Introduction
Overfishing has adversely affected fish communities worldwide, with large and valuable target species being replaced by smaller, lower-value fishes in the so-called fishing-down process (Welcomme, Reference Welcomme1999, Reference Welcomme2001; Winemiller, Reference Winemiller2005; Castello et al., Reference Castello, McGrath, Hess, Coe, Lefebvre and Petry2013). In marine environments fishing pressure has caused the mean trophic level to decline through the replacement of large predators by small planktivorous fishes, and decreased the mean size and the age of first maturation of exploited fishes (Pauly et al., Reference Pauly, Christensen, Dalsgaard, Froese and Torres1998; Rochet, Reference Rochet1998). These changes to fish communities driven by high fishing pressure may ultimately alter the structure of food webs and the flux of energy and matter in ecosystems (Andersen & Pedersen, Reference Andersen and Pedersen2010).
Protected areas have been proposed as an efficient way to manage fisheries while simultaneously preserving biodiversity in marine ecosystems (PDT, 1990; Gell & Roberts, Reference Gell and Roberts2003). The density, biomass, species richness and size of fishes are all expected to increase within protected areas relative to surrounding areas (Halpern, Reference Halpern2003). The reduced fishing pressure inside protected areas also benefits large fishes of upper trophic levels, which are usually targeted by fisheries (Claudet, Reference Claudet2011). Besides being established by the government through a top-down approach, protected areas can be co-managed by local communities, with the aim of maintaining traditional livelihoods and the sustainability of natural resources (Lausche, Reference Lausche2011; Lopes et al., Reference Lopes, Silvano and Begossi2011; Campbell et al., Reference Campbell, Cinner, Ardiwijaya, Pardede, Kartawijaya and Mukmunin2012; Gupta et al., Reference Gupta, Kanagavel, Dandekar, Dahanukar, Sivakumar, Mathur and Raghavan2016a). Protected areas managed by local people have been successful in some marine environments, increasing the abundance of commercially exploited invertebrates, with ancillary positive effects on the abundance and diversity of non-exploited reef fishes (Gelcich et al., Reference Gelcich, Godoy, Prado and Castilla2008). Furthermore, increased fish stocks in protected areas can result in increased catches and earnings for local fishers (Lockwood et al., Reference Lockwood, Worboys and Kothari2006). However, in the case of freshwater ecosystems the impacts of fishing and the effects of protected areas are still poorly known (Nel et al., Reference Nel, Roux, Maree, Kleynhans, Moolman and Reyers2007; Suski & Cooke, Reference Suski and Cooke2007; Gupta et al., Reference Gupta, Kanagavel, Dandekar, Dahanukar, Sivakumar, Mathur and Raghavan2016a). As most protected areas have been designed to protect terrestrial ecosystems (Rodríguez–Olarte et al., Reference Rodríguez–Olarte, Taphorn and Lobón–Cerviá2011), they may fail to mitigate threats to fisheries (Rodríguez–Olarte et al., Reference Rodríguez–Olarte, Taphorn and Lobón–Cerviá2011; Abraham & Kelkar, Reference Abraham and Kelkar2012). With such an emphasis on terrestrial protected areas, freshwater fishes have often been disregarded in conservation planning in important biodiversity hotspots, such as in the Brazilian Amazon (Castello et al., Reference Castello, McGrath, Hess, Coe, Lefebvre and Petry2013) and in India (Gupta et al., Reference Gupta, Kanagavel, Dandekar, Dahanukar, Sivakumar, Mathur and Raghavan2016a,Reference Gupta, Nautiyal, Borgohain, Sivakumar, Mathur and Chadwickb).
