Introduction
Exposure to childhood trauma, encompassing different forms of maltreatment early in life, is an important risk factor for most psychiatric disorders (Green et al., Reference Green, McLaughlin, Berglund, Gruber, Sampson, Zaslavsky and Kessler2010; Varese et al., Reference Varese, Smeets, Drukker, Lieverse, Lataster, Viechtbauer and Bentall2012). Childhood trauma may affect brain development, leading to aberrant cognitive functioning and emotional regulation and subsequent behavioral dysfunction (Lupien, McEwen, Gunnar, & Heim, Reference Lupien, McEwen, Gunnar and Heim2009; Sánchez, Ladd, & Plotsky, Reference Sánchez, Ladd and Plotsky2001; Teicher & Samson, Reference Teicher and Samson2016). Patients with a history of trauma may therefore form a specific group in terms of symptom presentation, response to treatment and clinical needs. As this subgroup is fairly large, roughly varying between 40% and 60% across psychiatric disorders, identification of trauma-related features of psychopathology, including their underlying neurobiological correlates, may inform individually tailored mental health care (Álvarez et al., Reference Álvarez, Roura, Osés, Foguet, Solà and Arrufat2011; Devi et al., Reference Devi, Shahwan, Teh, Sambasivam, Zhang, Lau and Subramaniam2019; Green et al., Reference Green, McLaughlin, Berglund, Gruber, Sampson, Zaslavsky and Kessler2010; Porter, Branitsky, Mansell, & Warwick, Reference Porter, Branitsky, Mansell and Warwick2020; Schäfer & Fisher, Reference Schäfer and Fisher2011; Wiersma et al., Reference Wiersma, Hovens, van Oppen, Giltay, van Schaik, Beekman and Penninx2009).
Evidence on trauma-related gray matter alterations is now accumulating from structural magnetic resonance imaging (sMRI) investigations. Prior literature has largely discussed the effect of childhood trauma on the hippocampus and amygdala (Lim, Radua, & Rubia, Reference Lim, Radua and Rubia2014; Paquola, Bennett, & Lagopoulos, Reference Paquola, Bennett and Lagopoulos2016). This primary focus was guided by early findings in animal studies on the effect of early life stress impacting these regions, and clinical observations that linked childhood trauma to reduced memory performance and anxiety/mood regulation (Myers, McKlveen, & Herman, Reference Myers, McKlveen and Herman2014). Early MRI studies investigating the effect of trauma in patient samples have often used a region-of-interest (ROI) approach with hippocampus and amygdala as only ROIs (Lim et al., Reference Lim, Radua and Rubia2014; Paquola et al., Reference Paquola, Bennett and Lagopoulos2016), overlooking the role of trauma in other areas. The gray matter correlates of childhood trauma may well extend beyond the hippocampus and amygdala, as suggested by (pre)clinical childhood trauma models that implicate more widespread corticostriatal-limbic involvement (Edmiston et al., Reference Edmiston, Wang, Mazure, Guiney, Sinha, Mayes and Blumberg2011; Monroy, Hernández-Torres, & Flores, Reference Monroy, Hernández-Torres and Flores2010). Clinical studies also indicate a more extensive negative impact of childhood maltreatment involving a wide range of affective and cognitive functions, such as reward processing, attention and executive functioning, which are not primarily supported by hippocampus and amygdala function (Hart & Rubia, Reference Hart and Rubia2012; Pechtel & Pizzagalli, Reference Pechtel and Pizzagalli2011). Such complex, higher-order functions are rather represented by networks that encompass both subcortical and cortical brain structures (Menon, Reference Menon2011). Thus, childhood trauma is hypothesized to lead to broader gray matter volume reductions in distributed cortical and subcortical areas, which is thought to impact cognition and emotion regulation and could be an independent risk factor for the development of psychopathology.
Despite the hypothesized general impact of childhood trauma, most of our knowledge on its neurobiological correlates stems from studies investigating individual diagnostic categories, including post-traumatic stress disorder, mood and anxiety disorders such as bipolar-I disorder, and schizophrenia (Teicher & Samson, Reference Teicher and Samson2016). As childhood trauma may affect patients with different diagnoses in a similar vein prior to the development of psychopathology, it is important to investigate its neurobiological consequences using a transdiagnostic approach (Bennett & Lagopoulos, Reference Bennett and Lagopoulos2018; Paquola et al., Reference Paquola, Bennett and Lagopoulos2016). Moreover, as only a minority of maltreated children will develop a psychiatric illness later in life, assessing the impact of childhood trauma both in the presence and absence of psychopathology will help disentangle the neurobiological effects of trauma from diagnostic classification (Begemann, Schutte, & Sommer, Reference Begemann, Schutte and Sommer2015b; Bennett & Lagopoulos, Reference Bennett and Lagopoulos2018; Paquola et al., Reference Paquola, Bennett and Lagopoulos2016).
