According to the WHO’s latest report, CVD claims the lives of 17·9 million people per year, representing the leading cause of mortality(Reference Roth, Abate and Abate1,Reference Kyu, Abate and Abate2) . It is well known that major risk factors for CVD such as obesity, diabetes, dyslipidaemia, hypertension and poor nutrition lead to a rise of atherosclerosis(Reference Timmis, Townsend and Gale3–Reference Aminde and Veerman8), widely considered to be the chief component in cardiovascular pathologies. Consequently, prevention and treatment strategies targeting the above-mentioned risk factors are pivotal avenues to reduce costs and mortality associated with CVD.
A growing body of evidence has suggested that dietary interventions can effectively treat and prevent CVD(Reference Mozaffarian, Appel and Van Horn9). One of these interventions is collagen, a type of extracellular protein that makes up 25–30 % of the total protein in the human body(Reference Ohara, Matsumoto and Ito10). This compound is also highly available in fish and meat and thus is broadly used as a dietary supplement. However, given that collagen is not hydrolysed, its oral absorption is minimal, requiring hydroxylation before it becomes a physiologically accessible supplement(Reference Oesser, Adam and Babel11,Reference Sibilla, Godfrey and Brewer12) . In recent years, collagen peptide has been used in the food industry as a functional component. The physiological benefits of circulating dipeptides reflect the importance of consuming collagen peptides(Reference Shigemura, Kubomura and Sato13). Collagen peptides play essential biological roles, which include inhibiting the activity of angiotensin I-converting enzyme(Reference Qiu, Li and Weeber14), acting as signal messengers in anabolic cellular processes in cartilage, tendons and ligaments(Reference Schunck and Oesser15–Reference McAlindon, Nuite and Krishnan17) and activating the mechanistic target of rapamycin signalling pathway(Reference Kitakaze, Sakamoto and Kitano18). In addition, collagen peptides may improve lipid metabolism and increase insulin sensitivity(Reference Wang, Xie and Pei19), reduce Cyp450, nitric oxide and prostaglandin I 2 while increasing bradykinin(Reference Koenig, Peterson and Jones20,Reference Marion-Letellier, Dechelotte and Iacucci21) . Over the last decade, evidence from various clinical investigations focused on the effects of CPS on CVD-related markers; however, this line of research produced conflicting findings. For instance, Zhu et al. (Reference Zhu, Li and Peng22) indicated that daily supplementation of 13 g of CPS for 12 weeks led to a significant reduction in LDL, HDL, TAG, total cholesterol, systolic blood pressure (SBP), diastolic blood pressure (DBP), fasting blood sugar and HbA1c in patients with Type 2 diabetes mellitus and primary hypertension. Zdzieblik et al. (Reference Zdzieblik, Jendricke and Oesser23) also reported that 15 g/d of specific CPS for 12 weeks significantly increased fat-free mass and decreased fat mass in middle-aged men. Moreover, Kouguchi et al. (Reference Kouguchi, Ohmori and Shimizu24) demonstrated that 2·9 g/d of chicken collagen hydrolysate supplementation for 12 weeks significantly decreased SBP and DBP in patients with mild hypertension. Conversely, another randomised controlled trial showed that collagen peptide supplementation (CPS) daily supplementation of 2 g of CPS for 12 weeks did not change body mass, fat mass, LDL, HDL, TAG, total cholesterol and fasting blood sugar in healthy volunteers(Reference Tak, Kim and Lee25). Moreover, Jendricke et al. (Reference Jendricke, Kohl and Centner26) indicated that 15 g/d of specific CPS for 12 weeks significantly increased fat-free mass but did not affect body mass and fat mass in active women. These discrepancies could be attributed to differences in study design, CPS dosage and/or participant characteristics (age, sex, health status, etc.), which highlights the importance of a comprehensive review on this topic.
There are currently no comprehensive reviews to systematically assess the effect of CPS on CVD-related markers (e.g. body mass, fat mass, fat-free mass, LDL, HDL, TAG, total cholesterol, blood pressure and fasting blood sugar), which denotes a gap in knowledge. Therefore, we completed the current systematic review and meta-analysis of randomized controlled trials to determine the effects of CPS on cardiovascular markers.
Materials and methods
Systematic search and study selection
This review followed the 2020 PRISMA guidelines(Reference Page, McKenzie and Bossuyt27). Description of population, intervention, comparator and outcome is displayed in Supplementary Table 1. A systematic search was conducted using Scopus, PubMed and ISI Web of Science databases. The medical subject headings were used in the search strategy to find the relevant studies. A systematic search was carried out by applying the reported strategy and the key terms displayed in Supplementary Table 2. The search process was conducted with no date or language restrictions. The databases were searched up to 1 November 2021. Database searches were completed in conjunction with searches of reference lists derived from individual studies. Two researchers (SM and HM) independently carried out the search strategy and screening. Any discrepancies were resolved via discussion with another author (CJ).