The Amazon forest is the most protected biome in Brazil, with nearly 2.2 million km2 (43.9%) within protected areas, 66% of which are designated for sustainable use (Veríssimo et al., Reference Veríssimo, Rolla, Vedoveto and de Furtada2011; ICMBIO, 2015). Nevertheless, intensive fishing in the Amazon Basin has resulted in one fish species becoming threatened, and there is evidence of overexploitation of four other fish species (Castello et al., Reference Castello, McGrath, Hess, Coe, Lefebvre and Petry2013). In some regions there is evidence of the fishing-down process (Welcomme, Reference Welcomme1999, Reference Welcomme2001; Castello et al., Reference Castello, McGrath and Beck2011), in which large commercial fish species have been replaced by smaller species (Garcia et al., Reference Garcia, Tello, Vargas and Duponchelle2009; Castello et al., Reference Castello, McGrath, Hess, Coe, Lefebvre and Petry2013), and the effectiveness of most existing protected areas in protecting fish stocks in the Amazon is still unclear. Amazonian fisheries are considered to be moderately selective and the main fish species exploited vary among major river basins (Hallwass & Silvano, Reference Hallwass and Silvano2015), which may favour management measures on a broad scale. However, the management of small-scale Amazonian fisheries is challenging because of the spatial and temporal heterogeneity of fishing grounds, the diversity of fish species, the variety of fishing gear, and the social context (Bayley & Petrere, Reference Bayley and Petrere1989; Isaac et al., Reference Isaac, Silva and Ruffino2008; Hallwass et al., Reference Hallwass, Lopes, Juras and Silvano2011, Reference Hallwass, Lopes, Juras and Silvano2013a). Integrated assessments (fish assemblages and fishing) of the effectiveness of protected areas in protecting fish stocks are therefore necessary to determine if protected areas are an appropriate tool for fisheries conservation in the Amazon and in other freshwater ecosystems (Gupta et al., Reference Gupta, Kanagavel, Dandekar, Dahanukar, Sivakumar, Mathur and Raghavan2016a,Reference Gupta, Nautiyal, Borgohain, Sivakumar, Mathur and Chadwickb). To our knowledge, no study has compared both the integrity of fish assemblages and fishing productivity among large protected and unprotected areas in this region of high fish diversity.
Our aim was to compare fishing productivity and descriptors of fish assemblage (Table 1) between two distinct protected areas of sustainable use and an unprotected area in the Tapajós River in the Brazilian Amazon. We analysed descriptors of fish assemblage that have been negatively affected by fisheries: fish biomass, abundance, body size, richness, presence of target species and mean trophic level (Table 1). We used a variety of descriptors to conduct a more comprehensive evaluation of the performance of sustainable-use protected areas, which aim to conserve biodiversity, improve fisheries and maintain ecological integrity. We also investigated the influence of local environmental variation on the fish assemblages, to control for potential confounding effects. We tested two hypotheses: (1) fishers from protected areas have higher fishing productivity (catch per unit effort) than those from unprotected areas, and (2) protected areas have higher biomass, abundance, presence of target species, species richness, fish size and mean trophic level (fish assemblage descriptors) than unprotected areas (Table 1).
Table 1 Indicators of fishing and fish assemblages analysed to compare fish assemblages and fisher communities in two protected areas and an unprotected area in the Tapajós River, in the Brazilian Amazon (Fig. 1), with the rationale for each indicator, based on evidence from marine or freshwater ecosystems; the literature source; and the associated hypothesis.

Study area
The study areas are located in the lower section of the Tapajós River (Fig. 1). The river water is clear; i.e. oligotrophic, with low levels of sediment and nutrient concentrations (Goulding et al., Reference Goulding, Barthem and Ferreira2003). The hydrodynamic characteristics (e.g. flow), water chemistry, and associated terrestrial ecosystems are relatively similar across the studied areas. The climate is tropical humid, with a mean annual temperature of 25.5°C and annual variation < 5°C (IBAMA, 2004). The mean annual precipitation is 1,820 mm, with high seasonal variation and abundant rains in January–March. Consequently, the water level of the river varies considerably throughout the year (c. 5.13 m). The water level peaks in May–June and then declines until November–December, when the lowest water levels occur (ANA, 2012). The floodplain of the lower section of the Tapajós River is a complex landscape of channels, small streams (igarapés), flooded forests, and lakes, which changes from season to season as the water level of the main river changes. Floodplain lakes are connected to the main river during the high-water season and then usually become isolated with the arrival of the low-water season. These lakes are also heterogeneous in terms of size (48.64–1,091.33 km2), depth (c. 1.72–5.78 m), shape (ranging from round to river-like shapes), water chemistry (e.g. pH, transparency) and habitat structure (e.g. dense macrophyte stands vs a completely open water environment).
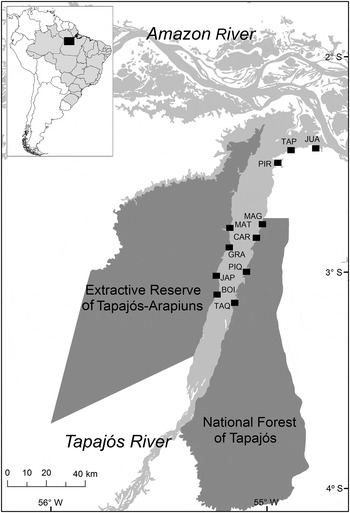
Fig. 1 Location of the 12 floodplain lakes (and associated fisher communities; Table 2) along the Tapajós River, in the Brazilian Amazon, where fish sampling was conducted.