In this study, we aimed to identify trauma-related gray matter alterations across the brain by investigating both cortical and subcortical regions in an exploratory manner. Moreover, we aimed to find correlates of trauma across diagnostic boundaries, by analyzing patients with disorders in two distinct DSM-V categories that share childhood adversity as an environmentally contributing factor. Relative to the general population, trauma-exposed individuals have a three-fold increased risk of developing bipolar disorder (Palmier-Claus, Berry, Bucci, Mansell, & Varese, Reference Palmier-Claus, Berry, Bucci, Mansell and Varese2016) or psychosis (Varese et al., Reference Varese, Smeets, Drukker, Lieverse, Lataster, Viechtbauer and Bentall2012). Therefore, we included 250 bipolar-I disorder patients and 84 patients with a schizophrenia-spectrum disorder. We also included a sample of healthy individuals (n = 220) to investigate possible correlates of trauma resilience.
Methods
Participants
Data were obtained from MRI studies conducted in the University Medical Center Utrecht (the Netherlands): Bipolar Genetics study (Vreeker et al., Reference Vreeker, Boks, Abramovic, Verkooijen, van Bergen, Hillegers and Riemersma-Van der Lek2016), Spectrum study (Sommer et al., Reference Sommer, Daalman, Rietkerk, Diederen, Bakker, Wijkstra and Boks2010) and Simvastatin for recent-onset psychosis (baseline data) (Begemann et al., Reference Begemann, Schutte, Slot, Doorduin, Bakker, van Haren and Sommer2015a). Participants were ⩾18 years of age. We included 554 participants: 220 healthy individuals without a current or past psychiatric diagnosis, 251 patients with type-I bipolar disorder, and 83 patients with a schizophrenia-spectrum disorder (DSM-IV-TR) (American Psychiatric Association, 2000). Presence or absence of psychopathology was established using the Structured Clinical Interview for DSM-IV (SCID-I) (First, Reference First1997), the Mini-International Neuropsychiatric Interview (Sheehan et al., Reference Sheehan, Lecrubier, Sheehan, Amorim, Janavs, Weiller and Dunbar1998) or the Comprehensive Assessment of Symptoms and History Interview (CASH) (Andreasen, Flaum, & Arndt, Reference Andreasen, Flaum and Arndt1992). Studies were approved by the medical ethical committee, participants gave written informed consent. Procedures are described in more detail in the eAppendix in Supplement.
Childhood trauma
Childhood maltreatment was assessed with the Dutch version of the Childhood Trauma Questionnaire–Short Form (CTQ-SF total score) (Bernstein et al., Reference Bernstein, Stein, Newcomb, Walker, Pogge, Ahluvalia and Zule2003; Thombs, Bernstein, Lobbestael, & Arntz, Reference Thombs, Bernstein, Lobbestael and Arntz2009). This 25-item version evaluates five trauma subtypes (sexual, physical and emotional abuse, physical and emotional neglect), summing up to a total score. Good internal consistency reliability has been reported in both clinical and community samples (Bernstein et al., Reference Bernstein, Stein, Newcomb, Walker, Pogge, Ahluvalia and Zule2003; Scher, Stein, Asmundson, McCreary, & Forde, Reference Scher, Stein, Asmundson, McCreary and Forde2001). Established cut-off scores for moderate to severe trauma were applied to classify the presence of a specific trauma subtype (emotional neglect ⩾15; physical neglect ⩾10; emotional abuse ⩾13; physical abuse ⩾10; sexual abuse ⩾8) (Bernstein et al., Reference Bernstein, Stein, Newcomb, Walker, Pogge, Ahluvalia and Zule2003).
Structural magnetic resonance imaging
Structural MRI scans of the whole brain were acquired on the same 3T Philips Achieva medical scanner, equipped with an 8-channel SENSE headcoil (Philips, The Netherlands). Three-dimensional high-resolution T1-weighted images (high-res T1) were obtained with the following parameters; 200 contiguous sagittal slices (TE = 4.6 ms, TR = 10 ms, flip angle = 8°, FOV = 240 mm,0.75 × 0.75 × 0.80 mm3 voxels). T1-weighted images (T1) with a slightly lower resolution (160 contiguous sagittal slices, TE = 4.6 ms, TR = 10 ms, flip angle = 8°, FOV = 224 mm, 1 × 1 × 1 mm3 voxels) were available for 43 healthy participants and 33 schizophrenia-spectrum disorder patients, yet no differences in total gray matter volume were observed when contrasting individuals with lower v. higher resolution T1 images within these groups (p = 0.651 in the healthy control group; p = 0.323 in the schizophrenia-spectrum group).
