at two climatically distinct locations in Nebraska between 2017 and 2019. Locations included the University of Nebraska-Lincoln East Campus Research Farm in Lincoln, NE (40.84 N, 96.65 W elevation = 351 m) and the Panhandle Research and Extension Center in Scottsbluff, NE (41.89 N, 103.68 W). The soil at Lincoln (LNK) is Zook silty clay loam, which is a fine, smectitic, mesic Cumulic Vertic Endoaquolls, and the climate is humid continental with mean annual precipitation of 735 mm and a mean daily temperature 10.8°C. The soil at Scottsbluff (SBF) is a Tripp very fine sandy loam, which is a coarse-silty, mixed, superactive, mesic Aridic Haplustoll, and the climate is semi-arid with mean annual precipitation of 399 mm and a mean daily temperature of 9.3°C. Relative precipitation and temperature differences observed between locations during the experiment were similar to historical trends (Fig. 1).

Fig. 1. Mean monthly air temperature (top) and cumulative precipitation (bottom) for Lincoln (LNK) and Scottsbluff (SBF) locations from Oct. 2017 through Aug. 2019 (Samuelson et al., Reference Samuelson, Reid, Drijber, Jeske, Blanco-Canqui, Mamo, Kadoma and Wortman2022).
The experiments were designed using a randomized complete block, split-split plot design with three replicate blocks at each location. The main plot was mulch type applied in 2017 for a sweet pepper (Capsicum annuum) crop. Mulches included a black starch-polyester bioplastic BDM (Mater-Bi®, Novamont S.P.A.; Shelton, CT, USA; hereafter abbreviated as STP) or a novel polylactic acid-based biofabric BDM (3M Company, St. Paul, MN, USA; hereafter abbreviated as PLA). The STP mulch was 0.015 mm thick and had an area density of 20 g m−2. The PLA mulch was comprised of two layers of spunbond polylactic acid, with a meltblown interior layer that included fine-grade wood particles (62% of total mulch mass) in the fiber matrix. The PLA mulch was 1.14 mm thick with an area density of 298 g m−2. The STP mulch is certified biodegradable in soil but is not 100% biobased and therefore cannot be incorporated in soil on certified organic farms in the U.S. (Miles et al., Reference Miles, DeVetter, Ghimire and Hayes2017). In contrast, the PLA mulch is 100% biobased and certified compostable, and was included to explore its potential as an allowable input and replacement for PE on certified organic farms (though it was later discovered that biodegradation rates in soil were unlikely to meet the required standards; Thompson et al., Reference Thompson, Samuelson, Kadoma, Soto-Cantu, Drijber and Wortman2019).
The subplot treatment in the experiment was the incorporation status of mulch, where the mulch was either incorporated in soil via a reciprocating spading machine (Celli Y70, Celli SpA; Forlì, Italy) after the initial 2017 growing season or removed from the field as a control. The experiment also included sub-subplot treatments to assess the effects of organic soil amendments (including compost, compost extracts, cover crops, and a combination of all amendments) on the degradation rate of mulch (Samuelson et al., Reference Samuelson, Reid, Drijber, Jeske, Blanco-Canqui, Mamo, Kadoma and Wortman2022), but data analyzed for this paper were limited to a comparison of compost-amended plots and control plots without compost to establish an extreme gradient of soil fertility within and between locations.
Mulch type main plots were 65.8 m long and 1.83 m wide and randomized within replicate blocks; incorporation status subplots were 32.9 m long and 1.83 wide and randomized within main plots; and compost and control sub-subplot experimental units measured 5.5 m long and 1.83 m wide and were randomized within subplots. The 1.83 m plot width included a 0.76 m raised bed top with a single crop row and half of the between row area on each side of the bed top (1.07 m). There was also a 0.3 m buffer between rows of plots which resulted in a total distance of 2.13 m between rows of crops in different sub-subplots.
Cropping systems management
In spring 2017, prior to crop planting, the STP and PLA mulches were applied with a raised bed mulch layer (RB448; Nolt's Produce Supplies, Leoloa, PA, USA) with dripline applied under the mulch for irrigation. Plots were irrigated regularly to maintain soil matric potential above 25 kPa at SBF and 75 kPa at LNK (Irmak et al., Reference Irmak, Payero, VanDeWalle, Rees, Zoubek, Martin, Kranz, Eisenhauer and Leininger2016). Sweet peppers (cv. ‘Carmen’) were started from seed in the greenhouse 8 weeks before transplanting into the field on 16 May 2017 at LNK and on 6 June 2017 at SBF. Peppers (and planting holes in the mulch) were spaced 46 cm between plants in a single row and 12 plants per sub-subplot. Fish emulsion fertilizer (2.9-3.5-0.3 NPK; OrganicGem, Advanced Marine Technologies, New Bedford, MA, USA) was applied at a rate of 0.3 g N, 0.4 g P and 0.03 g K per planting hole at planting and twice more throughout the season at the same rate. A cumulative total of 84 kg ha−1 N blood meal fertilizer (13-1-0 NPK, Earthworks Health, LLC, Norfolk, NE, USA) was added during the season. After the 2017 pepper harvest, crop residue was shredded and mulch was incorporated via tillage (incorporation plots) or removed by hand (control plots). Mulch was incorporated or removed on 29 September 2017 at LNK and on 5 October 2017 at SBF. Both mulch types were largely intact (free of major rips or tears) at the time of soil incorporation.
