Impact statement
Human society deeply values coasts and the ecosystem services they provide. But navigating the challenge of coastal management over the coming decades to sustain these connections depends on an ability to identify and quantify the ecological consequences of climate change. Aiding in this task has been a sequence of Assessment Reports by the Intergovernmental Panel on Climate Change (IPCC), the most recent of which is its Sixth Assessment Report. Both this IPCC Report and associated studies emphasise that human-caused climate change has driven our oceans into states unprecedented over millennia, and that these changes have led to fundamental ecological impacts across all coastal ecosystems. These impacts exacerbate and are exacerbated by other human-caused impacts in the coastal zone. Projections of future risk mirror the impacts already observed, but they escalate with cumulative greenhouse-gas emissions. Although these conclusions are supported by multiple lines of evidence, progress beyond qualitative assessments is hampered by our inability to confidently disentangle the effects of interacting drivers of change. Difficulties in this regard escalate as the number of drivers considered increases. Promising avenues for progress include emerging collaborative initiatives, such as model intercomparison projects, and the more inclusive use of multiple knowledge systems. In the interim, however, reducing risks over the remainder of this century depends on rapidly reducing emissions, restoring damaged habitats, designing and deploying climate-smart conservation actions that alleviate non-climate stressors, and carefully managing existing and future coastal development, with an emphasis on nature-based solutions.
Introduction
The world’s coasts hold special places in human history and culture: settlements and cities have sprung up close to the sea because of the rich resources the ocean and coastal ecosystems provide, the transport and trade they facilitate, and the sense of place they instil (Neumann et al., Reference Neumann, Vafeidis, Zimmermann and Nicholls2015; Cooley et al., Reference Cooley, Schoeman, Bopp, Boyd, Donner, Ghebrehiwet, Ito, Kiessling, Martinetto, Ojea, Racault, Rost, Skern-Mauritzen, Pörtner, Roberts, Tignor, Poloczanska, Mintenbeck, Alegría, Craig, Langsdorf, Löschke, Möller, Okem and Rama2022). As a direct result, these coastal ecosystems face multiple escalating threats from humanity, most of which exacerbate or are exacerbated by climate change. Navigating the challenge of coastal management over the coming decades therefore relies heavily on being able to identify and quantify the ecological consequences of climate change. Aiding in this task has been a sequence of Assessment Reports by the Intergovernmental Panel on Climate Change (IPCC), the most recent of which is its Sixth Assessment Report (IPCC, Reference Masson-Delmotte, Zhai, Pirani, Connors, Péan, Berger, Caud, Chen, Goldfarb, Gomis, Huang, Leitzell, Lonnoy, Matthews, Maycock, Waterfield, Yelekçl, Yu and Zhou2021, Reference Pörtner, Roberts, Tignor, Poloczanska, Mintenbeck, Alegría, Craig, Langsdorf, Löschke, Möller, Okem and Rama2022). Here, we explore the main findings of that work, providing illustrative examples of climate-driven impacts and risks, identifying key challenges to progress, and briefly discussing promising avenues that might lead to the development of more robust, quantitative projections of future risk to coastal ecosystems due to climate change.
Human-induced climate change has vastly altered the environmental conditions within which coastal ecosystems operate
Anthropogenic climate change has driven the physical and chemical conditions of coastal ecosystems (Table 1) to states that are unprecedented over millennia (Cooley et al., Reference Cooley, Schoeman, Bopp, Boyd, Donner, Ghebrehiwet, Ito, Kiessling, Martinetto, Ojea, Racault, Rost, Skern-Mauritzen, Pörtner, Roberts, Tignor, Poloczanska, Mintenbeck, Alegría, Craig, Langsdorf, Löschke, Möller, Okem and Rama2022). Approximately 93% of the excess incoming solar energy trapped by greenhouse gases is absorbed by the ocean. This has added 350 ZJ (1 ZJ = 1021 J) to the heat content of the oceans between 1958 and 2019, with an annual acceleration over the past decade. (Cheng et al., Reference Cheng, von Schuckmann, Abraham, Trenberth, Mann, Zanna, England, Zika, Fasullo, Yu, Pan, Zhu, Newsom, Bronselaer and Lin2022). This has driven the heat content of the upper ocean to reach record levels in 2022, exceeding the previous record (2021) by approximately 10 ZJ (Cheng et al., Reference Cheng, Abraham, Trenberth, Fasullo, Boyer, Mann, Zhu, Wang, Locarnini, Li, Zhang, Yu, Wan, Chen, Feng, Song, Liu, Reseghetti, Simoncelli, Gouretski, Chen, Mishonov, Reagan and Li2023). Associated warming has been almost ubiquitous along coastlines (Lima and Wethey, Reference Lima and Wethey2012) and within estuaries (Scanes et al., Reference Scanes, Scanes and Ross2020). The exception has been upwelling cells, where the increased prevalence of upwelling-favourable winds has resulted in local cooling, especially at higher latitudes (Bograd et al., Reference Bograd, Jacox, Hazen, Lovecchio, Montes, Pozo Buil, Shannon, Sydeman and Rykaczewski2023). Superimposed on this long-term trend of warming has been a rapid surge in the localised occurrence of anomalously warm waters that persists for days to many months, known as marine heatwaves (MHWs) (Hobday et al., Reference Hobday, Alexander, Perkins, Smale, Straub, Oliver, Benthuysen, Burrows, Donat, Feng, Holbrook, Moore, Scannell, Gupta and Wernberg2016; Laufkötter et al., Reference Laufkötter, Zscheischler and Frölicher2020; Sen Gupta et al., Reference Sen Gupta, Thomsen, Benthuysen, Hobday, Oliver, Alexander, Burrows, Donat, Feng, Holbrook, Perkins‐Kirkpatrick, Moore, Rodrigues, Scannell, Taschetto, Ummenhofer, Wernberg and Smale2020). The frequency of MHWs has at least doubled since the 1980s, and MHW intensity has increased rapidly with ocean warming, as has the proportion of time the global ocean is subject to MHW conditions (IPCC, Reference Masson-Delmotte, Zhai, Pirani, Connors, Péan, Berger, Caud, Chen, Goldfarb, Gomis, Huang, Leitzell, Lonnoy, Matthews, Maycock, Waterfield, Yelekçl, Yu and Zhou2021).
Table 1. Estimates of magnitudes of observed and projected changes in climate-induced drivers pertinent to coastal ecosystems, as assessed by the IPCC. These estimates are global averages and it should be noted that in each case, considerable spatial variability is anticipated, especially in coastal areas. Unless otherwise stated, ranges in brackets represent 90% confidence intervals.