All 554 scans were checked for radiological abnormalities by a radiologist blinded to subject status (healthy individual or patient). Structural images were processed using the automated segmentation pipeline of FreeSurfer version 5.3.0 (http://surfer.nmr.mgh.harvard.edu) (Fischl et al., Reference Fischl, Van Der Kouwe, Destrieux, Halgren, Ségonne, Salat and Kennedy2004). By automatic parcellation of the cortical and subcortical structures, gray matter was divided into 82 distinct anatomical volumes of the Desikan−Killiany atlas (Desikan et al., Reference Desikan, Ségonne, Fischl, Quinn, Dickerson, Blacker and Hyman2006). Segmentations were manually checked for volumes deviating significantly from the population mean [i.e. more than two standard deviations (s.d.)] regarding total gray matter volume, thickness (left and right), surface area (left and right) and cerebral brain volume. When visual inspection of these images showed gross scan or segmentation errors, these segmentations were deemed unfit for further analysis. Images from two participants showed radiological abnormalities, two scans showed segmentation faults and seven scans were of insufficient quality, resulting in 543 scans suitable for analyses. The eleven excluded participants (four healthy controls, two bipolar-I patients and five schizophrenia-spectrum disorder patients) did not significantly differ (>2 s.d.) from the remaining sample across demographic variables or childhood trauma score. For each participant, total gray and white matter volumes were combined to calculate a general measure of cerebral brain volume.
We hierarchically grouped brain regions in order to obtain sufficient statistical power for analyzing both cortical and subcortical areas. The 68 individual cortical regions first were grouped into five cortical lobes: frontal (n = 22), parietal (n = 10), occipital (n = 8), temporal (n = 18) and insula/cingulate (n = 10) (Allen, Damasio, & Grabowski, Reference Allen, Damasio and Grabowski2002; Allen, Damasio, Grabowski, Bruss, & Zhang, Reference Allen, Damasio, Grabowski, Bruss and Zhang2003; Collin et al., Reference Collin, de Reus, Cahn, Hulshoff Pol, Kahn and van den Heuvel2013; Desikan et al., Reference Desikan, Ségonne, Fischl, Quinn, Dickerson, Blacker and Hyman2006; Long et al., Reference Long, Liao, Jiang, Liang, Qiu and Zhang2012). The 14 subcortical regions were grouped into one subcortical lobe, including the bilateral nucleus accumbens, amygdala, caudate, hippocampus, pallidum, putamen and thalamus. For significant lobes, individual regions were evaluated to investigate their contribution to the effect.
Statistical analysis
Statistical analyses were performed using SPSS software, version 22.0. Group-differences in demographic characteristics were evaluated using chi-squared tests for categorical variables and F-tests for continuous variables. Differences in total cerebral brain volume were evaluated using ANCOVA (age and sex as covariates). Significant demographical findings were followed by post-hoc analyses to compare the three groups (Bonferroni corrected).
First, we used a linear approach by applying multivariate regression models to evaluate the association between cumulative childhood trauma severity (CTQ-SF total score) and total gray matter volume within the total sample, followed by the individual (sub)cortical lobes. All models were adjusted for group (dummy coding healthy controls, bipolar type-I and schizophrenia-spectrum disorder) and the covariates age, sex, cerebral brain volume, medication status (binary variable representing whether or not the patient was currently on an antipsychotic, antidepressant or lithium) and type of scan (dummy variable, high-res T1 v. T1). Analyses were Hochberg-adjusted (Benjamini & Hochberg, Reference Benjamini and Hochberg1995) for familywise multiple testing errors, using a false discovery rate (FDR) of 5% (q < 0.050). After identifying significant lobe(s), follow-up analyses were performed to specify which individual brain region(s) contributed to this effect (p < 0.050). Considering earlier sMRI findings focusing on the hippocampus and amygdala as single ROI's, we additionally defined these regions as specific outcomes of interest. Trauma-by-group interaction effects were examined by adding this interaction term in a separate set of analyses.