There are two protected areas of sustainable use (Fig. 1) in the lower section of the Tapajós River: the National Forest of Tapajós and the Extractive Reserve of Tapajós–Arapiuns. The National Forest was designated in 1974 (IBAMA, 2004), with the primary objective of sustainable use of timber, and since 1992 it has also included faunal protection. The Extractive Reserve was officially designated in 1998, after an almost 20-year struggle by local residents against illegal logging (ICMBIO, 2008). In both protected areas the human population relies on a diversified system of subsistence, including small-scale agriculture, extractive forest production, livestock farming (mainly chickens), fishing and hunting (IBAMA, 2004; ICMBIO, 2008). In relation to fisheries, both protected areas allow only artisanal fishing gear, such as longlines, gillnets, hand lines and harpoons. Larger scale commercial fishing, which generally uses large boats and purse seining to catch pelagic fish species, is prohibited in the main channel of the Tapajós River, between the two protected areas. In contrast, in the surrounding unprotected area the human population density is 10 times higher, and both commercial and artisanal fisheries are common.
Methods
Sampled lakes and fisher communities
Four fisher communities were selected in each of the three areas studied (Fig. 1), on the basis that there was a minimum distance of 10 km between each community and fishers from the community consented to participate in the study. The communities studied are similar to others in the region, which are usually composed of a small number of families (mean = 47.17 ± SD 42.63; Table 2; IBAMA, 2004; ICMBIO, 2008) of mixed origin (indigenous, black and Caucasian), low educational level and low mean wage. After the selection the leader of each fisher community was asked to indicate the floodplain lake most exploited by fishers. These lakes were selected for further analysis of fish assemblages.
Table 2 Riverine communities in the National Forest of Tapajós, the Extractive Reserve of Tapajós–Arapiuns and an unprotected area of the Tapajós River (Fig. 1), with the number of families in each community, the number of fishers who participated in the study, the lakes (Fig. 1) in which fish landings were recorded, and the number of fish landings recorded.

Measure of fisheries productivity
Fish landings of fisher communities were recorded using a participatory method over 12 months, to estimate the catch per unit effort (fish biomass/time spent × crew size). In an initial stage of the research we interviewed a total of 203 fishers in the 12 communities surveyed. After each interview we invited the fishers who had at least 5 years of elementary education and fished at least three times per week to record their fish landings over a period of 1 year. Fifty-one fishers agreed to participate in this study (Table 2). They were trained individually and each received a pencil, a watch, a scale and forms to record their first five fish landings of each month, starting in August 2013 and finishing in July 2014. For each fish landing, fishers were requested to record the composition and weight of the catch, the fishing site (lake or river), the time spent fishing, and the number of fishers in the crew. Every 15 days, phone calls were made to the fishers, where possible, to discuss and resolve any problems. We collected the fish landing forms at c. 3-month intervals. The fish landing data (fishing gear used, fish species caught) recorded by fishers was positively and significantly correlated with interview data (Hallwass, Reference Hallwass2015), indicating the reliability of fisheries data, as shown in a previous study (Hallwass et al., Reference Hallwass, Lopes, Juras and Silvano2013b). More details of this methodology are in Hallwass (Reference Hallwass2015).
Fish sampling and biological measures
Two samplings were undertaken in each of the 12 floodplain lakes indicated by the community leaders (Fig. 1; Table 2). The first sampling was in July (high-water season) and the second in November (low-water season) 2013. Fishes were collected using two sets of gillnets (c. 420 m2 each) with different mesh sizes (15–80 mm between opposite knots) during 9.30 ± SD 0.46 hours (approximately 08.30–18.00). Although our sampling included crepuscular hours, some nocturnal fishes may have been underrepresented (e.g. catfishes). The gillnets were checked every 2 hours and each individual fish captured was measured to standard length (cm), weighed (g), anaesthetized with clove oil, preserved in a 10% formalin solution and identified to species level. The sampled fishes were also dissected for diet analysis to estimate their trophic position. Ingested prey was identified to the lowest taxonomic level possible (generally family or order). The volumetric method (Herrán, Reference Herrán1998) was used to quantify prey importance. In cases where < 10 individuals of a species were analysed we gathered additional information on diet from the literature (Supplementary Table S1).
