Introduction
Aedes aegypti L. is a widespread mosquito responsible for the transmission of ever-increasing infections causing extensive though variable degree of health hazards in the world, especially in tropical and sub-tropical regions. In several countries, Aedes-borne disease, dengue, has become a principal health concern due to worrisome rise to 390 million annual dengue infections with 96 million clinical manifestations (Bhatt et al., Reference Bhatt, Gething, Brady, Messina, Farlow, Moyes, Drake, Brownstein, Hoen, Sankoh, Myers, George, Jaenisch, Wiliam Wint, Simmons, Scott, Farrar and Hay2013). India has recorded a total of 39,419 dengue cases and 56 deaths in year 2020 and 39,419 suspected cases of Chikungunya (NVBDCP, 2021a, 2021b).
In the absence of vaccines and adequate medication, mosquito-borne diseases are primarily kept under check via mosquito management, at larval as well as adult stage. The traditional ways of interventions, such as use of mosquito bed nets, window screens, etc., are still in practice widely. Yet, application of chemical-based measures is on rampant rise to manage complicated-resistant mosquitoes quickly and effectually (Liu et al., Reference Liu, Xu, Zhu and Zhang2006; Kumar et al., Reference Kumar, Thomas, Samuel, Saghal, Verma and Pillai2009). Various groups of chemical toxicants have been used against mosquitoes; however, the negative impact of these on the surroundings, and non-target organisms along with the appearance of high insecticide resistance levels among mosquitoes has caused concerns (Bonner et al., Reference Bonner, Coble and Blair2007; Moore et al., Reference Moore, Cooper, Smith, Cullum, Knight, Locke and Bennett2009). Several countries have reported insecticide resistance in Ae. aegypti, including India (Kushwah et al., Reference Kushwah, Dykes, Kapoor, Adak and Singh2015), Brazil (Lima et al., Reference Lima, Paiva, de Araújo, da Silva, da Silva, de Oliveira, Santana, Barbosa, de Paiva Neto, Goulart and Wilding2011), China (Li et al., Reference Li, Kaufman, Xue, Zhao, Wang, Yan, Guo, Zhang, Dong, Xing and Zhang2015), Colombia (Fonseca-González et al., Reference Fonseca-González, Quiñones, Lenhart and Brogdon2011), Malaysia (Ishak et al., Reference Ishak, Jaal, Ranson and Wondji2015) and Thailand (Yanola et al., Reference Yanola, Somboon, Walton, Nachaiwieng, Somwang and Prapanthadara2011); and revealed metabolic detoxification and decreased sensitivity of insecticide-target proteins as the prime cause for the resistance (Bansal et al., Reference Bansal, Barna and Chaudhry2012; Yang and Liu, Reference Yang and Liu2014).
Among the new approaches and chemical interventions, neonicotinoids, synthetic derivatives of nicotine, are one of the fastest-growing insecticides and considered a safe replacement of the conventional insecticides currently used in the mosquito management. These chemicals induce toxicity in the target insect pest by interacting with nicotinic acetylcholine receptors (nAChRs) of the insect nervous system mediating fast cholinergic transmission (Li et al., Reference Li, Zhang, Reid, Xu, Dong and Liu2012). Acetamiprid, a neonicotinoid, reacts with nAChRs located in the post-synaptic neural dendrites of central nervous system, ganglia and muscular junctions imparting contact as well as stomach toxicity (Jian-chu et al., Reference Jian-chu, Tian-ci, Jia-an and Xiao-gang2002; Kimura-Kuroda et al., Reference Kimura-Kuroda, Komuta, Kuroda, Hayashi and Kawano2012; Sanche-Bayo, Reference Sanchez-Bayo2012). It has been reported that acetamiprid is selectively toxic to the insects, does not bio-accumulate in the sediments and fish, and is safer to the environment relative to the other insecticides in use (Ambrose, Reference Ambrose2003). Despite a few reports of neonicotinoid resistance and cross-resistance in Bemisia tabaci (Gennadius) (Horowitz et al., Reference Horowitz, Kontsedalov and Ishaaya2004), Musca domestica (Kristensen and Jespersen, Reference Kristensen and Jespersen2008), Frankliniella occidentalis (Pergande) (Gao et al., Reference Gao, Ma, Shan and Wu2014) and Leptinotarsa decemlineata (Say) (Mota-Sanchez et al., Reference Mota-Sanchez, Hollingworth, Grafius and Moyer2006); reports of such resistance are negligible in Ae. aegypti. Yet, all insects including mosquitoes have the capability to develop resistance to any toxicant, sooner or later. Therefore, it becomes important to understand the mechanism of resistance to a particular insecticide based on which resistance management strategies can be devised.