Eligibility criteria
Two authors (SM and HM) identified the eligible records by screening the titles, abstracts and full text of eligible studies. All randomised, placebo-controlled trials in humans (either parallel or cross-over designs) that assessed the efficacy of CPS on a range of CVD-related markers were included in the review. These markers included: anthropometric and body composition (body mass, fat mass, fat-free mass and BMI), lipid profile (LDL, HDL, TAG and total cholesterol), blood pressure (both SBP and DBP) and glycaemic indices including fasting blood sugar, HbA1c and insulin resistance. The exclusion of the studies was based on the following criteria: (1) clinical trials with an intervention duration of less than two weeks, (2) clinical trials without a placebo group, (3) animal studies and (4) those with insufficient data for the outcomes of interest.
Data extraction
The extracted study characteristics are presented in Table 1. If there was no available relevant data, corresponding authors were contacted to obtain any missing data. The data extraction procedure was conducted separately by two researchers (SM and FJ) to ensure reliability. Any disagreements were resolved by consensus and discussion.
Table 1. Main characteristics of included studies

BM, body mass; NR, not reported; FM, fat mass; FFM, fat-free mass; TC, total cholesterol; SBP, systolic blood pressure; DBP, diastolic blood pressure; FBS, fasting blood sugar.
Quality assessment of studies
The Cochrane Collaboration’s tool(Reference Higgins, Altman and Gøtzsche28) was used to evaluate the quality of studies. This tool involved judging the degree of bias (either high, low or unclear) for a series of items covering different domains of potential bias. Two authors (SM and HM) assessed the quality (risk of bias) of the eligible studies individually. The quality evaluation procedure has been presented in previous works(Reference Moradi, Ziaei and Foshati29,Reference Hadi, Ghaedi and Moradi30) .
Meta-analysis of data
To evaluate the effect size of the anthropometric, lipid profile, blood pressure and glycaemic markers, the mean and standard deviation (sd) changes were extracted from the intervention and placebo groups. Sub-group analyses relating to the mean study participant age (less than 50 years or more), sex (women or men), body mass (normal, overweight or obese), dosage (less than 10 g or 10 g and over), type of intervention (with or without exercise), trial duration (6 or 12 weeks) and participant health status (healthy or unhealthy) were performed to establish possible sources of heterogeneity. For the random-effects model, the DerSimonian and Laird method was utilised(Reference DerSimonian and Kacker31). Within-group changes were calculated by subtracting the baseline mean from the final mean value in each group. The sd of the mean difference was calculated using the following formula:
sd change =
$$\sqrt {\left[ {{{\left( {{\rm{sd}}\;{\rm{baseline}}} \right)}^2}\; + \;{{\left( {{\rm{sd}}\;{\rm{final}}} \right)}^2}\; - \;\left( {2\; \times \;0\cdot8\; \times \;{\rm{sd}}\;{\rm{baseline}}\; \times \;{\rm{sd}}\;{\rm{final}}} \right)} \right]} $$
(Reference Wan, Wang and Liu32). For trials that reported only the standard error of the mean, sd was calculated using the following formula: sd =
$SEM \times \sqrt n $
, where ‘n’ represented the number of participants in each group. Heterogeneity between studies was assessed by Cochrane’s Q test (significance at P < 0·100) and I
2 index. Publication bias was evaluated by the visual inspection of funnel plots and formal testing by Egger’s regression asymmetry and Begg’s rank correlation tests(Reference Begg and Mazumdar33,Reference Egger, Smith and Schneider34) . All statistical analyses were conducted using STATA software, version 16 (Stata Corp LP).
Outcomes were considered significant at P < 0·05.
Quality of evidence
The general certainty of evidence across randomised-controlled trials was rated using the Grading of Recommendations Assessment, Development, and Evaluation working group guidelines. Based on the related assessment criteria, the quality of evidence was categorised into four classes: high, moderate, low and very low(Reference Guyatt, Oxman and Vist35).
Results
Selection and identification of studies
The study selection flow is displayed in Fig. 1. Our systematic database search yielded a total of 453 records, of which 239 were screened. Two hundred twenty-six studies failed to meet the inclusion criteria and were excluded from qualitative and quantitative analyses. One study was excluded from the quantitative assessment due to lacking a comparative placebo group(Reference Giglio, Schincaglia and da Silva36). Ultimately, twelve randomised-controlled trials, collectively comprising eleven outcome measures, were selected for the quantitative analysis(Reference Zdzieblik, Jendricke and Oesser23,Reference Kouguchi, Ohmori and Shimizu24,Reference Jendricke, Kohl and Centner26,Reference Han and Kang37–Reference Zhu, Li and Peng45) .