Measurement of environmental characteristics
Twelve measures of physical–chemical parameters were made at each lake in each season. The pH and conductivity were measured using a digital water quality tester, the euphotic zone was estimated using a Secchi disk, and the depth was measured using a graduated chord. The percentage of pelagic zone, flooded forest and macrophytes in each lake was estimated visually.
The surface area, shoreline development, and distance to the Amazon River were estimated using images from the Landsat5 satellite (INPE, 2014). The shoreline development was calculated using the following equation:

where L is the shoreline length and S is the surface area of the lake. In the absence of clear images (without clouds) during the study year, images from the same season but from different years were used (low-water season images from November 2008, and high-water season images from July 2009). The spectral band composition (bands 5, 4, 3 in RGB composition) and image analysis were performed using ArcGis v. 9.2 (ESRI, Redlands, USA). All environmental variables were chosen because of their reported influence on fish assemblages in previous studies (Rodriguez & Lewis, Reference Rodriguez and Lewis1997; Tejerina-Garro et al., Reference Tejerina-Garro, Fortin and Rodríguez1998; Petry et al., Reference Petry, Bayley and Markle2003).
Fish assemblage descriptors
The index of relative importance (IRI; Pinkas et al., Reference Pinkas, Oliphant and Iverson1971) was used to determine the importance of each species caught by fishers in the communities studied. It was calculated as follows:

where N i is the numerical percentage of the ith species in all fish landings, W i is the percentage by weight of the ith species in all fish landings, and FO i is the frequency of occurrence percentage of the ith species in all fish landings. The index of relative importance was used to calculate an indicator of valuable fish presence (IVFP) in each lake, as follows:

where IRI i is the index of relative importance of the ith fish, and RA ki is the relative abundance of the ith fish in the kth lake. A high indicator of valuable fish presence in a lake suggests a high proportion of species relevant for fisheries. Thus, we used this index as a surrogate for the presence of target species (Hypothesis 2).
The total biomass and abundance sampled in each lake and in each season (high and low water) was corrected for the sampling effort (time and size of nets). Fish species richness was estimated for each lake and each season by an individual-based rarefaction procedure (Gotelli & Colwell, Reference Gotelli, Colwell, Magurran and McGill2011). The mean fish size was also estimated for each lake and each season.
The trophic position of each species in the lakes studied was calculated as follows:

where DC ij is the fraction of each jth prey in the diet of the ith predator, and TL j is the trophic level of the jth prey (Pauly et al., Reference Pauly, Palomares, Froese, Sa-a, Vakily, Preikshot and Wallace2001). Trophic levels of 1, 2 and 3 were assigned to ingested basal items (detritus, plant, algae), invertebrates and fish, respectively. Although broad, this trophic classification has been used successfully in regions for which little information is available (Hoeinghaus et al., Reference Hoeinghaus, Agostinho, Gomes, Pelicice, Okada and Latini2009).
The trophic level calculated for each species was used to calculate the mean trophic level (MTL) of each lake and each season, as follows:

where TL i is the trophic level of the ith fish and RA ki is the relative abundance of the ith fish in the kth lake (Pauly et al., Reference Pauly, Palomares, Froese, Sa-a, Vakily, Preikshot and Wallace2001). It should be noted that the food webs (and mean trophic levels) of floodplain lakes are not independent of the main river channel (Winemiller & Jepsen, Reference Winemiller and Jepsen1998). In addition, some species present in the lakes may not have been sampled, given the selectivity of our sampling methodology, and some species may exhibit ontogenetic variations in diet. However, the mean trophic level has been considered to be a good surrogate to identify general trends in the disappearance of large predators from unprotected areas (Pauly et al., Reference Pauly, Christensen, Dalsgaard, Froese and Torres1998, Reference Pauly, Palomares, Froese, Sa-a, Vakily, Preikshot and Wallace2001). We analysed the diet of fishes of various sizes (for the most abundant species) and during both the low- and high-water seasons (when fishes move between lakes and rivers), and therefore our sampling considered to some extent the influences of connectivity and ontogenetic variations in fish diets. Moreover, the main river channel between the protected areas studied is also under protection from commercial fishing. Thus, we believe that this descriptor could adequately indicate any significant difference in mean trophic level between protected and unprotected areas for the purposes of this study.