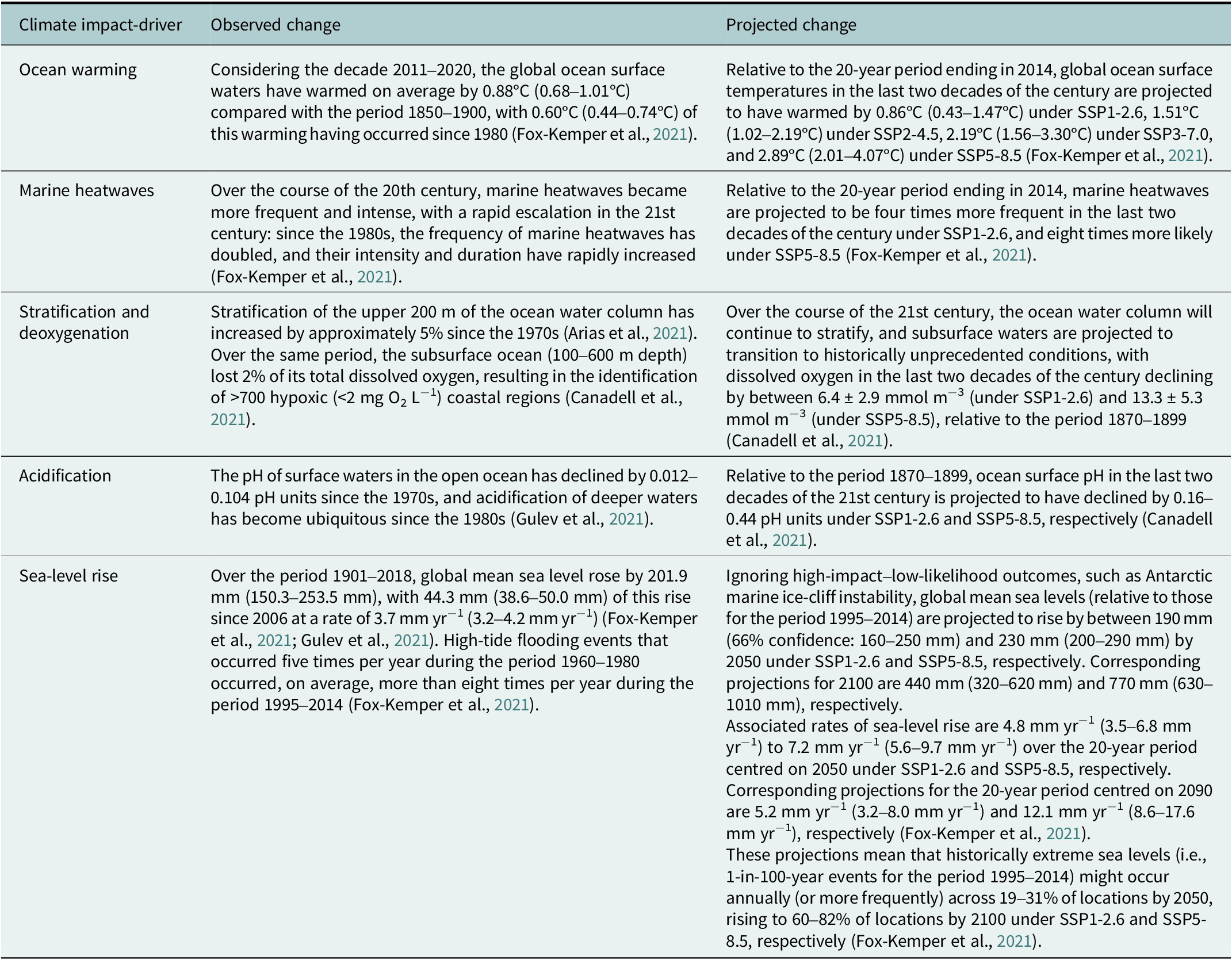
As the oceans have warmed, the solubility of gases has decreased, resulting in a strong trend in declining dissolved oxygen content – a process known as ocean deoxygenation (Canadell et al., Reference Canadell, Monteiro, Costa, Cotrim da Cunha, Cox, Eliseev, Henson, Ishii, Jaccard, Koven, Lohila, Patra, Piao, Rogelj, Syampungani, Zaehle, Zickfeld, Masson-Delmotte, Zhai, Pirani, Connors, Péan, Berger, Caud, Chen, Goldfarb, Gomis, Huang, Leitzell, Lonnoy, JBR, Maycock, Waterfield, Yelekçl, Yu and Zhou2021). Ocean stratification is enhanced by warming, reducing mixing (ventilation), altering nutrient redistribution, and exacerbating deoxygenation, especially in subsurface waters. Oxygen minimum zones (OMZs) – areas of low oxygen concentration in the upper ocean that are especially apparent in tropical regions – have been expanding at least since 1960 (Zhou et al., Reference Zhou, Gong and Zhou2022). An exception to warming-driven deoxygenation is found where intensifying upwelling brings cold, low-oxygen water to the surface (Canadell et al., Reference Canadell, Monteiro, Costa, Cotrim da Cunha, Cox, Eliseev, Henson, Ishii, Jaccard, Koven, Lohila, Patra, Piao, Rogelj, Syampungani, Zaehle, Zickfeld, Masson-Delmotte, Zhai, Pirani, Connors, Péan, Berger, Caud, Chen, Goldfarb, Gomis, Huang, Leitzell, Lonnoy, JBR, Maycock, Waterfield, Yelekçl, Yu and Zhou2021; Bograd et al., Reference Bograd, Jacox, Hazen, Lovecchio, Montes, Pozo Buil, Shannon, Sydeman and Rykaczewski2023). A further direct impact of warming is melting ice, both at sea and grounded on land. The latter – together with the thermal expansion of seawater – has contributed to accelerating rates of sea-level rise, now averaging >3 mm per year, faster than any time in at least the last 3,000 years (Fox-Kemper et al., Reference Fox-Kemper, Hewitt, Xiao, Aðalgeirsdóttir, Drijfhout, Edwards, Golledge, Hemer, Kopp, Krinner, Mix, Notz, Nowicki, Nurhati, Ruiz, Sallée, ABA, Yu, Masson-Delmotte, Zhai, Pirani, Connors, Péan, Berger, Caud, Chen, Goldfarb, Gomis, Huang, Leitzell, Lonnoy, JBR, Maycock, Waterfield, Yelekçl, Yu and Zhou2021; Le Cozannet et al., Reference Le Cozannet, Lawrence, Schoeman, Adelekan, Cooley, Glavovic, Haasnoot, Harris, Kiessling, Kopp, Mukherji, Nunn, Piepenburg, Schmidt, Simmons, Singh, Slangen, Supratid, Pörtner, Roberts, Tignor, Poloczanska, Mintenbeck, Alegría, Craig, Langsdorf, Löschke, Möller, Okem and Rama2022).
Increasing atmospheric CO2 concentrations have resulted in decreasing pH of ocean waters – known as ocean acidification – so that surface-water pH is now unusually low in the context of the past 2 million years (Arias et al., Reference Arias, Bellouin, Coppola, Jones, Krinner, Marotzke, Naik, Palmer, Plattner, Rogelj, Rojas, Sillmann, Storelvmo, Thorne, Trewin, Achuta Rao, Adhikary, Allan, Armour, Bala, Barimalala, Berger, Canadell, Cassou, Cherchi, Collins, Collins, Connors, Corti, Cruz, Dentener, Dereczynski, Di Luca, Diongue Niang, Doblas-Reyes, Dosio, Douville, Engelbrecht, Eyring, Fischer, Forster, Fox-Kemper, Fuglestvedt, Fyfe, Gillett, Goldfarb, Gorodetskaya, Gutierrez, Hamdi, Hawkins, Hewitt, Hope, Islam, Jones, Kaufman, Kopp, Kosaka, Kossin, Krakovska, Lee, Li, Mauritsen, Maycock, Meinshausen, Min, Monteiro, Ngo-Duc TOtto, Pinto, Pirani, Raghavan, Ranasinghe, Ruane, Ruiz, Sallée, Samset, Sathyendranath, Seneviratne, Sörensson, Szopa, Takayabu, Tréguier, van den Hurk, Vautard, von Schuckmann, Zaehle, Zhang, Zickfeld, Masson-Delmotte, Zhai, Pirani, Connors, Péan, Berger, Caud, Chen, Goldfarb, Gomis, Huang, Leitzell, Lonnoy, JBR, Maycock, Waterfield, Yelekçl, Yu and Zhou2021). Finally, changes in precipitation, stratification and ice-melt have enhanced contrasts in salinity between relatively salty and relatively fresh parts of the ocean (Cheng et al., Reference Cheng, Trenberth, Gruber, Abraham, Fasullo, Li, Mann, Zhao and Zhu2020).
Ocean conditions are projected to continue diverging from their pre-industrial state, with the magnitude of change depending on cumulative emissions
Most of the observed changes in ocean conditions due to anthropogenic climate change (Table 1) are irreversible on centennial to millennial scales, given present mitigation tools (IPCC, Reference Masson-Delmotte, Zhai, Pirani, Connors, Péan, Berger, Caud, Chen, Goldfarb, Gomis, Huang, Leitzell, Lonnoy, Matthews, Maycock, Waterfield, Yelekçl, Yu and Zhou2021). Warming of the upper ocean by 2100 is projected to range 2–8 times that experienced over the period 1971–2015, resulting in more frequent and intense MHWs, greater deoxygenation, increased stratification, faster ice-melt and accelerating sea-level rise. Ocean acidification is expected to intensify and salinity contrasts to be enhanced (Arias et al., Reference Arias, Bellouin, Coppola, Jones, Krinner, Marotzke, Naik, Palmer, Plattner, Rogelj, Rojas, Sillmann, Storelvmo, Thorne, Trewin, Achuta Rao, Adhikary, Allan, Armour, Bala, Barimalala, Berger, Canadell, Cassou, Cherchi, Collins, Collins, Connors, Corti, Cruz, Dentener, Dereczynski, Di Luca, Diongue Niang, Doblas-Reyes, Dosio, Douville, Engelbrecht, Eyring, Fischer, Forster, Fox-Kemper, Fuglestvedt, Fyfe, Gillett, Goldfarb, Gorodetskaya, Gutierrez, Hamdi, Hawkins, Hewitt, Hope, Islam, Jones, Kaufman, Kopp, Kosaka, Kossin, Krakovska, Lee, Li, Mauritsen, Maycock, Meinshausen, Min, Monteiro, Ngo-Duc TOtto, Pinto, Pirani, Raghavan, Ranasinghe, Ruane, Ruiz, Sallée, Samset, Sathyendranath, Seneviratne, Sörensson, Szopa, Takayabu, Tréguier, van den Hurk, Vautard, von Schuckmann, Zaehle, Zhang, Zickfeld, Masson-Delmotte, Zhai, Pirani, Connors, Péan, Berger, Caud, Chen, Goldfarb, Gomis, Huang, Leitzell, Lonnoy, JBR, Maycock, Waterfield, Yelekçl, Yu and Zhou2021).