Fig. 1. Study selection flow chart
Characteristics of studies
In total, the twelve eligible studies included a total of 748 participants (359 individuals in the CPS and 389 in the placebo group) (Table 1). The mean age ranged from 25·4 ± 4·2 years to 72·2 ± 4·7 years. Studies were conducted in Germany(Reference Zdzieblik, Jendricke and Oesser23,Reference Jendricke, Kohl and Centner26,Reference Jendricke, Centner and Zdzieblik40,Reference Zdzieblik, Oesser and Baumstark44) , Korea(Reference Han and Kang37,Reference Koizumi, Inoue and Shimizu41,Reference Tak, Kim and Lee43) , Japan(Reference Kouguchi, Ohmori and Shimizu24,Reference Igase, Kohara and Okada38,Reference Ishii, Okada and Matsuoka39) , China(Reference Zhu, Li and Peng45) and India(Reference Kumar, Sugihara and Suzuki42). Included studies were performed in healthy(Reference Zdzieblik, Jendricke and Oesser23,Reference Jendricke, Kohl and Centner26,Reference Han and Kang37–Reference Koizumi, Inoue and Shimizu41) and overweight(Reference Tak, Kim and Lee43) participants, as well as in individuals with type 2 diabetes mellitus(Reference Zhu, Li and Peng45), mild hypertension(Reference Kouguchi, Ohmori and Shimizu24), osteoarthritis(Reference Kumar, Sugihara and Suzuki42) and age-related sarcopenia(Reference Zdzieblik, Oesser and Baumstark44). All of the trials used a parallel arms design. The twelve eligible trials were published between 2008 and 2021. The dose of CPS ranged from 900 mg/d to 15 g/d, while the duration of the clinical trials ranged from 6 to 12 weeks.
Quality assessment of studies
According to the Cochrane risk of bias assessment, ten studies were ranked as high quality (demonstrating a low risk of bias on ≥ 3 domains)(Reference Zdzieblik, Jendricke and Oesser23,Reference Kouguchi, Ohmori and Shimizu24,Reference Jendricke, Kohl and Centner26,Reference Ishii, Okada and Matsuoka39–Reference Zhu, Li and Peng45) and two as low quality (low risk of bias < 3 domains)(Reference Han and Kang37,Reference Igase, Kohara and Okada38) (Table 2).
Table 2. Quality assessment

+ shows low risk of bias; - shows high risk of bias; ? shows unclear risk of bias.
Meta-analysis of data
Effects of collagen peptide supplementation on anthropometric and body composition parameters
As illustrated in Fig. 2, pooled data from six studies revealed that the CPS did not significantly change body mass (–0·54 kg; 95 % CI: −2·13, 1·06; I 2 = 65·8 %; P = 0·510; n 6) compared with the placebo group. Furthermore, results indicated that CPS significantly decreased fat mass (–1·21 kg; 95 % CI: −2·13, −0·29; I 2 = 0·0 %; P = 0·010; n 4), but not body fat percentage (–0·65 %; 95 % CI: −1·76, 0·47; I 2 = 75·5 %; P = 0·256; n 5) compared with the placebo group. Sub-group analyses suggested that body fat percentage significantly changed after CPS in men (–1·66 %; 95 % CI: −2·48, −0·47; I 2 = 0·0 %; P = 0·006; n 2), obese or overweight participants (–1·22 %; 95 % CI: −2·39, −0·06; I 2 = 0·0 %; P = 0·039; n 2) and in those supplementing in conjunction to exercise training (–1·36 %; 95 % CI: −2·60, −0·12; I 2 = 0·0 %; P = 0·032; n 3) (Table 3). Further sub-group analyses involving age demonstrated that fat mass significantly decreased after CPS for participants only aged 50 years and over (–1·50 kg; 95 % CI: −3·07, −0·18; I 2 = 0·0 %; P = 0·020; n 2) (Table 3). The observed between-study heterogeneity for anthropometric and body composition parameters was attenuated by all sub-group analysis (Table 3).