Data analysis
Fishing productivity
We grouped fish landings in four main seasons according to the water level: low, rising, high and falling. A linear mixed effects analysis was carried out using the package lme4 (Bates et al., Reference Bates, Maechler, Bolker and Walker2014) in R v. 3.2.3 (R Development Core Team, 2014) to test the influence of studied areas (protected and unprotected) on the mean productivity (catch per unit effort) of fishers in each season (Hypothesis 1). Areas, seasons and their interaction term were entered into the model as fixed effects, whereas fishers was entered as a random effect. The statistical significance (P) of each factor was determined by likelihood ratio tests comparing models with and without the variables of interest (Winter, Reference Winter2013). This mixed model analysis was conducted with two datasets: (1) fish landings from both lakes and the main river, and (2) fish landings from lakes only. This was necessary to understand the effects of fishing on these two environments, facilitating a more accurate comparison with fish sampling, which was conducted only in lakes. The catch per unit effort in both datasets was log-transformed to achieve the normality assumption of the mixed model analysis.
Fish assemblage descriptors
Model averaging (Burnham & Anderson, Reference Burnham and Anderson2002) was used to obtain robust estimates of relative importance value (I) for the relative effects of environmental variables and studied areas (Hypothesis 2). These two sets of independent variables (environment and areas) were used in linear models as predictors for each of the fish assemblage descriptors (fish biomass, abundance, richness, mean size, mean trophic level and indicator of valuable fish presence) in each season (high and low water). Interaction terms were not included in this analysis, given the limitation of our sampling unit and the high number of predictors. The model averaging analysis generated a relative importance of 0–1. To avoid multicollinearity between predictors we carried out a principal component analysis based on the correlation matrix, to group the following variables into principal component axes in both seasons: the percentage of pelagic zone, flooded forest and macrophytes (habitat coverage; Table 3), and the conductivity, pH, depth and euphotic zone (physical and chemical parameters; Table 3). The model averaging procedure was conducted in the package glmulti (Calcagno, Reference Calcagno2013) in R, and the principal component analysis was computed in the package stats (R Development Core Team, 2014).
Table 3 Results of principal component (PC) analysis (based on the correlation matrix, with percentage of variance accounted for in parentheses) carried out for habitat coverage (macrophytes, flooded forest, pelagic habitat) and physical and chemical (conductivity, pH, depth, euphotic zone) parameters in the low- and high-water periods in the Tapajos River, (Fig. 1). The environmental variables that contributed more for each axis are in bold. The percentage of explanation of each axis is given in parentheses.

Results
Fishing productivity
A total of 18,241 kg of fish was recorded, in 2,013 fish landings (9.07 ± SD 12.74 kg per landing). Of these fish landings, 67.2% were in the main river and 32.8% in lakes (Supplementary Table S2). The jaraqui (Semaprochilodus spp.; index of relative importance = 6.68) and pescada (Plagioscion spp.; index of relative importance = 6.35) were the main fish caught (Supplementary Table S2). The interaction term between season and areas was significant for the catch per unit effort (χ2(6) = 1,417.7, P < 0.001) when considering both lakes and the main river environments. The catch per unit effort was higher in fisher communities in the protected areas, as expected (Hypothesis 1; Fig. 2a). The only exception occurred in the low-water season, when the catch per unit effort was similar across all areas (Fig. 2a). However, when considering only fish landings from lakes, the catch per unit effort did not differ among areas (χ2(6) = 0.29, P > 0.05; Fig. 2b) and there was no interaction between areas and seasons (χ2(6) = 6.6, P > 0.05).

Fig. 2 Catch per unit effort (± SE) of fishers in the main river and floodplain lakes (a) and only in the lakes (b) in two protected areas (National Forest of Tapajós, Extractive Reserve of Tapajós–Arapiuns) and an unprotected area of the Tapajos River (Fig. 1) during the four seasons (high water, rising water, low water and falling water). Log-transformations were computed on base 10.
Fish assemblage descriptors
A total of 879 fishes of 67 species were collected (Supplementary Table S3). The area (National Forest of Tapajós, Extractive Reserve of Tapajós–Arapiuns, or unprotected) was one of the most important variables that influenced the three fish assemblage descriptors: mean fish size in the high-water season (I = 1), mean trophic level (I = 0.88) and indicator of valuable fish presence (I = 0.9) in the low-water season (Table 4; Fig. 3). However, fish size (Fig. 3d), mean trophic level (Fig. 3e) and indicator of valuable fish size (Fig. 3f) were not consistently higher inside protected areas, which indicates that Hypothesis 2 is not true.

