Introduction
The term “gut microbiota” refers to the whole population of microbes that live in the human gut and includes bacteria, fungi, archaea, protozoans and viruses (Sekirov et al., Reference Sekirov, Russell, Antunes and Finlay2010). Humans’ bacterial microbiota has been thoroughly studied, but research on the other kingdoms is still in its early stages. An overview of the diverse human gut microbiota’s constituents is shown in Figure 1 along with its predominant members. The gut has a large supply of micronutrients, a wide pH range and access to oxygen, hydrogen and methane, hence making it a preferred location for microbial colonisation and a suitable niche for horizontal gene transfer (HGT) (Kurokawa et al., Reference Kurokawa, Itoh, Kuwahara, Oshima, Toh, Toyoda, Takami, Morita, Sharma, Srivastava, Taylor, Noguchi, Mori, Ogura, Ehrlich, Itoh, Takagi, Sakaki, Hayashi and Hattori2007). Over the past 10 years, a number of studies have highlighted the interactions between bacteria and their hosts. Within the human gut, bacteria can be either commensal, symbiotic, pathobiont or pathogenic (Matijašić et al., Reference Matijašić, Meštrović, Paljetak, Perić, Barešić and Verbanac2020). The gut commensals play important roles in vitamin production, short-chain fatty acids (SCFA) synthesis, barrier function regulation, immunomodulation and many more, thus supporting the body’s homeostasis (Valdes et al., Reference Valdes, Walter, Segal and Spector2018). They are known to inhibit the growth of pathogenic bacteria through a process known as “colonisation resistance,” either by producing metabolites that inhibit the pathogen growth or by regulating the host immune system. However, there are also a few reports that suggest commensals may aid pathogen’s colonisation by secreting nutrients that feed these bacteria, allowing them to eventually outcompete the commensals and cause disease (Rolhion and Chassaing, Reference Rolhion and Chassaing2016). Additionally, previous research has shown that commensals produce small compounds from the host mucin layer that modulate the virulence of enterohaemorrhagic Escherichia coli (Jubelin et al., Reference Jubelin, Desvaux, Schüller, Etienne-Mesmin, Muniesa and Blanquet-Diot2018). It has been well reported that pathogenic microbes have the ability to exchange genes with the non-pathogenic residents of the gut (Messerer et al., Reference Messerer, Fischer and Schubert2017). To comprehend how infections evolve in the gut, one must have a thorough understanding of the ecology and genetic characteristics of the many bacteria that predominate in the human gut. The development of methods and technologies over the past 20 years, particularly the introduction of next-generation sequencing, has improved our capacity to comprehend and examine the contributions of the microbiota members and their roles in human health.
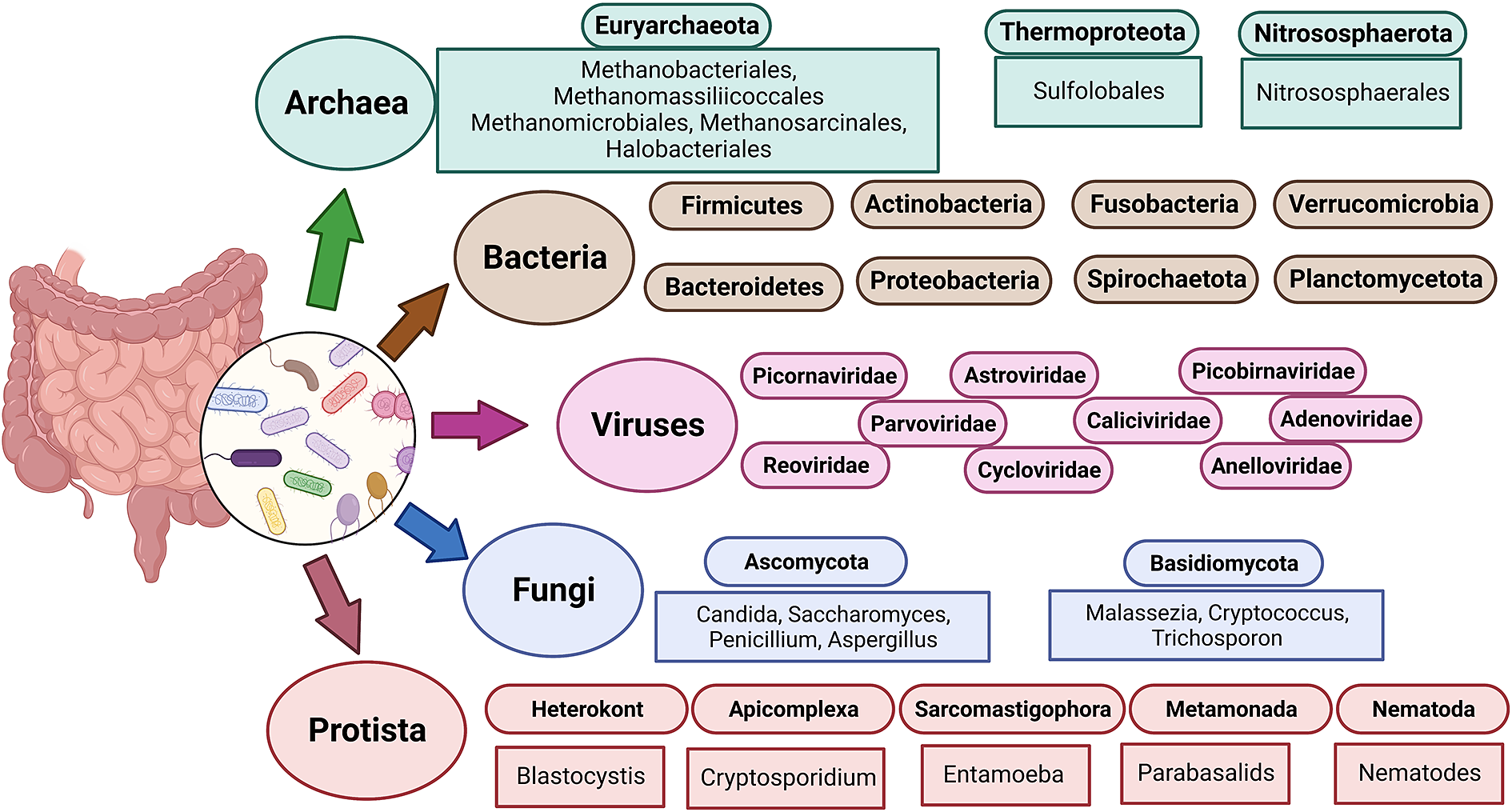
Figure 1 The diverse human gut microbiota. Population of Archaea, Bacteria, Fungi and Protists are part of the complex ecosystem of the human gut microbiota. The graphic shows the diverse compositions that dominate in different domains of life.
A bacterial genome is divided into two broad categories, such as the core and the accessory genome. The accessory genome is a growing gene pool with non-essential capabilities that provide an advantage for survival in a given niche, whereas the core genome encodes proteins that are required for metabolic functions (Rankin et al., Reference Rankin, Rocha and Brown2011). Antibiotic-resistant (Acman et al., Reference Acman, Wang, van Dorp, Shaw, Wang, Luhmann, Yin, Sun, Chen, Wang and Balloux2022) and virulence genes (Juhas et al., Reference Juhas2015) are among the gene pools found in the accessory genome, and they are mostly spread by horizontal transfer processes (Brito et al., Reference Brito, Yilmaz, Huang, Xu, Jupiter, Jenkins and Alm2016), that is, transduction, conjugation, natural transformation and fusion of outer membrane vesicles (Figure 2). Compared to the core genome, the exogenic DNA is easily distinguishable due to its unique G + C composition and specific insertion sites (Ochman et al., Reference Ochman, Lawrence and Groisman2000). Mobile genetic elements (MGEs) are one of the major facilitators of HGT (Gyles and Boerlin, Reference Gyles and Boerlin2013). MGEs include prophages, composite transposons, pathogenic islands, phages, integrative conjugative elements (ICEs), plasmids, etc. (Davis and Waldor, Reference Davis and Waldor2002). The transfer of MGEs within the gut results in the transmission of not only antibiotic resistance genes (ARGs) but also genes that code for metabolic competences such as bile salt detoxification, polysaccharide utilisation and mucus degradation (Broaders et al., Reference Broaders, Gahan and Marchesi2013). The richness and diversity of these MGEs in the human gut make it difficult to fully understand their ecological and biological identities. Many published articles have already established that the MGEs are acquired via HGT and linked with antibiotic resistance coding genes (Kent et al., Reference Kent, Vill, Shi, Satlin and Brito2020; von Wintersdorff et al., Reference von Wintersdorff, Penders, van Niekerk, Mills, Majumder, van Alphen, Savelkoul and Wolffs2016; Wang et al., Reference Wang, Luhmann, Yin, Sun, Chen, Wang and Balloux2022), but the MGEs linked to virulence genes are less highlighted (Partridge et al., Reference Partridge, Kwong, Firth and Jensen2018).

Figure 2 The mechanisms of gene exchange in human gut microbiota. The known mechanisms for mediating horizontal gene transfer (HGT) include transformation, transduction, conjugation and the fusion of outer membrane vesicles. Antibiotic resistance genes, virulence and pathogenicity determinants are transmitted by various mobile genetic elements (MGEs) through HGT. The widespread HGT in the human gut microbiome has a significant impact on both health and disease.