Confirming that climate change has caused ecological responses
While change in Earth’s climate system has been unambiguously attributed to anthropogenic greenhouse-gas emissions (IPCC, Reference Masson-Delmotte, Zhai, Pirani, Connors, Péan, Berger, Caud, Chen, Goldfarb, Gomis, Huang, Leitzell, Lonnoy, Matthews, Maycock, Waterfield, Yelekçl, Yu and Zhou2021), distinguishing the role of anthropogenic climate change in altering ecological systems from the roles of other potential drivers has proven more problematic and contentious (Brander et al., Reference Brander, Bruno, Hobday and Schoeman2011; Pielke, Reference Pielke2011; Stocker et al., Reference Stocker, Dahe, Plattner, Midgley and Tignor2011). Such attribution has been especially difficult in coastal systems, where human impact is ubiquitous (Williams et al., Reference Williams, Watson, Beyer, Klein, Montgomery, Runting, Roberson, Halpern, Grantham, Kuempel, Frazier, Venter and Wenger2022; Allan et al., Reference Allan, Levin and Kark2023) and drivers of change compete with each other amidst naturally variable conditions, confounding unambiguous interpretations (Cooley et al., Reference Cooley, Schoeman, Bopp, Boyd, Donner, Ghebrehiwet, Ito, Kiessling, Martinetto, Ojea, Racault, Rost, Skern-Mauritzen, Pörtner, Roberts, Tignor, Poloczanska, Mintenbeck, Alegría, Craig, Langsdorf, Löschke, Möller, Okem and Rama2022; Friess et al., Reference Friess, Adame, Adams and Lovelock2022). In such systems, attribution instead usually comprises a sequence of steps (Figure 1), often involving multiple lines of evidence (Parmesan et al., Reference Parmesan, Burrows, Duarte, Poloczanska, Richardson, Schoeman and Singer2013; Hansen et al., Reference Hansen, Stone, Auffhammer, Huggel and Cramer2016; Phillips, Reference Phillips2023).
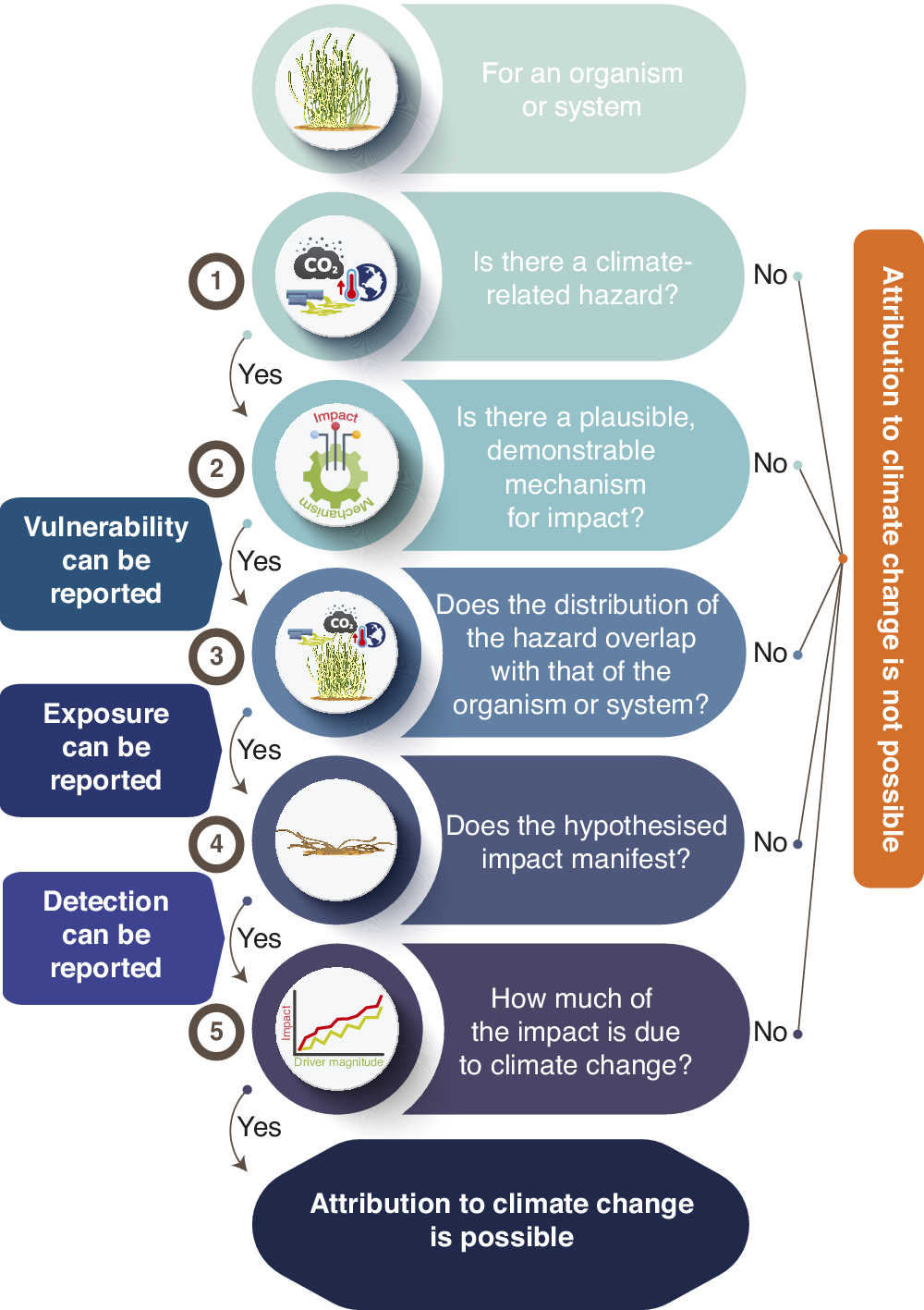
Figure 1. Steps involved in detecting and attributing an impact of climate change on an organism or ecological system. Note that the final step of attribution is seldom straightforward, instead often involving inference on the basis of multiple lines of evidence.
The first step involves identifying a hazard. This could be a change in a climate variable that is known to be affected by anthropogenic greenhouse-gas emissions (i.e., a climate-induced driver), but it could equally be a phenomenon caused by a change in climate, including a management action taken to mitigate an existing climate-change impact (e.g., the construction of a seawall, Simpson et al., Reference Simpson, Mach, Constable, Hess, Hogarth, Howden, Lawrence, Lempert, Muccione, Mackey, New, O’Neill, Otto, Pörtner, Reisinger, Roberts, Schmidt, Seneviratne, Strongin, van Aalst, Totin and Trisos2021). The second requirement is to demonstrate that the organism or system is vulnerable to that hazard – that is, that there is a plausible and demonstrable mechanism for the putative effect. Third, the organism or system must experience – be exposed to – the hazard. Fourth, an exposed organism or system must demonstrate the anticipated change – a process known as “detection” of a climate impact. Ideally, detection is accompanied by an analysis that separates the putative climate response from responses to other non-climate drivers experienced by the organism or system (Hansen et al., Reference Hansen, Stone, Auffhammer, Huggel and Cramer2016) – this constitutes formal “attribution” of a climate impact (Figure 1). Gonzalez et al. (Reference Gonzalez, Chase and O’Connor2023) provide a more detailed discussion of detection and attribution and propose a quantitative framework for these processes as they apply to changes in biodiversity and other impacts to ecosystems (Parmesan et al., Reference Parmesan, Burrows, Duarte, Poloczanska, Richardson, Schoeman and Singer2013; Ara Begum et al., Reference Ara Begum, Lempert, Ali, Benjaminsen, Bernauer, Cramer, Cui, Mach, Nagy, Stenseth, Sukumar, Wester, Pörtner, Roberts, Tignor, Poloczanska, Mintenbeck, Alegría, Craig, Langsdorf, Löschke, Möller, Okem and Rama2022).
Climate-induced drivers have greatly impacted life in the ocean and along its coasts
Ocean warming
Temperature is a central driver of metabolic processes and therefore a key driver of ecological responses (Pörtner, Reference Pörtner2021). Because the specific heat of seawater is around four times that of air, the oceans have warmed only half as much as the atmosphere as a result of anthropogenic climate change, despite absorbing more than nine times the thermal energy (Fox-Kemper et al., Reference Fox-Kemper, Hewitt, Xiao, Aðalgeirsdóttir, Drijfhout, Edwards, Golledge, Hemer, Kopp, Krinner, Mix, Notz, Nowicki, Nurhati, Ruiz, Sallée, ABA, Yu, Masson-Delmotte, Zhai, Pirani, Connors, Péan, Berger, Caud, Chen, Goldfarb, Gomis, Huang, Leitzell, Lonnoy, JBR, Maycock, Waterfield, Yelekçl, Yu and Zhou2021). But this property of seawater also means that ocean temperatures are generally less variable in space and time than those on land, resulting in marine organisms having narrower thermal tolerance ranges (Pinsky et al., Reference Pinsky, Eikeset, McCauley, Payne and Sunday2019; Pörtner, Reference Pörtner2021), and a greater predisposition towards occupying the full extent of these ranges than that of their terrestrial counterparts (Sunday et al., Reference Sunday, Bates and Dulvy2012). This renders marine biodiversity more vulnerable to warming than its terrestrial counterparts, causing a wide range of impacts. Among the more common consequences of the long-term trend in warming for coastal biodiversity are range shifts and tropicalisation, and changes in phenology.