A key module of resistance management is based on the use of synergists which may reduce as well as reverse the development of resistance development in insects. Piperonyl butoxide (PBO) is a well-known insecticide synergist which impedes the cytochrome P450-mediated metabolism of an insecticide and enhances its toxicity. Several insecticides, majorly synthetic pyrethroids, used against agricultural or public health pests contain PBO as an active ingredient (Cetin et al., Reference Cetin, Kocak, Oz, Koc, Polat and Arikan2019). Yet effective use of PBO as a synergist to other insecticide groups is being attempted to develop successful resistance management programme (Khan et al., Reference Khan, Akram and Shad2014).
Current study investigates the development of acetamiprid resistance in Ae. aegypti larvae and possible use of PBO as a synergist of acetamiprid to reduce or reverse the speed of development of resistance. Since PBO is an inhibitor of cytochrome P450 monooxygenases, the study will help to elucidate the involvement of metabolic enzyme (CYP450) in the development of acetamiprid resistance in Ae. aegypti and design an effective management strategy.
Materials and methods
Culture of Aedes aegypti L.
Pure line of Ae. aegypti has been maintained in the Rearing Unit of Acharya Narendra Dev College, New Delhi, India since last 10 years; without subjection to any insecticide selection pressure. The rearing conditions have been set at 28 ± 1°C temperature, 80 ± 5% relative humidity and 12 h:12 h (light:dark) photo-regime to ensure optimal growth, feeding, mating and oviposition (Warikoo et al., Reference Warikoo, Ray, Sandhu, Samal, Wahab and Kumar2012; Samal and Kumar, Reference Samal and Kumar2018). The pure line of Ae. aegypti, marked as the Base-Line, was considered as the parent susceptible strain (PS) (Samal et al., Reference Samal, Panmei, Lanbiliu and Kumar2020). General hygiene and sterility during rearing has been ensured to prevent infections, pest attack and infestations by potential predators and parasites, roaches and book lice, and to protect egg stocks and other stages (Zheng et al., Reference Zheng, Zhang, Damiens, Yamada and Gilles2015).
Adult Ae. aegypti were kept in the 45 × 40 × 40 cm clothed cages and fed on sugary juice of deseeded water-soaked raisins. Female mosquitoes were given blood meals on alternate days, for at least an hour, to ensure adequate egg maturation. Eggs were collected in an ovitrap; consisting of a small enamel/plastic bowl lined with Whatman filter paper strips and filled two-third with dechlorinated water. The egg strips were then transferred into enamel/plastic trays (25 × 30 × 5 cm) filled with at least 1.5–2.0 litres of dechlorinated water. A total of 200 larvae were reared in each tray and were provided with an artificial diet (15 mg) of powdered dog biscuits and active yeast in a ratio of 3:1 by weight (Warikoo et al., Reference Warikoo, Ray, Sandhu, Samal, Wahab and Kumar2012). Water was changed every day to avoid the formation of any froth on its surface.
Chemicals used
Technical grades of acetamiprid (99.9% purity) and PBO (99% purity) were procured from M/s Sigma-Aldrich, India. Desired concentrations were prepared in ethanol (eMerck) and stored at 4°C.
Larvicidal efficacy and larval selection with acetamiprid
The toxic level of acetamiprid was assessed against early fourth instars of the PS of Ae. aegypti, based on standard WHO protocol (Samal and Kumar, Reference Samal and Kumar2021).
The larval selection of the PS strain was carried out at early fourth instar stage by imparting the selection pressure of acetamiprid at LC90 level as described in our earlier reports (Samal and Kumar, Reference Samal and Kumar2021). Five batches of healthy instars, each batch containing 200 larvae, were subjected to the insecticide pressure for a day. Survived larvae were strained, cleaned and reared to adults, the generation marked as acetamiprid-selected strain (ACSF – Acetamiprid Larval-Selected Filial).
