Introduction
In the late 1950s, chemical companies ramped up production of insecticides, which proliferated in many agricultural crops (Osteen and Szmedra, Reference Osteen and Szmedra1989). Concurrently, Stern et al. (Reference Stern, Smith, van den Bosch and Hagen1959) introduced a strategy called ‘integrated control’ to harmonize chemical and naturally occurring biological control in a unified, ecologically sound insect management system. Stern et al. (Reference Stern, Smith, van den Bosch and Hagen1959) influenced crop protection worldwide and moulded the conceptual basis for modern integrated pest management (IPM). In 1981, when its second author Ray F. Smith was elected to the US National Academy of Sciences for advancing IPM worldwide, the Stern et al. (Reference Stern, Smith, van den Bosch and Hagen1959) article was pronounced as ‘the single most important paper published on crop protection in this century’ (Warnert, Reference Warnert2009). In 1972, President Nixon directed federal government agencies in the USA to advance the concept and application of IPM in agriculture, urban areas, forestry and other relevant sectors (Nixon, Reference Nixon1972). In 1979, President Carter established an inter-agency IPM coordinating committee to ensure development and implementation of IPM practices by all federal agencies in the USA (Bottrell, Reference Bottrell1979). Previously, the Food and Agriculture Organizations of the United Nations launched an FAO–UNEP cooperative global programme for the development and application of integrated pest control in developing countries (FAO, 1975; Brader, Reference Brader1979).
The seminal integrated control strategy of Stern et al. (Reference Stern, Smith, van den Bosch and Hagen1959) systematically combined insecticides and biological control to manage the spotted alfalfa aphid [Therioaphis maculata (Buckton)] of alfalfa [Medicago sativa (L.)]. Eventually, other chemical, biological and physical tactics were incorporated to manage insect, plant pathogenic, weed, snail, vertebrate and other pests of dozens of agricultural crops (Kogan, Reference Kogan1998; Way and van Emden, Reference Way and van Emden2000; Maredia et al., Reference Maredia, Dakouo and Mota-Sanchez2003; Kogan and Jepson, Reference Kogan and Jepson2007; Koul et al., Reference Koul, Cuperus and Elliott2008; Peshin and Dhawan, Reference Peshin and Dhawan2009a, Reference Peshin and Dhawan2009b; Abrol and Shankar, Reference Abrol and Shankar2012; Abrol, Reference Abrol2014; Coll and Wajnberg, Reference Coll and Wajnberg2017a). In addition to entomologists, plant pathologists, weed scientists, nematologists and other pest specialists, economists and sociologists have contributed to the concept, design, implementation and evaluation of IPM programmes.
In the first major review of integrated pest control schemes in developing countries, Brader (Reference Brader1979) reported that the integrated strategy had reduced pesticide use and provided more durable crop protection in agricultural crops of Africa, Asia, Latin America and the South Pacific. More than three decades later, Pretty and Bharucha (Reference Pretty and Bharucha2015) assessed outcomes on productivity and reliance on pesticides using published data from 85 IPM projects in 24 countries in Asia and Africa. Except for Japan, all were developing countries. The IPM projects had been implemented on several food and cash crops since 1990; the time elapsed from project start to measurement of reported impact varied from 1 to 5 years. Pretty and Bharucha (Reference Pretty and Bharucha2015) found a highly variable but mean yield increase across projects and crops of 40.9% (sd 72.3) and a highly variable but mean decline in pesticide use of 30.7% (sd 34.9), compared with the baseline. They estimated that 10–20 million farmers on 10–15 million hectares had adopted IPM in these projects.
Despite IPMs demonstrated potential in developing countries, its adoption rate has been low and its advances short-lived. Brader's (Reference Brader1979) review noted the lack of an effective independent extension service, de-linked from pesticide merchandizing, as a major hindrance. More than 35 years later, Parsa et al. (Reference Parsa, Morse, Bonifacio, Chancellor, Condori, Crespo-Pérez, Hobbs, Kroschel, Ba, Rebaudo, Sherwood, Vanek, Faye, Herrera and Dangles2014) used structured concept mapping (Trochim, Reference Trochim1989) to survey IPM specialists for open-ended opinions on obstacles that prevent IPM's adoption in developing countries. Respondents from 96 countries identified 51 unique obstacles. Subsequently, 163 participants of an international workshop rated the obstacles according to importance and remediation difficulty. Developing country participants rated ‘IPM requires collective action within a farming community’ as the top obstacle whereas high-income country participants prioritized ‘shortage of well-qualified experts and extensionists’ (Parsa et al., Reference Parsa, Morse, Bonifacio, Chancellor, Condori, Crespo-Pérez, Hobbs, Kroschel, Ba, Rebaudo, Sherwood, Vanek, Faye, Herrera and Dangles2014). Developing country participants also rated ‘shortage of practices and products as effective as chemical pesticides’ and ‘IPM research poorly oriented to needs of farmers’ as two other priorities that differed significantly from high-income country participants. Notwithstanding its low adoption rate in developing countries, IPM potentially offers the best route to economically efficient crop protection that increases and sustains farm productivity while minimizing threats to humans and the environment.
Aims of the paper
The current review highlights obstacles hindering IPM's adoption and sustainability on resource-limited farms in developing countries and presents pathways for overcoming them. Firstly, the conceptual basis of IPM and the criteria for determining if control tactics have been integrated harmoniously in IPM programmes are examined. The effects of local and regional landscape patterns on pests and why it is important to consider these effects in designing IPM are then reviewed, as well as the ecological features of the agroecosystem and procedures for making IPM an integral and durable component of this living system, and the huge challenge of connecting the vast population of resource-limited farmers to reliable information and technical sources required to sustain IPM.
Integrating integrated pest management tactics: theory v. application
Integrated pest management's conceptual foundation
Stern et al. (Reference Stern, Smith, van den Bosch and Hagen1959) clearly articulated a concept for integrating chemical control and biological control in a cohesive, ecologically based insect management scheme using decision-making guidelines. These and many other entomologists recognized biological control as a key tactic of the ecologically based strategy. van den Bosch et al. (Reference van den Bosch, Messenger and Gutierrez1982), for example, asserted that ‘biological control is in its essence an ecological phenomenon and in its practice is an example of “applied” ecology’. However, as additional control tactics were incorporated into pest management schemes and specialists other than entomologists contributed, IPM often veered from the original paradigm (Ehler, Reference Ehler2006; Coll and Wanjberg, Reference Coll, Wajnberg, Coll and Wajnberg2017b). It even became a convenient term to rationalize the unilateral use of pesticides without considering alternative methods (Barfield and Swisher, Reference Barfield and Swisher1994; Kogan, Reference Kogan1998; Power, Reference Power1999; Ehler and Bottrell, Reference Ehler and Bottrell2000; Ehler, Reference Ehler2006). Although IPM has no uniformly accepted definition (Coll and Wajnberg, Reference Coll, Wajnberg, Coll and Wajnberg2017b), Kogan (Reference Kogan1998) defined IPM thus: ‘IPM is a decision support system for the selection and use of pest control tactics, singly or harmoniously coordinated into a management strategy, based on cost/benefit analyses that take into account the interests of and impacts on producers, society and the environment.’ (Kogan, Reference Kogan1998). This definition would foster broad unanimity among those who view ecologically based IPM as a necessary, integral component of profitable, environmentally safe sustainable agriculture. The current paper upholds Kogan's (Reference Kogan1998) definition and also his conceptual framework for an IPM strategy. Asian rice (Oryza sativa L.) and insect biases are acknowledged in the examples and discussions in the present paper, although the principles of IPM apply to the management of a broad range of potentially harmful organisms – insects, plant pathogens, plant nematodes, snails, weeds, rodents, etc. – that interfere with profitable production of all crops.
Stern et al. (Reference Stern, Smith, van den Bosch and Hagen1959) systematically combined only insecticides and biological control to manage a single pest species. Integrated pest management specialists later devised management strategies for multiple species of pests and embedded additional pest avoidance and control tactics such as pest-resistant crops, crop rotation and water and fertilizer management. The IPM concept eventually evolved with a focus on the entire agroecosystem and consideration of social, economic, marketing and political factors affecting IPM adoption. Prokopy (Reference Prokopy1993) likened this evolution to climbing a stepladder: the first step was limited to the judicious and educated use of insecticides for managing a single class of pests; later steps evolved in hierarchical order with ascending levels of complexity and expanding spatial scales.
Different levels of integration
Using Kogan's (Reference Kogan1988, Reference Kogan1998) hierarchical system (Table 1), integration of pest control methods and decision making in IPM occurs at three basic levels: I – one pest species or a group of related pest species; II – multiple pest categories (e.g. insects, pathogens and weeds); and III – within the context of the total cropping system (ecosystem level integration) (Kogan, Reference Kogan1988, Reference Kogan1998). Prokopy and Croft (Reference Prokopy, Croft, Metcalf and Luckmann1994) added a fourth level: IV – integration of psychological, social, political and legal constraints to IPM (Table 1). Most IPM schemes have targeted a single pest species or a group of taxonomically related species (level I integration) or multiple pest species (level II integration) and not the ecosystem (level III integration) or local farming community (level IV integration). Without successful integration at all levels, IPM will not deliver fully on its promises.
Table 1. Levels of integration in IPM after Kogan (Reference Kogan1988, Reference Kogan1998) and Prokopy and Croft (Reference Prokopy, Croft, Metcalf and Luckmann1994)
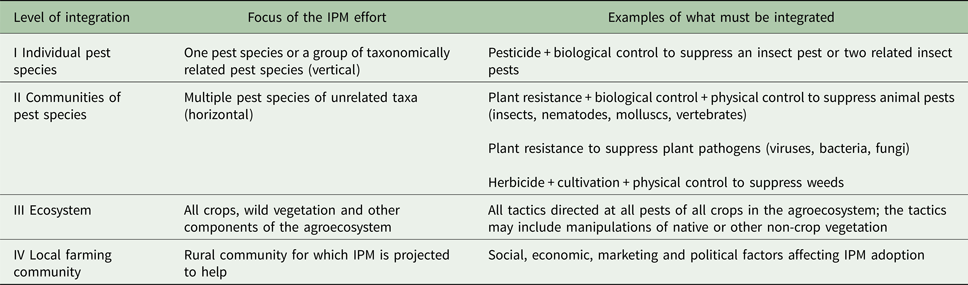
Harmonization of tactics
A fundamental paradigm of IPM is that the use of multiple tactics provides crop protection that is less risky, more technically efficient and more cost-effective than the use of a single tactic. Stern et al. (Reference Stern, Smith, van den Bosch and Hagen1959) advocated applying insecticides sparingly and only as necessary to prevent the spotted alfalfa aphid's population from surpassing an established economic injury level. Frugal use of insecticides saved the farmers money and caused less harm to natural enemies operating against the spotted alfalfa aphid and other alfalfa insect pests. Integrated crop protection programmes that have combined pesticides and biological control, as advocated by Stern et al. (Reference Stern, Smith, van den Bosch and Hagen1959), have been used successfully against many agricultural insects and also spider mites (Croft, Reference Croft1990). Harmonizing pesticides and biological control or other tactics to control a pest theoretically reduces the risk of crop loss if one of the tactics fails. If unfavourable weather reduced the effectiveness of natural enemies or chemical control, for example, the farmer should still benefit from a control tactic that is less weather-dependent such as plant resistance or crop rotation. Furthermore, by combining different tactics the probability that a pest will adapt to any one tactic should be less; this is based on evolutionary models which predict that the less a given control method challenges a pest, the longer it will take for the pest to adapt to the control method (Gould, Reference Gould1991). Liu et al. (Reference Liu, Chen, Collins, Onstad, Roush, Zhang, Earle and Shelton2014) provided empirical evidence that natural enemies slowed the development of insect pest resistance to ‘Bt crops’ engineered with genes of the soil bacterium Bacillus thuringiensis Berliner. In greenhouse studies, Liu et al. (Reference Liu, Chen, Collins, Onstad, Roush, Zhang, Earle and Shelton2014) showed that the insect predator Coleomegilla maculata (DeGeer), combined with non-Bt and unsprayed refuge plants, delayed resistance in the diamondback moth [Plutella xylostella (Linnaeus)] population to Bt broccoli (Brassica olercaea L.) plants, when compared with treatments without the predator.