Numerous bacterial chromosomes and mobile genetic components have toxin–antitoxin (TA) systems (Peltier et al., Reference Peltier, Hamiot, Garneau, Boudry, Maikova, Fortier, Dupuy and Soutourina2020; Schmidt and Hensel, Reference Schmidt and Hensel2004; Weaver et al., Reference Weaver, Chen, Miiller, Johnson, Dangler, Manias, Clem, Schjodt and Dunny2017). Commensals usually lack virulence features, and they actively produce substances that promote stable interactions with other bacteria that prevent their entry into potentially harmful pathways. Changes in ecologies that create new habitats or the transmission of virulence genes from pathogens lead to the transformation of commensal to pathogenic. Acquisition of toxins or genes linked to disease, such as pathogenicity islands, are examples of mechanisms that contribute to the transformation of commensals into pathogens and the destabilisation of the commensal/host interaction (Gilmore et al., Reference Gilmore, Lebreton and van Schaik2013). Alternatively, loss of commensal functions can lead to virulence, as appears to have happened in the cases of Yersinia pestis (Chain et al., Reference Chain, Carniel, Larimer, Lamerdin, Stoutland, Regala, Georgescu, Vergez, Land, Motin, Brubaker, Fowler, Hinnebusch, Marceau, Medigue, Simonet, Chenal-Francisque, Souza, Dacheux, Elliott and Garcia2004) and Bordetella pertussis (Parkhill et al., Reference Parkhill, Sebaihia, Preston, Murphy, Thomson, Harris, Holden, Churcher, Bentley, Mungall, Cerdeño-Tárraga, Temple, James, Harris, Quail, Achtman, Atkin, Baker, Basham, Bason and Maskell2003). The present review summarises the different MGEs present in the genomes of enteric pathogens and other commensal bacteria present in the gut and their roles in toxin production, pathogenesis and disease development. This comprehensive review sheds light on the role of MGEs in shaping the ecology and evolution of the gut microbiome and how they result in community adaptations to the gut environment.
The dynamic human gut microbiome
Human gut is an abode to a complex and dynamic microbial community. A wide range of variables, including the delivery method at birth (Reyman et al., Reference Reyman, van Houten, van Baarle, Bosch, Man, Chu, Arp, Watson, Sanders, Fuentes and Bogaert2019), diet (David et al., Reference David, Maurice, Carmody, Gootenberg, Button, Wolfe, Ling, Devlin, Varma, Fischbach, Biddinger, Dutton and Turnbaugh2014; Muegge et al., Reference Muegge, Kuczynski, Knights, Clemente, González, Fontana, Henrissat, Knight and Gordon2011), lifestyle and host genetics (Qin et al., Reference Qin, Havulinna, Liu, Jousilahti, Ritchie, Tokolyi, Sanders, Valsta, Brożyńska, Zhu, Tripathi, Vázquez-Baeza, Loomba, Cheng, Jain, Niiranen, Lahti, Knight, Salomaa, Inouye and Méric2022) influence the composition of the gut microbiota. Evolutionary dynamics like mutation, HGT, drift and selection, as well as ecological factors like changes in species abundance or strain replacements, influence the gut microbiome (Garud and Pollard Reference Garud and Pollard2020). However, even today, a major gap exists in our knowledge of the global microbiome variability. It has been established that industrialisation, westernisation and the rural–urban divide within a nation are the main causes of this heterogeneity (De Filipo et al., 2017). Environmental factors, genetics, food, illnesses and antibiotic exposure all play a significant role in determining the diversity and composition of microorganisms in various body locations. The diverse range of factors that can affect gut homeostasis and microbial diversity is depicted in Figure 3.
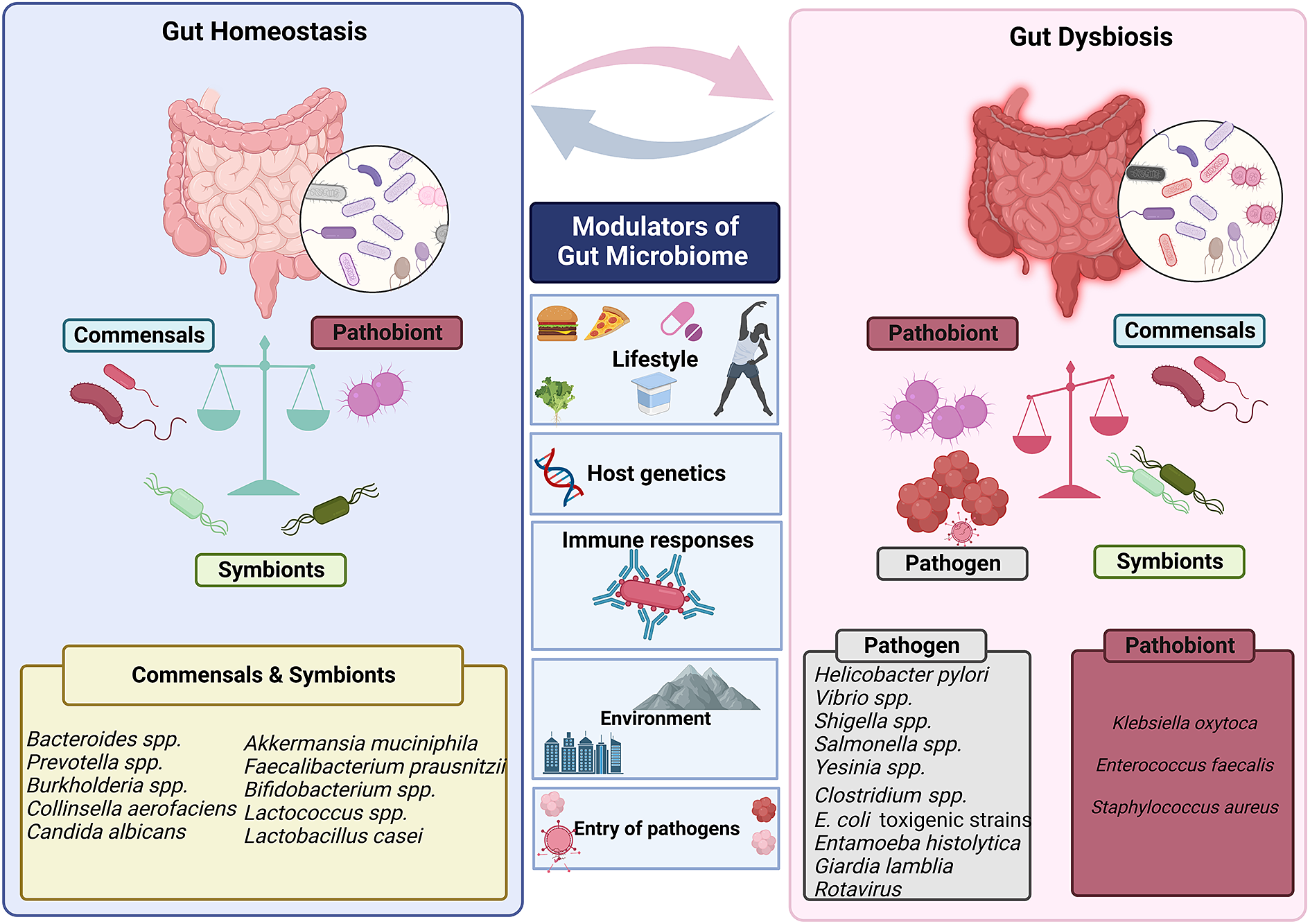
Figure 3 Factors that modulate gut’s microbial ecosystem. Numerous variables including lifestyle, age, genetics of the host, environment, pathogen infiltration, immune responses and so forth result in dynamic changes that may put the gut microbiota in a dysbiotic state. The dysbiosis of the gut microbiota leads to change in the abundance of commensals and symbionts, which is associated with a diverse range of human illnesses and disorders.
The five major phyla of gut bacteria are Firmicutes, Bacteroidetes, Actinobacteria, Proteobacteria, Fusobacteria and Verrucomicrobia, with Firmicutes and Bacteroidetes accounting for 90 per cent of the gut microbiota of a healthy human being (Arumugam et al., Reference Arumugam, Raes, Pelletier, Le Paslier, Yamada, Mende, Fernandes, Tap, Bruls, Batto, Bertalan, Borruel, Casellas, Fernandez, Gautier, Hansen, Hattori, Hayashi, Kleerebezem, Kurokawa and Bork2011). The Firmicutes phylum is composed of more than 200 different genera composing Lactobacillus, Bacillus, Clostridium, Enterococcus and Ruminococcus. Clostridium genera represent 95 per cent of the Firmicutes phyla. Bacteroidetes consist of predominant genera such as Bacteroides and Prevotella. The Actinobacteria phylum is proportionally less abundant and mainly represented by the Bifidobacterium genus. The major gut pathogens are Bacteroides fragilis, Clostridium perfringens, Clostridium botulinum, C. difficile, Enterococcus faecalis, Staphylococcus aureus, Salmonella sp., Shigella sp., Vibrio parahaemolyticus, V. cholerae, Yersinia sp. and Helicobacter pylori belonging to Bacteroidetes, Firmicutes and Proteobacteria phyla. The gut microbiota also differs according to the anatomical areas of the intestine, which also have different physiological characteristics, pH and oxygen tension, substrate abundance and host secretions (Zhang et al., Reference Zhang, Li, Gan, Zhou, Xu and Li2015).
Due to the dynamic nature of the gut microbiome, there are significant differences in the composition and diversity of the gut microbiome among people of different nations. Some bacteria are specific to people of a particular geographical location. Further, specific genes of bacteria have also been identified to be solely present in people of a specific geographical location or ethnic group. According to research performed by Chen and colleagues (Chen et al., Reference Chen, Collij, Jaeger, van den Munckhof, Vich Vila, Kurilshikov and Fu2020), higher abundance of the ppsA gene was only observed in Pseudomonas stutzeri of the European population. Further, Burkholderia pseudomallei S13 is known to be more widespread in the European population. Additionally, it has been found that the prevalence of Bacteroides is higher in European and American populations than in Asian people, and the gene MH0053_GL0075770 has been associated with fat metabolism and could be correlated with the high-fat diet of European and American populations (Chen et al., Reference Chen, Zhang, Huang and Cai2016). Furthermore, research has shown that Prevotella and Treponema are more prevalent in people of Burkina Faso, an African country whose people strictly adhere to a vegetarian diet (De Filippo et al., Reference De Filippo, Cavalieri, Di Paola, Ramazzotti, Poullet, Massart and Lionetti2010). Interestingly, children in Japan were identified to possess a unique microbiome with high prevalence of Bifidobacteriaceae and low presence of Enterobacteriaceae, highlighting the highly hygienic lifestyle of the Japanese population and their eating habits (Nakayama et al., Reference Nakayama, Watanabe, Jiang, Matsuda, Chao, Haryono and Lee2015). The prevalence of Bacteroides plebeius in the gut microbiome of Japanese population which can metabolise porphyran present in seaweeds establishes the link between diet and GI microbiome (Hehemann et al., Reference Hehemann, Correc, Barbeyron, Helbert, Czjzek and Michel2010).
