INTRODUCTION
Acute infectious diarrhoea is frequently found in children and young animals [Reference Clark and McKendrick1, Reference Lennon2]. Enteric viruses are associated with approximately half the cases of gastroenteritis in humans, with rotavirus and calicivirus accounting for at least 60% of them [Reference Giaquinto3]. Recent reports reveal that noroviruses (Caliciviridae family) have become a major causes of hospitalization for gastroenteritis in children worldwide, with prevalence and clinical impact in similar ranges to those observed for rotaviruses [Reference Koopmans4]. This is not the case for calicivirus infections in porcine populations, where the infections are often asymptomatic [Reference Wang5, Reference Konig, Bank and Thiel6]. It has been reported that oral administration of human norovirus GII to gnotobiotic piglets infected 60% of the animals and caused mild lesions in the proximal small intestine of only one piglet (1/7) [Reference Cheetham7, Reference Souza8]. Direct zoonotic transmission appears to be rare although genetic recombination between animal and human caliciviruses has been described [Reference Barry, Alfieri and Alfieri9]. The application of molecular biology techniques is revealing more viruses associated with gastroenteritis, facilitating the analysis of viral diversity and the detection of viral recombination events leading to the emergence of new variants that could propagate through different countries. Sapoviruses are emerging as important pathogens that cause diarrhoea in humans and animals. Sapoviruses are genetically variable and, based on the VP1 gene, they have been classified into seven genogroups (GI–GVII); GI, GII, GIV and GV infect humans, whereas GIII, GVI and GVII infect pigs.
Rotavirus infections are associated with diarrhoea in piglets [Reference Flewett10, Reference Svensmark11] and the severity of the clinical signs (diarrhoea) decreases with age [Reference Gelberg12]. Gene reassortment and recombination between animal and human rotaviruses have been described in several research reports [Reference Varghese13, Reference Matthijnssens14]. The most prevalent rotavirus group in humans and pigs is group A (90% of outbreaks) [Reference Will15, Reference Parashar16].
The objectives of our study were to determine the resemblance – if any – between rotavirus and calicivirus infections in intensive porcine production units in northern Spain, regarding (a) their respective prevalence and association with diarrhoea and (b) existing genomic similarities between human and porcine calicivirus (norovirus and sapovirus) strains.
MATERIALS AND METHODS
Sampling
A total of 221 faecal samples were collected from 12 porcine production farms (n=93) during 2005, two swine farms (n=40) and from a slaughterhouse (n=88) in 2006. The farms were located in Aragón (northern Spain) and the slaughterhouse in the city of Zaragoza (province capital). For statistical convenience, we divided the samples in five age groups and scored their consistency as diarrhoeic or non-diarrhoeic. Age groups roughly matched the management changes these animals undergo: group I (suckling 0–4 weeks), group II (weaning >4–8 weeks) group III (transition >8–16 weeks), group IV (fattening >16–24 weeks) and group V (adults >24 weeks). Specimens were obtained from asymptomatic (n=108) and diarrhoeic animals (n=113) and were maintained at −20°C until processing. Faecal suspensions were prepared as 10% (w/v) suspensions in PBS and clarified by centrifugation at 3000 g for 10 min.
RNA extraction
The viral nucleic acid was extracted from faecal suspensions using a commercial kit for RNA extraction (SpeedTools Total RNA Extraction kit; Biotools, Spain). A stool sample containing a Cowden-like strain was used as a calicivirus-positive control, and for norovirus, a human strain identified by reverse transcription–polymerase chain reaction (RT–PCR) and sequencing as a norovirus GII.4 genotype. Aliquots of ultra-pure sterile water were included as negative controls in all RNA extraction procedures. In each round of extraction, positive and negative controls were included.