Fig. 3 (a) Mean biomass, (b) mean species richness, (c) mean abundance, (d) mean standard length of fish, (e) mean trophic level and (f) indicator of valuable fish presence (IVFP) of fish catches in floodplain lakes in two protected areas (National Forest of Tapajós, Extractive Reserve of Tapajós–Arapiuns) and an unprotected area of the Tapajos River (Fig. 1) in the high-water (solid lines) and low-water seasons (dashed lines). Asterisks indicate high importance according to the model averaging approach. Error bars indicate ± SE values. Log-transformations were computed on base 10.
Table 4 Model-averaged importance of predictors for the dependent variables biomass, abundance, richness, mean size, mean trophic level, and indicator of valuable fish presence for both high- and low-water seasons in the Tapajos River (Fig. 1). The more important terms are in bold; the parameter estimates (slope) for each variable are in parentheses.

Environmental variables were good predictors of five of the descriptors studied (biomass, abundance, fish size, mean trophic level and species richness; Table 4). The surface area of the lake was slightly correlated with the richness of fish in the low-water season (I = 0.87, slope = 0.04). Shoreline development was positively correlated with the mean fish size (I = 0.86, slope = 0.25) and abundance (I = 0.75, slope = −0.42) in the high-water season. The principal component (PCA1) with values of physical–chemical parameters was an important predictor in the high-water season, being negatively correlated with the mean trophic level (I = 0.99, slope = −0.09). In the low-water season the first axis of physical–chemical parameters was an important predictor of the decrease in fish biomass (I = 0.82, slope = −0.08) and abundance (I = 0.87, slope = −1.49), and the second axis was important in explaining the increase in species richness (I = 0.53, slope = 0.33). The principal component (PCA1) of habitat coverage was strongly correlated with a decrease in fish biomass (I = 0.74, slope = −0.47) in the low-water season and a decrease in abundance (I = 0.65, slope = −4.21) in the high-water season. The distance to the Amazon River was not an important predictor of any of the fish assemblage descriptors in either season (Table 4).
Discussion
Our results show that fishers’ catch per unit effort was usually higher in the protected areas, confirming Hypothesis 1. It is unlikely that this result is attributable to the use of different fishing strategies in protected and unprotected areas, as gillnets were the most commonly used fishing gear in all communities studied (Hallwass, Reference Hallwass2015). Besides, local fishers on the Tapajós River fish in small paddle canoes or in canoes with underpowered engines, which limits their foraging area to the community surroundings (Hallwass, Reference Hallwass2015). Therefore, we conclude that the conditions provided by the protected areas in the Tapajós River, such as lower human population density and some management rules (e.g. a ban on commercial fishing) may act synergistically to reduce the levels of fishing pressure and increase fishing productivity (catch per unit effort) for local fishers. Increased fishing productivity has also been observed at smaller spatial scales, such as in protected areas established in lakes in other regions of the Brazilian Amazon (Almeida et al., Reference Almeida, Lorenzen and McGrath2009; Silvano et al., Reference Silvano, Hallwass, Lopes, Ribeiro, Lima and Hasenack2014). The accumulated evidence suggests that involving local people in management decisions for protected areas may be an efficient way to increase fish stocks in large tropical rivers.
Differences in the fishing productivity between the protected and unprotected areas were more pronounced during periods of high water level than periods of low water level. This seasonal difference may be caused by the pulsing dynamic of tropical rivers (Junk et al., Reference Junk, Bayley and Sparks1989). Fishing efficiency is generally higher in the low-water season (Begossi et al., Reference Begossi, Silvano, Amaral and Oyakawa1999; Maccord et al., Reference Maccord, Silvano, Ramires, Clauzet and Begossi2007), whereas when the water level is high, fishes become more difficult to catch and fishers’ catch per unit effort is higher in areas with higher fish density, such as protected areas. Another possible explanation is that higher fishing pressure in unprotected areas during the low-water season affects the abundance of fish stocks in the high-water season. In this scenario the recovery of fish stocks in the low-water season could be the result of the interaction between river flow and fish spillover from protected areas, as has been recorded in marine ecosystems (Gell & Roberts, Reference Gell and Roberts2003). Silvano et al. (Reference Silvano, Ramires and Zuanon2009) suggested a similar phenomenon to explain the similarity in terms of fish biomass of non-fished and fished lakes in a co-managed protected area in the Brazilian Amazon (Mamirauá Reserve): non-fished lakes could be a source of fishes for fished lakes in the high-water season, when connectivity between lakes increases. Nevertheless, there is little available information about the spillover effect in freshwater ecosystems (Ounboundisane et al., Reference Ounboundisane, Ainsley and Patricio2013), and further studies are needed to quantify this effect in freshwater protected areas.