Second, a categorical approach was used to follow up on significant findings by means of the Jonckheere−Terpstra tests for ordered alternatives (J−T, one-tailed test) (Jonckheere, Reference Jonckheere1954). This, to address the general observations of low trauma scores in healthy control cohorts, in addition to a substantial number of patients not reporting any type of trauma. In the current sample, about 70% of the healthy control subgroup did not report any type of trauma according to CTQ cutoff-scores, in addition to more than 50% of both patient subgroups. For clinical relevance, individuals were first grouped according to the number of childhood trauma subtypes they had experienced using the established cut-off scores for moderate to severe trauma (Bernstein et al., Reference Bernstein, Stein, Newcomb, Walker, Pogge, Ahluvalia and Zule2003). This resulted in four categories: no trauma; one trauma subtype, two trauma subtypes; and three or more trauma subtypes (three, four or five trauma subtypes were combined due to the low number of participants in these categories). Moreover, to address potential concerns on the use of predefined cut-off values, we created quartiles based on the total sample distribution of cumulative childhood trauma scores. After splitting the total sample by subgroup, J-T tests were repeated for the bipolar-I patients, schizophrenia-spectrum patients and healthy controls separately.
Results
Demographic characteristics
The subgroup of schizophrenia-spectrum disorder patients (n = 79) was on average younger and included more male participants than the other subgroups (Table 1). Healthy controls (n = 216) were significantly younger than bipolar patients (n = 248). Mean childhood trauma severity (CTQ-total score) was significantly higher for both patient groups as compared to healthy controls (Table 1). Figure 1 displays the prevalence of the five childhood trauma subtypes across the three subgroups. The number of individuals reporting to at least one form of trauma was similar in the bipolar patients (49.2%) and the schizophrenia-spectrum group (43%), this percentage was significantly lower in the healthy control group (29.6%). Total cerebral brain volume was significantly smaller in the bipolar and schizophrenia-spectrum subgroups, as compared to controls (Table 1).

Fig. 1. Number of individuals (%) exposed to different childhood trauma subtypes across the three subgroups.
Table 1. Demographic characteristics of the included participants

s.d., standard deviation; CTQ, Childhood Trauma Questionnaire; BP, Bipolar-I patients; SSP, Schizophrenia-Spectrum Patients; HC, healthy controls.
a Individuals were categorized as having a history of childhood trauma when scoring above the cut-off for one or more trauma subtype(s). Bold values should be p < 0.001.
Linear trauma-related gray matter volume alterations across the brain
Considering the total sample (n = 512), the negative association between childhood trauma severity and total gray matter volume was not significant (β = −0.021, p = 0.124), correcting for group (p = 0.361) and including age, sex, cerebral brain volume, medication status and type of scan. Childhood trauma was significantly related to reduced gray matter volume of the frontal lobe (β = −0.049, p = 0.008; FDR-corrected, q = 0.048, scatter plot provided in eFigure 1 in Supplement), but not to the volume of other (sub)cortical lobes (Table 2). Childhood trauma severity was not associated with gray matter volume of the hippocampus (left: β = 0.005, p = 0.883; right: β = −0.009, p = 0.802) or amygdala (left: β = 0.002, p = 0.953; right: β = −0.054, p = 0.133). Analyses did not show any significant group effects on gray matter volume and there was no evidence for trauma-by-group interactions in a separate set of analyses.
Table 2. Linear associations between childhood trauma severity and gray matter volumes

s.e., standard error; FDR, False-Discovery Rate.
a Group, age, sex, cerebral brain volume, type of scan (high-resolution T1 v. T1), and medication status were included as covariates. Bold values should be p < 0.001.
Sensitivity analyses showed that the trauma-related gray matter volume reductions within the frontal lobe were localized in the right medialorbitofrontal region (β = −0.105, p = 0.002), right paracentral region (β = −0.088, p = 0.018), right superior frontal area (β = −0.058, p = 0.027) and left precentral region (β = −0.057, p = 0.0498) (eTable 1 in Supplement). Group had a significant effect on the left caudal middle frontal region (β = 0.129, p = 0.022) and bilateral rostral middle frontal regions (left: β = 0.143, p = 0.002; right: β = 0.087, p = 0.049). Post-hoc testing indicated larger gray matter volumes in the bipolar subgroup v. healthy controls (p = 0.013, p = 0.002 and p = 0.025, respectively). No trauma-by-group effects were observed.