Range shifts, tropicalisation and depth shifts
Warming of the ocean surface since the 1950s has shifted marine taxa and communities poleward at an average (± 90% confidence interval) of 59.2 ± 15.5 km per decade (Lenoir et al., Reference Lenoir, Bertrand, Comte, Bourgeaud, Hattab, Murienne and Grenouillet2020; Fredston et al., Reference Fredston, Pinsky, Selden, Szuwalski, Thorson, Gaines and Halpern2021). Susceptibility to range shifts varies strongly by functional group, with short-lived, fast-growing planktonic organisms shifting their ranges much faster than longer-lived and sessile species, or species with fixed breeding sites (Poloczanska et al., Reference Poloczanska, Brown, Sydeman, Kiessling, Schoeman, Moore, Brander, Bruno, Buckley, Burrows, Duarte, Halpern, Holding, Kappel, O’Connor, Pandolfi, Parmesan, Schwing, Thompson and Richardson2013; Pinsky et al., Reference Pinsky, Selden and Kitchel2020). Although range edges of coastal species in tropical to temperate waters generally maintain the species’ thermal niches as the climate warms (Lenoir et al., Reference Lenoir, Bertrand, Comte, Bourgeaud, Hattab, Murienne and Grenouillet2020; Fredston et al., Reference Fredston, Pinsky, Selden, Szuwalski, Thorson, Gaines and Halpern2021), this tendency is both stronger and more common at the leading (cool) range edge than at the trailing (warm) range edge (Poloczanska et al., Reference Poloczanska, Brown, Sydeman, Kiessling, Schoeman, Moore, Brander, Bruno, Buckley, Burrows, Duarte, Halpern, Holding, Kappel, O’Connor, Pandolfi, Parmesan, Schwing, Thompson and Richardson2013; Fredston-Hermann et al., Reference Fredston-Hermann, Selden, Pinsky, Gaines and Halpern2020; Pinsky et al., Reference Pinsky, Selden and Kitchel2020). Pinsky et al. (Reference Pinsky, Selden and Kitchel2020) provide a detailed discussion of potential explanations for this phenomenon, including physiology, behaviour, evolution, dispersal and species interactions, but answers are elusive, and this question remains a topic of active research (e.g., Usui et al., Reference Usui, Lerner, Eckert, Angert, Garroway, Hargreaves, Lancaster, Lessard, Riva, Schmidt, van der Burg and Marshall2023).
Nevertheless, arguably the most ubiquitous consequence of differential range shifts at leading and trailing range edges is the arrival of warm-affinity species in communities previously dominated by – and still occupied by – species of cooler provenance (Vergés et al., Reference Vergés, Steinberg, Hay, Poore, Campbell, Ballesteros, Heck, Booth, Coleman, Feary, Figueira, Langlois, Marzinelli, Mizerek, Mumby, Nakamura, Roughan, Sebille, Gupta, Smale, Tomas, Wernberg and Wilson2014; Chaudhary et al., Reference Chaudhary, Richardson, Schoeman and Costello2021; Favoretto et al., Reference Favoretto, Sánchez and Aburto-Oropeza2022; Fujiwara et al., Reference Fujiwara, Simpson, Torres-Ceron and Martinez-Andrade2022). This process, known in low- to mid-latitudes as tropicalisation, in combination with the closely related process of deborealisation – the loss of cool-associated species from high-latitude places – results in the continual reassembly of biotic communities in coastal waters (McLean et al., Reference McLean, Mouillot, Maureaud, Hattab, MacNeil, Goberville, Lindegren, Engelhard, Pinsky and Auber2021). When tropicalisation involves the arrival of herbivores, seagrasses and macroalgal habitat can be rapidly transformed (Vergés et al., Reference Vergés, Doropoulos, Malcolm, Skye, Garcia-Pizá, Marzinelli, Campbell, Ballesteros, Hoey, Vila-Concejo, Y-M and Steinberg2016, Reference Vergés, Lanham, Kono, Okumura and Nakamura2022; Schuster et al., Reference Schuster, Stuart-Smith, Edgar and Bates2022; Santana-Garcon et al., Reference Santana-Garcon, Bennett, Marbà, Vergés, Arthur and Alcoverro2023), reshaping entire ecosystems and their functioning (Peleg et al., Reference Peleg, Guy-Haim, Yeruham, Silverman and Rilov2020). But other arriving taxa can have equally profound impacts (de et al., Reference de Vargas Ribeiro, Pessarrodona, Tucket, Mulders, Pereira and Wernberg2022). Despite evidence that “healthy” ecosystems with relatively intact biodiversity can resist climate change of the magnitude already witnessed (Bates et al., Reference Bates, Barrett, Stuart-Smith, Holbrook, Thompson and Edgar2014; Frid et al., Reference Frid, Malamud, Franco, Guidetti, Azzurro, Claudet, Micheli, Yahel, Sala and Belmaker2023; Ziegler et al., Reference Ziegler, Johnson, Brooks, Johnston, Mohay, Ruttenberg, Starr, Waltz, Wendt and Hamilton2023), the inevitability of range shifts identifies the network effects of tropicalisation and deborealisation as an urgent research priority.
Although range shifts are the most commonly studied ecological response to warming, some coastal species might respond to warming surface waters by seeking refuge at depth (Giraldo-Ospina et al., Reference Giraldo-Ospina, Kendrick and Hovey2020). Evidence of such depth shifts, however, remains somewhat contradictory (Chaikin and Belmaker, Reference Chaikin and Belmaker2023; Rubenstein et al., Reference Rubenstein, Weiskopf, Bertrand, Carter, Comte, Eaton, Johnson, Lenoir, Lynch, Miller, Morelli, Rodriguez, Terando and Thompson2023).
Marine heatwaves (MHWs)
MHWs can expose marine life to conditions beyond those projected for the end of this century for periods ranging from days to months (Sen Gupta et al., Reference Sen Gupta, Thomsen, Benthuysen, Hobday, Oliver, Alexander, Burrows, Donat, Feng, Holbrook, Perkins‐Kirkpatrick, Moore, Rodrigues, Scannell, Taschetto, Ummenhofer, Wernberg and Smale2020; Koehlinger et al., Reference Koehlinger, Newton, Mickett, Thompson and Klinger2023). It is therefore unsurprising that MHWs impact all levels of marine life, from the ecophysiology of individual organisms to the structure of marine communities (Smith et al., Reference Smith, Burrows, Hobday, King, Moore, Gupta, Thomsen, Wernberg and Smale2023). Although some effects can be beneficial, especially at high latitudes – for example, increased productivity or reproductive success in populations close to their leading range edge (Smith et al., Reference Smith, Dowling and Brown2019; Favoretto et al., Reference Favoretto, Sánchez and Aburto-Oropeza2022; Magel et al., Reference Magel, Chan, Hessing-Lewis and Hacker2022) – many effects are detrimental. MHWs can reduce breeding success (Hays et al., Reference Hays, Chivers, Laloë, Sheppard and Esteban2021; Rogers et al., Reference Rogers, Wilson, Duffy-Anderson, Kimmel and Lamb2021), cause trailing-edge extirpations and consequent regime shifts (Arafeh-Dalmau et al., Reference Arafeh-Dalmau, Montaño-Moctezuma, Martínez, Beas-Luna, Schoeman and Torres-Moye2019; Wernberg, Reference Wernberg, Canadell and Jackson2021; Félix-Loaiza et al., Reference Félix-Loaiza, Rodríguez-Bravo, Beas-Luna, Lorda, de La Cruz-González and Malpica-Cruz2022), facilitate geographic shifts at leading range edges (Smith et al., Reference Smith, Dowling and Brown2019; Favoretto et al., Reference Favoretto, Sánchez and Aburto-Oropeza2022; Coulson et al., Reference Coulson, Leary, Chandrapavan, Wakefield and Newman2023) and benefit non-native invasive species (Arafeh-Dalmau et al., Reference Arafeh-Dalmau, Montaño-Moctezuma, Martínez, Beas-Luna, Schoeman and Torres-Moye2019), drive mass mortalities in natural (Piatt et al., Reference Piatt, Parrish, Renner, Schoen, Jones, Arimitsu, Kuletz, Bodenstein, García-Reyes, Duerr, Corcoran, Kaler, McChesney, Golightly, Coletti, Suryan, Burgess, Lindsey, Lindquist, Warzybok, Jahncke, Roletto and Sydeman2020) and aquaculture (Smith et al., Reference Smith, Burrows, Hobday, Gupta, Moore, Thomsen, Wernberg and Smale2021) settings, exacerbate infectious disease epidemics (Harvell et al., Reference Harvell, Montecino-Latorre, Caldwell, Burt, Bosley, Keller, Heron, Salomon, Lee, Pontier, Pattengill-Semmens and Gaydos2019; Claar and Wood, Reference Claar and Wood2020; Genin et al., Reference Genin, Levy, Sharon, Raitsos and Diamant2020), and impact habitat-forming taxa, including seagrasses (Strydom et al., Reference Strydom, Murray, Wilson, Huntley, Rule, Heithaus, Bessey, Kendrick, Burkholder, Holmes, Fraser and Zdunic2020), kelps (Arafeh-Dalmau et al., Reference Arafeh-Dalmau, Montaño-Moctezuma, Martínez, Beas-Luna, Schoeman and Torres-Moye2019; Wernberg, Reference Wernberg, Canadell and Jackson2021) and corals (Goreau and Hayes, Reference Goreau and Hayes2021; Speare et al., Reference Speare, Adam, Winslow, Lenihan and Burkepile2022; van Woesik et al., Reference Woesik, Shlesinger, Grottoli, Toonen, Thurber, Warner, Hulver, Chapron, McLachlan, Albright, Crandall, DeCarlo, Donovan, Eirin‐Lopez, Harrison, Heron, Huang, Humanes, Krueger, Madin, Manzello, McManus, Matz, Muller, Rodriguez‐Lanetty, Vega‐Rodriguez, Voolstra and Zaneveld2022). However, the magnitude of effects varies by taxon, location, ecosystem type and health, and even genotype, as well as the intensity, duration, abruptness of onset and return interval of the MHW event (Smith et al., Reference Smith, Dowling and Brown2019; Fox et al., Reference Fox, Cohen, Rotjan, Mangubhai, Sandin, Smith, Thorrold, Dissly, Mollica and Obura2021; Suryan et al., Reference Suryan, Arimitsu, Coletti, Hopcroft, Lindeberg, Barbeaux, Batten, Burt, Bishop, Bodkin, Brenner, Campbell, Cushing, Danielson, Dorn, Drummond, Esler, Gelatt, Hanselman, Hatch, Haught, Holderied, Iken, Irons, Kettle, Kimmel, Konar, Kuletz, Laurel, Maniscalco, Matkin, McKinstry, Monson, Moran, Olsen, Palsson, Pegau, Piatt, Rogers, Rojek, Schaefer, Spies, Straley, Strom, Sweeney, Szymkowiak, Weitzman, Yasumiishi and Zador2021; Magel et al., Reference Magel, Chan, Hessing-Lewis and Hacker2022; Shlesinger and van Woesik, Reference Shlesinger and van Woesik2023; Ziegler et al., Reference Ziegler, Johnson, Brooks, Johnston, Mohay, Ruttenberg, Starr, Waltz, Wendt and Hamilton2023).