According to Das et al. (Reference Das, Ghosh, Kedia, Rampal, Saxena, Bag and Ahuja2018), Firmicutes, Bacteroidetes, Actinobacteria and Proteobacteria dominate the gut microbiome in Indian communities. Prevotella and Candida were more prevalent in Indians than in Japanese because of the plant-based diet of the Indian population (Pareek et al., Reference Pareek, Kurakawa, Das, Motooka, Nakaya, Rongsen-Chandola and Takeda2019). Another intriguing study by Rothschild et al. (Reference Rothschild, Weissbrod, Barkan, Kurilshikov, Korem, Zeevi, Costea, Godneva, Kalka, Bar, Shilo, Lador, Vila, Zmora, Pevsner-Fischer, Israeli, Kosower, Malka, Wolf, Avnit-Sagi and Segal2018) supports the idea that environmental factors dominate in the formation of the gut microbiome. Individual single nucleotide polymorphisms (SNPs) or genetic ancestry do not significantly influence the microbiota, and previously reported relationships are not consistently observed across investigations (). Although environmental influences are thought to be the main element influencing the development of the gut microbiome, individual genetics also have a role in microbiome composition (Jakobsson et al., Reference Jakobsson, Jernberg, Andersson, Sjölund-Karlsson, Jansson and Engstrand2010). Several genome-wide association studies have linked host genetic variations in immunity-related pathways to microbiome composition in healthy and diseased conditions (Blekhman et al., Reference Blekhman, Goodrich, Huang, Sun, Bukowski, Bell, Spector, Keinan, Ley, Gevers and Clark2015). Mutations in the Mediterranean fever gene (MEFV) were found to be associated with changes in the gut microbiome community structure (Khachatryan et al., Reference Khachatryan, Ktsoyan, Manukyan, Kelly, Ghazaryan and Aminov2008). Various host genetic factors and host immune factors identified to have a role in shaping human microbiome are listed in the “Host Genetic and Immune factors shaping human Microbiota (GIMICA)” database (Tang et al., Reference Tang, Wu, Mou, Wang, Wang, Li, Guo, Yin, Xie, Wang, Wang, Ding, Xue and Zhu2021). Further, differential exposure to a variety of antibiotics also alters a person’s microbial profile. Observational studies have found a negative relationship between the prevalence of microbial communities and antibiotic exposure (Korpela et al., Reference Korpela, Salonen, Virta, Kekkonen, Forslund, Bork and de Vos2016). Interestingly, non-antibiotic drugs like metformin used majorly to treat Type 2 diabetes were also identified to cause dysbiosis of commensal bacteria within the gut (Forslund et al., Reference Forslund, Hildebrand, Nielsen, Falony, Le Chatelier, Sunagawa and Meta2015). The review discusses the functions of commensal bacteria in the gut, the major gut pathogens and dynamics of MGEs between the commensals and pathogens that are important in gut homeostasis and disease progression.
Functions of commensal bacteria in the gut
The presence of commensal bacteria in the gut is known to maintain gut homeostasis and have a significant impact on human health and disease. Kyoto Encyclopedia of Genes and Genomes (Kanehisa and Goto, Reference Kanehisa and Goto2000) analysis of 1520 Culturable Genome Reference of commensal bacteria revealed that they were more involved in carbohydrate and amino acid metabolism (Zou et al., Reference Zou, Xue, Luo, Deng, Qin, Guo, Sun, Xia, Liang, Dai, Wan, Jiang, Su, Feng, Jie, Guo, Xia, Liu, Yu, Lin, Tang, Huo, Xu, Hou, Liu, Wang, Yang, Kristiansen, Li, Jia and Xiao2019). The phyla Fusobacteria, Bacteroidetes, Proteobacteria and other Gram-negative bacteria were identified to possess a wide range of lipopolysaccharide biosynthesis genes (ko00540). Genes that function towards the glycan degradation (ko00531 and ko00511) were identified to be abundant in Bacteroidetes suggesting its involvement in carbohydrate catabolism. Further, genes involved in sphingolipid metabolism (ko00600) and steroid hormone synthesis (ko00140) were identified to be abundant in Bacteroidetes. Proteobacteria were identified to be rich in genes involved in xenobiotic degradation (ko01220) (Zou et al., Reference Zou, Xue, Luo, Deng, Qin, Guo, Sun, Xia, Liang, Dai, Wan, Jiang, Su, Feng, Jie, Guo, Xia, Liu, Yu, Lin, Tang, Huo, Xu, Hou, Liu, Wang, Yang, Kristiansen, Li, Jia and Xiao2019). However, many virulence factors and ARGs were also mapped by virulence factor database (Chen et al., Reference Chen, Yang, Yu, Yao, Sun, Shen and Jin2005) and Comprehensive Antibiotic Resistance Database (Alcock et al., Reference Alcock, Raphenya, Lau, Tsang, Bouchard, Edalatmand, Huynh, Nguyen, Cheng, Liu, Min, Miroshnichenko, Tran, Werfalli, Nasir, Oloni, Speicher, Florescu, Singh, Faltyn and McArthur2020) in bacteria belonging to the Proteobacteria phylum suggesting its ability to cause diseases.
Essential coenzymes like cobalamin are captured by commensals in the gut using surface-exposed lipoproteins (Wexler et al., Reference Wexler, Schofield, Degnan, Folta-Stogniew, Barry and Goodman2018). Biosynthesis of queuosine, a substitute for guanine, having relevance in many physiological defects like cancer progression, neurological deformities and increased cell proliferation was identified to be performed by E. coli and Bacillus subtilis. This was established by studying a queuosine biosynthesis gene mutant E. coli which accumulated epoxyqueuosine (Miles et al., Reference Miles, McCarty, Molnar and Bandarian2011). The main products of the saccharolytic fermentation of carbohydrates, known as SCFA, are formate, acetate, propionate and butyrate, which have a variety of functions in maintaining healthy intestinal physiology, including barrier integrity, immunomodulation, epithelium proliferation and appetite regulation (Chambers et al., Reference Chambers, Morrison and Frost2014; Magne et al., Reference Magne, Gotteland, Gauthier, Zazueta, Pesoa, Navarrete and Balamurugan2020; Morrison and Preston, Reference Morrison and Preston2016).
It has been understood that intestinal commensals breakdown dietary fibres to release indole derivatives, which activates AhR (aryl hydrocarbon receptor) and initiates ILC3 (type 3 innate lymphoid cells) cells to strengthen intestinal mucosa by interleukin-22 (Postler and Ghosh, Reference Postler and Ghosh2017). Further, intestinal commensal bacteria metabolise arginine to secrete polyamines which inhibit NLRP6 (NOD-like receptor family pyrin domain containing 6) inflammasome and also alleviate pro-inflammatory cytokines (Levy et al., Reference Levy, Thaiss, Zeevi, Dohnalová, Zilberman-Schapira, Mahdi, David, Savidor, Korem, Herzig, Pevsner-Fischer, Shapiro, Christ, Harmelin, Halpern, Latz, Flavell, Amit, Segal and Elinav2015). Gut microbiome metabolites are also known to inhibit nuclear factor-κB-dependent synthesis of pro-inflammatory genes that modulates cytokines (Zhang et al., Reference Zhang, Paul and Kundu2022). Additionally, it is known that gut bacteria can alter bile salts generated by the host, which are important for signalling and increasing epithelial barrier function (Sayin et al., Reference Sayin, Wahlström, Felin, Jäntti, Marschall, Bamberg and Bäckhed2013). Polysaccharide A synthesised by B. fragilis acts as an anti-inflammatory molecule which induces the secretion of IL-10 by CD4+ T cells (Johnson et al., Reference Johnson, Jones and Cobb2015). It has been determined that Clostridia maintains the level of retinoic acid in the gut by inhibiting the activity of retinol dehydrogenase 7 (Rdh7) in intestinal epithelial cells (Grizotte-Lake et al., Reference Grizotte-Lake, Zhong, Duncan, Kirkwood, Iyer, Smolenski and Vaishnava2018). Thus, gut commensals play a significant role in modulating the host health by various methods such as nutrient metabolism, drug clearance, barrier integrity maintenance and immunomodulation. Bifidobacteria and Lactobacillus spp. are also widely used as probiotics in the nutraceutical industry, and certain species have a long history of safe use in the manufacture of food, feed and effectiveness in rejuvenating dysbiotic gut due to infection or antibiotic use. However, their use is jeopardised by the erythromycin resistance gene erm(X) translocation, which is mediated by the genomic island BKGI1 (Li et al., Reference Li, Chen, Lin, Wu, Cao, Chen, Hu and Yin2022).
Pathogenic bacteria in the gut
Every year, GI tract infections kill millions of people worldwide. Since bacteria are the most common cause of GI illnesses, antibiotics are frequently used to treat them. The use of antibiotics results in intestinal dysbiosis and, in extreme situations, sepsis due to the release of antibiotic-induced endotoxins (Lepper et al., Reference Lepper, Held, Schneider, Bölke, Gerlach and Trautmann2002). Escherichia, Salmonella, Shigella, Vibrio, Yersinia, belonging to the phyla Proteobacteria, and Clostridia, belonging to Firmicutes, are some common genera of enteric pathogens. These bacterial pathogens have been identified to possess several toxin genes that have been found to be linked to MGEs that could facilitate its transfer to opportunistic pathogens and commensal bacteria of the gut. The toxin genes of enteric pathogens and their associations with various MGEs that can aid in the transfer of these toxin genes have been detailed in the sections below.