Categorical trauma-related gray matter alterations in the frontal lobe
Including the total sample, more pronounced frontal gray matter volume reductions were found in individuals reporting an increasing number of childhood trauma subtypes (J-T = −3.86, p < 0.001, eTable 2, Fig. 2). Cumulative trauma scores, independent from trauma subtype, revealed a similar dose-response pattern: higher quartiles of overall trauma rates were linked to more pronounced gray matter volume reductions in the frontal lobe (J-T = −3.25, p = 0.001, eTable 2, Fig. 2). When repeating these analyses for the individual subgroups, similar significant dose-response patterns were observed in the bipolar-I patients and healthy controls, but did not reach significance for the schizophrenia-spectrum subgroup (eTable 2, Fig. 3).
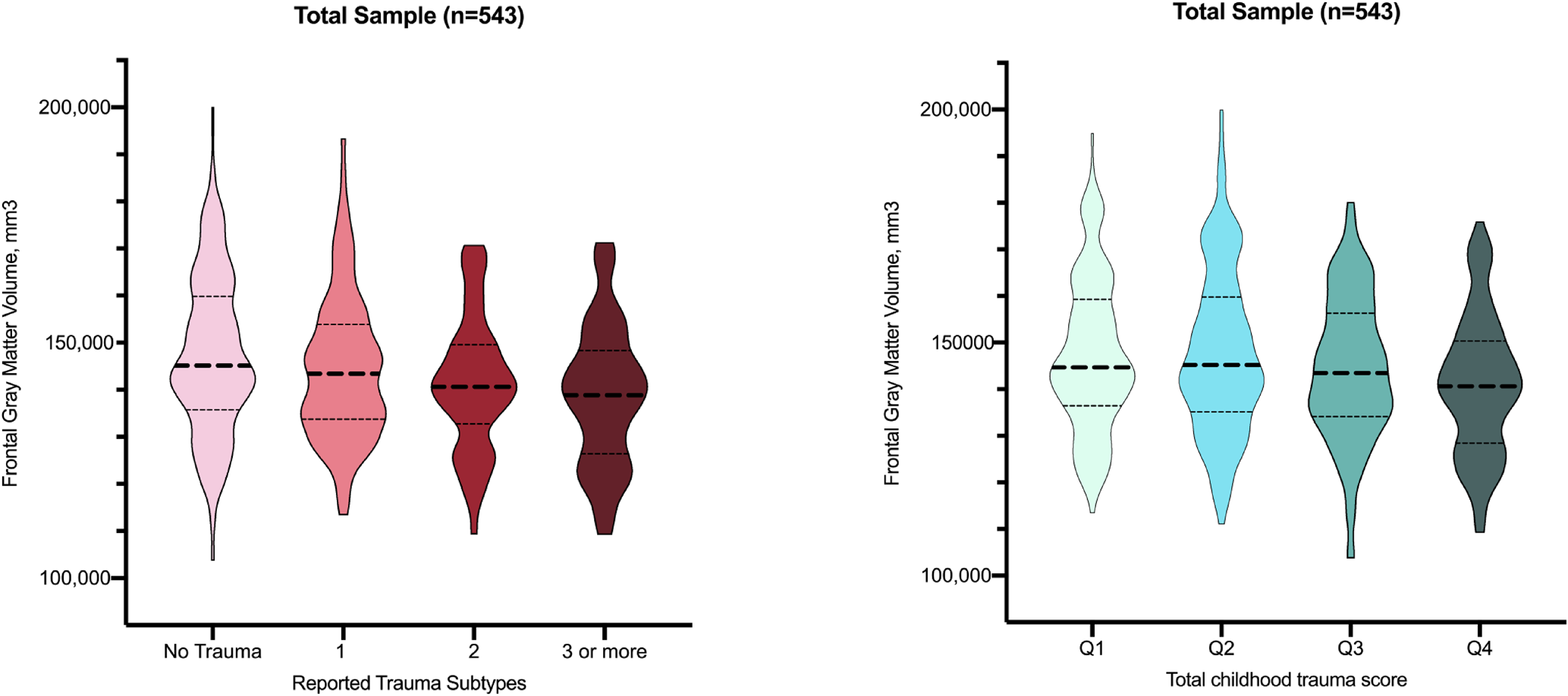
Fig. 2. Violin plots depicting frontal gray matter volume across categories of reported trauma subtypes and cumulative trauma score in the total sample.

Fig. 3. Violin plots depicting frontal gray matter volume across categories of reported trauma subtypes (a) and cumulative trauma score (b) within the three subgroups.