Even with lower levels of fishing and higher fisheries productivity, the National Forest of Tapajós and the Extractive Reserve of Tapajós–Arapiuns alone may be insufficient to conserve and maintain fish stocks in the Tapajós River. The effectiveness of protected areas for fish protection depends on fish movement and the size of the protected area: sedentary animals tend to be better protected than those that cross protected area boundaries (Palumbi, Reference Palumbi2004). Most fish species in the Amazon undertake lateral or longitudinal migrations at least once in their life cycle (Barthem & Goulding, Reference Barthem and Goulding1997; Fernandes, Reference Fernandes1997; Winemiller & Jepsen, Reference Winemiller and Jepsen1998; Galacatos et al., Reference Galacatos, Barriga-Salazar and Stewart2004). Some species make seasonal migrations from nutrient-rich waters to oligotrophic waters, such as the Tapajós River, to feed and spawn, increasing the fishing productivity of oligotrophic rivers and consequently the income of fishers (Barthem & Goulding, Reference Barthem and Goulding1997; Benedito-Cecilio & Araujo-Lima, Reference Benedito-Cecilio and Araujo-Lima2002). Fisheries in the Tapajós River exploit species that perform long migrations, such as Brachyplatystoma rousseauxii, Brachyplatystoma filamentosum and Semaprochilodus insignis (Hallwass, Reference Hallwass2015), and therefore to guarantee the sustainability of fisheries, and fish conservation in the long term, it may be necessary to create a network of protected areas on a broader scale to ensure connectivity between rivers with nutrient-poor and rich waters.
Although fishers from the two protected areas had a higher catch per unit effort than fishers from the unprotected area, we found no effect of protected areas on fish landings originating from floodplain lakes. In experimental fish samples most of the fish assemblage descriptors (e.g. biomass, richness and abundance) did not differ between the two protected areas and the unprotected area. Although there was some variation in mean fish size, mean trophic level and the presence of valuable fishes between the three areas studied, smaller values of these parameters were not consistently observed in the unprotected area; for example, the mean trophic level was higher in the Extractive Reserve but the mean trophic level in the National Forest was similar to that observed in the unprotected area in the low-water season. We therefore conclude that there is no consistent evidence for Hypothesis 2.
The observed low influence of protected areas on fish assemblages and on fishing productivity in floodplain lakes may be a result of the relatively low levels of fishing in this habitat, as most fishes were caught in the main river channel. Besides, local reports suggest that large commercial fishing boats focus mainly on the main river channel of the Tapajós River (Hallwass, Reference Hallwass2015). The floodplain of the Tapajós River is smaller, and has fewer lakes, than other rivers of the same order in the Amazon Basin (Goulding et al., Reference Goulding, Barthem and Ferreira2003), and the mean catch rate (0.54 g m−2 h−1, standardized catch per unit effort) found in floodplain lakes of the Tapajós River was low compared to other Amazonian rivers sampled using comparable methodology (e.g. Solimões, 13.5 g, Silvano et al., Reference Silvano, Ramires and Zuanon2009; Manacapurú, 14.5 g, Saint-Paul et al., Reference Saint-Paul, Zuanon, Correa, García, Fabré, Berger and Junk2000; Lower Tocantins, 2.84 g, Silvano et al., Reference Silvano, Hallwass, Lopes, Ribeiro, Lima and Hasenack2014; and Negro, 2.69 g, Silvano et al., Reference Silvano, Zuanon, Zahorcsak, Ramos and Begossi2005). Given the low density of fishes, the floodplain lakes of the Tapajós River may be less attractive for fishers compared to floodplain lakes elsewhere in other Amazonian river basins. Target species, such as Plagioscion spp. and B. filamentosum, were caught almost exclusively in the main river.