Compost was applied to the appropriate sub-subplots and incorporated in soil at the same time as mulch residues in fall 2017. Compost was applied a second time approximately 12 months later after crop harvest but was not incorporated in the soil. The compost used at LNK was a municipal yard waste compost and the compost used at SBF was a composted beef feedlot manure. To account for the different feedstocks, compost rate was standardized across locations by total organic nitrogen with a target application of 504 kg ha−1 N. This resulted in application rates of 57 Mg ha−1 in 2017 and 60 Mg ha−1 in 2018 (dry weight) at LNK; and 42 Mg ha−1 in 2017 and 51 Mg ha−1 in 2018 at SBF.
In preparation for planting sweet corn (Zea mays) in 2018, organic soybean meal fertilizer (7-1-2 NPK; Phyta-Grow, California Organic Fertilizers, Inc., Hanford, CA, USA) was applied at a rate of 67 kg ha−1 N to all plots. Sweet corn (cv. ‘Xtra-Tender 2171) was sown on 24 May 2018 at LNK and on 31 May 2018 at SBF. Two rows spaced 60 cm apart were planted on each bed top the length of the experiment. Weeds were managed throughout the season with hand hoes to avoid the disturbance of buried mulches. Sweet corn was harvested on 23 July 2018 at LNK and on 30 July 2018 at SBF. Total and marketable yield were measured by harvesting whole ears from all plants in a plot. After harvest, crop and weed residues were shredded on 12 September 2018 at LNK and on 21 September 2018 at SBF.
Cabbage (Brassica oleraceae var. sabauda cv. ‘Melissa’) followed sweet corn in the crop rotation. Bloodmeal fertilizer (13-1-0 NPK, Earthworks Health, LLC, Norfolk, NE, USA) was applied to all plots prior to planting at a rate of 34 kg ha−1 N and raked into the surface soil before application of a white on black PE mulch for weed management. As in sweet corn, the experimental areas were not mechanically tilled and beds were not created so as to avoid disturbing buried mulch residues. Cabbage seeds were started in the greenhouse approximately eight weeks prior to transplanting on 16 May 2019 at LNK and on 5 June 2019 at SBF. Plants were spaced 46 cm apart within rows and 12 plugs were planted in each sub-subplot. Fish emulsion fertilizer (2.9-3.5-0.3 NPK; OrganicGem, Advanced Marine Technologies, New Bedford, MA, USA) was applied after transplanting at a rate of 0.5 g N, 0.7 g P and 0.05 g K per planting hole. A B.t. insecticide (GardenSafe Spectrum Brands, Middleton, WI, USA) was sprayed weekly at a rate of 3.9 mL L−1 from 24 June through harvest at LNK and from 30 July through harvest at SBF. Cabbage was harvested on 5 August 2019 at LNK and on 19 August 2019 at SBF. Cabbage was separated as marketable (dense heads with no insect damage) or cull and then weighed.
Soil sampling and analysis
For the determination of nitrate and SOM concentrations, the soil was sampled in the spring and fall of 2018 and 2019 at approximately 6, 12, 18 and 22 months after mulch was incorporated or removed in fall 2017. Eight soil cores (1.9 cm diameter by 20 cm depth) were collected from each sub-subplot. These samples were analyzed for nitrate (KCl extraction), pH (1:1 soil:water dilution) and SOM (loss of weight on ignition) (Ward, Reference Ward2021).
Soil sorptivity was measured in all sub-subplots at both locations at 6 months after mulch incorporation and in control sub-subplots at 18 months after mulch incorporation in soil using methods detailed by Smith (Reference Smith1999). Metal rings (10.5 cm height by 9.8 cm diameter) were carefully pressed into undisturbed soil and 75 mL of water was poured into the ring along the edge to minimize soil disturbance. Four readings were taken from each sub-subplot at each time. The time required for infiltration of the 75 mL of water was recorded, and four readings were collected in each plot. From these readings, soil sorptivity was calculated as:

whereby H is the head of water in cm and t is the time to infiltration in seconds.
Soil penetration resistance was measured in all sub-subplots at both locations 6 and 18 months after mulch incorporation in soil using the force meter with an 8 mm diameter cone head. Three measurements per sub-subplot were taken to a depth of 5 cm with a speed of approximately 1 cm s−1, and readings were converted to MPa based on the basal cone area and averaged (Rakkar et al., Reference Rakkar, Blanco-Canqui, Drijber, Drewnoski, MacDonald and Klopfenstein2017).