B. fragilis
B. fragilis is a rod-shaped, Gram-negative obligate anaerobe belonging to the phyla Bacteroidota. Genome of B. fragilis National Collection of Type Cultures (NCTC) 9343 is widely studied and harbours one single circular chromosome of 5205140 bp harbouring 4274 genes and a plasmid pBF9343 (Pierce and Bernstein, Reference Pierce and Bernstein2016). Although this bacterium is commensal in humans, a subset of it called Enterotoxigenic B. fragilis (ETBF) has been linked to major human illnesses such as colorectal cancer and inflammatory diarrhoea. When clinical isolates of ETBF were compared to the reference strain NCTC 9343, it was identified that the clinical isolates had 23 per cent acquired genes that were responsible for toxins and antibiotic resistance (Pierce and Bernstein, Reference Pierce and Bernstein2016). Pathogenic island was identified to have a reduced G + C content (35 per cent) as compared to the flanking DNA (47–50 per cent) suggesting that the ETBF isolates acquired the pathogenicity island through HGT from some other bacteria in the gut or from another pathogen during a transient infection. Additionally, the toxin gene bft-2 and metalloprotease gene (mpII) (Moncrief et al., Reference Moncrief, Obiso, Barroso, Kling, Wright, Van Tassell and Wilkins1995) were identified to be flanked by putative mobilisation genes bfmA, bfmB and bfmC, and the BfPAI itself is flanked by a mobilisation region similar to that of the plasmid pIP417 known for 5-nitro-imdazole resistance and plasmid pBFTM10 known to provide clindamycin resistance (Haggoud et al., Reference Haggoud, Reysset, Azeddoug and Sebald1994). The proteins synthesised from the bfmC gene were identified to be similar to the TraD mobilisation protein of E. coli plasmid F and R100 (Franco Augusto et al., Reference Franco Augusto, Cheng Rodney, Chung, Wu, Oh and Sears Cynthia1999). Also, the ETBF strains possess a 20 kDa metalloprotease toxin gene called fragilysin responsible for cytotoxicity of intestinal cells in the fragilysin pathogenicity islet present on a transposable element CTn86. Apart from CTn86, there are other putative conjugative transposons CTn9343, CTn9343-like or CTn86-like elements in the regions flanking the pathogenicity islands of ETBF (Buckwold et al., Reference Buckwold, Shoemaker, Sears and Franco2007).
C. perfringens
C. perfringens is a spore forming, rod-shaped, Gram-positive anaerobe belonging to the Bacillota/Firmicutes phyla and is widely found in the gut of healthy humans. However, occasionally, C. perfringens causes various intestinal discomforts and enteric diseases like food poisoning, food independent diarrhoea and colitis (Uzal et al., Reference Uzal, Vidal, McClane and Gurjar2010). Complete genome sequence of 56 enterotoxin-producing C. perfringens isolated from patients having food poisoning demonstrated that they possessed a diverse pangenome with only 12.6 per cent core genome suggesting the occurrence of high rate of HGT and acquisition of new genes that contribute to toxin production, antibiotic resistance and persistence (Kiu and Hall, Reference Kiu and Hall2018). C. perfringens type A strains were identified to possess a putative open reading frame (ORF) showing homology to an ORF of Salmonella Typhimurium IS200 insertional element 1.5 kb upstream of cpe gene that codes for the C. perfringens enterotoxin responsible for the toxicosis (Brynestad et al., Reference Brynestad, Synstad and Granum1997). Further, it was identified that the epsilon toxin (etx) gene present in type B and D strains of C. perfringens is flanked by IS1151 and a gene linked with Tn3 transposon that shows similarity with the gene coding transposase in S. aureus and Lactococcus (Brynestad et al., Reference Brynestad, Synstad and Granum1997; Uzal et al., Reference Uzal, Vidal, McClane and Gurjar2010). The IS1151 located 96 bp upstream of the etx gene in C. perfringens type D strains was identified to be homologous to the insertion sequence (IS) elements of Bacillus thuringiensis and E. coli (Daube et al., Reference Daube, Simon and Kaeckenbeeck1993). Few C. perfringens type A strains were also identified to possess the cpe gene on a large plasmid that contained an IS1470 element in its chromosome (Brynestad et al., Reference Brynestad, Iwanejko, Stewart and Granum1994). The IS1470 element carried the gene coding for a 346 aa transposase enzyme which showed homology with the transpose carried by IS30 (Brynestad et al., Reference Brynestad, Iwanejko, Stewart and Granum1994). Also, the genome of C. perfringens was identified to be rich in phage elements such as ϕSM101, ϕ3626, ϕS9, ϕS63, ϕCP26F, ϕCP390, ϕCPV4, ϕZP2, ϕCP7R, ϕCPV1 and ϕCP24R (Kim et al., Reference Kim, Born, Lurz, Eichenseher, Zimmer, Loessner and Klumpp2012).
C. botulinum
C. botulinum is a rod-shaped, motile, spore forming, Gram-positive anaerobe belonging to the Bacillota/Firmicutes phyla that produce neurotoxin botulinum. Human botulism is caused due to the consumption of contaminated food and can cause neurotoxicity and even paralysis in humans (Nigam and Nigam, Reference Nigam and Nigam2010). There are four groups of C. botulinum of which group I and II cause botulism in humans. Group III causes botulism in animals and group IV has no association with botulism (Peck, Reference Peck2009). According to a 2017 report, the complete genome of only 13 strains of C. botulinum was available at NCBI (http://www.ncbi.nlm.nih.gov/genbank/). However, in 2022, there are about 35 complete genome sequences and 440 partial genome sequences of C. botulinum. The genome size of C. botulinum ranged from 3.2 to 4.2 Mb with a GC content of 27–29 per cent (Bhardwaj and Somvanshi, Reference Bhardwaj and Somvanshi2017). The bont gene cluster encodes for the botulinum neurotoxin (BoNT) that inactivates acetylcholine anchors in neuromuscular junctions and causes paralysis. The presence of bont genes in C. botulinum is identified due to HGT. The bont gene cluster is either present in the chromosome or plasmid of the bacterium. In C. botulinum strain A ATCC 3502, bont genes are present within oppA/brnQ operon, arsC operon or rarA operon (Skarin and Segerman, Reference Skarin and Segerman2011). Furthermore, BoNT is divided into types A, B, C, D, E, F and G. The group II C. botulinum is largely isolated from food-borne infections and is known to produce B, E and F neurotoxin. In C. botulinum, A, B and F toxins are chromosomally encoded, toxin G is encoded by plasmid and prophages encode C1, D and E (Brüssow et al., Reference Brüssow, Canchaya and Hardt2004; Skarin and Segerman, Reference Skarin and Segerman2011). The C. botulinum G toxin was identified to be present on an 81 MDa plasmid, and C. botulinum type C strain (C)-203 U28 was identified to possess the C2 toxin on a large plasmid designated as pC2C203U28. Further, in-depth genomic analysis of C. botulinum revealed that group III strains possess a variety of other toxins encoded in plasmids (Nawrocki et al., Reference Nawrocki, Bradshaw and Johnson2018). A recent report suggests that the group I and II C. botulinum have many bont clusters flanked by IS elements, which allows the mobility of these genes within the genome and also could be transferred to other bacteria (Sakaguchi et al., Reference Sakaguchi, Hayashi, Yamamoto, Nakayama, Zhang, Ma, Arimitsu and Oguma2009). Additionally, the C2 toxin genes were identified to be linked with IS elements like ISCbt5 and ISCbt6 (Sakaguchi et al., Reference Sakaguchi, Hayashi, Yamamoto, Nakayama, Zhang, Ma, Arimitsu and Oguma2009). Though a large number of IS elements and plasmids have been identified in the C. botulinum genome, not much information is present on the prevalence of phage elements in the genome apart from those that harbours the botC and D genes (Hill et al., Reference Hill, Xie, Foley, Smith, Munk, Bruce, Smith, Brettin and Detter2009). However, five phages c-st, c-468, c-203, c-d6f and d-1873 were identified to be responsible for converting non-toxigenic strains of C. botulinum type C and D to toxigenic strains (Sakaguchi et al., Reference Sakaguchi, Hayashi, Kurokawa, Nakayama, Oshima, Fujinaga, Ohnishi, Ohtsubo, Hattori and Oguma2005). Additionally, infection of two bacteriophages, CEβ and Ceγ, was revealed to convert non-toxigenic strains to toxigenic (Eklund et al., Reference Eklund, Poysky, Reed and Smith1971).
C. difficile
C. difficile is an anaerobic, Gram-positive, rod-shaped bacterium belonging to phyla Bacillota, Firmicutes known to cause diarrhoeal disease and colitis in humans. There are more than 2600 genomes of C. difficile deposited in Genbank as of 2022. The complete pangenome of C. difficile was estimated to have around 9640 genes acquired mainly through HGT events which constitute around 11 per cent of the total genome (Eyre et al., Reference Eyre, Cule, Wilson, Griffiths, Vaughan, O’Connor and Walker2013; Scaria et al., Reference Scaria, Ponnala, Janvilisri, Yan, Mueller and Chang2010). Many plasmids have been identified to possess genes that confer antibiotic resistance to C. difficile. Many studies have reported the presence of transposons that confer antibiotic resistance like Tn5397 or CTn3 (tetracycline resistance), Tn5398 (macrolide–lincosamide–streptogramin resistance) in the past. Virulence factors of C. difficile are toxin A (clostridial cytotoxin) and B, encoded by tcdA and tcdB genes on a 19.6 kb long region of chromosome forming a distinct pathogenic locus (PaLoc). Further, tcdB and cdtAB that code for the binary toxin with ADP-ribosyltransferase activity were identified to be coded by putative conjugative plasmids. C. difficile Clade C-I strains were identified to carry a monotoxin tcdB + PaLoc next to a full CdtLoc on extrachromosomal molecules that resemble conjugative plasmids (Ramírez-Vargas and Rodríguez, Reference Ramírez-Vargas and Rodríguez2020). Additionally, the PaLoc encodes proteins that regulate and help in the secretion of the toxin. The transfer of PaLoc was identified to convert a non-toxigenic strain to toxigenic (Brouwer et al., Reference Brouwer, Roberts, Hussain, Williams, Allan and Mullany2013). PaLoc is absent in non-toxic strains. A 115-bp DNA fragment was found between two ISs cdu 2/2′ and cdd 2–3 located upstream and downstream to PaLoc (Braun et al., Reference Braun, Hundsberger, Leukel, Sauerborn and von Eichel-Streiber1996). While in other strains like VPI 10463 the toxigenic element is 19.6 kb in length and contains five ORFs. Four of these ORFs are toxin A, toxin B, ORFtxe2 and ORFtxe3 and ORFtxel (Hammond and Johnson, Reference Hammond and Johnson1995). Interestingly, the exact mechanism of transfer of the PaLoc among the strains is not fully understood. Till date, not much data are available for the presence of transposons that are linked with the mobility of virulence or toxin genes in C. difficile (Brouwer et al., Reference Brouwer, Warburton, Roberts, Mullany and Allan2011). IStrons are a combination of group I intron and an IS which can splice out entirely and transpose to a new location. IStrons are capable of possessing variant proteins as they have the unique splicing activity. Insertion of IStron into the C. difficile toxin A has been found to be responsible for the bacterium to produce alternative variant toxins. Rupnik et al. (Reference Rupnik, Janezic and Kraft2016) studied the various permutations of toxins produced by the different toxin types of C. difficile.