Phenology
Another conspicuous impact of ocean warming is the earlier attainment of typical spring temperatures and later attainment of autumn temperatures, which are both important in setting the timing of key seasonal events, such as breeding and migration. Although the timing of such seasonal events is not regulated by temperature signals alone (Ducklow et al., Reference Ducklow, Cimino, Dunton, Fraser, Hopcroft, Ji, Miller, Ohman and Sosik2022; Whelan et al., Reference Whelan, Hatch, Gaston, Gilchrist and Elliott2022), a meta-analysis of phenological studies indicates that ocean warming has driven spring events 4.3 ± 1.8 days to 7.5 ± 1.5 days earlier per decade among planktonic organisms and 3.0 ± 2.1 days earlier per decade for fish (Cooley et al., Reference Cooley, Schoeman, Bopp, Boyd, Donner, Ghebrehiwet, Ito, Kiessling, Martinetto, Ojea, Racault, Rost, Skern-Mauritzen, Pörtner, Roberts, Tignor, Poloczanska, Mintenbeck, Alegría, Craig, Langsdorf, Löschke, Möller, Okem and Rama2022). Although there is more and stronger evidence for climate-driven phenological shifts among small, short-lived taxa (Cooley et al., Reference Cooley, Schoeman, Bopp, Boyd, Donner, Ghebrehiwet, Ito, Kiessling, Martinetto, Ojea, Racault, Rost, Skern-Mauritzen, Pörtner, Roberts, Tignor, Poloczanska, Mintenbeck, Alegría, Craig, Langsdorf, Löschke, Möller, Okem and Rama2022), recent evidence confirms such responses among large, long-lived taxa, including whales (Ganley et al., Reference Ganley, Byrnes, Pendleton, Mayo, Friedland, Redfern, Turner and Brault2022; Pendleton et al., Reference Pendleton, Tingley, Ganley, Friedland, Mayo, Brown, McKenna, Jordaan and Staudinger2022; Shuert et al., Reference Shuert, Marcoux, Hussey, Heide-Jørgensen, Dietz and Auger-Méthé2022) and sharks (Hammerschlag et al., Reference Hammerschlag, McDonnell, Rider, Street, Hazen, Natanson, McCandless, Boudreau, Gallagher, Pinsky and Kirtman2022). Moreover, since both range shifts and phenological shifts are responses to warming, it is unsurprising that taxa with high geographic fidelity (e.g., those with specific spawning or nesting requirements) might demonstrate stronger phenological responses than those that more readily shift ranges (Chust et al., Reference Chust, Taboada, Alvarez and Ibaibarriaga2023).
Deoxygenation
Progressive loss of oxygen – deoxygenation – has been observed in the ocean interior since the mid-twentieth century (Canadell et al., Reference Canadell, Monteiro, Costa, Cotrim da Cunha, Cox, Eliseev, Henson, Ishii, Jaccard, Koven, Lohila, Patra, Piao, Rogelj, Syampungani, Zaehle, Zickfeld, Masson-Delmotte, Zhai, Pirani, Connors, Péan, Berger, Caud, Chen, Goldfarb, Gomis, Huang, Leitzell, Lonnoy, JBR, Maycock, Waterfield, Yelekçl, Yu and Zhou2021). Normally, oxygen enters the upper ocean from the atmosphere and from photosynthesis by aquatic vegetation (including phytoplankton), then vertical mixing moves oxygen into the deep ocean, where it is respired by heterotrophic marine organisms. About 15% of the observed deoxygenation is attributed to warming-induced decreases in oxygen solubility, and the rest is attributed to increased stratification (Canadell et al., Reference Canadell, Monteiro, Costa, Cotrim da Cunha, Cox, Eliseev, Henson, Ishii, Jaccard, Koven, Lohila, Patra, Piao, Rogelj, Syampungani, Zaehle, Zickfeld, Masson-Delmotte, Zhai, Pirani, Connors, Péan, Berger, Caud, Chen, Goldfarb, Gomis, Huang, Leitzell, Lonnoy, JBR, Maycock, Waterfield, Yelekçl, Yu and Zhou2021).
Deoxygenation is transforming marine communities by increasing individual species’ migration, replacement and loss (Cooley et al., Reference Cooley, Schoeman, Bopp, Boyd, Donner, Ghebrehiwet, Ito, Kiessling, Martinetto, Ojea, Racault, Rost, Skern-Mauritzen, Pörtner, Roberts, Tignor, Poloczanska, Mintenbeck, Alegría, Craig, Langsdorf, Löschke, Möller, Okem and Rama2022) by, for example, altering the dynamics of aquatic infectious diseases (Burge and Hershberger, Reference Burge, Hershberger, Behringer, Silliman and Lafferty2020; Byers, Reference Byers2021) and threatening tropical shallow-water coral reefs with lethal and sublethal effects (Hughes et al., Reference Hughes, Alderdice, Cooney, Kühl, Pernice, Voolstra and Suggett2020; Pezner et al., Reference Pezner, Courtney, Barkley, Chou, Chu, Clements, Cyronak, DeGrandpre, Kekuewa, Kline, Liang, Martz, Mitarai, Page, Rintoul, Smith, Soong, Takeshita, Tresguerres, Wei, Yates and Andersson2023). In mid-waters, deoxygenation is thought to compress habitat for pelagic oceanic fish species and temporarily increase catchability (Breitburg et al., Reference Breitburg, Levin, Oschlies, Grégoire, Chavez, Conley and Zhang2018).