Wet aggregate stability was determined by the wet sieving method to determine the proportion of macroaggregates (>0.25 mm diameter) and microaggregates (<0.25 mm diameter; (Nimmo and Perkins, Reference Nimmo, Perkins, Dane and Topp2002; Rakkar et al., Reference Rakkar, Blanco-Canqui, Drijber, Drewnoski, MacDonald and Klopfenstein2017). Two bulk soil samples for wet aggregate stability were collected to a depth of 5 cm from all sub-subplots at both locations at 6 months after mulch incorporation and from control sub-subplots at 18 months after mulch incorporation in soil. Air-dried soil samples were dry sieved through an 8 mm sieve and 50 ± 1 g were placed on top of a stack of sieves. The three sieves had openings of 2, 1 and 0.25 mm. Soil samples were saturated by capillary action for 10 min and then oscillated up and down 3 cm for 10 min in a water tank at 30 strokes per minute. Aggregates remaining on each sieve were collected into pre-weighed beakers, which were then oven-dried at 105°C and weighed again. For samples collected at SBF, the amount of soil aggregates was corrected for sand content (because of the sandy loam soil texture at SBF, compared to silty clay loam at LNK) (Nimmo and Perkins, Reference Nimmo, Perkins, Dane and Topp2002). Sand correction accounts for loose sand accumulated from sieving, which can cause inaccuracies in the ratio of total stable aggregates and make it difficult to distinguish differences in macroaggregates (Nimmo and Perkins, Reference Nimmo, Perkins, Dane and Topp2002). Sand and aggregates were collected from each sieve, dried and weighed, then treated with 30 mL of 0.5% (NaPO3)6 to break up aggregates. Samples were then washed in a 53-μm sieve. The remaining sand was dried at 105°C, weighed, and used to correct the original aggregate mass collected on each sieve.
Soil tensile strength was measured at 6 and 18 months after mulch incorporation in the soil in all plots with a force meter equipped with a rounded, flat head (FDX Force Ten, Wagner Instruments, Greenwich, CT, USA) following the methods of Öztaş et al. (Reference Öztaş, Canpolat and Sönmez1999) and Dexter and Kroesbergen (Reference Dexter and Kroesbergen1985). To minimize variation, 20 air-dried aggregates (collected from top 5 cm of surface) between 4.75 and 8 mm were crushed individually between a flat metal disk sitting on a metal plate and the force meter head. The maximum force required to crush each aggregate was measured and tensile strength was calculated as:

whereby F is the force required to break the aggregate (N), D is the diameter of the aggregate (m), and 0.576 is the value of the coefficient of proportionality between the applied compressive load and the inner tensile strength of the aggregate (Dexter and Kroesbergen, Reference Dexter and Kroesbergen1985).
Statistical analysis
Analysis of variance was conducted with the glimmix procedure in SAS 9.4 (SAS Institute, Cary, NC, USA) to test for fixed effects of mulch type, incorporation status, compost, and all possible two- and three-way interactions on soil properties and crop yield. Replicate block and the interaction of block and mulch type were included as random effects. Locations and sample dates were analyzed individually due to confounding and interacting effects (e.g., different sample dates across locations), and because many of the soil properties measured are strongly influenced by temporal dynamics beyond the scope of our objectives (e.g., soil nitrate abundance fluctuates depending on crop growth stage). Instead, analysis of mulch type, incorporation status, and compost at individual locations and sample times allows for a relative comparison of soil property changes independent of the expected spatial and temporal dynamics. Means separation was determined for significant effects and interactions using the Tukey's HSD test with a significance threshold of P < 0.05.
Results and discussion
Mulch residue in soil
Mulch was applied in spring 2017 at both locations at a rate of 1744 kg ha−1 for PLA and 133 kg ha−1 for STP (per ha estimate assumes 1.83 m spacing between crop rows). Based on mulch degradation estimates from Samuelson et al. (Reference Samuelson, Reid, Drijber, Jeske, Blanco-Canqui, Mamo, Kadoma and Wortman2022), the mass of PLA at LNK ranged from approximately 1302 kg ha−1 at 6 months after incorporation in soil to 580 kg ha−1 at 22 months; and mass of STP at LNK ranged from 127 kg ha−1 at 6 months and was fully degraded by 22 months. At SBF, the mass of PLA in soil ranged from 827 kg ha−1 at 6 months to 512 kg ha−1 by 22 months; and STP ranged from 132 kg ha−1 at 6 months to 64 kg ha−1 at 22 months. The mass of mulch residue added to soil was greater than Hu et al. (Reference Hu, Li, Gonçalves, Shi, Tian and Chen2020) where 51.6 kg ha−1 was added before rice production; however, residue levels added – particularly for STP – were consistent with Ghimire et al. (Reference Ghimire, Scheenstra and Miles2020) and followed similar trends in mulch degradation between 6 and 22 months. Effects of initial mulch residue levels in soil greater than 1000 kg ha−1, as in the case of PLA, have not been previously studied.
Soil chemical properties
Soil pH at LNK was affected by the interacting effects of mulch incorporation and compost at 6 months whereby the combination of compost and BDM residues incorporated in soil lowered soil pH (6.7 ± 0.2) compared to compost without BDM residues in soil (7.2 ± 0.2). However, there were no differences in soil pH between 12 and 22 months after mulch incorporation (Table 1). At SBF, soil pH was influenced by the interaction of mulch by incorporation at 12 months because incorporation of STP residues increased soil pH (8.44 ± 0.04) compared to removal (8.32 ± 0.04) (Table 2); no additional changes in pH were observed at SBF. Qi et al. (Reference Qi, Ossowicki, Yang, Huerta Lwanga, Dini-Andreote, Geissen and Garbeva2020b) reported a short-term increase in pH (6.72 to 6.90) during a two-month soil incubation of micro-fragments of a starch-polyester BDM; however, after four months, there were no differences in pH. In a similar field study, Li et al. (Reference Li, Moore-Kucera, Miles, Leonas, Lee, Corbin and Inglis2014a) did not observe any effect of BDM residues (including starch-polyester bioplastics, a polylactic acid biofabric, and a paper mulch) on soil pH between 0 and 18 months after mulch incorporation across three diverse locations throughout the U.S.