E. faecalis
E. faecalis is a Gram-positive, belonging to the phyla Bacillota, Firmicutes and is a natural resident of the GI tract of humans and is frequently observed in the faecal material. Though the bacteria is considered to be a commensal, it has also been associated with many nosocomial (healthcare-associated) infections including urinary tract infections, bacteremia, wound infections and endocarditis (Fowler et al., Reference Fowler, Miro, Hoen, Cabell, Abrutyn, Rubinstein, Corey, Spelman, Bradley, Barsic, Pappas, Anstrom, Wray, Fortes, Anguera, Athan, Jones, van der Meer, Elliott, Levine and Investigators2005; Murray, Reference Murray1990; Tleyjeh et al., Reference Tleyjeh, Steckelberg, Murad, Anavekar, Ghomrawi, Mirzoyev, Moustafa, Hoskin, Mandrekar, Wilson and Baddour2005). The reference strain of E. faecalis V583, a clinical isolate, was first reported, sequenced and published in 2003 in the USA. It contained 3337 ORFs that encode for proteins in its chromosome and three plasmids pTEF1, pTEF2 and pTEF3. Chromosomal G + C content of the strain was 37.5 per cent, whereas plasmids revealed a G + C content of 33.3–34.4 per cent and encoded for 3240 proteins. A total of the 25 per cent of E. faecalis genome mainly consist of several MGEs such as 38 insertional elements, 7 phage regions, pathogenicity islands and regions for composite transposable elements. Majority of the MGEs were identified to carry ARGs and virulence genes (Giridhara Upadhyaya et al., Reference Giridhara Upadhyaya, Ravikumar and Umapathy2009; Paulsen et al., Reference Paulsen, Banerjei, Myers, Nelson, Seshadri, Read, Fouts, Eisen, Gill, Heidelberg, Tettelin, Dodson, Umayam, Brinkac, Beanan, Daugherty, DeBoy, Durkin, Kolonay, Madupu and Fraser2003). In E. faecalis, the virulence factors mainly include the adherence, biofilm formation, quorum sensing and the toxin genes. Adherence factors such as the ebpA/B/C (pili aiding in bacterial adherence to host proteins), ace (collagen adhesin) and asa1 (aggregation substance) were associated with the virulence of the organism (Fiore et al., Reference Fiore, Van Tyne and Gilmore2019). The toxin cytolysin of E. faecalis was identified to be produced by the genes present in the cyl operon (toxin cytolysin) which comprises 8 genes cylA/B/I/M/R1/R2/S (Fiore et al., Reference Fiore, Van Tyne and Gilmore2019). Additionally, few strains of E. faecalis were identified to produce bacteriocins, which is encoded by a conjugative plasmid pMB1 of 90 kb in size and responsive to sex pheromones released by other bacteria that facilitate its transfer (Martínez-Bueno et al., Reference Martínez-Bueno, Valdivia, Gálvez and Maqueda1992).
Previous studies have unveiled that the most virulent strains of E. faecalis are MDR and strong biofilm formers since they get an upper hand in surviving in the gut as compared to other susceptible enteric bacteria (Mundy et al., Reference Mundy, Sahm and Gilmore2000). The esp gene-encoded enterococcal surface protein (Esp) is responsible for the biofilm formation that allows its colonisation in the GI tract (Kristich Christopher et al., Reference Kristich Christopher, Li, Cvitkovitch Dennis and Dunny Gary2004). Clinical strains of E. faecalis were observed to contain pathogenicity islands that harboured both cytolysin and esp when compared with non-infective oral-derived isolates (Gold et al., Reference Gold, Jordan and van Houte1975). Isolates were also identified to harbour prophage-like elements which are mostly associated with virulence and pathogenicity. Strain V583 contains seven prophage-like elements which fall under the category of temperate phages V583-pp1 to V583-pp7 with size ranging from 12 to 43 Kb (Matos et al., Reference Matos, Lapaque, Rigottier-Gois, Debarbieux, Meylheuc, Gonzalez-Zorn and Serror2013). Apart from the temperate phages, lysogenic phages were also reported, namely GQ478081 (ΦFL1A), GQ478082 (ΦFL1B), GQ478083 (ΦFL1C), GQ478084 (ΦFL2A), GQ478085 (ΦFL2B), GQ478086 (ΦFL3A), GQ478087 (ΦFL3B) and GQ478088 (ΦFL4A) (Stevens et al., Reference Stevens, Ektefaie and Fouts2011). Phage DNA integrates into the host bacteria via integrase belonging to the serine recombinase family at att sites in the chromosome. Proteins encoded from the gene of the phages are either involved in lysogeny maintenance, adhesion and virulence (Brede et al., Reference Brede, Snipen, Ussery, Nederbragt and Nes2011).
S. aureus
S. aureus is a Gram-positive bacterium, again in the phyla Bacillota, Firmicutes and an opportunistic pathogen that colonises different parts of the human body. However, the bacterium is also known to cause diseases like food poisoning, toxic shock syndrome, pneumonia, sepsis and endocarditis. S. aureus is a major contributing cause for the hospital-acquired infections and is notoriously known for acquiring virulence genes encoded by MGEs (Lindsay and Holden, Reference Lindsay and Holden2004). The genome of S. aureus ranges from 2.8 Mb to 2.9 Mb. About 75 per cent of the S. aureus genome was identified to be conserved which forms the core genome and is involved in regular metabolism of the cell. About 25 per cent of the genome was identified to be an accessory genome that contained a lower G + C content as juxtaposed to the core genome (Turner et al., Reference Turner, Sharma-Kuinkel, Maskarinec, Eichenberger, Shah, Carugati, Holland and Fowler2019).
Like in other bacteria, genes associated with virulence and pathogenicity comprise the accessory genome. S. aureus isolates contain one or more plasmids naturally and are classified into three classes, I, II and III. It was identified that in S. aureus most plasmid transfer occurs through transduction as S. aureus is not conjugatively competent. Many ARGs of S. aureus have been associated with plasmids. van A operon that contains genes that confer resistance against vancomycin is understood to be attained by E. faecalis as a result of conjugal transfer (Hiramatsu et al., Reference Hiramatsu, Hanaki, Ino, Yabuta, Oguri and Tenover1997). Apart from the genes that codes for vancomycin resistance, genes that code for resistance against beta-lactam antibiotics were also identified to be present in the plasmids of S. aureus (Altboum et al., Reference Altboum, Hertman and Sarid1985). Additionally, enterotoxin B, bacteriocin and exfoliative toxin B were identified to be plasmid encoded in the pathogen (Bukowski et al., Reference Bukowski, Wladyka and Dubin2010). Six genes (seg, sei, sem, sen, seo and seu) encoding enterotoxins are located on the enterotoxin gene cluster (egc), which is part of the S. aureus genomic island vSaβ (also known as SaPI3/m3). The transfer of vSaβ is facilitated by Staphylococcal temperate phage, Φ SaBov (Moon et al., Reference Moon, Park and Hwang2015).
Apart from plasmids, genetic elements like transposons and IS elements that aid in the bacterial evolution were also identified to be present in S. aureus genome in single or tandem copies. ISs and unit transposons are also known to greatly contribute to antibiotic resistance in S aureus (Byrne et al., Reference Byrne, Rouch and Skurray1989). Apart from antibiotic resistance, the transposons also confer resistance to heavy metals like cadmium (Kuroda et al., Reference Kuroda, Ohta, Uchiyama, Baba, Yuzawa, Kobayashi, Cui, Oguchi, Aoki, Nagai, Lian, Ito, Kanamori, Matsumaru, Maruyama, Murakami, Hosoyama, Mizutani-Ui, Takahashi, Sawano and Hiramatsu2001). Phage elements of S. aureus are of three types, lytic, temperate and chronic. Furthermore, based on the size of the phage element, it is divided into class I (16–20 kb), II (35–40 kb) and III (125–140 kb) (Kwan et al., Reference Kwan, Liu, DuBow, Gros and Pelletier2005). In S. aureus, temperate bacteriophages contain genes like staphylokinase (sak), chemotaxis inhibition protein (scn), enterotoxins and exfoliative toxin (eta) (Deghorain and Van Melderen, Reference Deghorain and Van Melderen2012). Virulence factors such as Panton-Valentine leucocidin, enterotoxin A and exfoliative toxin A are encoded by lysogenic prophages. Virulence-associated genes are generally present near the attachment (att) site and integrative (int) site of the phage element. Helper phages Φ11 and Φ 80 α aid in the replication, mobilisation and excision of Staphylococcal pathogenicity islands (SaPI), which is a non-mobile pathogenic island of S. aureus (Mir-Sanchis et al., Reference Mir-Sanchis, Martínez-Rubio, Martí, Chen, Lasa, Novick and Penadés2012; Ram et al., Reference Ram, Chen, Kumar, Ross, Ubeda, Damle, Lane, Penadés, Christie and Novick2012). Many SaPIs have been sequenced, which encode enterotoxins and toxic shock syndrome toxin (TSST) (Xia and Wolz, Reference Xia and Wolz2014).