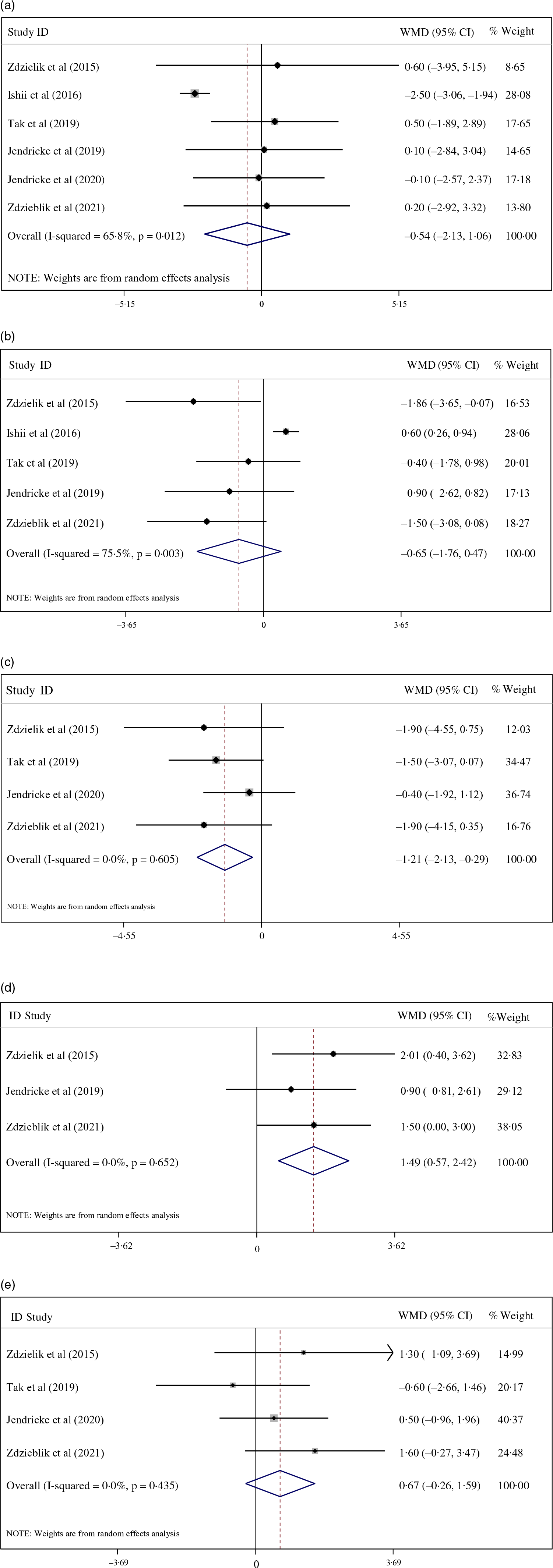
Fig. 2. (a) Forest plot displaying weighted mean difference and 95 % CI for the effects of collagen peptide supplementation v. placebo on body mass.
Table 3. Sub-group meta-analyses for the effects of collagen peptide supplementation on metabolic parameters

WMD, weighted mean differences.
In addition, CPS significantly increased free fat mass based on body mass percentage (1·49 %; 95 % CI: 0·57, 2·42; I 2 = 0·0 %; P = 0·002; n 3), but not based on kg (weighted mean differences: 0·67 kg; 95 % CI: −0·26, 1·59; I 2 = 0·0 %; P = 0·441; n 4) compared with the placebo group (Fig. 2). Due to the lack of studies measuring fat-free mass, sub-group analyses were not feasible.
Effects of collagen peptide supplementation on lipid profile
Pooled data from studies assessing lipid profile demonstrated that CPS led to a significant decrease in LDL concentrations (–4·09 mg/dl; 95 % CI: –8·13, –0·04; I 2 = 93·4 %; P = 0·048; n 5), unlike HDL (3·13 mg/dl 95 % CI: –0·18, 6·43; I 2 = 64·8 %; P = 0·064; n 3), total cholesterol (–5·28 mg/dl; 95 % CI: –14·31, 3·75; I 2 = 98·1 %; P = 0·252; n 7) and TAG (–6·51 mg/dl; 95 % CI: –13·71, 0·70; I 2 = 92·1 %; P = 0·077; n 5), which remain unchanged compared with the placebo (Fig. 3). Sub-roup analyses according to dosage of intervention suggested that CPS significantly decreased LDL (–9·08 mg/dl; 95 % CI: –10·21, –7·94; I 2 = 93·4 %; P =< 0·001; n 2), total cholesterol (–23·39, mg/dl; 95 % CI: –26·60, –19·98; I 2 = 90·7 %; P =< 0·001; n 2), TAG (–12·84, mg/dl; 95 % CI: –15·45, –10·23; I 2 = 95·3 %; P =< 0·001; n 2) and significantly increased HDL (6·76 mg/dl; 95 % CI: 2·63, 10·89; I 2 = 0·0 %; P =< 0·001; n 2) following a 10 g and over CPS intervention, in contrast to a less than 10 g intervention (Table 3). The observed extreme between-study heterogeneity for lipid profile was attenuated by sub-group analysis based on the dosage of intervention (Table 3).