Table 1. Soil pH, organic matter (SOM) and nitrate sampled approximately 6, 12, 18 and 22 months after mulch residues (a starch-polyester bioplastic [STP] or a polylactic acid biofabric with embedded wood particles [PLA]) were removed or incorporated in soil in Lincoln, NE

Results of analysis of variance for effects of mulch (STP or PLA), incorporation (removed or incorporated), and compost for each soil property are included at the bottom of the table and significance of each factor is indicated as: ‘ns’ = not significant; * = P < 0.05; ** = P < 0.01, *** = P < 0.001.
Table 2. Soil pH, organic matter (SOM) and nitrate sampled approximately 6, 12, 18 and 22 months after mulch residues (a starch-polyester bioplastic [STP] or a polylactic acid biofabric with embedded wood particles [PLA]) were removed or incorporated in soil in Scottsbluff, NE
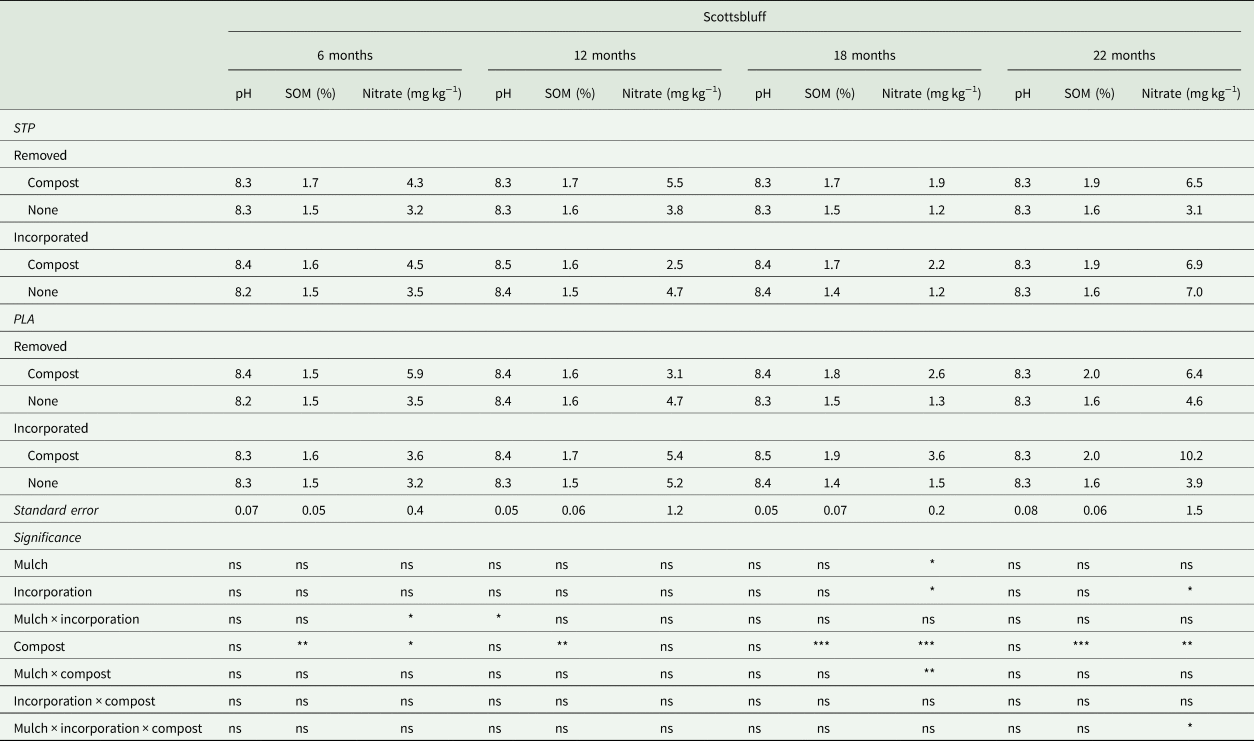
Results of analysis of variance for effects of mulch (STP or PLA), incorporation (removed or incorporated), and compost for each soil property are included at the bottom of the table and significance of each factor is indicated as: ‘ns’ = not significant; * = P < 0.05; ** = P < 0.01, *** = P < 0.001.
Biodegradation and hydrolysis of polylactic acid releases lactic acid and should acidify soil, but this hydrolysis is unlikely to occur under typical field soil conditions because the glass-transition temperature of polylactic acid is 49.2°C (Karamanlioglu and Robson, Reference Karamanlioglu and Robson2013; Hayes et al., Reference Hayes, Wadsworth, Sintim, Flury, English, Schaeffer and Saxton2017). The PLA residues in soil, excluding degraded wood fibers, were relatively stable throughout the 22 months of this experiment (Samuelson et al., Reference Samuelson, Reid, Drijber, Jeske, Blanco-Canqui, Mamo, Kadoma and Wortman2022); thus, we would not expect that polylactic acid in the PLA mulch would have driven changes in soil pH. One difference in the polylactic acid-based mulch studied here, compared to other biofabrics (e.g., Li et al., Reference Li, Moore-Kucera, Miles, Leonas, Lee, Corbin and Inglis2014a), is that PLA contained 62% wood fibers by weight. However, wood chip mulch and soil amendments do not typically alter soil pH, even as the rate of amendment or thickness of mulch increases (Greenly and Rakow, Reference Greenly and Rakow1995; Tahboub et al., Reference Tahboub, Lindemann and Murray2008). Given the minor and inconsistent changes in soil pH observed here and the unexplained connections to mulch type or incorporation, it is unlikely that biodegradation of STP or PLA residues alter soil pH enough to impact productivity in the short term.