Salmonella spp
Salmonella is an enterobacterial Gram-negative, rod-shaped bacteria that come under the phylum Pseudomonadota, that is, Proteobacteria. They are facultative anaerobes, which are responsible for a significant amount of disease burden globally. Salmonella spp. is known as one of the major causes of GI illness worldwide. Globally, 1.3 billion instances of gastroenteritis, 3 million fatalities and 16 million cases of typhoid fever are all attributed to Salmonella each year (Pui et al., Reference Pui, Wong, Chai, Tunung, Jeyaletchumi, Hidayah and Son2011). Salmonella enterica and Salmonella bongori are two of the species that make up the genus Salmonella. More than 2600 serotypes of S. enterica are further split into six subspecies, and they are distinguished from one another by differences in their flagellar (H) and somatic (O) features. The majority of human infections are caused by S. enterica subspecies I (enterica), and it is also the most isolated subspecies in animals (Brenner et al., Reference Brenner, Villar, Angulo, Tauxe and Swaminathan2000). On the other hand, S. bongori has been found mostly in “cold-blooded” animals such as amphibians, fish and reptiles and is also known to cause less than 1 per cent of human infection (Tomastikova et al., Reference Tomastikova, Romero, Knotek and Karpiskova2017). Salmonellae are categorised medically into typhoidal (S. Paratyphi A, S. Paratyphi B, S. Typhi) and non-typhoidal Salmonella (e.g., Enteritidis). S. Typhi murium is known to cause typhoid fever, S. Paratyphi A, B and C cause enteric fever and other serotypes of S. Paratyphi cause salmonellosis. Salmonella serovars that are known to cause gastroenteritis can spread through contaminated food or water or directly through the faecal–oral route. The majority of Salmonella serotypes can cause gastroenteritis, whereas a small number, like S. Typhi, can result in an invasive infection (Rabsch et al., Reference Rabsch, Tschäpe and Bäumler2001). The pathogenicity of Salmonella infections involves a wide range of virulence factors such as Salmonella pathogenicity islands SPI-1, SPI-2 and other SPIs that are encoded with type 3 secretion systems (T3SS), as well as flagella, capsules, plasmids and adhesion systems. The development of a T3SS-2 and intracellular reproduction takes place in a membrane-bound compartment known as the Salmonella-containing vacuole (SCV). Two conserved and stable PAIs, known as Salmonella pathogenicity islands 1 and 2 (SPI-1 and SPI-2, respectively), are present in all S. enterica species. SPI-1 expressed a secretion system of type 3 (TTSS-1), containing invasion genes that enable the bacteria to enter its host intestinal epithelial cells via a process involving actin polymerisation and cytoskeleton remodeling (Raffatellu et al., Reference Raffatellu, Wilson, Chessa, Andrews-Polymenis, Tran, Lawhon, Khare, Adams and Bäumler2005, Jajere Reference Jajere2019). Furthermore, SPI-2, a TTSS-2 encoder, is synthesised when Salmonella infects host phagocytic cells such as dendritic cells and macrophages which facilitates the survivability of Salmonella in the vacuole known as a “SCV” by delaying the development of the vacuole and its fusion with lysosomes. Salmonella proliferation in conditions with low magnesium levels such as in the macrophages depends on SPI-3 (Amavisit et al., Reference Amavisit, Lightfoot, Browning and Markham2003, Foley et al., Reference Foley, Johnson, Ricke, Nayak and Danzeisen2013). Genes located on the SPI-4 are necessary for intra-macrophage survival, apoptosis and the release of toxins. SPI-5 genes encode a variety of T3SS effector proteins, while genes encoded by SPI-6 transport proteins into the cellular environment or host cells in response to external stimuli. Moreover, S. enterica subsp. enterica possessed a large excisable PAI, Salmonella pathogenicity island 7 (SPI-7) containing around 150 genes. The SPI-7 is about 134 kb in size and has a GC content of approximately 49.7 per cent. SPI-7 was identified to be highly mosaic and appears to have been derived by sequential acquisition of different genes. The pathogenicity island apart from possessing genes that are involved in its mobilisation has also been identified to harbour virulence genes such as the Vi antigen, SopE phage and a type IVB pilus locus (Bueno et al., Reference Bueno, Santiviago, Murillo, Fuentes, Trombert, Rodas and Mora2004). The sopE virulence gene (STY4609) encodes SopE protein, an effector protein released by the TTSS-1 that causes actin rearrangement in epithelial cells was identified to be a part of a P2-like prophage located in the middle of SPI-7. S. enterica serovar Enteritidis (S. enteritidis) is a pathogenic bacterium which possesses an unstable pathogenicity island of 26.5 kb named Region of Difference 21 or ROD21 (SPI19). The ROD21, pathogenicity island was identified to be present in the chromosome of S. enteritidis linked to a number of virulence genes (Pardo-Roa et al., Reference Pardo-Roa, Salazar, Noguera, Salazar-Echegarai, Vallejos, Suazo and Bueno2019). Salmonella and various distinct serotypes have been discovered to contain temperature-dependent, diversified and host-limited IncC, IncF, IncHI and IncI1 conjugative plasmids, comprising AR genes. In particular, the IncF conjugative virulence plasmid was acquired from an avian pathogenic E. coli strain (Lindsey et al., Reference Lindsey, Fedorka-Cray, Frye and Meinersmann2009).
Vibrio parahaemolyticus
Vibrio parahaemolyticus is a Gram-negative, curved, rod-shaped, halophilic bacterium belonging to the phyla Pseudomonadota, Proteobacteria that causes food-borne GI illness in humans on the consumption of improperly cooked seafood (Daniels et al., Reference Daniels, MacKinnon, Bishop, Altekruse, Ray, Hammond and Slutsker2000). V. parahaemolyticus was first discovered in 1950 after an outbreak of seafood poisoning in Japan (International Symposium on Vibrio parahaemolyticus, 1974 ). Additionally, V. parahaemolyticus has been linked to cause septicaemia and wound infections in humans (Santos et al., Reference Santos, Tsai, Maquiling, Tayo, Mariatulqabtiah, Lee and Chuang2020). Apart from infections in humans, the pathogen also causes infection in shrimp [acute hepatopancreatic necrosis disease (AHPND)], which is an emerging disease, initially named as early mortality syndrome (Tena et al., Reference Tena, Arias, Álvarez, Mauleón, Jiménez and Bisquert2010). AHPND is not only caused by V. parahaemolyticus but also caused by other members of Vibrio sp. such as V. campbellii, V. owensii and V. punensis. Interestingly, it has been identified that pVA1-type plasmid carries the pirABvp toxin gene responsible for the disease. Further, it was identified that the plasmid can be transferred among the Vibrio spp. through conjugation. The pVA1-type plasmid was identified to have a GC content of roughly 45.9 per cent with a copy number of 37 per bacterial cell, and it comprised of 92 ORFs that encode virulence-associated proteins, mobilisation proteins, replication enzymes, transposases and other proteins which are related to the toxins from the Photorhabdus insect-related (Pir) toxins (Lee et al., Reference Lee, Chen, Yang, Ko, Huang, Huang, Huang, Lin, Chen, Lin, Lightner, Wang, Wang, Wang, Hor and Lo2015). Two genes, pirA- and pirB-like, which are located within a 3.5 kb fragment region, are flanked by 1 kb inverted repeats transposon-coding sequence and are associated for encoding Pir toxin-like proteins in V. parahaemolyticus. The GC content of these 2 genes was found to be substantially lower (38.2 per cent) than the remainder of the plasmid, which suggests that these genes have been acquired through horizontal transfer. V. parahaemolyticus and V. cholerae, the cholera-causing agent, share a phylogenetic relationship. They both have two circular chromosomes. V. parahaemolyticus genome has two chromosomes, which are about 3288558 bp and 1877212 bp and possess 4832 genes, with a G + C content of 45·4 per cent for each chromosome. The chromosome I of both V. parahaemolyticus and V cholerae is identified as not much different in size (3·3 vs 3·0 Mb), but the chromosome II of V. parahaemolyticus was identified to be larger in size than that of V. cholerae (1·9 vs 1·1 Mb) (Tagomori et al., Reference Tagomori, Iida and Honda2002). There are several plasmids identified in V. parahaemolyticus such as pSA19, pZY5 and p22702B. Most of the genes in these plasmids were known to encode hypothetical proteins. The studies on ICEs of V. parahaemolyticus are sparse; however, in 2019, a study by He et al. (Reference He, Wang, Zhang, Zhang, Sun, He and Liu2019) identified ICE positive V. parahaemolyticus isolated from aquacultured shrimp. The ICE was reported to harbour mainly genes that code for antibiotic resistance and heavy metal resistance. In contrast to the limited studies of V. parahaemolyticus plasmids and ICEs, there have been numerous studies on the phage elements that have been acquired by the pathogen and its contribution to its pathogenicity. There have been reports of filamentous vibriophages such as the f237 identified from O3:K6 pandemic clones of V. parahaemolyticus. Other well-characterised phage elements in V. parahaemolyticus include KVP40, VP882, VP93, pO3K6, Vf12, Vf33, VfO3K6, VfO4K68 and VpV262. There has been significant amino acid similarity identified among the V. parahaemolyticus filamentous phages and the phages identified from other species of the Vibrionaceae family (Chang et al, Reference Chang, Taniguchi, Miyamoto and Yoshida1998). Additionally, there has been evidence of other HGT events in the V. parahaemolyticus genome. There has been high similarity observed in the T3SS located on chromosome II of V. parahaemolyticus and non-O1/non-O139 V. cholerae strains. The second T3SS2 of V. parahaemolyticus located on chromosome II was identified to harbour two copies of tdh (thermostable direct haemolysin) flanked by Tn7-like transposase genes. Further, evidence suggests that the V. parahaemolyticus acquired the trh (TDH-related haemolysin) from V. alginolyticus in an event of HGT (González-Escalona et al, Reference González-Escalona, Blackstone and DePaola2006; Xie et al, Reference Xie, Hu, Chen, Zhang and Ren2005). HGT has been identified to cause emergence of pathogenic clones of V. parahaemolyticus from the environment.