The acetamiprid selection was continued till 15 successive generations (ACSF-1 to ACSF-15). The resistance level to acetamiprid induced was estimated in each generation according to equation 1 (Kumar et al., Reference Kumar, Thomas, Sahgal, Verma, Samuel and Pillai2002; Samal and Kumar, Reference Samal and Kumar2021).

Larvicidal efficacy and larval selection with acetamiprid synergized with PBO
Three different combinations of acetamiprid and PBO (1:1, 1:5 and 1:10) were evaluated for their larvicidal efficacy against ACSF-10 strain of Ae. aegypti. The bioassays were run as per the protocol described in section ‘Larvicidal efficacy and larval selection with acetamiprid’. Based on the efficacy and aim to use less toxic component in the combination, the acetamiprid added with PBO in 1:10 ratio was selected for further studies.
The early fourth instars of ACSF-10 population were selected with synergized acetamiprid (1:10) at LC90 value and the resultant strain was marked as APSF (Acetamiprid + PBO Larval-Selected Filial). The selection pressure was continued for next five successive generations to obtain APSF-15 strain.
The synergistic efficacy of PBO when combined with acetamiprid was evaluated by calculating the synergistic ratio and per cent suppression of acetamiprid resistance in each selected generation (equation 2).

SR > 1 denotes synergistic effect; SR < 1 shows antagonistic effect; SR = 1 signifies additive effect.
Estimation of CYP450 monooxygenase level in selected strains
Larvae of the following strains were selected for the estimation of the level of cytochrome P450 monooxygenase.
(a) PS: Parent susceptible strain
(b) ACSF-5: PS strain selected with acetamiprid at larval stage for five successive generations
(c) ACSF-10: PS strain selected with acetamiprid at larval stage for ten successive generations
(d) ACSF-15: PS strain selected with acetamiprid at larval stage for 15 successive generations
(e) APSF-15: ACSF-10 strain selected with acetamiprid + PBO (1:10) at larval stage for five successive generations
Synergistic ratios-based estimation of CYP450 monooxygenase level in selected strains
The SR-based monooxygenase levels in vivo were estimated in the strains according to the methodology of Osman and Brindley (Reference Osman and Brindley1981) as adopted by Kumar et al. (Reference Kumar, Thomas and Pillai1991) using synergistic difference (SD) and per cent dependency of mosquitoes on monooxygenase (%D) as the parameters. The observed synergistic differences (OSD) were calculated as per equation 3.

The expected SD (ESD) value being different from the observed SD value was calculated from a regression line expressing the linear relationship between LC50 value of acetamiprid and synergistic difference (Osman and Brindley, Reference Osman and Brindley1981). The calculation was made according to the following equation 4:

The calculated ESD value indicates the measurement which would have been if the mosquitoes were primarily dependent upon the monooxygenase system. Hence, this deviation expresses the relative dependency of mosquitoes upon monooxygenases and was calculated as follows (equation 5):

Biochemical estimation of CYP450 monooxygenase levels in selected strains
A total of newly emerged early fourth instars (100 larvae in five batches; each batch of 20 replicates) from PS, ACSF-5, ACSF-10, ACSF-15 and APSF-15 were selected for the CYP450 monooxygenase level estimation to assess the correlation between enzyme and acetamiprid-resistance level. The methodology of Brogdon et al. (Reference Brogdon, McAllister and Vulule1997) and WHO (1998) modified by Kona et al. (Reference Kona, Kamaraju, Donnelly, Bhatt, Nanda, Chourasia, Swain, Suman, Uragayala, Kleinschmidt and Pandey2018) was adopted for the assay. Each larva was homogenized in 200 μl of ice-cold autoclaved water with the help of a micro-homogenizer. The homogenate was spun at 17,000 × g for 30 s in a refrigerated microfuge (Hanil Science Smart R17 micro refrigerated centrifuge) and supernatant was used for monooxygenase estimation. The volume of 20 μl of the supernatant of each larval homogenate was pipetted out in separate microtiter plate wells. To each well, 80 μl of 0.625 M potassium phosphate buffer was added to establish a reaction system. The mixture was supplemented with 200 μl of solution comprising one part of 8 mM methanolic solution of tetramethyl benzidine and three parts of 0.25 M sodium acetate buffer (pH 5.0) followed by addition of 25 μl of 0.88 M hydrogen peroxide (Brogdon et al., Reference Brogdon, McAllister and Vulule1997; WHO, 1998; Kona et al., Reference Kona, Kamaraju, Donnelly, Bhatt, Nanda, Chourasia, Swain, Suman, Uragayala, Kleinschmidt and Pandey2018). The final solution was incubated at room temperature for 10–15 min (Kona et al., Reference Kona, Kamaraju, Donnelly, Bhatt, Nanda, Chourasia, Swain, Suman, Uragayala, Kleinschmidt and Pandey2018). The absorbance was measured at 620 nm to estimate the concentration of monooxygenase in each larval strain.