Level I integration challenges
Mixing different control tactics randomly will not necessarily produce better results than a single tactic; in some cases, the combination may trigger antagonistic effects and potentially reduce crop yields and farmer profits (Kogan, Reference Kogan1988, Reference Kogan1998; Thomas, Reference Thomas1999; Ehler and Bottrell, Reference Ehler and Bottrell2000; Ehler, Reference Ehler2006). For example, while host-plant resistance and biological control are usually compatible, the morphological, physiological or chemical traits of insect-resistant cultivars may discourage or even cancel out the potentially beneficial effects of a natural enemy (Gould et al., Reference Gould, Kennedy and Johnson1991; Hare, Reference Hare, Fritz and Simms1992; Johnson and Gould, Reference Johnson and Gould1992; Bottrell, et al., Reference Bottrell, Barbosa and Gould1998; Thomas, Reference Thomas1999; Tandon, Reference Tandon, Upadhyay, Mukerji and Chamola2001; Kennedy and Gould, Reference Kennedy, Gould, Kogan and Jepson2007). The outcome of the interaction will depend on the specific relationship between the pests and their natural enemies and how the resistant plants affect both. Hare's (Reference Hare, Fritz and Simms1992) models show that, without natural enemies, the equilibrium density of a pest declines linearly with increasing levels of plant resistance. But when the relationship is additive, host-plant resistance and biological control act independently on a pest's equilibrium density. In other words, the biological interaction between the two tactics does not occur and mortality due to natural enemies is uniform across plant genotypes with different levels of resistance. By contrast, in antagonistic and synergistic relationships, resistance affects both pests and natural enemies. In extreme cases of antagonism (i.e. disruptive relationships), plant resistance could affect natural enemies more than pests and therefore reduce the effectiveness of biological control (Tandon, Reference Tandon, Upadhyay, Mukerji and Chamola2001).
Table 2 provides examples of possible outcomes when two pest control tactics, A and B, are combined. If tactic A alone achieved 40% pest control and tactic B alone achieved 50% control, then deployed together 90% control would indicate additive effects, >90% control would indicate synergistic (multiplicative) effects and <90% control would indicate antagonistic effects. Either additive or synergistic effects would suggest that the two IPM tactics are compatible. Antagonism, on the other hand, would suggest that the tactics may not be compatible. Some antagonism may be acceptable but not if one of the tactics significantly disrupts the effectiveness of another, resulting in hardship to the farmer. A commonly reported unacceptable level I antagonistic interaction is when an insecticide, which is effective against an insect pest, destroys natural enemies that contribute significantly to the pest's regulation (Hardin et al., Reference Hardin, Benrey, Coll, Lamp, Roderick and Barbosa1995). Fagan et al. (Reference Fagan, Hakim, Ariawan and Yuliyantiningsih1998) found that when rice insect pests were exposed simultaneously to the wolf spider [Lycosa pseudoannulata (Bösenberg and Strand)] and the insecticide monocrotophos, the treatments cancelled each other out. Szczepaniec et al. (Reference Szczepaniec, Raupp, Parker, Kerns and Eubanks2013) discovered that neonicotinoid insecticides have the potential to suppress plant defence genes, alter plant defence phytohormones and decrease plant resistance to unsusceptible herbivores on distantly related crop plants.
Table 2. Examples of possible outcomes when tactics A and B are used alone and in combination to control a pest

Level II integration challenges
Combining tactics horizontally across different pest groups can produce potentially complex interactions (Ehler and Bottrell, Reference Ehler and Bottrell2000; Ehler, Reference Ehler2006). Examples of negative interactions would be when an insecticide kills natural enemies that protect crops from insect pests or destroys insects that suppress crop weeds; when a fungicide destroys microbial antagonists of plant pathogens or kills predatory mites that control plant feeding mites; and, as recently discovered, when a pesticide suppresses plant resistance (Szczepaniec et al., Reference Szczepaniec, Raupp, Parker, Kerns and Eubanks2013). Entomologists have documented broad-spectrum ecological effects of pesticides on multiple pest and natural-enemy populations. van den Bosch et al. (Reference van den Bosch, Messenger and Gutierrez1982) coined this phenomenon the ‘trifaceted insecticide treadmill’ in reference to a target pest's resurgence, a secondary pest's outbreak and resistance in the target pest, or even one or more non-target pests. A non-chemical tactic could also trigger negative interactions. For example, switching to an improved crop cultivar with genetic resistance to an insect pest may control the insect effectively but aggravate a plant pathogen or vice versa (Bonman et al., Reference Bonman, Khush and Nelson1992). Or, as previously discussed, a crop cultivar that is resistant to an insect pest could deter natural enemies that regulate its abundance.
Levels III and IV integration challenges
Level III integration involves integrating all crops, wild vegetation and other components of the agroecosystem. Sections ‘Effects of local and regional landscape’ and ‘The agroecosystem as a living system’ of the present paper are devoted entirely to level III integration. However, as emphasized in the section ‘Sustaining the Integrated Pest Management Effort’, even if all pest control tactics are integrated in a compatible manner, the IPM scheme will not endure unless it is tailored for the local socioeconomic environment and can resist conflicting market forces (level IV integration).
Integrated pest management and sustainable agriculture
Integrated pest management is a complementary and necessary feature of sustainable agriculture, which aims to assure equitable, secure, sufficient and stable flows of both food and ecosystem services (Tilman et al., Reference Tilman, Cassman, Matson, Naylor and Polasky2002). Agriculture will achieve sustainability only if the agroecosystem maintains stable productivity while resisting major disturbances including pest ravages (Conway, Reference Conway and Barbier1993). Ecologists generally equate ecological stability with ecological resilience, defined as the degree to which a population or community returns to its previous configuration once the perturbation is removed (Pimm, Reference Pimm1991). Populations and communities with high resilience are expected to show relatively low levels of fluctuation and recover relatively quickly from disruptions (May, Reference May and May1976). Although ecological communities consistently reveal low resistance to species introduction (Herbold and Moyle, Reference Herbold and Moyle1986; Savidge, Reference Savidge1987; Nichols et al., Reference Nichols, Thompson and Schemel1990; Knapp et al., Reference Knapp, Matthews and Sarnelle2001), some exhibit relatively high levels of resilience following the initial disruption (Knapp et al., Reference Knapp, Hawkins, Ladau and McClory2005). In agroecosystems, IPM theoretically reduces the frequency and intensity of pest turbulence by eliminating disruptive pest control methods and enhancing ecosystem services that contribute to ecological resilience. For example, routine use of insecticides reduces the inherent resilience in high-yielding rice in tropical Asia by triggering ecological disruptions that cause major insect pest outbreaks (Kenmore et al., Reference Kenmore, Cariño, Perez, Dyck and Gutierrez1984; Heinrichs and Mochida, Reference Heinrichs and Mochida1984; Heong and Hardy, Reference Heong and Hardy2009; Bottrell and Schoenly, Reference Bottrell and Schoenly2012). Minimizing insecticide use and relying on plant resistance, natural enemies and habitat management as primary management components can contribute to the restoration of ecological resilience in high-yielding rice (Gallagher et al., Reference Gallagher, Kenmore, Sogawa, Denno and Perfect1994, Reference Gallagher, Ooi, Kenmore, Peshin and Dhawan2009; Matteson, Reference Matteson2000).
Ludwig et al. (Reference Ludwig, Hilborn and Walters1993) argued that achieving sustainability through scientific and technological progress is a daunting task, due primarily to wide variability and unpredictability of different components of the exploited ecosystem. Resource managers have traditionally used deterministic (non-random) age- or stage-structured population models to estimate harvest sizes (Clark, Reference Clark1985). However, as they develop and age, all biological populations experience random (stochastic) variation from abiotic (environmental) and biotic (ecological, anthropogenic) sources (Gatto, Reference Gatto1995). Incorporating stochastic variation into structured population models (i.e. stochastic demographic models) alters estimates of population growth rates, persistence and resilience that can ultimately affect community composition, species interactions, distributions and harvesting (Boyce et al., Reference Boyce, Haridas and Lee2006). An example of ecological complexity and unpredictability that upholds this ‘precautionary principle’ in agroecosystems is intraguild predation (IGP), which occurs when two or more predators, parasitoids or pathogens compete for the same prey or hosts. Intraguild predation is widespread in natural and agricultural systems (Polis et al., Reference Polis, Myers and Holt1989; Rosenheim et al., Reference Rosenheim, Kaya, Ehler, Marois and Jaffee1995; Arim and Marquet, Reference Arim and Marquet2004; Denno and Finke, Reference Denno, Finke, Brodeur and Boivin2006). However, many factors affect IGP's strength during the season and create difficulty in predicting if the multiple natural enemies are interacting harmoniously or antagonistically against crop pests. Integrated pest management will not contribute to long-term sustainability if it fails to find alternative tactics that reduce such uncertainty.
Ideas for increasing sustainability and reducing uncertainty have emerged from long-term agricultural trials and by infusing core evolutionary principles (Denison, Reference Denison2012). Because causal relationships are difficult to detect on working farms, determining which farmer interventions bring consistency in crop yields depends on scientific data from decades-long experiments on research farms. For example, at the Rothamsted Agricultural Research Station in the UK (the longest continuously active research farm), long-term data show that grain yields of wheat (Triticum aestivum L.) and barley (Hordeum vulgare L.) were sustained (and even increased) for nearly the entire 160 years in monoculture under annual applications of either organic or inorganic fertilizers (Jenkinson, Reference Jenkinson1991). The 160-year archive included enough DNA from two fungal pests to reveal past crop–pathogen epidemics linked to changes in air pollution (Bearchell et al., Reference Bearchell, Fraaije, Shaw and Fitt2005). By viewing sustainability through an evolutionary lens, Denison (Reference Denison2012) argued that solutions for improving crop yields may come from a better understanding of the trade-offs and how agroecosystems influence pest evolution. During the Green Revolution, when crop breeders ‘reversed’ past natural selection by developing shorter-stature, higher-yielding wheat and rice varieties, trade-offs came in the form of lower competitiveness from taller plants. Similar reversals, Denison (Reference Denison2012) argued, could involve breeding plants with less aggressive roots, leaving them with more resources to invest in grain. Many questions that agroecologists must address will require understanding adaptations of wild species in their natural environments (Denison, Reference Denison2012). Taking the long view invites IPM practitioners to build on lessons learned from long-term experiments and to apply evolutionary principles and ecological concepts leading towards improved agricultural productivity and sustainability.