Compost generally increased soil nitrate throughout the experiment as expected, but mulch type and incorporation also influenced soil nitrogen dynamics across both locations (Tables 1 and 2). The most notable changes in soil nitrate were observed at 6 months at SBF and at 18 to 22 months across both locations. Soil nitrate at 6 months at SBF was reduced following incorporation of PLA residues (3.4 ± 0.3 mg kg−1) compared to removal (4.7 ± 0.3 mg kg−1), but there was no difference between removal and incorporation of STP residues (e.g., no N immobilization). At 18 months, soil nitrate was greatest in the PLA × compost plots at LNK and SBF. At LNK, soil nitrate in PLA × compost plots was 10.1 ± 1.0 mg kg−1 compared to 6.3 ± 1.0 mg kg−1 in STP × compost plots and 4.3 ± 0.9 mg kg−1 in plots without compost. Similarly, soil nitrate in PLA × compost plots was 3.1 ± 0.2 mg kg−1 compared to 2.0 ± 0.2 mg kg−1 in STP × compost plots and less in plots without compost. And the trend continued at SBF at 22 months where soil nitrate was greatest in plots where PLA residues were incorporated with compost (10.2 ± 1.6 mg kg−1 compared to ≤7.1 mg kg−1 in all other treatments) (Tables 1 and 2).
Given the mass of PLA added and its high C/N, we expected short-term N immobilization following incorporation in soil. Immobilization was observed at SBF but not at LNK, and only 6 months after incorporation. This nitrogen immobilization, though modest and site-specific, contrasts with Li et al. (Reference Li, Moore-Kucera, Miles, Leonas, Lee, Corbin and Inglis2014a) where no BDM residues influenced soil nitrate between 6 and 18 months after mulch incorporation. Instead, Li et al. (Reference Li, Moore-Kucera, Miles, Leonas, Lee, Corbin and Inglis2014a) found that differences in soil nitrate were driven by location, time and production system. Nitrogen immobilization following soil amendment with C-rich organic materials (e.g., wheat straw or wood chips) is most often observed when applied at rates (2500 to 5000 kg ha−1; Döring et al., Reference Döring, Brandt, Heß, Finckh and Saucke2005) or depths (>15 cm; Hoagland et al., Reference Hoagland, Carpenter-Boggs, Granatstein, Mazzola, Smith, Peryea and Reganold2008) greater than what was added here (effective depth of 1.14 mm and mass of approximately 1744 kg ha−1 before incorporation in soil). Much of the C in polylactic acid is not bioavailable in soil due to, as previously exposed, the high glass-transition temperature (49.2°C; Hayes et al., Reference Hayes, Wadsworth, Sintim, Flury, English, Schaeffer and Saxton2017), which suggests that 38% of the 1744 kg ha−1 in the PLA mulch (approximately 663 kg ha−1; the proportion of PLA mass as polylactic acid polymer) would not contribute to N immobilization in the short term. However, the remaining addition of approximately 1081 kg ha−1 wood particles from PLA residues was apparently enough to immobilize soil nitrate in this study at SBF (lower soil fertility), but not at LNK (higher soil fertility) (Tables 1 and 2). However, repeated applications or increased wood particle loading rates in the mulch could increase the potential for immobilization in high fertility soils.
Increased soil nitrate associated with PLA and compost 18 or more months after mulch use was surprising given the carbon-rich nature of the polylactic acid and embedded wood particles. Saprophytic fungi abundance was greater on PLA mulch residues and adjacent soil (data not shown; Samuelson, Reference Samuelson2019), and perhaps the fungi contributed to accelerated decomposition and eventual mineralization of nitrogen from more recalcitrant organic materials in the compost (Masunga et al., Reference Masunga, Uzokwe, Mlay, Odeh, Singh, Buchan and De Neve2016). Moreover, saprophytic fungi have been shown to contribute to significant nitrogen immobilization in soil (Beare et al., Reference Beare, Parmelee, Hendrix, Cheng, Coleman and Crossley1992); thus, it is possible that the nitrate initially immobilized in fungal biomass after use of PLA mulch was mineralized as the PLA, wood fiber carbon source and microbial biomass declined over time.
Compost amendment increased SOM at SBF, but SOM was not affected by mulch type or incorporation at either location or any of the sampling events between 6 and 22 months after mulch incorporation or removal (P > 0.05; Tables 1 and 2). Gao et al. (Reference Gao, Xie and Yang2021) reported a small increase in SOM after incorporation of BDM in soil (1.47 mg kg−1 compared to 1.33 mg kg−1 in the no residue control), but only measured to a depth of 5 cm. SOM in this study was measured to a depth of 20 cm and given the relatively small one-time addition of organic matter, we did not expect to observe measurable changes in SOM among treatments. Li et al. (Reference Li, Moore-Kucera, Lee, Corbin, Brodhagen, Miles and Inglis2014b) reported modest variation in total organic C after BDM residue incorporation in soil at one of three locations in a high tunnel (e.g., 1.27 mg kg−1 in the control compared to 1.64 mg kg−1 18 months after the incorporation of a paper mulch in soil), but data were not analyzed for differences among mulch types over time. Though small and potentially indetectable, any short-term changes to SOM following the incorporation of BDM residues are likely to be positive.