Results
Larvicidal efficacy and larval selection with acetamiprid
The selection of Ae. aegypti with acetamiprid for 15 successive generations, at early fourth instar stage resulted into a continued decrease in susceptibility to acetamiprid. Out of 1000 larvae tested (200 larvae in five batches), only 157 larvae survived the selection pressure. The larvae showed reduced susceptibility by 94.93% in ACSF-10 and by 97.28% in ACSF-15 as compared to the PS larvae. The tolerance level of the larvae increased to 8.83-fold in ACSF-5 (P < 0.05) and 19.74-fold in ACSF-10 (P < 0.05) (reported earlier in Samal and Kumar, Reference Samal and Kumar2021) which drastically rose to 36.71-fold in ACSF-15 (P < 0.05) (table 1). The gradual right shift of the d-m-r (dosage mortality regression) lines of ACSF strains denoting the speed of acetamiprid resistance development in larvae shows maximum shift in last five generations indicating the rapid development of resistance (fig. 1).

Figure 1. Dosage-mortality regression lines on selection of Aedes aegypti early fourth instars with acetamiprid for successive generations. PS, parent susceptible strain; ACSF-5, Acetamiprid Larval-Selected Filial-5; ACSF-10, Acetamiprid Larval-Selected Filial-10; ACSF-15, Acetamiprid Larval-Selected Filial-15.
Table 1. LC50 and LC90 (in mg litre−1) values of acetamiprid against early fourth instars of Aedes aegypti when selected with acetamiprid for 15 successive generations

PS, Parental Strain; ACSF, Acetamiprid Larval-Selected Filial; RR, resistance ratio; LC50, lethal concentration at which 50% larvae are killed; LC90, lethal concentration at which 90% larvae are killed; SEM, standard error of mean; df, degree of freedom; RR, resistance ratio; SR, synergistic ratio; LC values in each column followed by different letters are significantly different P < 0.05; one-way ANOVA followed by Tukey's all pair wise multiple comparison test.
Larvicidal efficacy and larval selection with acetamiprid synergized with PBO
Larvicidal bioassay with synergized acetamiprid
Larvicidal bioassay of ACSF-10 early fourth instars with acetamiprid combined with PBO in three different ratios (1:1, 1:5 and 1:10) enhanced the toxic effects of acetamiprid and reduced the developed resistance. The maximum synergistic effect was evident with acetamiprid + PBO (1:10) drastically depleting the acetamiprid resistance in ACSF-10 larvae from 19.74- to 1.24-fold (table 2). Other two combinations of synergized acetamiprid, 1:1 and 1:5, decreased the resistance ratio by 6.22- and 1.72-fold, respectively (P < 0.05) (table 2). Since the synergized acetamiprid (1:10) imparted 1.39 times higher synergistic effects than the 1:5 combination and 5.02 times more than 1:1 ratio, further selection of ACSF-10, for acetamiprid resistance management, was held with acetamiprid and PBO in 1:10 ratio.
Table 2. Larval LC50 and LC90 (in mg ml−1) of ACSF-10 strain of Aedes aegypti when assayed with acetamiprid combined with PBO in different ratios
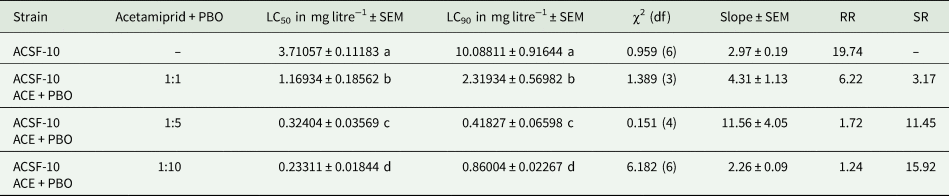
ACSF, Acetamiprid Larval-Selected Filial generation; ACE, acetamiprid; PBO, piperonyl butoxide; LC50, lethal concentration at which 50% larvae are killed; LC90, lethal concentration at which 90% larvae are killed; SEM, standard error of mean; df, degree of freedom; RR, resistance ratio; SR, synergistic ratio. LC values in each column followed by different letters are significantly different P < 0.05; one-way ANOVA followed by Tukey's all pair wise multiple comparison test.