Acidification
The decrease in surface ocean pH observed over the past 40 years due to the uptake of anthropogenically released atmospheric CO2 has altered the water chemistry surrounding upper-ocean ecosystems more than in the past 26,000 or more years (Arias et al., Reference Arias, Bellouin, Coppola, Jones, Krinner, Marotzke, Naik, Palmer, Plattner, Rogelj, Rojas, Sillmann, Storelvmo, Thorne, Trewin, Achuta Rao, Adhikary, Allan, Armour, Bala, Barimalala, Berger, Canadell, Cassou, Cherchi, Collins, Collins, Connors, Corti, Cruz, Dentener, Dereczynski, Di Luca, Diongue Niang, Doblas-Reyes, Dosio, Douville, Engelbrecht, Eyring, Fischer, Forster, Fox-Kemper, Fuglestvedt, Fyfe, Gillett, Goldfarb, Gorodetskaya, Gutierrez, Hamdi, Hawkins, Hewitt, Hope, Islam, Jones, Kaufman, Kopp, Kosaka, Kossin, Krakovska, Lee, Li, Mauritsen, Maycock, Meinshausen, Min, Monteiro, Ngo-Duc TOtto, Pinto, Pirani, Raghavan, Ranasinghe, Ruane, Ruiz, Sallée, Samset, Sathyendranath, Seneviratne, Sörensson, Szopa, Takayabu, Tréguier, van den Hurk, Vautard, von Schuckmann, Zaehle, Zhang, Zickfeld, Masson-Delmotte, Zhai, Pirani, Connors, Péan, Berger, Caud, Chen, Goldfarb, Gomis, Huang, Leitzell, Lonnoy, JBR, Maycock, Waterfield, Yelekçl, Yu and Zhou2021). Ocean acidification can have a variety of effects on biological processes: higher aquatic dissolved CO2 concentrations tend to increase photosynthesis of some primary producers, while higher H+ ion concentrations (i.e., greater acidity or lower pH) tend to challenge calcification – the biological creation of calcium carbonate shells and skeletons – for several animals or planktonic species, especially juveniles (Doney et al., Reference Doney, Busch, Cooley and Kroeker2020). Loss of juvenile Pacific oysters in aquaculture facilities (Barton et al., Reference Barton, Waldbusser, Feely, Weisberg, Newton, Hales, Cudd, Benoit Eudeline, Langdon, Jefferds, King, Suhrbier and McLaughlin2015) and increased bioerosion and dissolution of tropical corals in nature have been attributed to ocean acidification, but the complexity and variety of the effects of acidification on marine species, along with species’ exposure to multiple simultaneous drivers, makes attributing many individual and most ecosystem-scale outcomes to ocean acidification extremely challenging (Doo et al., Reference Doo, Kealoha, Andersson, Cohen, Hicks, Johnson, Long, McElhany, Mollica, Shamberger, Silbiger, Takeshita and Busch2020).
Sea-level rise
Thermal expansion of the ocean and, more recently, freshwater input from the loss of ice mass from terrestrial glaciers are driving up the global mean sea level (Fox-Kemper et al., Reference Fox-Kemper, Hewitt, Xiao, Aðalgeirsdóttir, Drijfhout, Edwards, Golledge, Hemer, Kopp, Krinner, Mix, Notz, Nowicki, Nurhati, Ruiz, Sallée, ABA, Yu, Masson-Delmotte, Zhai, Pirani, Connors, Péan, Berger, Caud, Chen, Goldfarb, Gomis, Huang, Leitzell, Lonnoy, JBR, Maycock, Waterfield, Yelekçl, Yu and Zhou2021). Because coastlines can be subsiding (e.g., due to freshwater extraction) or experiencing isostatic rebound from the last glaciation (Durand et al., Reference Durand, van den Broeke, Le Cozannet, Edwards, Holland, Jourdain, Marzeion, Mottram, Nicholls, Pattyn, Paul, Slangen, Winkelmann, Burgard, van Calcar, Barré, Bataille and Chapuis2022), this sea-level rise is experienced as the change in the mean sea level relative to the land – relative sea-level rise. Almost all intertidal and shallow subtidal coastal ecosystems are sensitive to relative sea-level rise (Cooley et al., Reference Cooley, Schoeman, Bopp, Boyd, Donner, Ghebrehiwet, Ito, Kiessling, Martinetto, Ojea, Racault, Rost, Skern-Mauritzen, Pörtner, Roberts, Tignor, Poloczanska, Mintenbeck, Alegría, Craig, Langsdorf, Löschke, Möller, Okem and Rama2022). Observed impacts include flooding at high-tide extremes (Lawrence et al., Reference Lawrence, Mackey, Chiew, Costello, Hennessy, Lansbury, Lansbury, Nidumolu, Pecl, Rickards, Tapper, Woodward, Wreford, Pörtner, Roberts, Tignor, Poloczanska, Mintenbeck, Alegría, Craig, Langsdorf, Löschke, Möller, Okem and Rama2022); salinisation of coastal soils, wetlands and the upper reaches of estuaries, with associated ecosystem transitions (Peteet et al., Reference Peteet, Nichols, Kenna, Chang, Browne, Reza, Kovari, Liberman and Stern-Protz2018; Andres et al., Reference Andres, Savarese, Bovard and Parsons2019; Kirwan and Gedan, Reference Kirwan and Gedan2019; Grieger et al., Reference Grieger, Capon, Hadwen and Mackey2020; Eswar et al., Reference Eswar, Karuppusamy and Chellamuthu2021); increased erosion (e.g., Peteet et al., Reference Peteet, Nichols, Kenna, Chang, Browne, Reza, Kovari, Liberman and Stern-Protz2018); and coastal storm and flood damage (e.g., Strauss et al., Reference Strauss, Orton, Bittermann, Buchanan, Gilford, Kopp, Kulp, Massey, de Moel and Vinogradov2021). Counterintuitively, relative sea-level rise can also result in accretion of intertidal sediments in areas where wetland vegetation can generate or trap sediments at rates exceeding those of relative sea-level rise (e.g., Marx et al., Reference Marx, Knight, Dwyer, Child, Hotchkis and Zawadzki2020; Saintilan et al., Reference Saintilan, Khan, Ashe, Kelleway, Rogers, Woodroffe and Horton2020).
Despite these observations, the impacts of relative sea-level rise are compounded with and confounded by other anthropogenic stressors at the coast, as well as the widespread deployment of countermeasures, including beach nourishment and other forms of coastal restoration and protection (Cooley et al., Reference Cooley, Schoeman, Bopp, Boyd, Donner, Ghebrehiwet, Ito, Kiessling, Martinetto, Ojea, Racault, Rost, Skern-Mauritzen, Pörtner, Roberts, Tignor, Poloczanska, Mintenbeck, Alegría, Craig, Langsdorf, Löschke, Möller, Okem and Rama2022). Global analyses of relatively coarse-scale imagery suggest the net effects of these processes have resulted in the loss of 15% of tidal flats since 1984 (Mentaschi et al., Reference Mentaschi, Vousdoukas, Pekel, Voukouvalas and Feyen2018; Murray et al., Reference Murray, Worthington, Bunting, Duce, Hagger, Lovelock, Lucas, Saunders, Sheaves, Spalding, Waltham and Lyons2022), but with a corresponding number of the world’s beaches accreting (28%) as eroding (24%) (Luijendijk et al., Reference Luijendijk, Hagenaars, Ranasinghe, Baart, Donchyts and Aarninkhof2018).
Ice loss
The effects of ice loss on coastal ecosystems are so far most keenly felt in the Arctic (Meredith et al., Reference Meredith, Sommerkorn, Cassotta, Derksen, Ekaykin, Hollowed, Kofinas, Mackintosh, Melbourne-Thomas, MMC, Ottersen, Pritchard, EAG, Pörtner, Roberts, Masson-Delmotte, Zhai, Tignor, Poloczanska, Mintenbeck, Alegría, Nicolai, Okem, Petzold, Rama and Weyer2019; Cooley et al., Reference Cooley, Schoeman, Bopp, Boyd, Donner, Ghebrehiwet, Ito, Kiessling, Martinetto, Ojea, Racault, Rost, Skern-Mauritzen, Pörtner, Roberts, Tignor, Poloczanska, Mintenbeck, Alegría, Craig, Langsdorf, Löschke, Möller, Okem and Rama2022). Here, the formation, melting and persistence of sea ice drives seasonal patterns of coastal productivity, breeding and feeding opportunities, and connectivity (Le Moullec and Bender, Reference Le Moullec, Bender, Finger and Rekvig2022). Sea ice can be disruptive, through processes like benthic scouring, but can also be protective, through processes like buffering of coastal erosion (Lebrun et al., Reference Lebrun, Comeau, Gazeau and Gattuso2022). Irrespective, loss of ice in Arctic coastal systems can have cascading impacts (Meredith et al., Reference Meredith, Sommerkorn, Cassotta, Derksen, Ekaykin, Hollowed, Kofinas, Mackintosh, Melbourne-Thomas, MMC, Ottersen, Pritchard, EAG, Pörtner, Roberts, Masson-Delmotte, Zhai, Tignor, Poloczanska, Mintenbeck, Alegría, Nicolai, Okem, Petzold, Rama and Weyer2019; Cooley et al., Reference Cooley, Schoeman, Bopp, Boyd, Donner, Ghebrehiwet, Ito, Kiessling, Martinetto, Ojea, Racault, Rost, Skern-Mauritzen, Pörtner, Roberts, Tignor, Poloczanska, Mintenbeck, Alegría, Craig, Langsdorf, Löschke, Möller, Okem and Rama2022), including the poleward movement of primary productivity driven by spring melt, with concomitant impacts for benthic and pelagic communities and the predators that feed on these (Brandt et al., Reference Brandt, Wassmann and Piepenburg2023), including iconic species such as polar bears and walruses (Lebrun et al., Reference Lebrun, Comeau, Gazeau and Gattuso2022; Alabia et al., Reference Alabia, García Molinos, Hirata, Mueter and David2023; Kellner et al., Reference Kellner, Atwood, Douglas, Breck and Wittemyer2023). Changes in ice phenology also impact phenology and breeding success among seabirds (Cusset et al., Reference Cusset, Fort, Mallory, Braune, Massicotte and Massé2019; Descamps et al., Reference Descamps, Ramírez, Benjaminsen, Anker-Nilssen, Barrett, Burr, Christensen-Dalsgaard, Erikstad, Irons, Lorentsen, Mallory, Robertson, Reiertsen, Strøm, Varpe and Lavergne2019; Golubova, Reference Golubova2021). Despite these examples of impact, there is considerable variation among taxa and locations (Gutowsky et al., Reference Gutowsky, Baak, Gaston and Mallory2022; Grémillet and Descamps, Reference Grémillet and Descamps2023). Trends in ice loss and their attribution to climate change are both more uncertain in the Antarctic (Fox-Kemper et al., Reference Fox-Kemper, Hewitt, Xiao, Aðalgeirsdóttir, Drijfhout, Edwards, Golledge, Hemer, Kopp, Krinner, Mix, Notz, Nowicki, Nurhati, Ruiz, Sallée, ABA, Yu, Masson-Delmotte, Zhai, Pirani, Connors, Péan, Berger, Caud, Chen, Goldfarb, Gomis, Huang, Leitzell, Lonnoy, JBR, Maycock, Waterfield, Yelekçl, Yu and Zhou2021; Cooley et al., Reference Cooley, Schoeman, Bopp, Boyd, Donner, Ghebrehiwet, Ito, Kiessling, Martinetto, Ojea, Racault, Rost, Skern-Mauritzen, Pörtner, Roberts, Tignor, Poloczanska, Mintenbeck, Alegría, Craig, Langsdorf, Löschke, Möller, Okem and Rama2022).