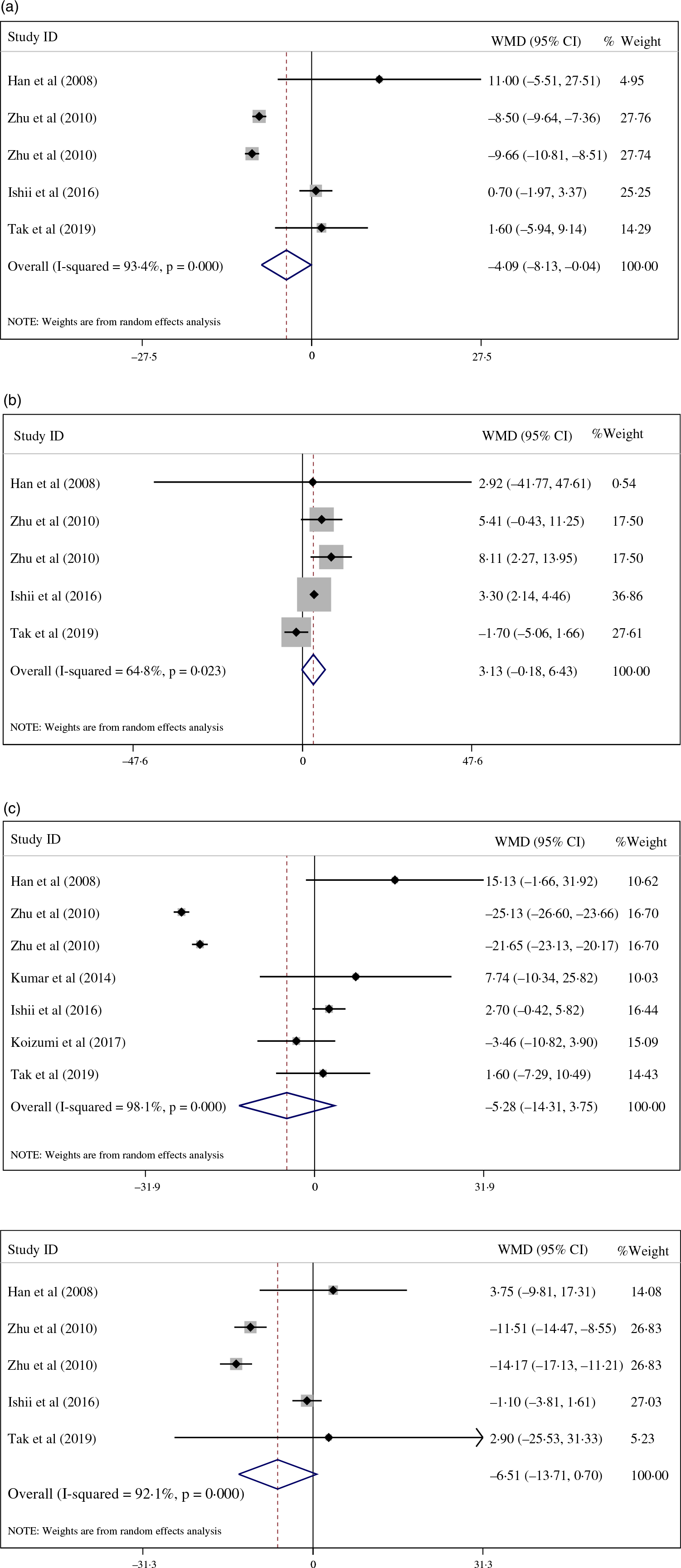
Fig. 3. (a)Forest plot displaying weighted mean difference and 95 % CI for the effects of collagen peptide supplementation v. placebo on low-density lipoprotein.
Effects of collagen peptide supplementation on blood pressure
CPS significantly decreased SBP (–5·04 mmHg; 95 % CI: −9·22, −0·85; I 2 = 98·9 %; P = 0·018; n 6), but not DBP (0·71 mmHg; 95 % CI: −0·25, 1·68; I 2 = 86·7 %; P = 0·148; n 6) compared with the placebo (Fig. 4). Sub-group analyses relating to the duration of intervention indicated that CPS significantly decreased SBP following a six-week intervention (–7·00 mmHg; 95 % CI: −13·80, −0·20; I 2 = 0·0 %; P = 0·044; n 2), in contrast to a 12-week intervention duration, which failed to produce a significant results (Table 3). Sub-group analyses according to dosage of intervention suggested that CPS significantly decreased SBP (–9·35, mmHg; 95 % CI: −11·28, −7·43; I 2 = 95·3 %; P =< 0·001; n 2) and significantly increased DBP (1·15 mmHg; 95 % CI: 0·25, 1·35; I 2 = 55·1 %; P =< 0·001; n 2) following a 10 g and over CPS intervention, in contrast to a less than 10 g intervention (Table 3). The observed extreme between-study heterogeneity for DBP was attenuated by sub-group analysis based on the trial duration and dosage of intervention (Table 3).

Fig. 4. (a) Forest plot displaying weighted mean difference and 95 % CI for the effects of collagen peptide supplementation v. placebo on systolic blood pressure.
Effects of collagen peptide supplementation on glycaemic indices
Our results failed to show a significant effect of CPS on glycaemic indices which included fasting blood sugar (–11·15, mg/dl; 95 % CI: –26·15, 3·84; I 2 = 99·8 %; P = 0·145; n 6) and HbA1c (–0·26 %; 95 % CI: –0·58, 0·06; I 2 = 98·3 %; P = 0·106; n 3) compared with the placebo group (Fig. 5). Sub-group analyses according to dosage of intervention suggested that CPS significantly decreased fasting blood sugar (–29·24, mg/dl; 95 % CI: –30·19, –28·28; I 2 = 0·0 %; P =< 0·001; n 2) and HbA1c (–0·39 %; 95 % CI: –0·58, –0·20; I 2 = 88·4 %; P =< 0·001; n 2) following a 10 g and over CPS intervention, in contrast to a less than 10 g intervention (Table 3). The observed extreme between-study heterogeneity for fasting blood sugar was attenuated by sub-group analysis based on the dosage of intervention (Table 3).