Selection of ACSF-10 larvae with acetamiprid + PBO (1:10)
A reversion in the acetamiprid resistance levels as well as reduction in the rate of resistance development was observed on continuous selection of the early fourth instar of ACSF-10 with acetamiprid and PBO (1:10). The APSF population developed insignificant levels of acetamiprid resistance, just 1.24-fold in APSF-11 and 1.72-fold in APSF-12, as against 16.22- and 23.66-fold on selection with acetamiprid alone (P < 0.05). Further selection with synergized acetamiprid reversed the resistance to 1.35-fold in APSF-15 (table 3). Notably, the selections with synergized acetamiprid led to 86.71–96.33% suppression in acetamiprid resistance (table 3, fig. 2).

Figure 2. Resistance ratios in successive generations of Aedes aegypti larvae. ACSF, parent susceptible strain selected with acetamiprid alone (ACSF) for 15 generations; APSF, ACSF-10 strain selected with acetamiprid + PBO(1:10) for next five generations.
Table 3. Larval LC50 and LC90 (in mg litre−1) of ACSF-10 strain of Aedes aegypti when selected with acetamiprid alone and acetamiprid + PBO (1:10) for five successive generations

ACSF, Acetamiprid Larval-Selected Filial; APSF, Acetamiprid + PBO Larval-Selected Filial; LC50, lethal concentration at which 50% larvae are killed; LC90, lethal concentration at which 90% larvae are killed; SEM, standard error of mean; df, degree of freedom; RR, resistance ratio; SR, synergistic ratio; LC values in each row followed by different letters are significantly different P < 0.05; one-way ANOVA followed by Tukey's all pair wise multiple comparison test.
Synergistic ratios-based estimation of CYP450 monooxygenase level in selected strains
The Ae. aegypti APSF strains showed %monooxygenase dependency ranging from 86.71 to 96.72%; the minimum dependency observed in APSF-13 (table 4). A positive correlation (r = 1.48–3.06) was recorded in the monooxygenase activity in the APSF strains and the respective LC50 value.
Table 4. Per cent dependency on monooxygenase in Aedes aegypti early fourth instar when selected with acetamiprid + PBO (1:10)

ACSF, Acetamiprid Larval-Selected Filial; APSF, Acetamiprid + PBO Larval-Selected Filial; OSD, observed synergistic ratio; ESD, expected synergistic ratio; LC50, lethal concentration at which 50% larvae are killed; SEM, standard error of mean. LC values followed by different letters are significantly different P < 0.05; one-way ANOVA followed by Tukey's all pair wise multiple comparison test.
Biochemical estimation of CYTP450 monooxygenase levels in selected strains
The estimation of monooxygenases in PS revealed 0.0036 (±0.0002) mmoles mg−1 of protein in different larval groups, while the total protein in the larval body was 3.8876 μg μl−1 (table 5). In comparison, the ACSF larvae displayed upsurge in the enzyme levels, 2.42-fold in ACSF-5 (P < 0.05) and 2.68-fold in ACSF-10 (P < 0.05). A similar elevation in the monooxygenase activity (2.87-fold) was observed in ACSF-15 which was 1.07-fold higher than that in PS and ACSF-10, respectively. Alternatively, a sudden and significant decline in the monooxygenase activity was observed in APSF-15 with respect to PS (9.28%) and ACSF-15 (57.95%) indicating the role of monooxygenases in imparting acetamiprid resistance to Ae. aegypti larvae. The box plot distribution of monooxygenase activity in all the five strains implied the heterogeneity in the population of each strain; more variation observed in ASCF-5 and ACSF-10 in comparison to the ACSF-15 and APSF-15 (fig. 3).