RT–PCR for calicivirus detection
Two-step RT–PCR assays were performed using the primer pairs JV12/JV13 [Reference Vinje and Koopmans17] and p289/p290 [Reference Jiang18] directed to the RNA-dependent RNA polymerase (RdRp) region of norovirus and calicivirus (both norovirus and sapovirus). For RT, 25 μl of reaction mixture (22 μl of master mixture plus 3 μl of sample RNA) containing PCR buffer, 2·5 mm MgCl2, 400 μm of dNTPs, 1 μm of reverse primer NVp110, 10 U RNasin and 2 U AMV RT was incubated at 42–45°C for 60 min followed by heat inactivation at 94°C for 3 min then chilling to 4°C. Afterwards, another 25 μl of reaction mixture containing (2×) PCR buffer, 2·5 mm MgCl2, 1 μm of primer set p289/p290 or primer set JV12/JV13, and 1 U Taq DNA polymerase was added into the above RT mixture to a final volume of 50 μl. PCR reactions were performed for 40 cycles with each cycle at 94°C for 30 s, 48°C (for p289/290) or 50°C (for JV12/JV13) for 30 s and 72°C for 30 s, and a final extension for 10 min at 72°C. The RT–PCR products were analysed on 1·7% agarose gel electrophoresis stained with ethidium bromide and visualized using ultraviolet light.
TaqMan probes for real-time RT–PCR designed for human GI and GII genotypes of norovirus detection
We have adapted the real-time RT–PCR assay to detect GI and GII with primers directed to human norovirus capsid genes to be used in an Applied Biosystems thermocycler [Reference Svraka19]. Primers and probes were from Applied Biosystems (UK) (Table 1). Reverse transcription of sample RNA was performed similarly to the first step previously described for conventional calicivirus RT–PCR using the reverse primer from each set of primers and probes (GI or GII). Optimal primer and probe concentration and amplification conditions were determined. The PCR thermal profile consisted of an initial step of 50°C for 5 min, followed by 3 min at 95°C, and 40 cycles of 15 s at 94°C and 60 s at 60°C. Amplification, detection, and data analysis were performed on an ABI Prism 7000 real-time thermocycler, with ‘FAM or VIC’ selected as the detector. The baseline was set at where no fluorescence emissions were detectable and five cycles before the start of amplification was detected. As a general rule of thumb we considered a C t value of <38·00 as positive detection by amplification of the target template.
Table 1. Sequences, polarities, targets, and amplicon sizes corresponding to the primers and probes used for norovirus GI and GII detection directed to the virion capsid region

FAM, 6-carboxyfluorescein, TAMRA, 6-carboxytetramethylrhodamine.
* Norwalk virus complete coding sequence; accession number M87661.
† Norwalk-like virus strain GIFU99 complete genome; accession number AB084071.
Sequencing and sequence analysis
Purification of the PCR products was performed with GFX™ PCR DNA and Gel Band Purification kit (GE Healthcare, USA), then quantified by 2% agarose gel electrophoresis using Low DNA Mass Ladder (Invitrogen™, USA). MegaBACE™ 1000 and Thermo Sequenase™ II DNA Polymerase, DYEnamic™ ET Dye Terminator kit (GE Healthcare) were used for sequencing in both directions using the forward and reverse primers. Sequences were analysed with Sequence Analyzer and BioEdit (v. 7.0.5.3) software programs. Similarity searches were carried out using the NCBI databases and the basic analysis was done in BLAST (http:\\www.ncbi.nlm.nih.gov/BLAST/) followed by a multiple alignment with Clustal W (version 1.4).
RT–PCR for rotavirus detection
Viral RNA was extracted from a selection of rotavirus-positive samples to characterize the G and P types using VP7- and VP4-specific primers. For amplification of rotavirus genome segments the reverse transcription reaction was performed as previously described, but using random primers instead of gene-specific primers. G- and P-typing was carried out by multiplex nested amplification of the VP7 and the VP4 genes with primers specific for nine VP7 genotypes (G1-4, G6, G8, G9, G10, G12) and six VP4 genotypes (P[4], P[6], P[8], P[9], P[10], P[11]) following the instructions of the original publications describing these primers [Reference Arguelles20–Reference Gouvea22].