Ludwig et al. (Reference Ludwig, Hilborn and Walters1993) argued that achieving sustainability will also require increased collaboration among environmental and social scientists. Such efforts can help guide decision makers and the public with options, costs and benefits (Ehrlich and Daily, Reference Ehrlich and Daily1993). Although collaboration will not guarantee the best possible outcome, it should lessen the level of farmer uncertainty, particularly if the precautionary principle (cited above) is exercised to guide actions (Costanza, Reference Costanza1993).
Effects of local and regional landscape
The landscape in which a crop is embedded plays an important role in defining and developing the IPM system (Goodell, Reference Goodell2009). Although three-quarters of the estimated 280 million farms in low-income and lower middle-income countries are <2 ha in size (Lowder et al., Reference Lowder, Skoet and Raney2016), they are often highly diverse with a dominant food crop such as rice, maize (Zea mays L.), or potato (Solanum tuberosum L.), garden vegetables and fruit trees and often wandering or fenced livestock (Vandermeer, Reference Vandermeer1989). Larger farms may include a cash crop such as cotton (Gossypium sp.). The neighbouring crops, weeds and wild habitat on or surrounding the farm potentially influence the dynamics of a crop's pests and natural enemies. Indeed, local diversification, from within field (polyculture) and or around field (e.g. hedgerow) sources, may enhance natural pest control and compensate for low-complexity at the larger landscape level (Chaplin-Kramer and Kremen, Reference Chaplin-Kramer and Kremen2012; Morandin and Kremen, Reference Morandin and Kremen2013). An insect pest with a wide plant host range may feed or reproduce on several or even all of the area's crops and sometimes crop weeds and vegetation in nearby wild habitat and the pest may be ‘tracked’ by natural enemies as it switches host plants (Settle et al., Reference Settle, Ariawan, Asuti, Cahyana, Hakim, Hindayana and Lestari1996; Barbosa, Reference Barbosa and Barbosa1998; Letourneau, Reference Letourneau and Barbosa1998; Landis et al., Reference Landis, Wratten and Gurr2000; Kennedy and Gould, Reference Kennedy, Gould, Kogan and Jepson2007). Some crops serve as aggregation and reproduction sites for natural enemies and will sustain the enemies until food and habitat requisites are exhausted (Kennedy and Gould, Reference Kennedy, Gould, Kogan and Jepson2007). The enemies will then disperse to other crops or wild habitats. Weeds within or near a crop may host pathogens and invertebrate pests. Weeds are particularly important in maintaining or increasing populations of non-mobile pest organisms such as Verticillium and Fusarium pathogens, and root-knot and cyst nematodes (Norris, Reference Norris, Kogan and Jepson2007). They also may serve as refuges for natural enemies (Norris and Kogan, Reference Norris and Kogan2000). In tropical irrigated Asian rice, for example, egg parasitoids Anagrus spp. and Oligosita spp. of pest leafhoppers and planthoppers, respectively, live in nearby Echinochloa- and Leptochloa-rich habitats, respectively (Yu et al., Reference Yu, Heong, Hu, Barrion, Hokyo and Norton1996). Other weedy grasses such as Paspalum spp. bordering tropical rice support sizeable populations of gryllid crickets [e.g. Anaxipha longipennis (Serville) and Metioche vittaticollis (Stål])] that are efficient predators of rice leaffolder [Cnaphalocrocis medinalis (Guenée) and Marasmia spp.] eggs (de Kraker, Reference de Kraker1996). Tropical Asian rice also harbours a rich mix of aquatic predators (Settle et al., Reference Settle, Ariawan, Asuti, Cahyana, Hakim, Hindayana and Lestari1996; Schoenly et al., Reference Schoenly, Justo, Barrion, Harris and Bottrell1998; Wilson et al., Reference Wilson, Watts and Stevens2008) that attack the crop's pests (Nakasuji and Dyck, Reference Nakasuji and Dyck1984; Settle et al., Reference Settle, Ariawan, Asuti, Cahyana, Hakim, Hindayana and Lestari1996; Schoenly et al., Reference Schoenly, Justo, Barrion, Harris and Bottrell1998). Therefore, an IPM programme developed for a crop without considering the effects of the neighbouring crops, wild vegetation and aquatic systems in or nearby the crop could produce disappointing results.
Relationship in plant diversity and pest abundance
Pimentel's (Reference Pimentel1961) seminal paper, which showed that plant mixtures prevented outbreaks of insect pests in collards (Brassica oleracea L.), stimulated interest in determining the relationship in the vegetational diversity of agroecosystems and pest abundance. Risch et al. (Reference Risch, Andow and Altieri1983) evaluated 150 published studies that measured the effect of crop diversification on insect pests in 140 annual crops (harvested and destroyed in 1 year or less of planting) and 58 perennial crops such as fruit trees that grew in the same place for years. Of the 198 species of pests in the studies, 53% were less abundant in the more diverse cropping systems, 18% were more abundant in the diverse systems, 9% showed no difference and 20% showed a variable response. In Andow's (Reference Andow1991) evaluation of 209 published studies, 52% of the 287 herbivore species had lower densities on plants in polycultures than in monocultures; only 15% (44 species) had higher densities on plants in polycultures than monocultures. Letourneau et al. (Reference Letourneau, Armbrecht, Rivera, Lerma, Carmona, Daza, Escobar, Galindo, Gutiérrez, López, Mejía, Rangel, Rangel, Rivera, Saavedra, Torres and Trujillo2011) used meta-analysis to evaluate 552 experiments in 45 journal articles to test if plant diversification schemes reduced crop-feeding pests and/or increased their natural enemies. Overall, pest suppression, natural enemy enhancement and crop damage suppression effects were significantly stronger in the more diverse cropping systems. Although analysis by Poveda et al. (Reference Poveda, Gómez and Martínez2008) of 62 published studies of crop diversification practices showed that diversification enhanced natural enemies, reduced pest pressure and increased yield respectively in only 52, 53 and 32% of the cases, the authors concluded that the appropriate mix of plant diversity could reduce pests and maintain yield effectively.
Manipulating plant diversity to manage pests
Before modern pesticides, crop protection specialists often sought plant diversification strategies that would mitigate pest damage. The emphasis waned after modern post-World War II pesticides proliferated, but contemporary researchers have renewed efforts to combine biological pest control with habitat manipulation and landscape-level management (Barbosa, Reference Barbosa and Barbosa1998; Pickett and Bugg, Reference Pickett and Bugg1998; Philips et al., Reference Philips, Rogers and Kuhar2014). Recent examples of successful plant diversification schemes aimed at reducing pests on resource-limited farms are the ‘push–pull’ strategy for African cereal crops and the planting of nectar-rich companion plants in monocultures of rice in Asia. The push–pull strategy in Africa (Khan et al., Reference Khan, Midega, Hooper and Pickett2016) uses native companion plants such as Napier grass (Pennisetum purpureum Schumach) – the ‘pull’ – to attract cereal stemborers (of the families Crambidae, Pyralidae and Noctuidae), while simultaneously driving them away from the cereal crop using a repellent intercrop like Desmodium spp. – the ‘push’. Chemicals released by the Desmodium spp. roots control the parasitic African witchweed [Striga hermonthica (Del.)] by inducing suicidal germination of the witchweed's seeds in the soil and thereby preventing the weed from attaching to the cereal plants’ roots. Both Napier grass and Desmodium spp. provide animal fodder, boosting milk production when fed to dairy animals, and Desmodium spp. enhance soil fertility by fixing nitrogen, while acting as a cover crop to retain soil moisture. The push–pull strategy complements traditional mixed cropping systems of Africa and thousands of resource-limited farmers have adopted it (Khan and Pickett, Reference Khan, Pickett, Gurr, Wratten and Altieri2004; Cook et al., Reference Cook, Khan and Pickett2007; Khan et al., Reference Khan, Midega, Amudavi, Hassanali and Pickett2008, Reference Khan, Midega, Hooper and Pickett2016; ICIPE, 2013).
In Thailand, China and Vietnam, multi-site 4-year studies replicated on farmers’ fields revealed impressive benefits when farmers planted locally appropriate nectar-producing dicotyledonous plants around their rice fields to increase biological control of insect pests (Gurr et al., Reference Gurr, Lu, Zheng, Xu, Zhu, Chen, Yao, Cheng, Zhu, Catindig, Villareal, Chien, Cuong, Channoo, Chengwattana, Lan, Hai, Chaiwong, Nicol, Perovic, Wratten and Heong2016). The flowering companion plants (species varied among locations) provided nectar, pollen and shelter to important species of natural enemies. Farmers planted the nectar-rich flowering plants on the rice bunds (narrow elevated bands of soil arranged around rice fields to retain water) and field margins to conserve natural enemies. This inexpensive intervention significantly reduced populations of two major insect pests, brown planthopper (Nilaparvata lugens Stål) and white backed planthopper [Sogatella furcifera (Horváth)], reduced insecticide applications by 70%, increased grain yields by 5% and enlarged farmer profits by 7.5%. Encouraged by the results, the Vietnamese government launched media campaigns to encourage adoption of the practice (Heong et al., Reference Heong, Escalada, Chien and Cuong2014).
Whether the recently adopted push–pull strategy for African cereal crops and the planting of nectar-rich companion plants in monocultures of rice in Asia will endure remains to be seen. Nevertheless, both are good examples of low-cost IPM programmes that have evolved from a local landscape view and integrated into the resource-limited farming operations.
From ecological theory to practice
Ecologists have offered various hypotheses to explain why pests and natural enemies often respond differently to plant mixtures than to pure stands (for a summary, see Poveda et al., Reference Poveda, Gómez and Martínez2008). For example, Root's (Reference Root1973) ‘resource concentration hypothesis’ postulates that lower herbivore abundance prevails in diverse vegetation because the herbivore's host plants are less concentrated and therefore harder to find. Also, some plant species in the diverse system may serve as repellents, as illustrated by Desmodium spp. in the African push–pull system (Cook et al., Reference Cook, Khan and Pickett2007). Root's (Reference Root1973) ‘enemy hypothesis’ advocates that natural enemies occupying the middle trophic levels determine the abundance of herbivores in a habitat. The diverse system offers more alternate prey and plant food sources such as pollen and nectar to generalist natural enemies, which in turn reduce pest abundance. The ‘trap crop hypothesis’ suggests that alternative host plants (e.g. Napier grass in the African push–pull system, Cook et al., Reference Cook, Khan and Pickett2007) lure pests away from the primary crop plants (Vandermeer, Reference Vandermeer1989). The ‘barrier crop hypothesis’ advocates that taller non-host plants in a mixed cropping system obstruct movement of insect pests, thereby reducing their chance of colonizing the primary crop plants (Perrin and Phillips, Reference Perrin and Phillips1978). The ‘associational resistance hypothesis’ states that non-host plants release odour-masking substances that make the crop ‘invisible’ to insect pests (Tahvanainen and Root, Reference Tahvanainen and Root1972).