Discussion
Exploring both cortical and subcortical brain regions in the largest transdiagnostic sample to date, we found that childhood trauma was associated with reduced gray matter volume in the frontal lobe, while no trauma-related volume reductions were observed in the hippocampus or amygdala. Sensitivity analyses showed that the right medial orbitofrontal, paracentral, superior frontal regions and the left precentral region specifically contributed to this effect. These frontal trauma-related reductions may be shared across diagnostic groups, as no trauma-by-group interaction effects were present. Ranked-analyses confirmed more pronounced frontal gray matter reduction in those reporting multiple forms of childhood trauma and across quartiles of cumulative trauma severity. Similar dose-response patterns were also significant within the subgroups of bipolar disorder patients and healthy controls.
The suggestion that the frontal regions are particularly sensitive to childhood trauma is supported by animal studies (Schubert, Porkess, & Dashdorj, Reference Schubert, Porkess and Dashdorj2009), showing structural changes in prefrontal dendrites after 1 week of induced stress (e.g. maternal separation or isolation rearing), or even a single traumatic incident, while changes in the hippocampus are observed only after enduring stress for several weeks (Arnsten, Reference Arnsten2009). Studies in maltreated children and adolescent samples have consistently shown frontal gray matter reductions, rather than hippocampal or amygdala reductions (De Brito et al., Reference De Brito, Viding, Sebastian, Kelly, Mechelli, Maris and McCrory2013; Edmiston et al., Reference Edmiston, Wang, Mazure, Guiney, Sinha, Mayes and Blumberg2011; Hanson et al., Reference Hanson, Chung, Avants, Shirtcliff, Gee, Davidson and Pollak2010; Kelly et al., Reference Kelly, Viding, Wallace, Schaer, De Brito, Robustelli and Mccrory2013). Results from prior adult whole-brain studies in smaller samples, including patients as well as healthy subjects, also support a dose-response effect of trauma on the frontal lobe (Lim et al., Reference Lim, Radua and Rubia2014; Paquola et al., Reference Paquola, Bennett and Lagopoulos2016). Moreover, an ENIGMA study with the largest multi-center sample to date (n = 3036, healthy controls and patients with major depressive disorder) (Frodl et al., Reference Frodl, Janowitz, Schmaal, Tozzi, Dobrowolny, Stein and Jahanshad2017) investigated the link between childhood adversity and subcortical brain volume and did not replicate previous reports on trauma-related hippocampal or amygdala reductions either (Lim et al., Reference Lim, Radua and Rubia2014; Paquola et al., Reference Paquola, Bennett and Lagopoulos2016).
Although brain functioning related to cognition and social-emotional behavior is complex and distributed across remote brain regions, the frontal lobe is generally implicated to support top-down regulations of affect, motivational processing and social-emotional behaviors as well as higher-order cognition (Bonelli & Cummings, Reference Bonelli and Cummings2007; Carrion & Wong, Reference Carrion and Wong2012; Dolan, Reference Dolan2007; Lim et al., Reference Lim, Radua and Rubia2014; Menon, Reference Menon2011). Trauma-related cognitive and affective deficits may be mediated by structural brain alterations, predominantly in the frontal cortex. A link between early maltreatment and affective problems as well as cognitive deficits has consistently been shown in patient samples and healthy cohorts (Barzilay et al., Reference Barzilay, Calkins, Moore, Wolf, Satterthwaite, Cobb Scott and Gur2019; Gould et al., Reference Gould, Clarke, Heim, Harvey, Majer and Nemeroff2012; McCrory, De Brito, & Viding, Reference McCrory, De Brito and Viding2011; Pechtel & Pizzagalli, Reference Pechtel and Pizzagalli2011; Wilson, Hansen, & Li, Reference Wilson, Hansen and Li2011). Our group previously reported that higher trauma severity is associated with reduced adaptive stress reactivity (Begemann, Stotijn, Schutte, Heringa, & Sommer, Reference Begemann, Stotijn, Schutte, Heringa and Sommer2017), high levels of neuroticism (So, Begemann, Gong, & Sommer, Reference So, Begemann, Gong and Sommer2016), and lower inhibitory control (Begemann, Daalman, Heringa, Schutte, & Sommer, Reference Begemann, Daalman, Heringa, Schutte and Sommer2016; Begemann, Heringa, & Sommer, Reference Begemann, Heringa and Sommer2016). Trauma-susceptible brain regions can be particularly impacted by adverse events during so called ‘sensitive periods’ (Anda et al., Reference Anda, Felitti, Bremner, Walker, Whitfield, Perry and Giles2006; Sánchez et al., Reference Sánchez, Ladd and Plotsky2001). Notably, the frontal lobe matures relatively late and its protracted development could make this region vulnerable to negative environmental influences for a longer time than other regions with more rapid maturation. Suboptimal functioning may not become apparent until full brain maturation in late adolescence or early adulthood, which is the time when bipolar disorder and schizophrenia-spectrum disorder are typically diagnosed (Bachevalier & Loveland, Reference Bachevalier and Loveland2006).