Soil physical properties
Soil sorptivity did not differ among mulch types or incorporation treatments across years or locations (P > 0.05; Tables 3 and 4). Jiang et al. (Reference Jiang, Liu, Wang, Zhou and Xin2017) observed increased infiltration rates due to PE film residues in soil because of paths of preferential flow and macropores created by residue fragments in the soil matrix. Similarly, Sintim et al. (Reference Sintim, Bandopadhyay, English, Bary, Liquet y González, DeBruyn, Schaeffer, Miles and Flury2021) found that PE and BDM residues increased infiltration rates by 10–12% compared to a no-mulch control at one of the two locations tested. Soil sorptivity, as a measure of initial water infiltration, influences irrigation wetting patterns, and reduced sorptivity could limit the effectiveness of drip irrigation in vegetable production (Minasny and Cook, Reference Minasny, Cook, Gliński, Horabik and Lipiec2011). However, residues of STP and PLA did not negatively impact soil sorptivity in this study.
Table 3. Soil sorptivity, aggregate tensile strength, penetration resistance and macroaggregate stability sampled approximately 6 and 18 months after mulch residues (a starch-polyester bioplastic [STP] or a polylactic acid biofabric with embedded wood particles [PLA]) were removed or incorporated in soil in Lincoln, NE

Results of analysis of variance for effects of mulch (STP or PLA), incorporation (removed or incorporated), and compost for each soil property are included at the bottom of the table and significance of each factor is indicated as: ‘ns’ = not significant; * = P < 0.05; ** = P < 0.01, *** = P < 0.001.
Table 4. Soil sorptivity, aggregate tensile strength, penetration resistance and macroaggregate stability sampled approximately 6 and 18 months after mulch residues (a starch-polyester bioplastic [STP] or a polylactic acid biofabric with embedded wood particles [PLA]) were removed or incorporated in soil in Scottsbluff, NE.

Results of analysis of variance for effects of mulch (STP or PLA), incorporation (removed or incorporated), and compost for each soil property are included at the bottom of the table and significance of each factor is indicated as: ‘ns’ = not significant; * = P < 0.05; ** = P < 0.01, *** = P < 0.001.
Compost reduced soil aggregate tensile strength at 18 months after soil incorporation at LNK, but soil aggregate tensile strength was not influenced by mulch or incorporation at either location (Tables 3 and 4). Organic soil amendments, including compost and biochar, can reduce aggregate tensile strength. Increasing application rates of corn cob biochar led to proportionate reductions in tensile strength of soil aggregates between 4 and 16 mm in diameter sampled approximately six months after application (Amoakwah et al., Reference Amoakwah, Frimpong and Arthur2017). The reduced aggregate tensile strength can improve soil tilth and workability, which are important soil physical properties in vegetable production for promoting the seedling establishment and root growth. However, longer-term changes in aggregate tensile strength are less common. After four decades of straw incorporation at rates between 0 and 12 Mg ha−1, Qi et al. (Reference Qi, Jensen, Christensen and Munkholm2022) found no difference in aggregate tensile strength among treatments.
Soil penetration resistance was generally not different among mulch types or incorporation treatments across years or locations. The one exception was a mulch by incorporation interaction at SBF at 6 months, where compost had different effects on soil penetration resistance depending on mulch type (Tables 3 and 4). Mulch residues are less dense than most soil minerals and prior to decomposition can reduce bulk density and increase aeration, which may facilitate root penetration (Zhou et al., Reference Zhou, Wen, Marshall, Zhao, Gui, Yang, Zeng, Jones and Zang2021). Sintim et al. (Reference Sintim, Bandopadhyay, English, Bary, Liquet y González, DeBruyn, Schaeffer, Miles and Flury2021) found that penetration resistance near the soil surface (0 to 5 cm) decreased after four consecutive years of BDM use and residue incorporation in soil compared to the no-mulch control. However, BDM soils were not different from PE plots where the mulch was removed annually, which suggests that BDM use – not residues in soil – reduced near-surface compaction. Mulch was only used in two of three years of this study (BDM in 2017 pepper and PE in 2019 cabbage), compared to four consecutive years in Sintim et al. (Reference Sintim, Bandopadhyay, English, Bary, Liquet y González, DeBruyn, Schaeffer, Miles and Flury2021). Our results and those from Sintim et al. (Reference Sintim, Bandopadhyay, English, Bary, Liquet y González, DeBruyn, Schaeffer, Miles and Flury2021) suggest that BDM residue from a single season is unlikely to alter soil penetration resistance in a predictable, consistent way.