Ecological theory helps to design field experiments that measure the effects of different non-host or alternative host plants on pests and natural enemies and their suitability in plant diversification schemes. However, high variability in the responses of pests and natural enemies to plant diversification impedes efforts to devise general rules about expected outcomes (Andow, Reference Andow and Lockeretz1983, Reference Andow1991; Kareiva, Reference Kareiva, Denno and McClure1983; Risch et al., Reference Risch, Andow and Altieri1983; Coll and Bottrell, Reference Coll and Bottrell1994; Landis et al., Reference Landis, Wratten and Gurr2000; Poveda et al., Reference Poveda, Gómez and Martínez2008; Letourneau et al., Reference Letourneau, Armbrecht, Rivera, Lerma, Carmona, Daza, Escobar, Galindo, Gutiérrez, López, Mejía, Rangel, Rangel, Rivera, Saavedra, Torres and Trujillo2011). The diversification schemes should be evaluated on a case-by-case basis. The crop species, non-crop plant species, pests to be managed, natural enemies, climate, farmer resources and other factors will determine what specific mix of vegetation is best suited for a specific farming system (Poveda et al., Reference Poveda, Gómez and Martínez2008). The need to develop practical schemes that harmoniously blend into individual farming operations and yield beneficial returns is implicit. Along with this line, Letourneau et al. (Reference Letourneau, Armbrecht, Rivera, Lerma, Carmona, Daza, Escobar, Galindo, Gutiérrez, López, Mejía, Rangel, Rangel, Rivera, Saavedra, Torres and Trujillo2011) found that while plant diversification schemes generally reduced pests and pest damage, and enhanced natural enemies, they slightly but significantly reduced crop production because the intercrops or non-crop plants effective in reducing pest abundance used space that would have otherwise been filled by the main crop. In some cases, the savings in pesticides may outweigh the loss in yield but in other cases, they may not. Individual farmers may not be able to implement some plant diversification schemes without the cooperation of farmer associations or regional organizations, for example, that control regional irrigation systems and water delivery rates.
The monoculture alternative
Diversifying the cropping system has many advantages and may be necessary to achieve the most effective crop protection in some situations. However, this does not mean that crop monocultures are necessarily inherently vulnerable to pests. For example, monocultures of rice in tropical Asia with reasonable levels of insect resistance rarely experience damaging insect outbreaks unless treated with natural enemy-disrupting insecticides (Heong and Hardy, Reference Heong and Hardy2009; Bottrell and Schoenly, Reference Bottrell and Schoenly2012). The brown planthopper, the potentially most damaging insect pest of tropical Asian rice, occasionally reaches moderately high densities even on resistant cultivars during periods of high predation (Schoenly et al., Reference Schoenly, Cohen, Heong, Litsinger, Barrion and Arida2010). However, unless disturbed by insecticides, natural enemies flourish in the rice monocultures and prevent the brown planthopper and other potentially harmful insect pests from attaining damaging levels (Kenmore et al., Reference Kenmore, Cariño, Perez, Dyck and Gutierrez1984; Cohen et al., Reference Cohen, Schoenly, Heong, Justo, Arida, Barrion and Litsinger1994; Settle et al., Reference Settle, Ariawan, Asuti, Cahyana, Hakim, Hindayana and Lestari1996). On the other hand, Gurr et al. (Reference Gurr, Lu, Zheng, Xu, Zhu, Chen, Yao, Cheng, Zhu, Catindig, Villareal, Chien, Cuong, Channoo, Chengwattana, Lan, Hai, Chaiwong, Nicol, Perovic, Wratten and Heong2016) showed that diversifying rice monocultures with nectar-rich plants may yield significant pest management benefits in some areas of tropical Asia.
Mixing different genetic forms (multiline cultivars and cultivar mixtures) of the same crop species might be a suitable alternative to plant species diversification, especially for managing certain diseases of small grains (Browning et al., Reference Browning, Simons, Torres, Horsfall and Cowling1977; Mundt, Reference Mundt2002). In China, rice blast disease caused by the fungus Pyricularia oryzae Cavara was 94% less severe in cultivar mixtures of rice than in single cultivar stands (Zhu et al., Reference Zhu, Chen, Fan, Wang, Li, Chen, Fan, Yang, Hu, Leung, Mew, Teng, Wang and Mundt2000). Disease-susceptible rice cultivars planted in mixtures with resistant cultivars had 89% greater yield than when planted alone. The mixtures achieved an overall higher yield of 18% with mixtures, but this may have been an artefact due to the sub-optimal density of the monoculture control (Denison et al., Reference Denison, Kiers and West2003). Mixing rice cultivars may be an effective alternative to fungicides and may also reduce the dependence on high levels of plant disease resistance (Zhu et al., Reference Zhu, Chen, Fan, Wang, Li, Chen, Fan, Yang, Hu, Leung, Mew, Teng, Wang and Mundt2000). However, cultivar mixtures are more useful under some conditions than others and experimental methodology (especially problems of scale) may be crucial in evaluating their potential (Mundt, Reference Mundt2002).
The agroecosystem as a living system
Although modern crops may contain fewer species than their wild relatives (Chen et al., Reference Chen, Langellotto, Barrion and Cuong2013, Reference Chen, Gols and Benrey2015), they still support highly complex communities of organisms and food webs. Agrobiodiversity plays essential roles in soil nutrient cycling, erosion control, mineral retention, regulation of pests, pollination, water quality and other ecological services (Power, Reference Power1999; Tilman et al., Reference Tilman, Cassman, Matson, Naylor and Polasky2002; Gurr et al., Reference Gurr, Wratten and Luna2003; Brussaard et al., Reference Brussaard, de Ruiter and Brown2007; Smukler et al., Reference Smukler, Sánchez-Moreno, Fonte, Ferris, Klonsky, O'Geen, Scow, Steenwerth and Jackson2010; Tomich et al., Reference Tomich, Brodt, Ferris, Galt, Horwath, Kebreab, Leveau, Liptzin, Lubell, Merel, Michelmore, Rosenstock, Scow, Six, Williams and Yang2011; Gliessman, Reference Gliessman2015). To capture the ecosystem services required for productive sustainable agriculture, agroecosystems must be managed as living systems (Matson et al., Reference Matson, Parton, Power and Swift1997).
Insights into the agrofoodweb
Heckman (Reference Heckman1979) documented 590 species of plants (besides rice) and animals in a single irrigated rice field in Thailand and his inventory excluded insects and spiders, which are the most common invertebrate species in rice (Cohen et al., Reference Cohen, Schoenly, Heong, Justo, Arida, Barrion and Litsinger1994). Cohen et al. (Reference Cohen, Schoenly, Heong, Justo, Arida, Barrion and Litsinger1994), Settle et al. (Reference Settle, Ariawan, Asuti, Cahyana, Hakim, Hindayana and Lestari1996), O'Malley (Reference O'Malley, Batzer, Rader and Wissinger1999) and Bambaradeniya et al. (Reference Bambaradeniya, Edirisinghe, De Silva, Gunatilleke, Ranawana and Wijekoon2004) recorded more than 640, 760, 500 and 490 taxa of macro-invertebrates in Philippine, Indonesian, American and Sri Lankan irrigated rice fields, respectively. Pathummal Beevi et al. (Reference Pathummal Beevi, Lyla and Narendran2000) and Sebastian et al. (Reference Sebastian, Mathew, Pathummal Beevi, Joseph and Biju2005) recorded 84 and 92 species, respectively, of hymenopteran parasitoids and spiders, respectively, in Indian irrigated rice. In a study aimed at estimating total macro-invertebrate richness in an irrigated Philippine rice field, Schoenly and Barrion (Reference Schoenly and Barrion2016) recorded 340 rice plant canopy taxa and 60 aquatic taxa over two cropping seasons, representing inventory completeness of approximately 82% for the canopy and 98% for the floodwater. Comparable inventories of crop soil organisms have been limited (Hughes et al., Reference Hughes, Hellman, Ricketts and Bohannan2001) although it is assumed that a handful of rich organic soil may contain millions of organisms representing hundreds of different species of bacteria, fungi, protozoa, algae and micro-invertebrates (Behan-Pelletier and Newton, Reference Behan-Pelletier and Newton1999).
Functional traits of individual species, rather than functional groups or species richness per se, is the dimension of biodiversity most directly related to ecosystem functioning (Tilman and Downing, Reference Tilman and Downing1994; Naeem and Wright, Reference Naeem and Wright2003; Cadotte et al., Reference Cadotte, Carscadden and Mirotchnick2011). The species may influence ecosystems directly (e.g. pollination, predation, parasitism, herbivory, competition) or indirectly by responding to local environmental changes such as microclimate or human disturbances (Chapin et al., Reference Chapin, Zavaleta, Eviner, Naylor, Vitousek, Reynolds, Hooper, Lavorel, Sala, Hobbie, Mack and Díaz2000). The functional traits govern the impacts of species on the environment and also species fitness (Lavorel and Garnier, Reference Lavorel and Garnier2002; Wood et al., Reference Wood, Karp, DeClerck, Kremen, Naeem and Palm2015). Erosion of the functional traits through species losses may negatively impact community and ecosystem processes (Chalcraft and Resetarits, Reference Chalcraft and Resetarits2003).
Studies of food webs are highly labour intensive and require specialized taxonomic services and trophic specialists but may be essential to clarify the functional traits of individual species (Neuenschwander et al., Reference Neuenschwander, Hennessey and Herren1987; Cohen et al., Reference Cohen, Schoenly, Heong, Justo, Arida, Barrion and Litsinger1994; Rosenheim et al., Reference Rosenheim, Kaya, Ehler, Marois and Jaffee1995). Understanding interactions in the food web offer insights into the food sources of individual species and to whom they, in turn, serve as food and can identify pathways to phenomena such as top-down, bottom-up and indirect effects (Morin, Reference Morin2011). Traditional food webs have been depicted as qualitative, who-eats-whom networks that rarely identify the strong interactors among community members (Bowman et al., Reference Bowman, Hacker and Cain2017). Beginning with Paine (Reference Paine1966), however, manipulative field experiments have determined that energy flow may be governed by one or more species coined by Paine (Reference Paine1969) as ‘keystone species’. These domineering species maintain community diversity by exerting strong direct and indirect effects on inferior species that could otherwise dominate the community (Bowman et al., Reference Bowman, Hacker and Cain2017). Keystone species have mixed impacts on different functional groups in cropping systems. In a cropping system in Mexico planted to maize and squash (Cucurbita moschata Duchesne), the dominant tropical fire ant [Solenopsis geminata (Fabricius)] significantly reduced populations of insect herbivores and, to a lesser extent, arthropod predators (Risch and Carroll, Reference Risch and Carroll1982). The aggressive fire ant had multiple impacts on the other predators, consuming some and competitively excluding others by eating their available prey. Kaplan and Eubanks (Reference Kaplan and Eubanks2002) found that the imported red fire ant [Solenopsis invicta (Buren)], an invasive species in the USA, increased survival and density of the cotton aphid (Aphis gossypii Glover) by releasing the aphid pest from predation by lady beetle larvae.