Other climate-induced drivers
Coastal ecosystems and their resident organisms are variously sensitive to a range of climate-induced drivers beyond those discussed above (Cooley et al., Reference Cooley, Schoeman, Bopp, Boyd, Donner, Ghebrehiwet, Ito, Kiessling, Martinetto, Ojea, Racault, Rost, Skern-Mauritzen, Pörtner, Roberts, Tignor, Poloczanska, Mintenbeck, Alegría, Craig, Langsdorf, Löschke, Möller, Okem and Rama2022). Included amongst these are drivers, such as changes in ocean salinity and stratification, that are not yet expected to elicit ecological responses large enough to be detectable as climate-change impacts. Also included are changes in wave height and power (Young and Ribal, Reference Young and Ribal2019; Odériz et al., Reference Odériz, Silva, Mortlock, Mori, Shimura, Webb, Padilla-Hernández and Villers2021) and ocean circulation (e.g., Hu et al., Reference Hu, Sprintall, Guan, McPhaden, Wang, Hu and Cai2020), which are yet to be confidently detected and attributed (Fox-Kemper et al., Reference Fox-Kemper, Hewitt, Xiao, Aðalgeirsdóttir, Drijfhout, Edwards, Golledge, Hemer, Kopp, Krinner, Mix, Notz, Nowicki, Nurhati, Ruiz, Sallée, ABA, Yu, Masson-Delmotte, Zhai, Pirani, Connors, Péan, Berger, Caud, Chen, Goldfarb, Gomis, Huang, Leitzell, Lonnoy, JBR, Maycock, Waterfield, Yelekçl, Yu and Zhou2021; Gulev et al., Reference Gulev, Thorne, Ahn, Dentener, Domingues, Gerland, Gong, Kaufman, Nnamchi, Quaas, Rivera, Sathyendranath, Smith, Trewin, von Schuckmann, Vose, Masson-Delmotte, Zhai, Pirani, Connors, Péan, Berger, Caud, Chen, Goldfarb, Gomis, Huang, Leitzell, Lonnoy, Matthews, Maycock, Waterfield, Yelekçl, Yu and Zhou2021), and atmospheric phenomena that are difficult to predict, let alone project, such as tropical cyclones and storms. The latter can impact coastal systems such as vegetated wetlands and exposed sandy beaches, but with effects that are case-specific, and sometimes counter-intuitive. For example, the precipitation, wind and wave action associated with storms can rearrange coastal sediments, causing erosion in some places and accretion elsewhere (Xie et al., Reference Xie, He, Zhang, Guo, Wang, Shen and Cui2017; Armitage et al., Reference Armitage, Weaver, Kominoski and Pennings2020; Mo et al., Reference Mo, Kearney and Turner2020; Wang et al., Reference Wang, Dai, Mei and Fagherazzi2020). In other cases, impacts can be indirect: heavy precipitation associated with storms can increase estuarine nutrient loads via runoff from adjacent land, causing or exacerbating eutrophication and stimulating HABs (Phlips et al., Reference Phlips, Badylak, Nelson and Havens2020; Dai et al., Reference Dai, Zhao, Chai, Chen, Chen, Chen, Cheng, Gan, Guan, Hong, Huang, Lee, Leung, Lim, Lin, Lin, Liu, Liu, Luo, Meng, Sangmanee, Shen, Uthaipan, Talaat, Wan, Wang, Wang, Wang, Wang, Wang, Wang, Wang, Wang, Xu, Yang, Yang, Yasuhara, Yu, Yu, Yu, Zhang and Zhang2023), sometimes causing large-scale marine mammal, bird, and fish kills (Adams et al., Reference Adams, Tremain, Paperno and Sonne2019). Similarly, strong winds from tropical storms and cyclones can be beneficial for mangroves (Castañeda-Moya et al., Reference Castañeda-Moya, Rivera-Monroy, Chambers, Zhao, Lamb-Wotton, Gorsky, Gaiser, Troxlera, Kominoski and Hiatt2020; Feher et al., Reference Feher, Osland, Anderson, Vervaeke, Krauss, Whelan, Balentine, Tiling-Range, Smith and Cahoon2020), or cause ephemeral damage (Armitage et al., Reference Armitage, Weaver, Kominoski and Pennings2020; Branoff, Reference Branoff2020), but they can also initiate regime shifts involving peat collapse and transition to mudflats (Chambers et al., Reference Chambers, Steinmuller and Breithaupt2019; Osland et al., Reference Osland, Feher, Anderson, Vervaeke, Krauss, Whelan, Balentine, Tiling-Range, Smith and Cahoon2020).
Compound events
Although discussion so far has focused on the direct impacts caused by individual climate-induced drivers, in real-world situations, none of these operate in isolation. Instead, they combine and interact in various ways. This complicates the task of attributing observed ecological responses to any single driver (Parmesan et al., Reference Parmesan, Burrows, Duarte, Poloczanska, Richardson, Schoeman and Singer2013). For example, because ocean acidification and deoxygenation both depend on the solubility of gases in seawater – which is temperature dependent – these climate-induced drivers operate in concert with ocean warming to change the physiological suitability of coastal waters for marine fish and invertebrates.
Because temperature fundamentally affects the metabolism, motility, feeding efficiency and breeding success of marine organisms (Grady et al., Reference Grady, Maitner, Winter, Kaschner, Tittensor, Record, Smith, Wilson, Dell, Zarnetske, Wearing, Alfaro and Brown2019), ocean warming can also modify ecological interactions. Among the many examples of this phenomenon, one is of increasing concern: the host–pathogen interactions that drive outbreaks of infectious diseases in a range of coastal and marine taxa (Harvell et al., Reference Harvell, Mitchell, Ward, Altizer, Dobson, Ostfeld and Samuel2002; Randall and van Woesik, Reference Randall and van Woesik2015; Cohen et al., Reference Cohen, James, Lee, Martinelli, Muraoka, Ortega, Sadowski, Starkey, Szesciorka, Timko, Weiss and Franks2018; Harvell et al., Reference Harvell, Montecino-Latorre, Caldwell, Burt, Bosley, Keller, Heron, Salomon, Lee, Pontier, Pattengill-Semmens and Gaydos2019). Since metabolic activity in ectothermic fish is temperature-dependent, warming temperatures, in concert with parasite exposure, are likely to facilitate the proliferation of disease-causing organisms and affect the health of fish hosts (Scharsack et al., Reference Scharsack, Wieczorek, Schmidt-Drewello, Büscher, Franke, Moore, Antoine Branca, Witten, Stoll, Bornberg-Bauer, Wicke and Kurtz2021). In some cases, parasites grow faster and produce more viable eggs and offspring, which can lead to a rise in infection pressure, increased virulence, pathogenicity or expanded ranges for the parasite (Harvell et al., Reference Harvell, Mitchell, Ward, Altizer, Dobson, Ostfeld and Samuel2002; Arriaza et al., Reference Arriaza, Reinhard, Araújo, Orellana and Standen2010; Cohen et al., Reference Cohen, James, Lee, Martinelli, Muraoka, Ortega, Sadowski, Starkey, Szesciorka, Timko, Weiss and Franks2018; Scharsack et al., Reference Scharsack, Wieczorek, Schmidt-Drewello, Büscher, Franke, Moore, Antoine Branca, Witten, Stoll, Bornberg-Bauer, Wicke and Kurtz2021), and reduced fitness and/or mortality for the host. This can indirectly lead to trophic cascades in the warming habitat through changes to predation rates, thereby affecting ecosystem functioning (Harvell et al., Reference Harvell, Montecino-Latorre, Caldwell, Burt, Bosley, Keller, Heron, Salomon, Lee, Pontier, Pattengill-Semmens and Gaydos2019; Scharsack et al., Reference Scharsack, Wieczorek, Schmidt-Drewello, Büscher, Franke, Moore, Antoine Branca, Witten, Stoll, Bornberg-Bauer, Wicke and Kurtz2021).