Figure 3. Box plot distribution of range of P450 monooxygenase (OD min−1 μg−1 of protein) in the larvae of PS, ACSF-5, ACSF-10, ACSF-15 and APSF-15 strains of Aedes aegypti. Middle line between the boxes represents the median; upper and lower boxes represent the 25 and 75 percentiles of the data; whiskers represent the standard error of the mean; the dots above and below the whiskers represent the outliers. PS, parent strain; ACSF-5, Acetamiprid Larval-Selected Filial-5; ACSF-10, Acetamiprid Larval-Selected Filial-10; ACSF-15, Acetamiprid Larval-Selected Filial-15; APSF-15, Acetamiprid + PBO Larval-Selected Filial-10.
Table 5. Level of monooxygenases in parent susceptible and selected strains of Aedes aegypti

PS, parental strain; ACSF, Acetamiprid Larval-Selected Filial; APSF, Acetamiprid + PBO Larval-Selected Filial; SEM, standard error of mean. Each strain had five replicates. Each replicate consisted of 20 larvae (N = 100 larvae). Figures in each column followed by different letters are significantly different (P < 0.05); one-way ANOVA followed by Tukey's all pair wise multiple comparison test.
The frequency distribution profiles of acetamiprid-selected larvae showed a drastic shift in the absorbance peak from 0.4 in PS to 1.0 in the selected larvae (ACSF-5) (fig. 4). In comparison to ACSF-5, 7% higher frequency of population in ACSF-10 and 20% more in ACSF-15 attained the peak. The profile also revealed the presence of high per cent of resistant individuals beyond the susceptible threshold in selected population, 80% in ACSF-5, 87% in ACSF-10 and 100% in ACSF-15. However, synergized acetamiprid-selected strain (APSF-15) did not show resistant individuals beyond the threshold level suggesting reversion of resistance (fig. 4).

Figure 4. Frequency distribution of absorbance values of P450 monooxygenase mmol min−1 mg−1 of protein in the PS, ACSF-5, ACSF-10, ACSF-15 and APSF-15 strains of Aedes aegypti. Susceptibility threshold based on maximum absorbance in PS strain. Shaded region represents the resistant population (beyond the threshold). N, number of larvae; RP, resistant population; PS, parent strain; ACSF-5, Acetamiprid Larval-Selected Filial-5; ACSF-10, Acetamiprid Larval-Selected Filial-10; ACSF-15, Acetamiprid Larval-Selected Filial-15; APSF-15, Acetamiprid + PBO Larval-Selected Filial-10.
Discussion
Insecticide resistance, considered a pre-adaptive phenomenon, has emerged as the greatest problem to control all insect groups including disease vectors. The prolonged and frequent usage of insecticides in various public health programmes, crop fields and domestic areas has eliminated susceptible individuals and selected resistant individuals resulting in the emergence of resistant strains (Uragayala et al., Reference Uragayala, Verma, Natarajan, Velamuri and Kamaraju2015). It is proposed that prior to experiencing high insecticide exposure, a few organisms can survive the stress due to altered genome and get selected post-exposure (Faucon et al., Reference Faucon, Dusfour, Gaude, Navratil, Boyer, Chandre, Sirisopa, Thanispong, Juntarajumnong, Poupardin and Chareonviriyaphap2015). These immune organisms carry the genetic variance to the successive generation contributing to the resistance gene pool. Gradual and sequential selection through inheritance increases the proportion of organisms possessing the resistance genes, alleles or polymorphisms. Ultimately, subjection to extended insecticide exposure outweighs the resistant organisms than the susceptible population.
Utilization of insecticides at a widespread scale resulting in resistance to that particular toxicant and cross-resistance to other insecticides has caused re-emergence of mosquito-borne disease in many parts of the world (Zaim and Guillet, Reference Zaim and Guillet2002). Consequently, neonicotinoids, considered relatively safe chemicals, are under exploration as the possible and efficient control interventions (Hemingway et al., Reference Hemingway, Field and Vontas2002). Though, a few lepidopterans, hemipterans and coleopterans have been recorded with neonicotinoid resistance (Liu et al., Reference Liu, Williamson, Landsdell, Denholm, Han and Millar2005; Qiong et al., Reference Qiong, Xu, Chen, Zhang, Jones, Devine, Gorman and Denholm2012), such resistance in mosquitoes, particularly to acetamiprid, is not yet cited in literature. Further, being approved by World Health Organization for its use in public health programmes (US-EPA, 2002), acetamiprid is being investigated as the probable control agent. The current study evaluated acetamiprid against Ae. aegypti larvae for imparting toxic effects. The possible development of acetamiprid resistance was assessed in Ae. aegypti and synergistic studies were carried out as a resistance management strategy.