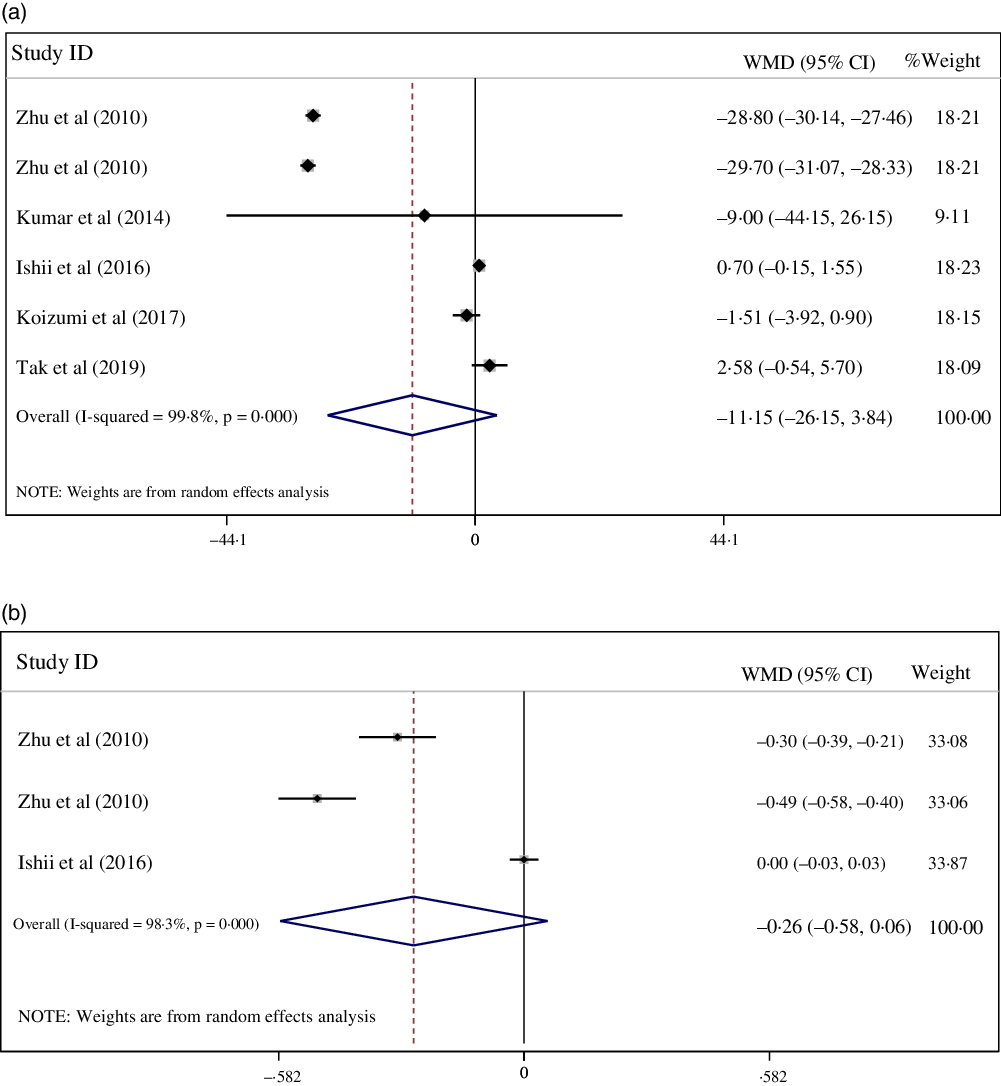
Fig. 5. (a) Forest plot displaying weighted mean difference and 95 % CI for the effects of collagen peptide supplementation v. placebo on fasting blood sugar.
Sensitivity analysis
Sensitivity analysis was performed by omitting each of the included studies. The results showed that the weighted mean differences was not changed significantly by omitting each of the studies. This indicated the meta-analysis results were stable and not sensitive to any one of the twelve studies (online Supplementary Fig. 1).
Publication bias
Moreover, no evidence of publication bias was detected regarding the efficacy of CPS on fat mass (P = 0·17, Begg’s test; P = 0·30, Egger’s test), fat-free mass based on body mass percentage (P = 0·69, Begg’s test; P = 0·60, Egger’s test), kilograms (P = 0·16, Begg’s test; P = 0·33, Egger’s test), LDL (p = 0·62, Begg’s test; P = 0·14, Egger’s test), HDL (P = 0·63, Begg’s test; P = 0·95, Egger’s test), TAG (P = 0·62, Begg’s test; P = 0·77, Egger’s test), total cholesterol (P = 0·65, Begg’s test; P = 0·095, Egger’s test), SBP (P = 0·57, Begg’s test; P = 0·39, Egger’s test), DBP (P = 0·57, Begg’s test; P = 0·37, Egger’s test), fasting blood sugar (P = 0·85, Begg’s test; P = 0·82, Egger’s test) and HbA1c (P = 0·12, Begg’s test; P = 0·16, Egger’s test). However, the Egger’s test results showed publication bias for body mass (P = 0·04) and body fat percentage (P = 0·002). These results were not confirmed by the Begg’s test for body mass (P = 0·34) and body fat percentage (P = 0·06).