An important finding of the current study is that these trauma-related frontal gray matter reductions were observed in a transdiagnostic sample of healthy individuals, bipolar disorder patients and schizophrenia-spectrum disorder patients, without significant trauma-by-group interactions. This could indicate that trauma-related reductions in the frontal (sub)regions are shared across psychiatric patients, independent of diagnosis. In healthy individuals, the neurobiological effects of trauma have been described as being more subtle (Souza-Queiroz et al., Reference Souza-Queiroz, Boisgontier, Etain, Poupon, Duclap, D'Albis and Houenou2016), with lower trauma rates and smaller variance compared to patient cohorts, requiring large samples to demonstrate these effects. Both our ranked analyses revealed a clear pattern of more pronounced frontal gray matter reduction in healthy individuals reporting multiple forms of childhood trauma and across quartiles of cumulative trauma severity. While few studies that investigated healthy subjects extended their search beyond the hippocampal and amygdala regions, some previous studies have reported trauma-related frontal gray matter reductions in healthy adults (n = 145) and healthy adolescents (n = 42) (Dannlowski et al., Reference Dannlowski, Stuhrmann, Beutelmann, Zwanzger, Lenzen, Grotegerd and Kugel2012; Edmiston et al., Reference Edmiston, Wang, Mazure, Guiney, Sinha, Mayes and Blumberg2011).
The present data provide strong evidence that childhood trauma contributes to the neurobiological changes commonly observed in psychiatric disorders. The categorical dose-response patterns were clearly observed in the bipolar disorder patients. In the schizophrenia-spectrum disorder subgroup, an identical association was found between more pronounced frontal gray matter reductions in individuals reporting multiple forms of trauma, with a more subtle pattern for quartiles of overall trauma rates – yet our subgroup of 79 patients appeared underpowered to demonstrate these associations (May & Looney, Reference May and Looney2020). Previous neuroimaging studies have shown frontal gray matter alterations in bipolar disorder (Duarte et al., Reference Duarte, Neves, Albuquerque, De Souza-Duran, Busatto and Corrêa2016; Souza-Queiroz et al., Reference Souza-Queiroz, Boisgontier, Etain, Poupon, Duclap, D'Albis and Houenou2016), schizophrenia-spectrum disorder (Hoy et al., Reference Hoy, Barrett, Shannon, Campbell, Watson, Rushe and Mulholland2012; Sheffield, Williams, Woodward, & Heckers, Reference Sheffield, Williams, Woodward and Heckers2013), major depression disorder (Lu et al., Reference Lu, Xu, Cao, Yin, Gao, Wang and Li2019; Vythilingam et al., Reference Vythilingam, Heim, Newport, Miller, Anderson, Bronen and Charney2002), posttraumatic stress disorder (O'Doherty et al., Reference O'Doherty, Tickell, Ryder, Chan, Hermens, Bennett and Lagopoulos2017), borderline personality disorder (Brunner et al., Reference Brunner, Henze, Parzer, Kramer, Feigl, Lutz and Stieltjes2010), and substance use disorder (Bachi et al., Reference Bachi, Parvaz, Moeller, Gan, Zilverstand, Goldstein and Alia-Klein2018; Van Dam, Rando, Potenza, Tuit, & Sinha, Reference Van Dam, Rando, Potenza, Tuit and Sinha2014). An overlap in frontal gray matter loss has been demonstrated when directly comparing patients with bipolar disorder and those with a schizophrenia-spectrum disorder (Arnone et al., Reference Arnone, Cavanagh, Gerber, Lawrie, Ebmeier and McIntosh2009), but also when evaluating bipolar disorder v. depression (Wise et al., Reference Wise, Radua, Via, Cardoner, Abe, Adams and Périco2017), and depression v. borderline personality disorder (Depping et al., Reference Depping, Wolf, Vasic, Sambataro, Thomann and Wolf2016). Thus, the so-called p-factor (Caspi et al., Reference Caspi, Houts, Belsky, Goldman-Mellor, Harrington, Israel and Poulton2014; Selzam, Coleman, Caspi, Moffitt, & Plomin, Reference Selzam, Coleman, Caspi, Moffitt and Plomin2018), reflecting the general effect of having a psychiatric illness including shared structural brain abnormalities across disorders (Opel et al., Reference Opel, Goltermann, Hermesdorf, Berger, Baune and Dannlowski2020; Parker et al., Reference Parker, Patel, Jackowski, Pan, Salum, Pausova and Paus2020), may overlap (perhaps for a large part) with the ‘t-factor’ reflecting the independent impact of childhood trauma.