The absence of effects of protected areas on fish assemblages in floodplain lakes may also be the result of management on a smaller spatial scale than that addressed by the protected areas. Evidence from qualitative interviews with fishers suggests that of the 12 communities studied, five have some local management initiative in adjacent lakes (Hallwass, Reference Hallwass2015). Although we have not assessed the effectiveness of local management here, other studies have shown that local organizations in co-management or common-based management have been effective in maintaining or even increasing fishing yields and fish abundance in the Brazilian Amazon (Maccord et al., Reference Maccord, Silvano, Ramires, Clauzet and Begossi2007; Almeida et al., Reference Almeida, Lorenzen and McGrath2009; Castello et al., Reference Castello, Viana, Watkins, Pinedo-Vasquez and Luzadis2009, Reference Castello, Arantes, McGrath, Stewart and De Sousa2015; Silvano et al., Reference Silvano, Ramires and Zuanon2009, Reference Silvano, Hallwass, Lopes, Ribeiro, Lima and Hasenack2014; Lopes et al., Reference Lopes, Silvano and Begossi2011), as well as in other tropical rivers (Gupta et al., Reference Gupta, Kanagavel, Dandekar, Dahanukar, Sivakumar, Mathur and Raghavan2016a) and marine ecosystems (Campbell et al., Reference Campbell, Cinner, Ardiwijaya, Pardede, Kartawijaya and Mukmunin2012).
The biological descriptors of fish assemblages in lakes were more related to environmental variables than to the existence of protected areas. Physical–chemical parameters, lake size and shape, and habitat coverage strongly affected the abundance, size and trophic levels of fishes. These results corroborate findings in other tropical rivers (Rodriguez & Lewis, Reference Rodriguez and Lewis1997; Tejerina-Garro et al., Reference Tejerina-Garro, Fortin and Rodríguez1998; Petry et al., Reference Petry, Bayley and Markle2003; Miranda, Reference Miranda2011). Our results also indicate that the response of fish assemblages to environmental conditions varies according to the season. The fish assemblage descriptors measured were correlated with different environmental variables in the low- and high-water seasons, and this may be attributable to several factors, including changes in fish density (Arrington et al., Reference Arrington, Winemiller and Layman2005), species composition (Fernandes, Reference Fernandes1997), and the range of environmental parameters (Junk et al., Reference Junk, Bayley and Sparks1989). Influential environmental variables should be recognized and addressed in the management programmes for tropical rivers, to ensure fish conservation and the sustainability of fisheries.
Our results indicate that protected areas of sustainable use in the Tapajós River, which were designed primarily to protect terrestrial ecosystems, increased the fishing productivity of local fishers. This provides novel insights for the management of large tropical rivers, reinforcing the importance of protected areas co-managed by local people to sustain the socio-ecological systems of small-scale fisheries. In the Brazilian Amazon the failure to achieve environmental and social objectives has been used as an argument to downsize or even change the status of protected areas to allow development projects to proceed (Ferreira et al., Reference Ferreira, Aragão, Barlow, Barreto, Berenguer and Bustamante2014). However, our study provides evidence that protected areas are important for social and environmental purposes and should be maintained. On the other hand, the biological parameters of fish assemblages in the floodplain lakes were correlated more with environmental factors than with the existence of protected areas. This lack of effect of protected areas may be partially explained by differences in fishing pressure between the floodplain lakes and the main river channel, by the effect of small-scale management in some lakes, and by the influence of environmental variables on fish assemblages. The environmental variation between lakes must therefore be considered in the establishment of protected areas and conservation programmes in tropical rivers.
Acknowledgements
We thank the Coordination for the Improvement of Higher Education Personnel (CAPES) and National Council for Scientific and Technological Development (CNPq) for scholarships to RAMS (309014/2013-1), a PhD scholarship to GH, and MSc and PhD (1286-15-13) scholarships to FWK; CAPES Programa Nacional de Cooperação Acadêmica (Procad)/Ação Novas Fronteiras (NF; 883/2010) for funding the research; and Instituto Chico Mendes de Conservação da Biodiversidade (ICMBio) for a permit to interview fishers and to conduct fish sampling in the protected areas. We also thank Frank Ribeiro, Cárlison Oliveira and André Canto for fish identification, Ana Bevilacqua and Natália Roos for help with field work, and Leonardo Maltchik, Érica Caramaschi and Sandra Hartz for helpful suggestions regarding the manuscript.
Biographical sketches
Friedrich Wolfgang Keppeler has a broad interest in the fields of ecology and evolution, with a particular concern for the sustainable use of natural resources and biological conservation. Gustavo Hallwass studies artisanal fisheries and their dynamics, use of natural resources, human ecology and fish ecology. Renato Azevedo Matias Silvano's research interests include artisanal fisheries, use of natural resources, ethnoecology and human ecology.