Quality of evidence
To evaluate the quality of evidence for results, the GRADE guideline was utilised, which demonstrated the effect of fat mass (kg) to be of high quality. The evidence about fat-free mass (%), fat-free mass (kg) and SBP were downgraded to a moderate level. Based on the GRADE framework, body mass and LDL evidence were categorised as low quality. Finally, the fat mass (%), HDL, TAG, total cholesterol, DBP, fasting blood sugar and HbA1c were established as very low quality (Table 4).
Table 4. Grade profile of collagen peptide supplementation for cardiovascular risk factors scores in adults

TC, total cholestrol; FBG, fasting blood glucose; SBP, systolic blood pressure; DBP, diastolic blood pressure.
Discussion
The present review is the first to investigate the effects of CPS on cardiovascular disease-related markers, including blood pressure, anthropometric indices, lipid profile and glycaemic parameters. The pooled data that was derived from twelve randomised, controlled trials found that CPS (900 mg/d to 15 g/d), for a duration of 6 to 12 weeks, led to the significant reduction in fat mass, SBP, serum LDL concentrations and increased fat-free mass (measured by body mass percentage). Additional sub-group analyses demonstrated that CPS significantly decreased body fat percentage in men, obese or overweight participants, and when CPS was administered in combination with exercise.
A narrative review performed by Cicero et al. (Reference Cicero, Fogacci and Colletti46) revealed that bioactive peptides play potential roles in preventing and treating chronic diseases, including cancer and cardiovascular disorders. Indeed, several beneficial effects of ingesting collagen peptides are attributed to the physiological properties of circulating dipeptides, which are derived from collagen(Reference Shigemura, Kubomura and Sato47). This includes the potential ability to alleviate a range of cardiovascular complications, including heart failure(Reference Florea and Anand48), a condition preceded by high blood pressure in 70 % of cases(Reference McKee, Castelli and McNamara49).
Our outcomes revealed that CPS significantly decreased SBP. Previous studies have documented that CPS can help improve blood pressure in various pathways(Reference Zhu, Li and Peng22,Reference Kouguchi, Ohmori and Shimizu24,Reference Igase, Kohara and Okada38) . Saiga et al. (Reference Saiga, Iwai and Hayakawa50) reported that the hydrolysed collagen peptides extracted from the drumstick significantly suppressed high blood pressure by inhibiting angiotensin I-converting enzyme in spontaneously hypertensive rats. Moreover, Kouguchi et al. (Reference Kouguchi, Ohmori and Shimizu24) suggested that CPS may have a beneficial effect on the vascular system by activating certain vasodilating agents such as nitric oxide, which decrease arterial stiffness. Zhu et al. (Reference Zhu, Li and Peng22) also showed that marine collagen peptides supplementation ameliorated hypertension by activating peroxisome proliferator-activated receptors, leading to down-regulation of nuclear factor-kB pathway and reduced production of cytochrome P450 and prostaglandin I 2. Their results also showed an upregulation of bradykinin, which confirmed the angiotensin I-converting enzyme-inhibitory effect of collagen on blood pressure regulation(Reference Zhu, Li and Peng22). Importantly, it has been established that a reduction of 2 mmHg in SBP can lower coronary heart disease and stroke mortalities by 4 % and 6 %, respectively(Reference Chobanian, Bakris and Black51). Hence, our findings indicating an SBP-lowering effect (∼5 mmHg) of CPS support its clinical significance as a nutritional strategy for blood pressure improvement.
Elevated concentrations of blood lipids are well-established risk factors for cardiovascular diseases(Reference Nelson52). Our results revealed that CPS led to a significant decrease in LDL concentrations. Several investigations have assessed the potential blood lipid modulation by CPS therapy(Reference Zhu, Li and Peng22,Reference Tak, Kim and Lee25,Reference Han and Kang37,Reference Ishii, Okada and Matsuoka39) . Lee et al. (Reference Astre, Deleruyelle and Dortignac53) indicated that fish-derived collagen peptide might improve lipid metabolism and accumulation by a reduction in genes expression of CCAAT enhancer-binding protein-α and peroxisome proliferator-activated receptor-γ and adipocyte protein 2. Furthermore, Woo et al. (Reference Woo, Song and Kang54) suggested that collagen peptide skate skin-derived with dose-dependent manner suppressed hepatic lipid accumulation and reduced the lipid droplet size in the adipose tissue. Prior investigations also reported that CPS could lower plasma and hepatic lipid concentrations by suppressing the hepatic protein expression for fatty acid cholesterol synthesis, including sterol regulatory element-binding protein-1, sterol regulatory element-binding protein-2, fatty acid synthase, acetyl-CoA carboxylase and 3-hydroxy-3-methylglutaryl-CoA reductase(Reference Woo, Song and Kang54–Reference Lammi, Aiello and Bollati56). Recently, animal research by Vijayan et al. (Reference Vijayan, Raman and Dara57) showed that fish-derived collagen peptide has anti-lipidaemic properties that are mediated by a down-regulation of 3-hydroxy-3-methylglutaryl-CoA reductase expression and up-regulation Lecithin–cholesterol acyltransferase. On other hand, CPS may enhance lipid breakdown by upregulating gene expression of PPAR-α, carnitine palmitoyltransferase 1 and synthesis of bile acid cytochrome P450 family 7 subfamily A member 1(Reference Woo, Song and Kang54–Reference Lammi, Aiello and Bollati56), which play a role in the process of β-oxidation.