Predicting how the environment and management practices affect important ecosystem services in the agroecosystem requires knowledge of which organisms produce specific services such as pollination or biological control (Zhang et al., Reference Zhang, Ricketts, Kreman, Carney and Swinton2007; Power, Reference Power2010; Kremen and Miles, Reference Kremen and Miles2012; Wood et al., Reference Wood, Karp, DeClerck, Kremen, Naeem and Palm2015). Traditional food webs only depict linkages between trophic levels (i.e. vertical relationships), such as predator–prey, plant–herbivore and parasitoid–host interactions (Bowman et al., Reference Bowman, Hacker and Cain2017). However, any complex ecological community includes many non-trophic (horizontal) interactions (Morin, Reference Morin2011), such as competition, host-switching and mutualism. The more recently introduced ‘interaction web’ depicts the broader set of trophic and non-trophic pathways that occur among members of an ecological community (Bowman et al., Reference Bowman, Hacker and Cain2017). The interaction web of an agroecosystem in Fig. 1 (modified from Norris et al., Reference Norris, Caswell-Chen and Kogan2003) shows the traditional set of interconnected food chains (i.e. detritus, crop, weed) plus non-trophic and other interactions that might be overlooked or sloughed off as unimportant (e.g. services of detritivores in biological control, host switching by insect herbivores, IGP among natural enemies of pest species, allelopathy between crop and weed species, plant pollination and other mutualistic networks). In each food chain, every organism occupies a trophic level defined by how many energy transfers separate it from the basic input of the chain. Crop plants and weeds make their own organic molecules and are the agroecosystem's foundation producers (‘basal species’). The assemblage of consumers (herbivores, predators, parasitoids, hyperparasitoids, vertebrates) supported by the crop plants and weeds must eat other organisms to obtain their organic molecules. Detritus, which is dead particulate organic matter (crop plant and weed debris, bodies or fragments of dead organisms, animal faeces, etc.), supports decomposers (microbes), scavengers (invertebrates, including alternate prey) and sometimes vertebrates.

Fig. 1. Simplified interaction web showing energy flow within and between the detritus, crop and weed chains (modified from Norris et al., Reference Norris, Caswell-Chen and Kogan2003). Vertical arrows depict trophic links (consumer–prey, omnivory) and horizontal arrows depict non-trophic links (competition, host switching) between members of the crop and weed food chains. A small circle on the top left corner of a secondary consumer box (i.e. middle species or top predator) depicts cannibalism or intraguild predation among its members.
Using standard food web terminology (Cohen, Reference Cohen1978; Briand, Reference Briand1983), the left-hand column of Fig. 1 shows the different links in the food chain, starting with basal species (crop or weed and detritus) at the bottom and ascending upwards with top predators at the summit. Insect and other herbivore pests and pathogens are ‘middle species’. Also included in the middle species categories are insects and other organisms that serve as beneficial predators and parasitoids, and prey and hosts for the predators and parasitoids. Certain insectivorous frogs, birds and bats, often ignored in IPM programmes, are major top predators of insect pests in cotton, cacao (Theobroma cacao L.) and rice ecosystems (Cleveland et al., Reference Cleveland, Betke, Federico, Frank, Hallam, Horn, López, McCracken, Medellín, Moreno-Valdez, Sansone, Westbrook and Kunz2006; Maas et al., Reference Maas, Clough and Tscharntke2013; Zou et al., Reference Zou, de Kraker, Bianchi, van Telgen, Xiao and van der Werf2017). Other species often overlooked in IPM programmes are the detritivorous insects that feed on decaying organic matter, including post-harvest crop residues left in the field (i.e. middle species of the detritus chain, Fig. 1). In tropical Asian rice, these insects play a crucial role in biological control by serving as alternate prey for important generalist predators (e.g. spiders) that regulate potentially damaging insect pests such as the brown planthopper (Settle et al., Reference Settle, Ariawan, Asuti, Cahyana, Hakim, Hindayana and Lestari1996).
Food webs would have greater application in IPM decision making if dynamics of pest–natural enemy interactions during the cropping cycle were better understood. A good starting point is to compare food webs that have been constructed at several sites on multiple sampling dates using the same set of methods and data organization. At five sites on Luzon, Philippines, Schoenly et al. (Reference Schoenly, Cohen, Heong, Litsinger, Aquino, Barrion and Arida1996b) assembled site- and time-specific food webs from a cumulative 687-taxa, 10 000 link web of wetland rice and weekly arthropod samples. A site-specific food web accumulates data over different sampling periods (e.g. one crop season) within specified spatial limits, whereas a time-specific web is based on sampling of a single, relatively short time period (e.g. one stage of crop maturity) (Schoenly and Cohen, Reference Schoenly and Cohen1991). At each of the Philippine sites, Schoenly et al. (Reference Schoenly, Cohen, Heong, Litsinger, Aquino, Barrion and Arida1996b) found that herbivores increased faster than predators and parasitoids and predators colonized rice earlier than parasitoids. Over the cropping cycle, the potential spider prey and the parasitoids averaged 42 and four taxa, respectively, in the five site webs. However, in the time-specific versions, average ranges of the prey taxa of spiders and the parasitoids dropped to 33–69% (13–28) and 25–50% (2–3), respectively, of the site-specific values. Because site-specific webs tend to overestimate numbers of prey per enemy, predators that are identified as polyphagous from site lists may be dismissed prematurely from consideration for biocontrol campaigns. For example, the polyphagy of spiders is one reason why they are less favoured than parasitoids as potential biocontrol agents for many cropping systems (Dent, Reference Dent2000). Clearly, time-specific webs are more sensitive than site-specific webs in revealing prey or host range of natural enemies and the timing of specific enemy–prey interactions (Schoenly and Cohen, Reference Schoenly and Cohen1991). Similarly, molecular tools (e.g. protein-marking enzyme-linked immunosorbent assay (ELISA), polymerase chain reaction (PCR)-based DNA barcoding) can improve the resolution of food web interactions in agroecosystems (Boyer et al., Reference Boyer, Snider and Wratten2016). Using the ELISA method, for example, Mansfield and Hagler (Reference Mansfield and Hagler2016) found that three common insect predators of cotton plantations were also facultative scavengers and that scavenging was more prevalent than predation. Building on field observations (Settle et al., Reference Settle, Ariawan, Asuti, Cahyana, Hakim, Hindayana and Lestari1996), molecular tools have also confirmed early-season predation in agroecosystems, often involving beneficial alternative prey that sustain predators before pest build-up (Athey et al., Reference Athey, Dreyer, Kowles, Penn, Sitvarin and Harwood2016). Clearly, the agrofoodweb can be used to clarify temporal changes in species interactions. However, understanding and predicting how changing abundances of a resurging pest, for example, influences the remaining members of the community will require manipulative field experiments to identify the mechanisms involved. When combined with path analysis and alternative food-web models (Wootton, Reference Wootton1994), this approach may help reveal the mechanisms by which resurgent pests directly and indirectly affect other species in the agroecosystem, evaluate alternative causal hypotheses and predict which remaining members are strong or weak interactors.
Designing integrated pest management for the living agroecosystem: level III integration
Any manipulation in the agroecosystem can potentially alter food webs. Application of a fertilizer or pesticide, adoption of a new crop variety, rotation of crops, modification of row-spacing or irrigation schemes, destruction of wild habitat abutting crops and other management practices may cause a shift in the status of one or more pest species and their natural enemies. The application of a pesticide can disrupt the population dynamics of multiple species, reshaping the entire food web (Cohen et al., Reference Cohen, Schoenly, Heong, Justo, Arida, Barrion and Litsinger1994; Schoenly et al., Reference Schoenly, Cohen, Heong, Arida, Barrion, Litsinger, Polis and Winemiller1996a). A short-term increase in a fertilizer can significantly modify community structure, trophic interactions and ecosystem function, although the immediate impact may be subtle and unpredictable. Fertilization is known to produce a direct bottom-up impact on the structure and composition of arthropod communities (Wimp et al., Reference Wimp, Murphy, Finke, Huberty and Denno2010). Fertilization may also have an indirect bottom-up impact on natural enemies via enrichment of detritivores, which serve as important food sources for predators in irrigated rice of tropical Asia (Yasumatsu et al., Reference Yasumatsu, Hashimoto and Chang1979; Settle et al., Reference Settle, Ariawan, Asuti, Cahyana, Hakim, Hindayana and Lestari1996). Certain nutrients can lead to feedback loops that not only impact detritivores but also ultimately change rates of plant production and decomposition via increasingly greater impacts on natural enemies. In a heavily fertilized crop such as hybrid rice, the detritivores and also natural enemies may especially benefit from the nitrogen input. However, nitrogen also increases the fitness and acceleration of rice pests such as planthoppers (Lu and Heong, Reference Lu, Heong, Heong and Hardy2009). Nitrogen-enriched plants can significantly enhance the size, performance and abundance of herbivorous insects (Awmack and Leather, Reference Awmack and Leather2002) and increase the potential for outbreaks of pests such as planthoppers in Asian rice (Lu and Heong, Reference Lu, Heong, Heong and Hardy2009).
The IPM strategy should incorporate non-disruptive practices that improve and maintain pest protection, pollination, nutrient cycling, water and soil conservation and other ecosystem services important in subsidizing sustainability of the agroecosystem (Nicholls et al., Reference Nicholls, Parrella and Altieri2001; Nicholls and Altieri, Reference Nicholls, Altieri, Kogan and Jepson2007). A good starting point is to concentrate on the natural factors (biological control agents, native habitat, etc.) and farming practices and inputs (cultivation, crop rotation, crop variety, fertilization, etc.) known to affect the primary pests (plant pathogens, insects, weeds, rodents, etc.) (Smith and van den Bosch, Reference Smith, van den Bosch, Kilgore and Doutt1967). It is especially important to exploit natural enemies, pest-resistant plants, allelopathy and companion plants that regulate the pests’ population growth. These natural agents may require little if any farmer investment and may reduce pesticide costs significantly.
With a reasonable understanding of the natural factors and management practices that operate against the primary pests, IPM specialists can test the IPM strategy on a small scale in representative farming areas – this is how every ecologically based IPM strategy has evolved. For pests that function as metapopulations spread over wide areas (Jervis, Reference Jervis1997; Winder and Woiwood, Reference Winder, Woiwood, Kogan and Jepson2007), the size of their migratory range may dictate the boundary of the agroecosystem to be managed (Apple, Reference Apple, Horsfall and Cowling1977). Regardless of the specific manipulations and scale, farmers must understand and accept the IPM strategy as an integral part of the management of their farms or they will resist it.