Synergists, the inhibitors of the detoxifying enzymes, are known to inhibit the metabolic enzymes – primarily P450s and esterases; and enhance the toxicity of an insecticide (Lorini and Galley, Reference Lorini and Galley2000; Cetin et al., Reference Cetin, Kocak, Oz, Koc, Polat and Arikan2019). These compounds thus have been frequently employed to combat resistance and effectively control target pest species (Lorini and Galley, Reference Lorini and Galley2000). In fact, comparative assessment of the toxic impact of synergized and unsynergized insecticides on target population can recognize and conclude the detoxification mechanisms involved in the development of resistance to that particular insecticide. Synergistic studies with the pyrethroid-resistant Aedes, Anopheles and Culex species have strongly backed the role of metabolic detoxification in imparting resistance (Brogdon et al., Reference Brogdon, McAllister, Corwin and Cordon-Rosales1999; Enayati et al., Reference Enayati, Vatandoost, Ladonni, Townson and Hemingway2003; Liu et al., Reference Liu, Cupp, Micher, Guo and Liu2004; Cuamba et al., Reference Cuamba, Morgan, Irving, Steven and Wondji2010).
The commonly used insecticide synergists include PBO, S,S,S-tributyl phosphorotrithioate (DEF) and N-Octyl bicycloheptene dicarboximide (MGK-264). The combination of PBO with pyrethroids and organophosphates is recommended in insecticide formulations to reduce the insecticide concentration in vector control, thereby declining the risk of bioaccumulation. Consequently, many PBO-containing pesticide formulations have been used against a variety of vectors (Cetin et al., Reference Cetin, Demir, Kocaoglu and Kaya2010). However, till date and as per our knowledge, the efficacy of PBO on acetamiprid susceptibility against Ae. aegypti has not been evaluated though synergistic activity of PBO with imidacloprid has been reported against Ae. aegypti (Riaz et al., Reference Riaz, Chandor-Proust, Dauphin-Villemant, Poupardin, Jones, Strode, Régent-Kloeckner, David and Reynaud2013) and Cx. pipiens (Ahmed and Othman, Reference Ahmed and Othman2020).
Thus, the present study evaluated the potential role of PBO in reducing and reversing the acetamiprid resistance and designing an effective resistance management strategy in Ae. aegypti. After continuous laboratory selection for 15 consecutive generations with unsynergized acetamiprid, the larvae developed 36.71-fold resistance to acetamiprid. However, selection of ACSF-10 strain of Ae. aegypti with synergized insecticide (1:10) reduced and reversed the rate of resistance development significantly. This supports the overproduction of P450 monooxygenase in selected strains which was further advocated by the high per cent dependency on monooxygenases demonstrated by strains selected with synergized insecticides. Synergistic effects of PBO causing significant decline in the acetamiprid LC50 values of Ae. aegypti resistant strains suggest the role of CYP450s due to the involvement of monooxygenase-based metabolic detoxification mechanism in imparting acetamiprid resistance.
These results are in alignment with those obtained with a field strain of Cx. pipiens, 3.8–38.4-fold resistant to pyrethroids (Al-Sarar, Reference Al-Sarar2010). Selecting the resistant larvae with pyrethroids synergized with PBO suppressed >90% pyrethroid resistance demonstrating the role of microsomal oxidases in reducing the pyrethroid toxicity. Similarly, larval treatment of deltamethrin-resistant (4–21-fold) strains of Ae. aegypti, An. culicifacies, An. stephensi, An. vagus, Cx. tritaeniorhynchus and Cx. pipiens with deltamethrin + PBO (1:6) suppressed the resistance by 75–95% (WHO, 2016).