Strengths and limitations
The current study has notable strengths, including its transdiagnostic design and extending the search for trauma-related gray matter alterations in brain regions other than the hippocampus and amygdala. Ideally, we would have implemented a fully data-driven approach based on individual brain regions. To ensure statistical power, we chose to conduct a lobe-wise analysis and follow up on significant results with explorative and uncorrected analyses to examine which individual regions would drive a particular result. Moreover, the three groups differed in age and gender distribution, in part because these are disease specific variables. Given the absence of trauma-by-group interactions in the total sample and the fact that we found a similar association between trauma and frontal brain volume across two out of three subgroups, suggests that age and gender effects do not to limit the interpretation of our current main findings. Moreover, we could not account for the plausible effect of cumulative medication use on gray matter volume, but instead evaluated current medication status. We also note that two different structured interviews were used to confirm clinical diagnosis in all included patients (SCID and CASH), their treating physician had already established diagnosis prior to enrollment. Furthermore, responses to the retrospective self-report questionnaire measuring childhood trauma may have been influenced by the inability of respondents to remember such experiences and subjective perception of the exposure to trauma. However, the CTQ-SF is currently indicated as one of the most reliable instruments for evaluating childhood trauma in healthy individuals as well as patients and our found trauma rates are in line with previous reports (Álvarez et al., Reference Álvarez, Roura, Osés, Foguet, Solà and Arrufat2011; Bernstein et al., Reference Bernstein, Stein, Newcomb, Walker, Pogge, Ahluvalia and Zule2003; Devi et al., Reference Devi, Shahwan, Teh, Sambasivam, Zhang, Lau and Subramaniam2019; Schäfer & Fisher, Reference Schäfer and Fisher2011; Wiersma et al., Reference Wiersma, Hovens, van Oppen, Giltay, van Schaik, Beekman and Penninx2009). Notably, the timing of maltreatment during childhood is not specifically assessed by the CTQ. The neurobiological effects on the brain, particularly limbic structures (Herzog et al., Reference Herzog, Thome, Demirakca, Koppe, Ende, Lis and Bohus2020), may depend on the timing of these experiences, which could be relevant for our null-findings regarding limbic structures. Important goals for future work should include setting up longitudinal cohort studies and analyzing large-scale transdiagnostic international consortium data sets.
In conclusion, our findings indicate that the frontal regions are most sensitive to the impact of childhood trauma and that these trauma-related frontal reductions are independent from psychiatric comorbidity. The frontal areas develop relatively late and the long window of high neuroplasticity may render these areas more vulnerable to adverse environmental circumstances during development. Emotional and cognitive deficits seen in individuals with a traumatic youth across diagnoses may at least partly stem from these frontal volume alterations and contribute to the development of psychopathology.
Supplementary material
The supplementary material for this article can be found at https://doi.org/10.1017/S0033291721002087
Trial registration
The study Simvastatin for recent-onset psychosis is registered in ClinicalTrails.gov (NCT01999309) and EudraCT (2013-000834-36).
Acknowledgments
We wish to thank all participants for their contribution and study team members for helping with data acquisition. We thank Dr Rachel Brouwer for her statistical input.
Financial support
The authors have no conflicts of interest relating to this article. The Spectrum study was funded by two personal grants for Professor Dr Sommer: De Nederlandse Organisatie voor Wetenschappelijk Onderzoek (NWO; Dutch Scientific Research Organization)/ZonMW Clinical Fellowship 2009 (grant number 4000703-97-270) and NWO/ZonMW Innovation Impulse (Vidi) 2009 (grant number 017.106.301). The Simvastatin for recent-onset psychosis is supported by the Stanley Medical Research Institute (to Prof. Dr Iris Sommer), grant number 12T-008 and is part of the research program TOP financed by NWO, grant number 40-00812-98-12154. The Bipolar Genetics study received funding from the National Institute of Mental Health, grant number R01MH090553 to Dr M. Boks.
Conflict of interest
The authors have declared that there are no conflicts of interest in relation to the subject of this study.