In larger crop landscapes that vary in degree of habitat fragmentation and isolation, metacommunity theory (Leibold et al., Reference Leibold, Holyoak, Mouquet, Amarasekare, Chase, Hoopes, Holt, Shurin, Law, Tilman, Loreau and Gonzalez2004; Holyoak et al., Reference Holyoak, Leibold, Mouquet, Holt, Hoopes, Holyoak, Leibold and Holt2005) may hold the key to determining if different farmer practices are compatible with established IPM practices across different spatial scales. Metacommunity theory seeks to identify important processes that generate multi-species patterns at different scales. At small spatial scales, crop field connectivity ensures between-field and between-season exchanges of crop invertebrates and pathogens and bordering vegetation provides year-round refugia for certain pests and their natural enemies. In irrigated rice, local water sources (e.g. irrigation canals, drainage ditches, streams) contain aquatic invertebrates that recolonize rice fields, including predators that eat rice pests (Settle et al., Reference Settle, Ariawan, Asuti, Cahyana, Hakim, Hindayana and Lestari1996; Schoenly et al., Reference Schoenly, Justo, Barrion, Harris and Bottrell1998, Reference Schoenly, Cohen, Heong, Litsinger, Barrion and Arida2010). At large spatial scales, spatially isolated populations within a metapopulation are linked by dispersal and wind-assisted migration and may explain, for example, how sustained synchronous outbreaks of the brown planthopper occur in different Asian countries (Bottrell and Schoenly, Reference Bottrell and Schoenly2012). Large patches of crop fields are more likely to receive immigrants and rescue populations from local extinction (Brown and Kodric-Brown, Reference Brown and Kodric-Brown1977; Thomas and Jones, Reference Thomas and Jones1993). When pests are mobile, successful management may require collective action by neighbouring farmers, particularly when farm sizes are small and close together (Parsa et al., Reference Parsa, Morse, Bonifacio, Chancellor, Condori, Crespo-Pérez, Hobbs, Kroschel, Ba, Rebaudo, Sherwood, Vanek, Faye, Herrera and Dangles2014; Coll and Wajnberg, Reference Coll, Wajnberg, Coll and Wajnberg2017b), such as in developing countries. Because of their recognizable spatial boundaries, agroecosystems make ideal candidates for testing metacommunity models developed around different assumptions of community assembly, such as patch dynamics, species sorting and mass effects (Baiser et al., Reference Baiser, Buckley, Gotelli and Ellison2013).
Taxonomic services
Accurate identification of pests, natural enemies and other organisms is essential in designing, implementing and sustaining IPM. Because taxonomic services are often inadequate in developing countries (Cock, Reference Cock2011), farmers may seek unqualified sources for identifying pests and natural enemies, as Heong (Reference Heong, Wong and De Los Reyes2013) found in rice-producing areas of tropical Asia. Various organizations are working to improve and increase taxonomic services in developing countries (Cock, Reference Cock2011). In addition, DNA barcoding, which uses specific gene tags (or barcodes) to distinguish one species from another (Hebert and Gregory, Reference Hebert and Gregory2005; Savolainen et al., Reference Savolainen, Cowan, Vogler, Roderick and Lane2005), could eventually have potential in improving identification services in IPM and other agricultural applications (Cock, Reference Cock2011). The Barcode of Life Data System (BOLD, htttp:// www.boldsystems.org) provides informatics tools and databases in support of researchers building libraries of DNA barcodes. However, DNA barcoding is no substitute for conventional taxonomy. It should be used alongside conventional taxonomy and alternative forms of molecular systematics so errors in identification can be detected. Considerable research investment is required before the taxonomic method has practical value in improving identification services in resource-limited agricultural areas. However, it has the potential to provide standardized and high-tech identification tools to large communities of end-users (Savolainen et al., Reference Savolainen, Cowan, Vogler, Roderick and Lane2005) including extension workers helping farmers to identify pests and natural enemies.
Apps for smartphones and other hand-held devices are being used in many areas to identify various groups of animals and plants and to collect, analyse and store complex ecological information (Teacher et al., Reference Teacher, Griffiths, Hodgson and Inger2013). Apps for birdwatchers such as The Sibley eGuide to the Birds of North America (http://www.sibleyguides.com/about/the-sibley-eguide-to-birds-app/) are packed with detailed information for identifying individual species of birds (photos, key traits for identification, flight patterns, habitat, range maps and song clips). A real-time, online checklist programme, eBird (http://www.ebird.org) has revolutionized the way the global birding community reports and accesses information about birds (Wood et al., Reference Wood, Sullivan, Iliff, Fink and Kelling2011). Launched in 2002 by the Cornell University Lab of Ornithology and National Audubon Society, eBird provides rich data sources for basic information on bird abundance and distribution at a variety of spatial and temporal scales. Apps designed to help farmers, extension agents and researchers identify crop pests and natural enemies in designated areas and to connect them with and provide input to a real-time checklist, modelled after eBird, should have tremendous value in resource-limited agricultural areas.
Sustaining the integrated pest management effort
Even the most scientifically, socially and economically viable IPM strategy sensibly designed to meet the needs of a given population of farmers will fail unless provisions are made to enable and sustain its adoption. Farmer networks can provide farmers access to essential information and also boost their confidence in alternative practices. Validation from peers can be a strong motivator for adopting an innovation (Rogers, Reference Rogers1983). Developing country respondents in the Parsa et al. (Reference Parsa, Morse, Bonifacio, Chancellor, Condori, Crespo-Pérez, Hobbs, Kroschel, Ba, Rebaudo, Sherwood, Vanek, Faye, Herrera and Dangles2014) survey emphasized this point, rating ‘IPM requires collective action within a farming community’ as the top obstacle facing IPM adoption.
A primary feature of the highly publicized multi-country FAO–IPM programme launched in tropical rice of Asia in 1980 was to link communities of farmers together in ‘farmer field schools’ (FFS) to learn how to use IPM on their farms. With technical assistance from the Food and Agriculture Organization of the United Nations (FAO), the programme eventually included 13 countries from South and Southeast Asia (Matteson et al., Reference Matteson, Gallagher, Kenmore, Denno and Perfect1994; Gallagher et al., Reference Gallagher, Ooi, Kenmore, Peshin and Dhawan2009). The FFS gave farmers first-hand experience with IPM practices and ecological concepts and organized farmer networks to implement rice IPM in rural communities of participating countries. The trained farmers reduced insecticide use by 50–80% while sustaining or increasing rice yield (Matteson, Reference Matteson2000). By reducing insecticides, the FAO–IPM programme averted insecticide-induced outbreaks of the brown planthopper that had plagued rice in much of the region. In Indonesia, more than 1.1 million farmers received IPM training in more than 48 000 field schools taught by over 30 000 facilitators and trainers (Braun et al., Reference Braun, Jiggins, Röling, van den Berg and Snijders2006). Training, combined with pesticide regulations, reduced insecticide use by 75% on rice of IPM-trained farmers in the province of Java (Gallagher et al., Reference Gallagher, Kenmore, Sogawa, Denno and Perfect1994). Encouraged by results of the FAO–IPM FFS in Asian rice, the FAO and national programmes expanded FFS training to additional crops and geographical areas. Some 4 million farmers in >70 countries had received training when Braun et al. (Reference Braun, Jiggins, Röling, van den Berg and Snijders2006) reviewed the FFS programmes. Braun et al. (Reference Braun, Jiggins, Röling, van den Berg and Snijders2006) concluded that the FFS had a ‘consistently positive picture of short- and medium-term impact, with farmers able to improve their agricultural productivity and to increase their leadership role in community-based activities’.
Opposing market forces
Gains from the FAO–IPM programme for rice diminished after donors ceased its funding in 1999 and national programmes were unable to sustain the effort. Insecticides resurfaced as the primary means for controlling rice insect pests and devastating planthopper outbreaks occurred in areas that had benefitted from the FAO–IPM programme (Heong and Hardy, Reference Heong and Hardy2009; Bottrell and Schoenly, Reference Bottrell and Schoenly2012; Thorburn, Reference Thorburn2014). Heong (Reference Heong, Wong and De Los Reyes2013) chronicled how pesticide marketing disrupted IPM in eight of those countries after the programme stopped. General merchandise retailers in rice-producing areas supplied 50–90% of the insecticides and also advised farmers on their use. Of the farmers surveyed, 20–80% relied on insecticide dealers for pest management information and insecticide recommendations. To entice farmers to buy the chemicals, some retailers offered incentives such as cash rewards, lottery tickets, bonus points and holiday trips. Some government workers earned income from chemical companies by promoting their products (Heong, Reference Heong, Wong and De Los Reyes2013). Hamburger (Reference Hamburger2002) reported that agricultural extension agents in China generated most of their salaries and office operating costs from pesticide sales. Decades earlier, Brader (Reference Brader1979) emphasized that the lack of an effective independent extension service de-linked from the pesticide industry was a major obstacle preventing wider adoption of integrated pest control in developing countries.
Pesticide marketing forces present particularly difficult challenges in countries lacking effective pesticide education programmes, laws and enforcement procedures (Ecobichon, Reference Ecobichon2001). Government and non-governmental organization (NGO) IPM projects have helped substantially in implementing IPM and dissuading farmers from using pesticides unnecessarily and unsafely. However, unless the special projects are allied with effective local extension services de-linked from pesticide marketing, pesticide suppliers may prevail as the farmers’ chief source of information on crop protection.
Reality of developing country extension services
According to Anderson and Feder (Reference Anderson, Feder, Evenson and Pingali2007), there were approximately 500 000 agricultural extension personnel worldwide in 2005, with >90% of them located in developing countries. Developing countries vary widely in the ratio of farmers per public extension agent (Table 3, data from Davis et al., Reference Davis, Swanson, Amudavi, Mekonnen, Flohrs, Riese, Lamb and Zerfu2010). It has been reported that India's extension services, on average, reach only about 7% of the country's farmers (GFRAS, 2012). An estimated 17% of the Indian farmers received agriculture-related information from other farmers and 13% from input (e.g. pesticide or fertilizer) dealers. Without proper training in IPM and a continuing source of relevant information, public service agents may not be qualified to provide effective technical assistance in IPM (Peshin et al., Reference Peshin, Jayaratne, Sharma and Abrol2014). Even if properly trained, the public agents may play a subservient role in areas where pesticide dealers have aggressive marketing (Heong, Reference Heong, Wong and De Los Reyes2013). Inadequate funding is a major factor hampering public extension efforts to boost IPM in resource-limited areas. Until national governments create policies and allocate funding sources to enact IPM, its long-term success is doubtful. Without these actions, as clearly illustrated when the FAO–IPM intercountry programme in tropical Asian rice terminated, farmers are likely to rely exclusively on pesticides and often apply them indiscriminately.