Macroaggregate stability was not affected by mulch type, incorporation or compost at either location (Tables 3 and 4). We had expected that greater fungal abundance and glomalin on and around PLA residues would contribute to improved soil aggregation, but that was not observed (Carrizo et al., Reference Carrizo, Alesso, Soares Franco, Bernabé Ferreira and Imhoff2018; Samuelson, Reference Samuelson2019). Sintim et al. (Reference Sintim, Bandopadhyay, English, Bary, Liquet y González, DeBruyn, Schaeffer, Miles and Flury2021) reported more consistently positive effects of BDMs on aggregate stability as annual use and incorporation of BDM residues increased aggregate stability by up to 16% compared to the no mulch control. However, as was the case with penetration resistance, aggregate stability was not different between BDM treatments and PE film (which was removed from the field at the end of each season). This suggests that improvements in aggregate stability observed by Sintim et al. (Reference Sintim, Bandopadhyay, English, Bary, Liquet y González, DeBruyn, Schaeffer, Miles and Flury2021) were related to the use of the BDM or PE during the growing season, not the result of BDM residue biodegradation in soil. The lack of residue effects on aggregate stability is consistent with two other recent studies where BDM and PE residues in soil did not affect aggregate stability (Qi et al., Reference Qi, Beriot, Gort, Huerta Lwanga, Gooren, Yang and Geissen2020a; Gao et al., Reference Gao, Xie and Yang2021).
Crop yields
Sweet corn yield at SBF in the first season following mulch use was influenced by the interacting effects of compost and incorporation (Table 5). In the absence of compost, mulch residue incorporation reduced yield by 16% ± 5% compared to removal; however, there was no difference between incorporation and removal when the soil was amended with compost (Fig. 2). Sweet corn yield at LNK was not different among treatments. Cabbage yield in 2019 was improved by compost at LNK, not SBF, but mulch type and incorporation had no effects on yield at either location (Table 5).

Fig. 2. Marketable sweet corn yield (kg plot−1) at Scottsbluff, NE in 2018 as influenced by mulch fate in the previous season (removal or incorporation in soil) and compost soil amendment. Error bars represent ± one standard error of the means and different letters above columns indicate significant differences among treatments (α = 0.05).
Table 5. Marketable yield of sweet corn (2018) and cabbage (2019) harvested at Lincoln or Scottsbluff, NE after mulch residues (a starch-polyester bioplastic [STP] or a polylactic acid biofabric with embedded wood particles [PLA]) were removed or incorporated in soil in 2017.

Results of analysis of variance for effects of mulch (STP or PLA), incorporation (removed or incorporated), and compost for each soil property are included at the bottom of the table and significance of each factor is indicated as: ‘ns’ = not significant; * = P < 0.05; ** = P < 0.01, *** = P < 0.001.
The soil nitrate results at SBF at 6 months after mulch incorporation provide the most likely explanation for the sweet corn yield differences in 2018 at SBF. Mulch incorporation, particularly PLA residues, immobilized soil nitrate at SBF due to the large mass of carbon-rich substrate added to an already low-nitrogen soil (Table 2). However, the addition of nitrogen from the compost soil amendment seemed to alleviate the nitrogen-limiting conditions caused by the incorporation of mulch residues. The lack of negative yield effects from BDM residues on cabbage in 2019 at SBF suggests nitrate was no longer limiting in residue incorporated plots, which is consistent with the data (Table 2).
Results from some greenhouse-scale, pot studies suggest that BDM residues in the soil can inhibit crop root growth and yield (Qi et al., Reference Qi, Yang, Pelaez, Huerta Lwanga, Beriot, Gertsen, Garbeva and Geissen2018; Samuelson et al., Reference Samuelson, Drijber and Wortman2019), but these effects are less commonly observed under field conditions (Hu et al., Reference Hu, Li, Gonçalves, Shi, Tian and Chen2020). Residues of PE, which are more persistent than most BDMs, are more likely to drive reductions in crop yields (Gao et al., Reference Gao, Xie and Yang2021). While polylactic acid is persistent in soil like PE, the effects of polylactic acid on soil chemical and physical properties are different, which in turn seems to influence crop yield differently. Any observed yield reductions from PE or BDM residue are often attributed to reduced soil quality, including reduced porosity and water use efficiency (Hu et al., Reference Hu, Li, Gonçalves, Shi, Tian and Chen2020; Gao et al., Reference Gao, Xie and Yang2021). However, the only yield reduction detected in this study was likely driven by nitrogen immobilization. While similar research was designed to study the impacts of repeated mulch use and soil incorporation on soil properties and crop yield (Moore and Wszelaki, Reference Moore and Wszelaki2019; Ghimire et al., Reference Ghimire, Scheenstra and Miles2020; Sintim et al., Reference Sintim, Bandopadhyay, English, Bary, Liquet y González, DeBruyn, Schaeffer, Miles and Flury2021), the unique value of this study is that management of the subsequent crops was identical within locations and years (i.e., no continued or additional treatments were imposed and tested in the original experimental area). Thus, estimates of yield were not confounded by continued use of the mulches and differences (or lack thereof) can be attributed to the BDM residues alone.
The BDM residues in this study had relatively few impacts on soil chemical or physical properties for two years following incorporation in soil, which is consistent with Sintim et al. (Reference Sintim, Bandopadhyay, English, Bary, Liquet y González, DeBruyn, Schaeffer, Miles and Flury2021). However, yield loss in a low fertility soil environment (i.e., SBF, no compost) in the season immediately following incorporation of BDM residue in soil (regardless of mulch type) should inform future BDM residue management. Specifically, growers should manage BDM residues like other carbon-rich crop residues and supplement with nitrogen fertilizer to mitigate potentially negative effects of nitrogen immobilization (Gao et al., Reference Gao, Li, Zhang, Liu, Dang, Cao and Qiang2009).