Statistical analysis
Winepi software www.winepi.net was used for data analysis. Direct odds ratio, Mantel–Haenszel method (ORMH) for ponderate OR and χ2 were calculated with a 95% confidence interval (CI).
RESULTS
Rotavirus
Thirty-seven out of 221 samples were positive for rotavirus. The rates of positive samples in the suckling and weaning piglets (groups I and II) were 26% and 47%, respectively, with a clear decreasing tendency in finishing piglets (groups III and IV) of 14·5% and 6·3%, respectively. All adult samples were negative by both VP4 and VP7 RT–PCR assays (Table 2).
Table 2. Prevalence (%) of rotavirus and calicivirus as calculated for each age group (Materials methods section)

Rotavirus prevalence is significantly different for each age group: P<0·001.
For OR estimation, we further regrouped the data into two categories: samples from animals aged <8 weeks or ⩾8 weeks. Applying the χ2 test in an observational study revealed an OR of 4·3 (95% CI 2·06–8·97) associating the age to the presence of rotavirus in a given sample, meaning that animals aged <8 weeks are at higher risk than those aged ⩾8 weeks of excreting rotavirus (Table 3). When we considered rotavirus presence and faecal consistency, the OR revealed a clear association between diarrhoeic samples and rotavirus presence in the sample (OR 4·98, 95% CI 2·08–11·95), meaning that the probability of detecting rotavirus from a diarrhoeic sample is fivefold higher than that from a non-diarrhoeic sample (Table 4). Since diarrhoea is more frequent in younger animals, we applied the Mantel–Haenszel (ORMH) statistical test to account for the age factor distortion in the OR value. In fact the OR value fell to 3·5 (95% CI 1·2–9·7), meaning that the weighting of age influence makes the real risk of associated diarrhoea and rotavirus shedding to be around 3·5-fold instead of fivefold (Table 5).
Table 3. Prevalence of rotavirus and calicivirus in relation to age

Values in parentheses are 95% confidence intervals.
OR=4·3 for rotavirus prevalence indicates that the risk decreases fourfold when piglets are ⩾8 weeks.
* Exposed group.
Table 4. Prevalence of rotavirus and calicivirus in relation to faecal consistency
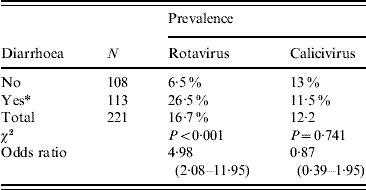
Values in parentheses are 95% confidence intervals.
Odds ratio (OR)=4·98 indicating that in a diarrhoeic sample rotavirus shedding probability is increased fivefold.
* Exposed group.
Table 5. Stratified odds ratio using as variable rotavirus detection and age influence on diarrhoea

OR, Odds ratio; CI, confidence interval; MH, Mantel–Haenszel.
Thirty out of 37 rotavirus-positive stool samples were G- and P-typed. The G/P genotype combinations detected were G10 P[6] (six samples), G12 P[8] (eight samples), G4 P[23] (three samples), and G9 P[8] (ten samples). Three strains were G3, although the VP4 genome segment could not be determined.
Calicivirus
Twenty-seven out of 221 samples were positive for calicivirus. Similar prevalence rates were found in different age groups (12%) (Table 2). In our study we found a positive sow with two of its suckling piglets positive for calicivirus using a p289/p290 primer set, indicating a possible transmission from a calicivirus-shedding sow to its progeny. Partial sequence of the RNA polymerase region (GenBank acc. no. FN397821) showed an 89% homology to a porcine enteric sapovirus isolated in The Netherlands (GenBank acc. no. AY615805).
It should be noted, that none of the samples in our study were positive by the real-time RT–PCR technique used to detect mainly human noroviruses GI and GII. Positive control human samples were always reactive. In fact, we checked norovirus RNA transcripts from 19 different types including nine GI, nine GII and one GIV. All RNA transcripts from GI and GII were detected by real-time RT–PCR, except for the GIV isolate. We received the norovirus reference panel (NRP) from Dr M. Koopmans, as part of the ‘Enteric Virus Emergence, New Tools’ (EVENT) European Project. The NRP contained RNA transcripts from Norwalk, White-Rose, Southampton, Birmingham, Malta, Musgrove, Mikkeli, Winchester and Boxer for GI, and Hawaii, Melksham, Toronto, Grimsby, Seacroft, Leeds, Erfurt, GGIIb and GGIIc for GII and Alphatron for GIV norovirus.