H. pylori
H. pylori is a microaerophilic Gram-negative, helical bacteria belonging to the phyla Campylobacterota, Proteobacteria. This bacterium is present in the mucus that colonises the stomach’s epithelium in more than 50 per cent of the world’s population (Bravo et al., Reference Bravo, Hoare, Soto, Valenzuela and Quest2018; Proença-Modena et al., Reference Proenca-Modena, Acrani and Brocchi2009). The disease severity mainly depends upon both the host factors and the bacterial factors. Most of the time, the infection is asymptomatic, but occasionally it can develop into peptic ulcers, mucosa-associated lymphoid tissue lymphoma and even stomach cancer (GC). In 1994, H. pylori was categorised by the World Health Organization as a class I carcinogen (“Schistosomes, liver flukes and Helicobacter pylori (1994). IARC Working Group on the Evaluation of Carcinogenic Risks to Humans. Lyon, 7–14 June 1994,” ). H. pylori are spiral, rod-shaped, curved bacteria having flagella and a membrane sheath outer covering. Motility is another crucial virulence component of their pathogenicity that allows the bacteria to pass through the mucin layer of the gastric epithelium (Josenhans and Suerbaum, Reference Josenhans and Suerbaum2002). Once the bacterium attaches to the gastric epithelial cells, it causes vacuolation of the epithelial cells resulting in cell injury. This vacuolation is the result of the production of a cytotoxin called vacuolating cytotoxin A (VacA), a pore-forming, secreted toxin that is responsible for causing extensive vacuolation in epithelial cells, cell death and epithelial integrity disruption (Szabò et al., Reference Szabò, Brutsche, Tombola, Moschioni, Satin, Telford, Rappuoli, Montecucco, Papini and Zoratti1999). Vacuolisation may differ significantly from strain to strain, and there has been correlation between the severity of H. pylori pathogenesis and the existence of a cytotoxin-associated gene pathogenicity island (PAI). An important virulence factor is the cagA that is present within an island of approximately 30 genes, most probably acquired by H. pylori from other organisms. The clinically important H. pylori has been divided into type I and type II strains. All type I strains have genes that can make both the cytotoxins CagA and VacA, while type II strains only have genes which are necessary that can make VacA. H. pylori has a quite complicated pathophysiology. There are several MGEs in the genome of H. pylori, and several studies have reported that there has been genetic rearrangement within the genome of the pathogen that helps it adapt to the harsh gastric condition and also express virulence and resistance genes. A recent study reported the ICEs of H. pylori type four secretion system (ICEHptfs) are a conserved genomic area in H. pylori. Though the region was identified to be conserved, it was reported to be able to mobilise via conjugation. Additionally, the region portrayed high allele diversity. The ICE element was identified to harbour genes that code for the type 4 secretory system (T4SS), VirB, D and C genes. Apart from the ICEs in the genome of H. pylori, the pathogen is also known to possess cryptic plasmids which provide regions that are hot spots for site specific recombination. Interestingly, the pathogen is also identified to possess plasmids that reveal homology to those of Gram-positive organisms which replicate via rolling circle mechanism and also possess plasmids that replicate via the theta mechanism. Additionally, there have been several IS elements identified in H. pylori that harbours genes that show homology to the genes of other pathogens such as Salmonella (virulence gene gipA) and E. coli (Vale et al., Reference Vale, Encarnação and Vítor2008).
Other enteric pathogens
It has been estimated that half of all the diarrhoeal diseases are due to enteric Gram-negative bacteria. They contribute a significant portion of the burden of diarrhoea and enteric fever which cause more than three million fatalities annually. The major cause of the diarrhoeal infection is the production of one or more bacterial enterotoxins. Other important gut pathogens belonging to the phylum Proteobacteria, Pseudomonadota are V. cholerae and E. coli. V. cholerae have been associated with one of the most severe diarrhoeal infections, cholera, while infections caused by Enterotoxigenic E. coli (ETEC) are responsible for the greatest number of traveller’s diarrhoea. The other important GI diarrhoeal diseases caused by enteric pathogens include Shigella spp., which belongs to the phyla Pseudomonadota, Proteobacteria and Campylobacter jejuni that belongs to Campylobacterota, Proteobacteria. Among viruses, rotavirus is known to cause the most severe diarrhoeal illness among kids under the age of 2–3. Caliciviruses and several adenovirus varieties are further significant GI viruses. Parasitic enteric pathogens also cause diarrhoeal cases that include Entamoeba histolytica, Giardia lamblia and Cryptosporidium spp. These pathogens cause infections by different methods. In general, the conventional infectious cycle includes (1) entry of the pathogen, (2) the establishment and growth of pathogens inside the host cell, (3) evasion of host defences and (4) damage to host and exit. Majority of these functions are achieved by the enteric pathogens with the help of a diverse array of effector molecules. The effector molecules broadly can be classified as those that help the bacterium in the colonisation and establishment of the pathogen in the host gut and the others that help the pathogen for transmission which is achieved by damaging the host cells. The pathogen also produces effector molecules that help the pathogen to evade host immunity.
The enterotoxin that the ETEC strains produce is similar to the cholera toxin (CT), and both cholera and ETEC diarrhoea result in large amounts of water and electrolytes, secreted by the small intestine’s upper fifth. ETEC infection requires adhesion initially and then followed by the synthesis of toxins. ETEC produces two varieties of enterotoxins, a 84-kd heat-labile toxin (LT) and the other ETEC toxin is heat stable (ST) STa and STb. ST has a temperature tolerance of 100 °C and only STa, a peptide with a size of around 2 kD, has been linked to human disease (Joffré et al., Reference Joffré, von Mentzer, Svennerholm and Sjöling2016). Both human and swine genomes have a wide range of genes that encode for various LT variations. Heat-labile enterotoxin (LT) variants LTIp, LTIh, LTIc and LTIIa, encoded by the eltAB gene, have reportedly been related to plasmids, chromosomes and prophages (Jobling et al., Reference Jobling and Holmes2012, Reference Jobling2016; Lasaro et al., Reference Lasaro, Rodrigues, Mathias-Santos, Guth, Balan, Sbrogio-Almeida and Ferreira2008), while the majority of heat-stable toxin variants in humans and pigs have been related to plasmids (Joffré et al., Reference Joffré, von Mentzer, Svennerholm and Sjöling2016; Taillon et al., Reference Taillon, Nadeau, Mourez and Dubreuil2008). Both ETEC and V. cholerae have comparable fimbriae, which are crucial for bacterial adhesion and colonisation in the host’s small intestine. Colonisation factors, which are encoded on plasmids, play an essential role in mediating adhesion, tia an outer membrane adhesin molecule is another important virulence factor, encoded within a pathogenicity island (Fleckenstein, et al., Reference Fleckenstein, Kopecko, Warren and Elsinghorst1996). In addition to the contrasts, there are similarities. The fluid secretion in cholera is largely, though not exclusively caused by a single enterotoxin. But the LT (heat-labile toxin) and ST (heat-stable toxin) enterotoxins are the one(s) or both that induce acute toxicity-related diarrhoeal disease. CT genes are encoded by a prophage (CT phage) located chromosomally whereas in case of ETEC, both the ST and LT genes are found on plasmids and are not phage associated. Majority of the GI pathogens, including the EPEC, Salmonella Shigella and Yersinia use its T3SS to deliver the effector proteins into the host cells. Shigella readily invades the epithelial cells of the human intestine from the basolateral surface. The Shigella sp. contains a single circular chromosome and a virulence plasmid. The virulence plasmid has been associated with the virulence and pathogenesis of the pathogen. Majority of the virulence factors of Shigella are situated in a 30 kb region termed as the “entry region” which contains mxi-spa locus, which encodes a T3SS. This large plasmid also encodes for the proteins (IpaB and IpaC) that help the bacteria to enter the host cells, multiply and spread to adjacent cells (Sansonetti et al., Reference Sansonetti, Van Nhieu and Égile1999). In addition to the virulence plasmid, pathogenicity islands (PAI) on the Shigella chromosome also harbour genes that contribute to the virulence of the pathogen. Interestingly, it has been identified that the genes and other elements in the PAI can be found in a variety of combinations depending on the Shigella species and subtype. A combination of both chromosomal virulence factors and plasmid virulence factors mediate the invasiveness and virulence of the pathogen. Shigella enterotoxin 1 (ShET1) and Shigella enterotoxin 2 (ShET2) are major virulence factors for mediating early fluid secretion in the jejunum and then subsequently in the colon. ShET1 is encoded by set1A and set1B genes on the Shigella chromosome as part of the SHI-1 PAI. The PAI is specific to only S. flexneri 2a isolates (Vargas et al., Reference Vargas, Gascon, Jimenez De Anta and Vila1999; Yavzori et al., Reference Yavzori, Cohen and Orr2002). The two toxin subunits together form the holo-AB-type toxin complex in an A1-B5 configuration, similar to that of the cholera holotoxin, and are secreted via Sec pathway and Type II secretion (Faherty et al., Reference Faherty, Redman, Rasko, Barry and Nataro2012).
Another major enteric pathogen is the Yersinia again a member of phyla Pseudomonadota, Proteobacteria, and three species, namely Y. pestis, Y. enterocolitica and Y. pseudotuberculosis, are known to cause lethal disease in humans. The pathogen is associated with causing infection in regional lymph nodes or lungs and also a broad range of GI diseases, from enteritis to mesenteric lymphadenitis (Bibikova, Reference Bibikova1977; Putzker et al., Reference Putzker, Sauer and Sobe2001; (Pujol and Bliska, Reference Pujol and Bliska2005). Virulent Yersinia species have several virulence factors, like a 70-kb virulence plasmid, pCD1 in Y. pestis and pYV in enteropathogenic Yersinia. They also encode for the yersiniabactin (Ybt) system (Brubaker, Reference Brubaker1991; Cornelis et al., Reference Cornelis, Boland, Boyd, Geuijen, Iriarte, Neyt and Stainier1998; Heesemann et al., Reference Heesemann, Hantke, Vocke, Saken, Rakin, Stojiljkovic and Berner1993). The 70-kb virulence plasmid in Y. pestis has been identified to harbour several genes that code for the structural components of a T3SS, and also the T3SS effector proteins called Yersinia outer proteins (Yops) (Bliska et al., Reference Bliska, Wang, Viboud and Brodsky2013; Schwiesow et al., Reference Schwiesow, Lam, Dersch and Auerbuch2015). The Yops protein is known to help the pathogen in immune evasion. Yersinia species also possess a number of T6SSs with distinct biological functions. The T6SSs delivers multiple effector proteins while other secretory systems are known to deliver a single type of effector protein. In addition to effector proteins that are toxins, some effector molecules delivered via the T6SS system also enhance the persistence of the pathogen. The T6SS is also identified to have a role in the biofilm formation of a bacteria (Southey-Pillig et al., Reference Southey-Pillig, Davies and Sauer2005). The different toxin genes associated with MGEs in different bacterial enteric pathogens have been summarised in Table 1.
Table 1. Mobile genetic elements associated with toxin genes in bacterial pathogens causing enteric diseases.