Overall, we would not recommend the annual incorporation of the PLA mulch in soil. The potential for soil nitrogen immobilization and reduced yield combined with the high initial mass (1744 kg ha−1) and persistence in soil (29 to 33% initial mass remaining after 22 months in soil; Samuelson et al., Reference Samuelson, Reid, Drijber, Jeske, Blanco-Canqui, Mamo, Kadoma and Wortman2022) will lead to the accumulation of residues at unsustainable rates that could eventually degrade soil productivity and disrupt agronomic logistics like planting. In contrast, the STP mulch degrades more readily in soil and is unlikely to accumulate at unsustainable rates (Samuelson et al., Reference Samuelson, Reid, Drijber, Jeske, Blanco-Canqui, Mamo, Kadoma and Wortman2022; Sintim et al., Reference Sintim, Bandopadhyay, English, Bary, Liquet y González, DeBruyn, Schaeffer, Miles and Flury2021); thus, we recommend the use and incorporation of STP residues except in particularly low fertility soils. The soil in this study was not mechanically disturbed again following the incorporation of BDM residues in 2017, whereas most vegetable growers (especially those using PE and BDM) will till soils at least one time per year in preparation for planting. Tillage may facilitate greater mechanical shredding and biodegradation (Ghimire et al., Reference Ghimire, Scheenstra and Miles2020) of STP residues than observed here (Samuelson et al., Reference Samuelson, Reid, Drijber, Jeske, Blanco-Canqui, Mamo, Kadoma and Wortman2022); and faster degradation may help to mitigate any potentially negative effects of BDM residues on soil nutrient availability or yield.
While not suitable for biodegradation in soil, the experimental PLA mulch studied here has promising potential for weed suppression in high-density plantings of carrots (Daucus carota subsp. sativus), spinach (Spinacia oleracea), lettuce (Lactuca sativa) and strawberry (Fragaria × ananassa) (Tofanelli et al., Reference Tofanelli, Kadoma and Wortman2021) and in establishing fruit tree orchards. In these production scenarios, PLA could likely be used for at least two growing seasons (personal observations) and then removed from the field and composted at temperatures greater than 60°C and adequate moisture (Chamas et al., Reference Chamas, Moon, Zheng, Qiu, Tabassum, Jang, Abu-Omar, Scott and Suh2020). As with any agricultural mulch, complete removal from the field is impractical, and some residues will remain. However, the results of this study, where the entire mulch was incorporated in soil, suggest that any smaller proportion of PLA residues left after field removal (approximately 11%; He et al., Reference He, Wang, Li and Malhi2018) are unlikely to have deleterious effects on soil quality or subsequent crop yield.
While PLA is persistent in soil, Thompson et al. (Reference Thompson, Samuelson, Kadoma, Soto-Cantu, Drijber and Wortman2019) demonstrated depolymerization and reduced molecular weight of polylactic acid in soil over time, even as the mass loss of mulch slowed. Moreover, depolymerization was accelerated by the addition of plant residues in the mulch matrix like the wood particles included in the PLA mulch of this study. The PLA residues will not degrade fast enough to be certified as biodegradable in soil but are unlikely to persist as long as most PE residues (Chamas et al., Reference Chamas, Moon, Zheng, Qiu, Tabassum, Jang, Abu-Omar, Scott and Suh2020). However, further research is needed to determine the long-term fate of PLA residues in soil and their impacts on soil health, particularly when repeated applications are made in the same field where the accumulation of residues beyond the levels studied here is possible.
Conclusions
Biodegradable and biobased mulches are a potentially sustainable alternative to PE because they can be incorporated into the soil or removed and composted at the end of their useful life. While biodegradation in soil or at a commercial composting facility is a preferred environmental outcome compared to burning or landfilling PE, the results of this study suggest that incorporating BDM residues in soil carries some short-term risk to soil productivity. In the season immediately following initial BDM use, sweet corn yield was reduced by 16% when BDM residues were incorporated instead of removed from the soil. However, this negative effect was only observed at SBF in the absence of compost soil amendment where SOM (1.5%) and pre-plant soil nitrate (3.4 mg kg−1) were relatively low. Cabbage yields were not different in the second year following BDM use at SBF and neither sweet corn nor cabbage yields were affected by mulch use or incorporation at LNK. Incorporation of BDM residues may immobilize nitrogen in the short-term in low fertility soils, but no additional negative effects of BDM residues on soil chemical and physical properties were observed. Results contribute to a growing body of evidence that together suggests biobased and biodegradable mulch residue can be safely incorporated in the soil so long as growers take steps to minimize the potential for nitrogen immobilization.
Financial support
This research was supported by the Nebraska Agricultural Experiment Station with funding from the Hatch Act (accession 1014303) through the USDA National Institute of Food and Agriculture (NIFA), and the USDA NIFA Organic Transitions Program (award 2016-51106-25711).
Conflict of interest
Author I. Kadoma is employed at 3M Company. There are no other competing interests to declare.