Table 3. Ratio of farmers per public extension agent in selected developing countries (Davis et al., Reference Davis, Swanson, Amudavi, Mekonnen, Flohrs, Riese, Lamb and Zerfu2010)

Women comprise ⩾40% of the agricultural labour force in developing countries (GFRAS, 2012). Yet only about 15% of the world's extension agents are women and only about 5% of women farmers benefit from extension services. This gender disparity reduces opportunities for women to adopt IPM and other innovations and to create sustainable livelihoods (GFRAS 2012).
Krell et al. (Reference Krell, Fisher and Steffey2016) proposed partnerships between public extension and private industry with shared responsibilities for providing information and technical assistance to farmers. Partnerships between public extension and a company selling pesticide products, for example, may pan out satisfactorily in some situations in developed countries. However, such partnerships would be highly questionable in developing countries that lack the means to guard against unscrupulous marketing practices by pesticide dealers. A major need in those countries is an effective independent extension service, de-linked completely from pesticide merchandizing, as Brader's (Reference Brader1979) review highlighted and the survey of Parsa et al. (Reference Parsa, Morse, Bonifacio, Chancellor, Condori, Crespo-Pérez, Hobbs, Kroschel, Ba, Rebaudo, Sherwood, Vanek, Faye, Herrera and Dangles2014) re-emphasized.
Innovations in training and outreach
Providing IPM information and technical assistance to large populations of resource-limited farmers requires a ‘new way’ of doing business. Although the FFS initiated by the FAO trained some 4 million farmers in over 70 countries (Braun et al., Reference Braun, Jiggins, Röling, van den Berg and Snijders2006), they included <2% of the estimated 280 million farms from low income and lower-middle income countries (data from Appendix A of Lowder et al., Reference Lowder, Skoet and Raney2016). Of the estimated 280 million resource-limited farms, 77% are in Asia, 17% in sub-Saharan Africa, 3% in the Middle East and North Africa and 1% in Latin America and the Caribbean (Lowder et al., Reference Lowder, Skoet and Raney2016).
Innovative dissemination networks that convey information electronically via mobile phones and other mobile devices have considerable potential to reach vast numbers of farmers. Although initial adopters were primarily affluent, urban and more educated consumers, in recent years, mobile phones have been adopted by rural and urban consumers in the world's poorest countries, due in part to the introduction of lower-priced handsets and airtime cards (Aker and Mbiti, Reference Aker and Mbiti2010). Mobile apps for agriculture and rural development are now used commonly in developing countries (Qiang et al., Reference Qiang, Kuek, Dymond and Esselaar2011) and some are related to IPM (Shen et al., Reference Shen, Cheng, Zhang, Gao, Abrol and Shankar2012). In some areas of India, farmers using mobile apps can access real-time data about weather, local markets, seeds, fertilizers, etc. and get guidance from agricultural experts. As Internet connectivity and mobile phone usage become more affordable, the reach is expected to expand to the remotest areas of India (Mittal and Mehar, Reference Mittal and Mehar2012). Zhang et al. (Reference Zhang, Wang and Duan2016) reported that 3G network coverage in rural areas of China in 2012 had reached 70%, the number of smartphones increased by > 30% per year in the rural market and rural Internet users increased to 146 million with 60% using smartphones.
Cheaper and wider access to broadband in developing countries should greatly expand the opportunities for designing mobile apps that convey information on IPM for specific crops in designated areas. Using eBird (http://www.ebird.org) as a reference, the apps could become important crowdsourcing tools (Dickinson et al., Reference Dickinson, Zuckerberg and Bonter2010) for alerting farmers, extension workers and research scientists about emerging problems and also for pinpointing needs for technical assistance in given areas. For example, the mobile device's built-in GPS could identify the location of an extension agent reporting the detection of a new invasive pest. The submitted report along with a photo of the invasive pest could be disseminated quickly to other extension workers, researchers and farmers in the affected area. Regardless of the innovations employed, networks must be designed to meet the specific needs of vast numbers of farmers in resource-limited areas. Training technical specialists in those areas is also needed. Offering IPM training, pest and natural enemy identification, etc. through open access platforms that offer online courses or workshops may have considerable potential when on-site, hands-on training is not possible.
However, the point that Deichmann et al. (Reference Deichmann, Goyal and Mishra2016) made about digital technology should be underscored: ‘…technology can always only address some, but not all of the barriers faced by farmers in poorer countries’. This caveat applies to web-based training, mobile apps, DNA barcoding and other technological innovations designed to help users in resource-limited agricultural areas. Conventional extension and education programmes have played and will continue to play an invaluable role in enabling farmers to take up innovations, improve production and protect the environment (GFRAS, 2012).
Discussion
Stern et al. (Reference Stern, Smith, van den Bosch and Hagen1959) had an unparalleled impact, stimulating innovations in multi-tactical crop protection and moulding the conceptual basis for modern IPM worldwide. The authors’ ecological foundation for integrated crop protection is as valid today as it was in 1959. Moreover, IPM offers potentially the best path to crop protection that increases and sustains agricultural productivity while minimizing threats to humans and the environment. Farmer associations, environmental organizations, national governments, international research and education enterprises and the agroindustry worldwide have recognized the advantages of IPM. Why, then, has the adoption rate been disappointing in developing countries and why, in those situations where IPM was adopted but abandoned, were the farmers unable or unwilling to sustain it?
Morse and Buhler (Reference Morse and Buhler1997) concluded perceptively that, while IPM can work under certain conditions in developing countries, the situation of most developing farmers does not match the necessary criteria that IPM specialists expect for its implementation. The authors emphasized that the focus should be to determine what is achievable under the farmers’ circumstances rather than what is technically perfect. The current review has focused on what Morse and Buhler (Reference Morse and Buhler1997) would probably call ‘technically perfect’ IPM. The technical features that make IPM function efficiently and help sustainable agriculture succeed in resource-limited farming areas have been highlighted. However, the current authors agree completely with Morse and Buhler (Reference Morse and Buhler1997) that IPM will not be adopted and sustained unless it is designed to conform to the farmers’ circumstances.
Determining compatibility and farmer acceptance of integrated pest management tactics
Referring to a multi-tactical crop protection programme as ‘IPM’ without first verifying compatibility of the tactics and their combined effects on crop yields and farmer profits compared to their singular effects is ‘false labelling’. Combining tactics to achieve the best long-term results requires considerable ecological finesse (Thomas, Reference Thomas1999). Researchers must devise inventive experimental designs to disentangle the complicated mix of possible outcomes before the technical compatibility of the different tactics is known. Much more difficult is determining if farmers will accept and sustain the IPM strategy. The most technically sound strategy will not endure if it fails to adjust to local cultures, politics and opposing market forces. Quoting Levins (Reference Levins, Kogan and Jepson2007), ‘The ultimate goal of IPM is not the pest, or even the crop, but the viability of rural life as a whole and a safe, sustainable food supply in the face of many kinds and increasing intensity of uncertainty’. Researchers can determine, albeit with difficulty, if the IPM tactics are technically compatible. However, whether the IPM system survives is at the mercy of the farmers and local cultures. Efforts to determine the compatibility and efficiency of IPM tactics are merely academic exercises unless farmers and local communities are the central focus.
Integrated pest management requires a landscape perspective
To achieve its greatest potential in resource-limited agriculture, IPM should evolve from a total landscape view, considering the effects of farm size, neighbouring crops, wild vegetation and aquatic systems. Designing IPM programmes around a landscape perspective provides both opportunities and challenges for innovations such as the ‘push–pull’ strategy in Africa for cereal crops (Khan et al., Reference Khan, Midega, Hooper and Pickett2016) and the planting of nectar-rich companion plants in monocultures of rice in Asia (Gurr et al., Reference Gurr, Lu, Zheng, Xu, Zhu, Chen, Yao, Cheng, Zhu, Catindig, Villareal, Chien, Cuong, Channoo, Chengwattana, Lan, Hai, Chaiwong, Nicol, Perovic, Wratten and Heong2016). Once farmers understand the interchange of pests and natural enemies among the different vegetation units, they may be willing to modify the vegetation mix (different plant species or different genetic forms of one crop species) in ways that IPM specialists have found to reduce pests and enhance natural enemies. However, to re-emphasize Morse and Buhler's (Reference Morse and Buhler1997) argument, the focus should be to determine what is achievable under the farmers’ circumstances rather than what is technically perfect.
Donor funding for IPM projects in developing countries has usually focused on a single crop such as rice, cotton, or maize. Given existing knowledge about pest and natural enemy interchange among different crops, projects that approach IPM from a total crop landscape perspective with all crops included would be a better investment in many cases. Furthermore, donor funding has given limited attention to the potential of mixing multiline cultivars and different cultivars of the same crop species as an IPM strategy. The approach has considerable promise for managing certain diseases of small grains (Browning et al., Reference Browning, Simons, Torres, Horsfall and Cowling1977; Mundt, Reference Mundt2002). However, its potential for managing insect and other pests of small grains or other crops is generally unknown.
Common ground for integrated pest management, sustainable agriculture and conservation biology
Increasing and sustaining agricultural productivity for the growing global population rests heavily on agrobiodiversity at the genetic, population, community, ecosystem and landscape levels. Integrated pest management specialists and sustainable agriculture specialists share an interest in understanding, enhancing and preserving agrobiodiversity. Together and separately, the two groups have made valuable contributions measuring the effects of agricultural practices (e.g. fertilizers, pesticides, cultivar selection, crop rotation, crop plant density, irrigation, cultivation, harvest practices) on pests, natural enemies, pollinators, N-producing microbes and other organisms. Some progress has been made unravelling food webs in selected cropping systems to provide useful insights about interactions of the crop plants, pests and natural enemies and to pinpoint functional traits that different species perform in the agroecosystem (Neuenschwander et al., Reference Neuenschwander, Hennessey and Herren1987; Cohen et al., Reference Cohen, Schoenly, Heong, Justo, Arida, Barrion and Litsinger1994). An important next step is to integrate food web structure with community dynamics, using interaction strength as the conduit (Berlow et al., Reference Berlow, Neutel, Cohen, De Ruiter, Ebenman, Emmerson, Fox, Jansen, Jones, Kokkoris, Logofet, McKane, Montoya and Petchey2004) and molecular methods as fine tools (Boyer et al., Reference Boyer, Snider and Wratten2016), to clarify how crop food webs change over time and space, particularly in response to introduced species, farming practices, genetic technology and climate change.
The functional traits of species may influence ecosystems directly (e.g. pollination, predation, parasitism, herbivory, competition) or indirectly through responses to local environmental changes such as microclimate or human disturbances (Chapin et al., Reference Chapin, Zavaleta, Eviner, Naylor, Vitousek, Reynolds, Hooper, Lavorel, Sala, Hobbie, Mack and Díaz2000). Understanding linkages between agrobiodiversity and ecosystem services are crucial for predicting how changes in environment and management practices will impact the multiple ecosystem services essential for increasing and sustaining crop yields (Zhang et al., Reference Zhang, Ricketts, Kreman, Carney and Swinton2007; Power, Reference Power2010; Kremen and Miles, Reference Kremen and Miles2012; Wood et al., Reference Wood, Karp, DeClerck, Kremen, Naeem and Palm2015). Wood et al. (Reference Wood, Karp, DeClerck, Kremen, Naeem and Palm2015) outlined a seven-step trait-based approach leading to the development of farm management strategies that provide multiple ecosystem services. The proposed work would culminate in quantitative models that generate target distributions of species based on their functional traits and identify management strategies that achieve specific ecosystem services.