A few studies have revealed the synergism between neonicotinoids and PBO against public health pests like house fly, whereas this relationship has not been confirmed in the mosquitoes yet. Ma et al. (Reference Ma, Li, Zhang, Shan and Gao2017) showed that 78-fold imidacloprid-resistant population of housefly registered 3.34-fold synergism with PBO. Resistance to imidacloprid and other neonicotinoid, thiamethoxam, has also been suppressed by PBO-synergized insecticides in Danish populations of M. domestica (Markussen and Kristensen, Reference Markussen and Kristensen2010). In thiamethoxam-resistant strains of Musca collected from Pakistan, Khan et al. (Reference Khan, Akram, Iqbal and Naeem-Ullah2015) reported a significant synergism with S,S,S-tri-butylphosphorotrithioate and PBO resulting in respective 2.94- and 5.00-fold reversion in resistance.
The larval selection of Ae. aegypti with acetamiprid alone for 15 generations caused an elevation in P450 monooxygenase level by 2–3-fold. Similar inhibitory effects of cytochrome P450 on the toxicity of imidacloprid in housefly were reported by Ma et al. (Reference Ma, Li, Zhang, Shan and Gao2017). The association of elevated P450 monooxygenase with pyrethroid resistance has been deduced in various mosquito species; Ae. aegypti, An. stephensi and Cx. quinquefasciatus (Kumar et al., Reference Kumar, Thomas and Pillai1991); and An. stephensi and An. gambiae (Hemingway et al., Reference Hemingway, Miyamoto and Herath1991; Vulule et al., Reference Vulule, Beach, Atieli, Roberts, Mount and Mwangi1994; Brogdon et al., Reference Brogdon, McAllister and Vulule1997). The pyrethroid-resistant South African strain of An. funestus showed upregulation of primarily P450 monooxygenase system (Brooke et al., Reference Brooke, Kloke, Hunt, Koekemoer, Temu, Taylor, Small, Hemmingway and Coetzee2001; Wondji et al., Reference Wondji, Morgan, Coetzee, Hunt, Steen, Black, Hemingway and Ranson2007, Reference Wondji, Irving, Morgan, Lobo, Collins, Hunt, Coetzee, Hemingway and Ranson2009; Amenya et al., Reference Amenya, Naguran, Lo, Ranson, Spillings, Wood, Brooke, Coetzee and Koekemoer2008). High levels of permethrin resistance in Cx. pipiens has been solely conferred to P450-mediated detoxification (Hardstone et al., Reference Hardstone, Lazzbaro and Scott2009). Imidacloprid-resistant Drosophila showed increased expression of CYP6G1, indicating the involvement of CYP isozyme in detoxifying imidacloprid and perhaps other neonicotinoids (Daborn et al., Reference Daborn, Boundy, Yen and Pittendrigh2001).
Formulation of novel insecticides with unique mode of action is difficult as well as expensive necessitating to devise resistance management strategies in order to preserve the efficiency of pre-existing insecticides. Since, elucidation of the mechanisms governing insecticide resistance could be a dynamic step towards the creation of more effective and safer interventions to combat resistance, bring resistant populations below threshold and ultimately reduce the incidences of mosquito-borne diseases; current studies are of extreme implications.
Conclusion
Development of insecticide resistance in mosquitoes caused by continuous selection pressure has led to failure of vector control programmes necessitating employment of new chemicals in the fields. In the current study, selection pressure of acetamiprid on Ae. aegypti larvae for 15 generations caused development of considerable resistance which, however, could be reduced and reversed by selection with PBO synergized acetamiprid. It suggests the appearance of CYP450-mediated resistance in Ae. aegypti larvae which could be managed by synergistic approach. Use of synergized acetamiprid as a control intervention approach is recommended which is effective, safe and sustainable.
Data
Not applicable.
Acknowledgements
The authors are highly grateful to the Council of Scientific Research (CSIR), New Delhi, India for providing financial assistance to carry out the present investigations. We are also highly grateful to the Principal, Acharya Narendra Dev College for providing infrastructure and research facilities.
Author contributions
RRS conceived the idea, conducted the experiments and wrote the manuscript. SK designed and guided the experiments. RRS, KP and PL analysed the results and SK helped in the analysis. RRS and SK were involved in the finalization of manuscript.
Financial support
This research was supported by the contingent grant from Council of Scientific and Industrial Research, New Delhi, India (Award No. 08/529(0003)/2015-EMR-I).
Conflict of interest
None.
Ethical standards
Not applicable.
Consent for publication
Not applicable.
Code availability
Not applicable.