Interestingly, the results of the current study revealed that CPS significantly increased free fat mass and decreased fat mass. A potential mechanism for the improvement of fat-free mass and decrease in fat mass is that hydroxyprolyl-glycine levels can be elevated in blood after CPS(Reference Shigemura, Akaba and Kawashima58). These dipeptides seem to have signalling effects and promote C2C12 myoblast differentiation and increase myotube hypertrophy by activating the mTOR signalling pathway(Reference Kitakaze, Sakamoto and Kitano18). The prolyl-hydroxyproline, as other collagen peptides, contain dipeptides(Reference Shigemura, Akaba and Kawashima58,Reference Jimi, Koizumi and Center59) , accelerates muscle regeneration by inducing several myogenically differentiated cells, including myogenin-positive myoblasts and myoglobin-positive myocytes(Reference Jimi, Koizumi and Center59). Furthermore, it has been revealed that specific collagen peptides could act as signal messengers in anabolic cellular activities in ligaments, tendons and cartilage(Reference Schunck and Oesser15). It also might be helpful to improve pain symptoms and performance in activity-related joint discomforts(Reference Zdzieblik, Jendricke and Oesser23,Reference Zdzieblik, Oesser and Baumstark44) . Hence, CPS could enhance body composition and increase functional performance by improving joint-related disorders and their symptoms. Other potential mechanisms for the effects of CPS on improving body composition are its anti-inflammatory and antioxidant effects. The collagen peptides’ amino acids content, such as glycine, has been found to have powerful anti-inflammatory properties which can modulate muscle wasting in various models(Reference Koopman, Caldow and Ham60,Reference Sun, Wu and Ji61) . Additionally, Vijayan et al. (Reference Vijayan, Sreerekha and Dara62) suggested that CPS can attenuate oxidative stress by improving enzymatic antioxidant defense catalase and superoxide dismutase, leading to decreased levels of membrane lipid peroxidation. Chen et al. (Reference Chen, Liang and Wu63) also indicated that CPS enhanced the anti-inflammatory process by decreasing lipoxygenase activity and nitric oxide radicals. Moreover, it is possible that the decrease in fat mass in our results might be associated with higher resting energy expenditure affected by the significant increase in fat-free mass.
Several strengths can be derived from the present review. Firstly, the reviewed studies considered a body of literature that measured several important markers associated with cardiovascular disease. A majority of the included studies appeared to be of high quality, as distinguished by a validated risk of bias assessment. In addition, there were no signs of publication bias according to Begg’s and Egger’s tests. Despite these strengths, the current review consisted of some potential limitations. Participants were supplemented with different collagen peptide doses between studies and undertook supplementation for varying timeframes. The cardiovascular markers were only considered as secondary outcomes in some of the included trials, potentially impacting the measurement of these markers. There was high heterogeneity between enrolled study participants, with studies comprising of participants displaying diverse health statuses and ages. Finally, lifestyle modifications throughout the study periods were not controlled in some of the included studies. Lifestyle modifications such as dietary intake and physical activity may be confounding factors by influencing the effects of CPS on the assessed markers.
Conclusion
Taken together, this comprehensive review of collated data suggests that CPS may significantly decrease fat mass, fat-free mass (based on body mass percentage), SBP and serum LDL levels. Additional sub-group analyses revealed an important decline in fat mass among men, obese or overweight participants and those consuming collagen peptides while exercising. Additional long-term, well-designed randomised, placebo-controlled trials are recommended further to examine the impact of CPS on CVD-related markers and expand on our findings.
Acknowledgements
None.
This research received no specific grant from any funding agency, commercial or not-for-profit sectors.
SM and CJ designed this study. SM, MHK, and HM conducted the literature search. SM and FJ performed the statistical analysis and interpretation of the data. ZJ, FN, SPM, and SMG wrote the manuscript. RB, AW, and NT critically revised the manuscript. All authors approved the final version of the manuscript.
Supplementary material
For supplementary material/s referred to in this article, please visit https://doi.org/10.1017/S0007114522001301