Identifying and augmenting the species responsible for ecosystem services in resource-limited agriculture will require the collective expertise of IPM and sustainable agriculture specialists. Conservation biologists can also play an important role through collaborative research aimed at conserving key species that provide essential ecosystem services (Paul and Robertson, Reference Paul and Robertson1989; Gall and Orians, Reference Gall and Orians1992; Miller and Rossman, Reference Miller and Rossman1995; O'Malley, Reference O'Malley, Batzer, Rader and Wissinger1999). The work will also require a boosted effort in taxonomic services (conventional and DNA-based methods) to identify species responsible for ecosystem functions. In addition to research benefits of the union, the cooperation of IPM and sustainable agriculture specialists and conservation biologists would bolster the taxonomic capability required for agrobiodiversity surveys.
Can integrated pest management turn the pesticide tide?
Globally, pesticide use has increased over the last several decades; however, crop losses have not significantly decreased over the same period (Oerke, Reference Oerke2006). The United Nations (2017) reported that 65% of global pesticide sales are controlled by only three companies, made possible through recent mergers involving Monsanto and Bayer, Dow and Dupont and Syngenta and ChemChina. Recent trends suggest that pesticides are increasing in many developing countries. Pesticide imports into Southeast Asian countries, for example, increased nearly sevenfold in value between 1990 and 2010 (FAO data, cited in Normile, Reference Normile2013). Often, developing countries import formulated pesticides or their active ingredients that have been banned, severely restricted or never patented in nations of origin because of environmental or health concerns (Ecobichon, Reference Ecobichon2001). Developing countries may have pesticide laws but resources to enforce them and to train farmers on the safe and efficient use of pesticides are usually inadequate.
Stern et al. (Reference Stern, Smith, van den Bosch and Hagen1959) envisioned IPM, not as a way to eliminate insecticides but to reconcile insecticide applications with biological control. However, they and other early IPM pioneers emphasized that pesticide applications were corrective measures to be used as a last line of defence after the non-chemical primary management components failed to keep the pests at non-damaging levels. That view is diametrically opposed by today's policymakers of the three major scientific professional societies involved with pest management in the USA – the Weed Science Society of America (WSSA), American Phytopathological Society (APS) and Entomological Society of America (ESA). The WSSA–APS–ESA policy statement rejects the notion that pesticides should be used ‘as a last resort’ in IPM (http://www.entsoc.org/press-releases/issues-associated-least-toxic-pesticides-applied-last-resort). Coll and Wajnberg (Reference Coll, Wajnberg, Coll and Wajnberg2017b) argued that this statement ‘ignores the extensive research, regulatory, educational and stewardship efforts that make important pesticide tools available and define their proper and safe use in Integrated Pest Management programmes’.
Parallel with increased use of pesticides in developing countries is the proliferation of genetically engineered (GE) crops with ‘pesticide’ genes. Of the 26 countries planting GE crops in 2016, 19 were developing countries and seven were industrial countries (ISAAA, 2016). Developing countries grew 99.6 million hectares of GE crops, compared with 85.5 million hectares grown by industrial countries (ISAAA, 2016). Millions of hectares are now planted to GE Bt crops (Tabashnik, Reference Tabashnik1994).
Some view GE crops as indispensable for future food security (Leyser, Reference Leyser2014). Others are less optimistic about their potential for increasing crop yield (Sinclair et al., Reference Sinclair, Purcell and Sneller2004). Ecologists have been concerned that the GE crop plants may cause ecological disruptions by killing non-target pests (Romeis et al., Reference Romeis, Bartsch, Bigler, Candolfi, Gielkens, Hartley, Hellmich, Huesing, Jepson, Layton, Quemada, Raybould, Rose, Schiemann, Sears, Shelton, Sweet, Vaituzis and Wolt2008); hybridizing with wild relatives and thereby modifying the relatives’ natural gene pool (Pilson and Prendeville, Reference Pilson and Prendeville2004); producing weedy, exotic-like hybrids (Pilson and Prendeville, Reference Pilson and Prendeville2004); or by accelerating the rate at which pests evolve strains that defy the resistance traits (Tabashnik, Reference Tabashnik1994; Gould, Reference Gould1998; Pilson and Prendeville, Reference Pilson and Prendeville2004). These and other concerns have received substantial research in developed countries but much less in developing countries. Researchers in developing countries have considerable work ahead before the yield potential of the GE crops is known and their ecological effects (in agroecosystems and in communities of nearby wild habitats) are realized. Ecologists and IPM specialists working with sustainable agricultural specialists and local farmers can play an important role by determining if, when and how to integrate Bt-crops and other engineered crops with pest control genes into IPM systems in resource-limited areas.
Reaching the vast population of resource-limited farmers
According to Lowder et al. (Reference Lowder, Skoet and Raney2016), half of the world's estimated 570 million farms are in developing countries (i.e. low income plus lower-middle income countries). About three-quarters of the farms in low- and lower-middle income countries are smaller than 2 ha, occupy about 30–40% of the land and have decreased in size from 1960 to 2000 (Lowder et al., Reference Lowder, Skoet and Raney2016). Farmer field schools, media campaigns and other training and outreach efforts have proven effective in familiarizing resource-limited farmers with IPM and encouraging them to adopt it. However, such efforts are expensive and can be expected to reach only a tiny portion of the hundreds of millions of resource-limited farmers. In Senegal, Witt et al. (Reference Witt, Pemsl and Waibel2008) showed that small proportions of IPM-trained farmers in dispersed villages are insufficient to spread changes outward to other farmers. A critical mass of trained farmers spread among nearby villages is required before information is disseminated effectively and adopted by non-participating farmers (Witt et al., Reference Witt, Pemsl and Waibel2008).
Special projects like the FAO–IPM programme for rice in South and Southeast Asia make a big swath during the funding period. However, once farmers are disconnected from reliable and continuing sources of IPM information, chemical companies may be quick to fill the gaps, as illustrated when funding for the FAO–IPM programme ceased. What needs to be done to ensure that farmers have the support needed to adopt and sustain a practical and profitable system of IPM? This is the foremost question facing advancement of IPM in developing countries. Meeting the challenge is a huge undertaking. Coll and Wajnberg (Reference Coll, Wajnberg, Coll and Wajnberg2017c) argued persuasively, and used case history examples to support their argument, that government commitment is critical for the sustainable deployment of environmental pest management. Obviously, international donors, research centres, NGOs and farmer associations are also critical actors. Launching a long-term sustainable approach requires the collective input of IPM extension, education and research specialists and specialists in information technology, media development, crowdsourcing, rural sociology, government policy and regulations, etc. Broadband applications using mobile devices will become increasingly important in disseminating IPM information and connecting communities of farmers, extension workers and researchers. Relative to the revolution in biotechnology, Fischer et al. (Reference Fischer, Byerlee and Edmeades2009) argued that the potential of information and communication technologies to boost productivity and contribute to more sustainable production systems has received too little attention. As emphasized in the current review, the educational and outreach programmes must be tailored to meet the farmers’ circumstances in a given location. The farmers currently face many kinds of uncertainties that affect their perceptions about pests and ways to deal with them. Climate change, the appearance of invasive species, the emergence of pesticide resistance and introductions of new technology such as GE crops intensify the uncertainties and increase pressure to produce higher yields. Therefore, the best support system, regardless of the form it takes, is one that gives farmers confidence that IPM will enhance yields and profits and reduce risks to themselves and the environment in light of the many uncertainties and challenges.
Conclusions
(1) Stern et al. (Reference Stern, Smith, van den Bosch and Hagen1959) influenced the direction of multi-tactical crop protection worldwide and moulded the conceptual basis for modern IPM, which combines various chemical, biological and physical control tactics in a cohesive ecologically based pest management scheme. By helping to ensure equitable, secure, sufficient and stable crop protection, IPM is a complementary and necessary feature of sustainable agriculture.
(2) National programmes, farmer associations, international organizations and donors have promoted IPM in resource-limited areas since the 1970s. IPM has reduced pesticide use and provided more durable crop protection on a range of food and cash crops. However, its adoption rate by developing country farmers has been low and its advances short lived.
(3) The conceptual basis of IPM and the criteria for determining if its control tactics have been integrated harmoniously have been reviewed and it has been shown how local and regional landscape patterns affect pests and influence the design of IPM. It is argued that the agroecosystem must be understood and managed as a living system from a landscape perspective with the goal of enhancing and conserving agrobiodiversity and keeping ecosystem services intact. The obstacles hindering IPM's adoption and sustainment on resource-limited farms in developing countries were discussed and pathways for overcoming them presented.
(4) Despite its low adoption rate in developing countries, IPM potentially offers the best route to economically efficient crop protection that increases and maintains farm productivity while minimizing threats to humans and the environment. Increasing and sustaining wider adoption will require significant investment in research, extension and policy innovations. Closer cooperation between IPM, sustainable agriculture and other specialists would synergize efforts to identify the functional traits of agrobiodiversity that provide essential ecosystem services for increased agricultural productivity and to map out farm management strategies for effectively augmenting these services.
(5) The overarching challenge is devising communication and support systems that will allow resource-limited farmers to try, adopt and sustain IPM that enhances yields and profits and reduces risks in light of the many uncertainties and challenges they face. Public extension services are not currently equipped to assist the vast population of resource-limited farmers. The farmers often rely on pesticide dealers for information on pest management decisions and may be subject to unscrupulous pesticide marketing practices.
(6) Providing reliable information and supporting hundreds of millions of resource-limited farmers who would potentially benefit from IPM requires the collective input of IPM extension and education specialists and also specialists in information technology, media development, crowdsourcing, rural sociology, government policy, etc. The current authors advocate the use of information technology (e.g. broadband apps for smartphones), media development, crowdsourcing and rural sociology to connect farmers to the technical sources. Regardless of the communication medium, the educational and outreach programmes need to be tailored to meet the farmers’ circumstances in a given location.
Acknowledgements
DGB is grateful to the University of Maryland libraries for assistance in providing published literature. He is also grateful to the many graduate students in his University's Master of Chemical and Life Sciences online course in biodiversity and conservation biology, which he taught yearly from 2000 to 2015, for sharing valuable insights into species interactions in managed tropical systems. KGS is grateful to Joel E. Cohen for introducing him to the IRRI food web in 1989, Deborah Childers and Julie Reuben, librarians of the California State University, Stanislaus, for assistance in securing published literature used in this review and CSU-Stanislaus for providing sabbatical support in the Spring 2015.
Financial support
DGB received no funding for the work. KGS received initial support for this work from CSU-Stanislaus in the form of a university sabbatical in Spring 2015.
Conflicts of interest
None.
Ethical standards
Not applicable.