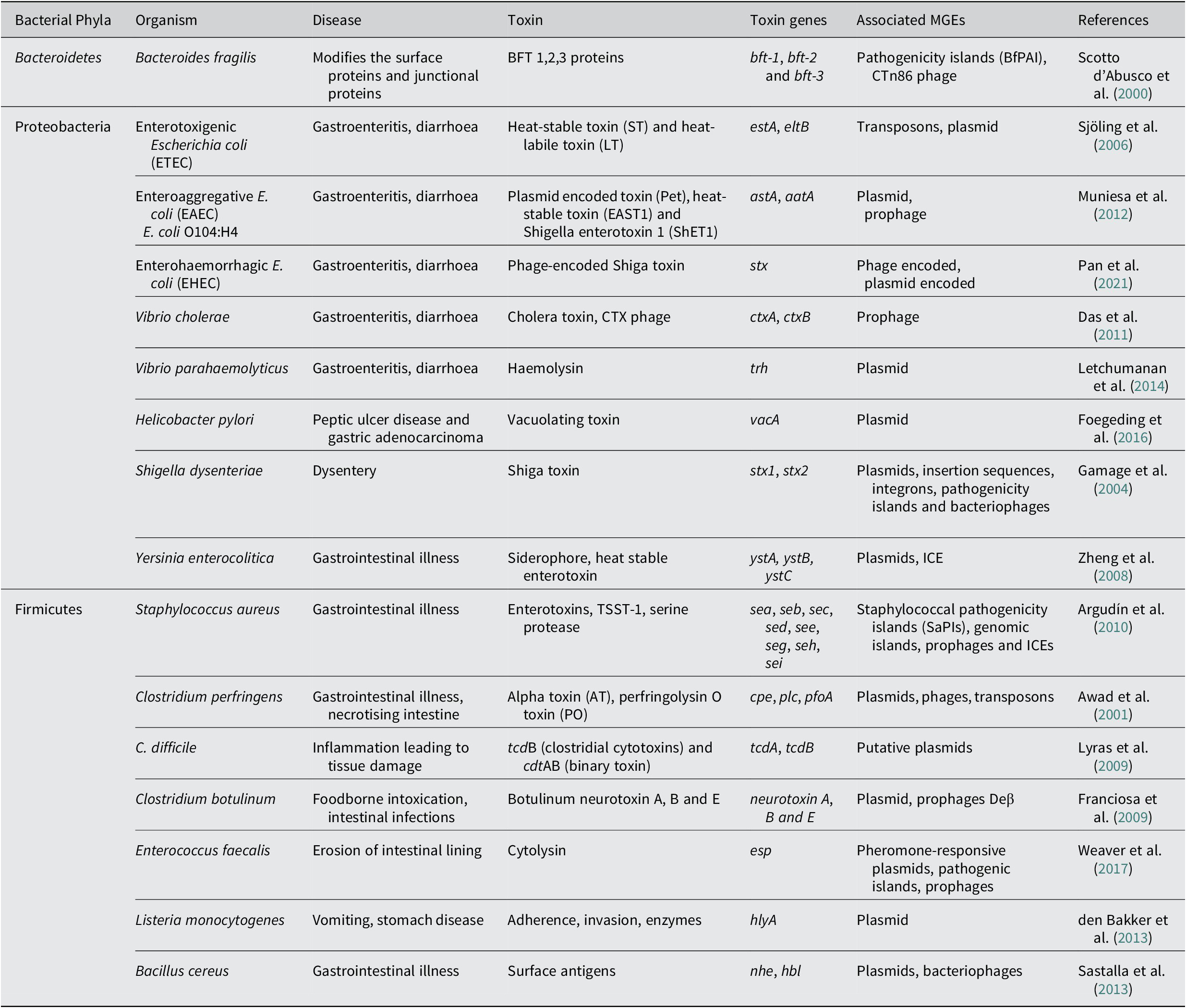
Dynamics of toxin-linked MGEs
As discussed in the above sections of the review, a large number of virulence determinants have been associated with MGEs in important gut pathogens. Though there are several studies and reviews that highlight the importance of MGEs associated with ARGs and their transmission dynamics among the commensals and the pathogens, studies discussing the importance of MGEs associated with virulence genes and their dynamics is sparse. Though HGT between species from different phyla is considered a rare event, it is common within the same species. However, there are also interesting reports of HGTs between even kingdoms where bacterial genes and its protein homologous have resulted in gain of functions in higher order organisms such as fungi, nematodes and eukaryotes (Mayer et al., Reference Mayer, Schuster, Bartelmes, Dieterich and Sommer2011; Moran and Jarvik Reference Moran and Jarvik2010). Jaramillo et al. (Reference Jaramillo, Sukno and Thon2015) identified 11 HGT events of toxin genes from bacteria to the plant fungi Colletotrichum gloeosporioides. Many toxin genes homologous to subtilisin genes were acquired by the fungi from Bacillus pumilus. Further, there are also reports of cross phyla dynamics of toxins with the striking example of aerolysin, a pore forming toxin present in Aeromonas hydrophila also identified in many pathogens belonging to the phyla Firmicutes and Proteobacteria (Kennedy et al., Reference Kennedy, Lyras, Cordner, Melton-Witt, Emmins, Tweten and Rood2009). Further, broad host range MGEs have been observed to transcend phyla and mobilise from the commensals to the pathogen isolates (Forster et al., Reference Forster, Liu, Kumar, Gulliver, Gould, Escobar-Zepeda, Mkandawire, Pike, Shao, Stares, Browne, Neville and Lawley2022). Forster and his team compared more than 1000 commensal strain genomes belonging to 540 species and more than 45,000 pathogens belonging to 12 species and found more than 64,000 MGE-mediated transfer events between the commensals and the pathogens.
A well-studied transfer of toxin gene within the same bacterial species is the transfer of CT gene from toxigenic V. cholerae O1 to environmental non-O1/O139 V. cholerae (Choi et al., Reference Choi, Dunams and Jiang2010). Vibrio phages that are a common inhabitant of aquatic systems are known to play an important role in the transfer of CT genes (CTX-AB) from a toxigenic strain to a non-toxigenic strain and modulate dynamics and evolution of V. cholerae. Transduction experiments were conducted using toxigenic V. cholerae O395 and E4 strains to determine the ability of vibrio phages to transfer CTXɸ genes to non-toxigenic strains (Choi et al., Reference Choi, Dunams and Jiang2010). The NetB pore-forming toxin produced by the C. perfringens when co-cultured with netB negative C. perfringens isolates has been identified to acquire the toxin gene through the transfer of the conjugative plasmid pJIR3535 and pNetB-Ne10 (Lacey et al., Reference Lacey, Keyburn, Ford, Portela, Johanesen, Lyras and Moore2017).
Further, cross species HGT of toxin genes has been demonstrated by Muthukrishnan et al. (Reference Muthukrishnan, Defoirdt, Shariff, Ina-Salwany, Yousoff and Natrah2019) through co-culture experiments of pirAB positive V. parahaemolyticus isolates and pirAB negative Algoriphagus sp. Strain. The transfer of pirAB gene occurs through the conjugative transfer of pVA1 plasmid. The toxin that causes sloughing and degeneration of the hepatopancreas of shrimp has been identified not only in V. parahaemolyticus but also in several other Vibrio sp. and also non-Vibrios (Dong et al., Reference Dong, Wang, Xie, Zou, Guo, Liang and Huang2017; Restrepo et al., Reference Restrepo, Bayot, Arciniegas, Bajaña, Betancourt, Panchana and Reyes Muñoz2018). Another example of transfer of toxin genes among different species of bacteria is the conjugal plasmid (pVT1) of V. tapetis that causes the brown ring disease. The mosaic plasmid is known to contain DNA regions similar to that of V. vulnificus, Photobacterium profundum, Listonella anguillarum and Shewanella sp. The dynamics of MGE-linked toxin genes among gut pathogens and non-pathogenic bacteria can happen within the gut and also in the environment. Environmental parameters are known to play a significant impact in the HGT and expression regulation of virulence genes. A biofilm environment is known to increase the rate of HGTs due to the close proximity of the bacterial cells within the biofilm (Gyles and Boerlin, Reference Gyles and Boerlin2013). Additionally, TA systems are also known to contribute in the selection and maintenance of MGEs (Aminov et al., Reference Aminov2011). The TA system comprises a stable toxin present in the chromosome and a labile anti-toxin usually located on plasmids. When the bacterial cells lose the plasmid, the anti-toxin expression ceases and the toxin expression causes cell death. Thus, this two-component system selectively eliminates plasmid-free bacterial cells in a population (Aminov et al., Reference Aminov2011). Hence, understanding the environmental factors affecting the transfer, genetics and dynamics of virulence associated with MGEs will shed light on the evolution of bacteria as well as understand futuristic emerging bacterial pathogens. Further, comprehending broad host MGEs can allow researchers to identify natural and synthetic molecules that can reduce its mobility, preventing virulence and ARG transfer.
Conclusion
The breakthrough in sequencing technologies has opened the door to examining myriads of microbes inhabiting the human gut. Insights into their genomes have helped in understanding the ecology of different microbes, their functions as well as the dynamics of MGEs linked with various fitness traits. In most of the bacterial enteric pathogens, the reason for the diseased condition is the toxin production, which is encoded by these MGEs. These MGEs include mostly phages, pathogenicity islands, plasmids and transposons. The present review summarises the different MGEs associated with virulence traits of clinically important enteric pathogens. Further studies on the MGEs will pave the way for a better understanding of their bacterial specificity, integration–excision mechanisms as well as inheritance. This understanding can further aid the researchers in designing strategies to prevent the spread of these MGEs that have an important role in alleviating the diseased condition. Further, various strategies like screening of natural and synthetic compounds that can cure the MGEs from bacterial pathogens, thereby making them less virulent and sensitive to existing antibiotics, can be formulated. These strategies, designed to combat the stability of these MGEs, could help to reduce the disease burden and prevent the emergence of antibiotic-resistant mutants in the future.
Acknowledgement
We thank Dr. G. Balakrish Nair for his valuable comments. Ms. Shashi Kumari is thankful to CSIR-GOI for her PhD fellowship. Dr. Deepjyoti Paul and Dr. Lekshmi N are thankful to the DBT-GOI for the MK Bhan fellowship program.
Author contribution
S.P., S.K. and B.D. drafted the original review; J.V., S.B., D.P., L.N. and B.D. edited and finalised the review.
Funding
This study received financial support from the Dept. of Biotechnology (DBT), Govt. of India (Grant No. BT/PR38173/MED/97/474/2020).
Competing interest
The authors declare that there is no conflict of interest.
Notes on contributors
SP, SK, JV, SB, LN, and DP created the first draft of the review. BD edited and finalize the review.