An interesting result is that there were no statistically significant differences in calicivirus detection between diarrhoeic faecal samples or non-diarrhoeic faecal samples, meaning that diarrhoea is not associated with calicivirus shedding in any age group (Tables 3 and 4).
DISCUSSION AND CONCLUSIONS
Our data indicate that rotavirus is frequently associated with diarrhoea in piglets, a fact established many years ago [Reference Svensmark23, Reference Davidson24]. A recent broader study in Japan indicated that rotavirus was the most prevalent agent in suckling (67·3%) and weaned (65·5%) piglets with diarrhoea, followed by E. coli, sapovirus, and C. parvum [Reference Katsuda25]. We found a lower prevalence of rotavirus with a similar decrease in age. This lower prevalence rate is probably due to the non-diarrhoeic samples included in our study.
It is remarkable that 12 rotavirus-positive samples from piglets had a G9 genotype. This genotype was the most frequent G-type circulating among children at the same time in Spain. G9 was also reported as the predominant porcine rotavirus genotype in Japanese piglets with diarrhoea in a study performed between 2000 and 2002, with a close relationship of their VP7 genes with those of human G9 strains [Reference Teodoroff26]. In addition, a porcine rotavirus strain was found with a VP4 sharing 87% amino-acid identity with an A34 strain, considered as the prototype of P14[Reference Svensmark23] [Reference Liprandi27].
In regard to calicivirus, our data suggest two conclusions: first, that the presence of sapovirus or norovirus in stools is not associated with diarrhoea. Second, that the porcine calicivirus detected from this region by RT–PCR with primers p289/p290, which are specific for the viral RNA-dependent RNA polymerase region and would produce products of 319 nt for norovirus and 331 nt for sapovirus, was not recognized by real-time RT–PCR designed for the capsid region that was used successfully to detect 18/19 human norovirus RNA. However, none of the positive samples with p289/290 primers were positive using JV12/JV13 primers. An epidemiological study [Reference Farkas28], using norovirus RT–PCR as well as norovirus antigen detection by EIA in an attempt to find antigenically related human noroviruses in pigs, ended without any norovirus detection by RT–PCR. This occurred while antibody prevalence of human norovirus in the pigs was similar to that found in the human population. Similar low detection rates of swine noroviruses are reported by others [Reference Sugieda29, Reference van Der Poel30], and the failure to detect any noroviruses in this study by real-time RT–PCR indicates that porcine noroviruses – if they are present in this region of Spain – could not be detected by the sets of primers that were used. Inhibiting factors that might have caused false-negative results are discarded since a statistically significant number of diarrhoeic and non-diarrhoeic samples from different age piglets were evaluated, using two different regions and different PCR sets of primers that would cover most human noroviruses. On the other hand, there is no reason to believe that inhibitory compounds are present in piglet faecal samples, since sapovirus and rotavirus were detected in the same samples.
We conclude that, rotavirus and calicivirus are circulating in the swine production units in northern Spain despite the new technical advances in their management. While rotavirus shedding is associated with onset of diarrhoea, mainly in piglets (suckling and weaning), calicivirus is homogeneously distributed in different age groups of swine and is not associated with diarrhoea. Specifically designed primer pairs based on swine norovirus isolates are needed to search for these viruses. Direct transmission of norovirus from pigs to humans is not supported by our data.
ACKNOWLEDGEMENTS
This study was supported by the European Commission Project ‘Providing tools to prevent emergence of enteric viruses’ (EVENT) grant SP22-CT-2004-502571 and a specific type A grant for N. Halaihel on rotavirus from the University of Zaragoza, Spain.
DECLARATION OF INTEREST
None.