Such compounded combinations of hazards in marine systems result in rates of extirpation twice as high as those experienced by terrestrial taxa (Pinsky et al., Reference Pinsky, Eikeset, McCauley, Payne and Sunday2019). On a global scale, the progressive loss of tropical biodiversity Chaudhary et al. (Reference Chaudhary, Richardson, Schoeman and Costello2021) provides stark evidence of these aggregate impacts of changes in the physical and chemical state of the ocean, in line with data from experiments and the palaeorecord (Reddin et al., Reference Reddin, Nätscher, Kocsis, H-O and Kiessling2020; Penn and Deutsch, Reference Penn and Deutsch2022).
The effects of climate change worsen and are worsened by the impacts on marine life of non-climate anthropogenic drivers
Not only do climate-induced drivers interact with each other, but their effects also modify and are modified by the effects of non-climate anthropogenic drivers (Sage, Reference Sage2020; Gissi et al., Reference Gissi, Manea, Mazaris, Fraschetti, Almpanidou, Bevilacqua, Coll, Guarnieri, Lloret-Lloret, Pascual, Petza, Rilov, Schonwald, Stelzenmüller and Katsanevakis2021; Cooley et al., Reference Cooley, Schoeman, Bopp, Boyd, Donner, Ghebrehiwet, Ito, Kiessling, Martinetto, Ojea, Racault, Rost, Skern-Mauritzen, Pörtner, Roberts, Tignor, Poloczanska, Mintenbeck, Alegría, Craig, Langsdorf, Löschke, Möller, Okem and Rama2022). This is particularly true – but under-recognised – in coastal ecosystems, few of which remain untouched by human activities (Williams et al., Reference Williams, Watson, Beyer, Klein, Montgomery, Runting, Roberson, Halpern, Grantham, Kuempel, Frazier, Venter and Wenger2022; Allan et al., Reference Allan, Levin and Kark2023), rendering them especially vulnerable to the coupled climate and biodiversity crises (Pörtner et al., Reference Pörtner, Scholes, Arneth, Barnes, Burrows, Diamond, Duarte, Kiessling, Leadley, Managi, McElwee, Midgley, Ngo, Obura, Pascual, Sankaran, Shin and Val2023).
The escalation of interactive effects of climate-induced drivers and other anthropogenic stressors is ubiquitous in coastal ecosystems (Halpern et al., Reference Halpern, Frazier, Afflerbach, Lowndes, Micheli, O’Hara, Scarborough and Selkoe2019; He and Silliman, Reference He and Silliman2019; Gissi et al., Reference Gissi, Manea, Mazaris, Fraschetti, Almpanidou, Bevilacqua, Coll, Guarnieri, Lloret-Lloret, Pascual, Petza, Rilov, Schonwald, Stelzenmüller and Katsanevakis2021). Although examples abound (Table 2), we will restrict our brief discussion here to impacts on tropical coral reefs as a case study.
Table 2. Examples of interactions among climate-induced drivers and other anthropogenic stressors in coastal ecosystems.
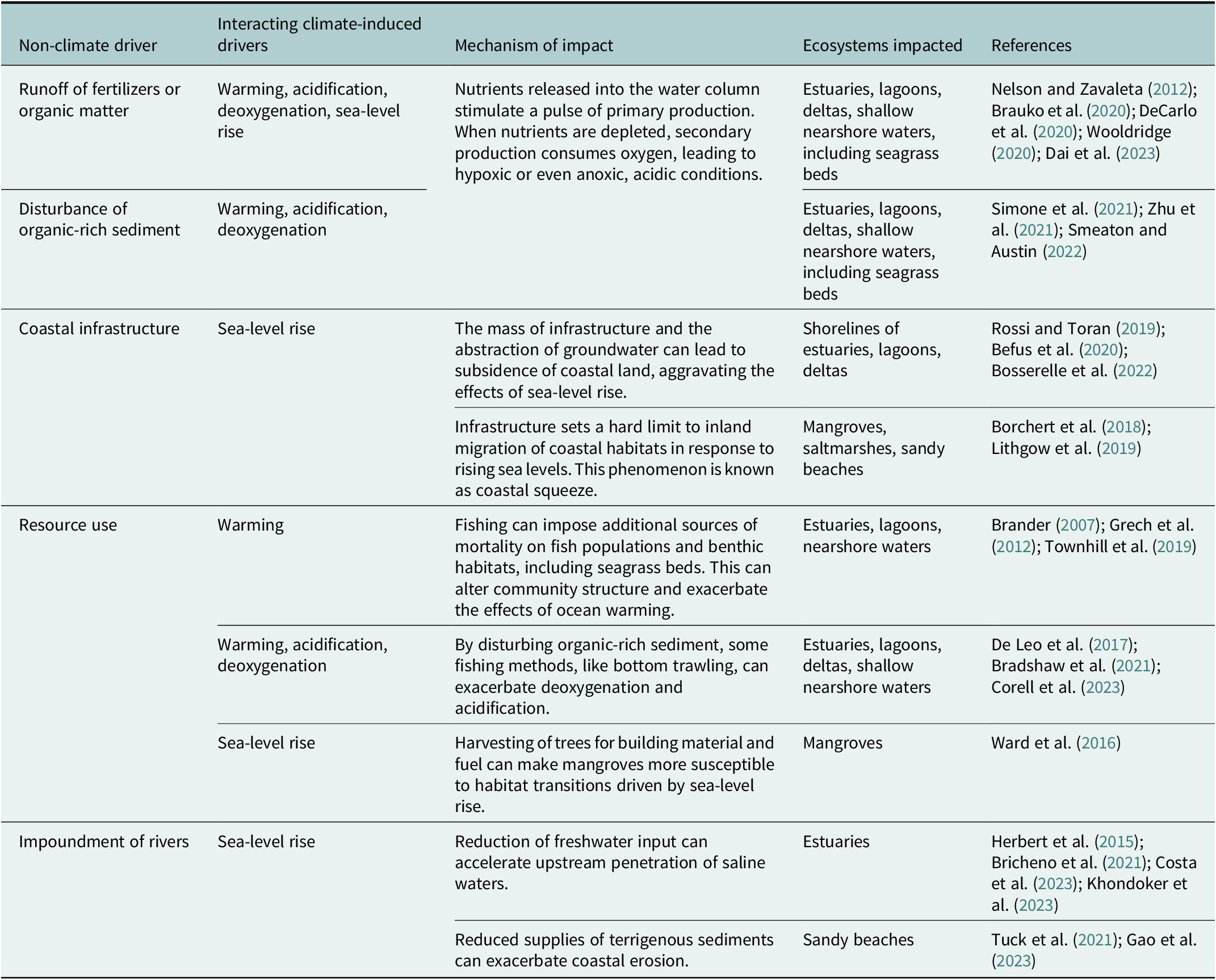
Corals are important habitat-forming species in tropical waters that support exceptionally high biodiversity (Fisher et al., Reference Fisher, O’Leary, Low-Choy, Mengersen, Knowlton, Brainard and Caley2015; Hughes et al., Reference Hughes, Barnes, Bellwood, Cinner, Cumming, Jackson, Kleypas, Leemput, Lough, Morrison, Palumbi, van Nes and Scheffer2017) and provide extensive ecosystem services (Eddy et al., Reference Eddy, Lam, Reygondeau, Cisneros-Montemayor, Greer, Palomares, Bruno, Ota and Cheung2021). Yet many coral taxa are sensitive to climate change, especially through ocean warming and acidification, as well as to other anthropogenic stressors, such as nutrient and sediment loading (Hughes et al., Reference Hughes, Barnes, Bellwood, Cinner, Cumming, Jackson, Kleypas, Leemput, Lough, Morrison, Palumbi, van Nes and Scheffer2017; Ellis et al., Reference Ellis, Jamil, Anlauf, Coker, Curdia, Hewitt, Jones, Krokos, Kürten, Hariprasad, Roth, Carvalho and Hoteit2019; Cornwall et al., Reference Cornwall, Comeau, Kornder, Perry, van Hooidonk, DeCarlo, Pratchett, Anderson, Browne, Carpenter, Diaz-Pulido, D’Olivo, Doo, Figueiredo, Fortunato, Kennedy, Lantz, McCulloch, González-Rivero, Schoepf, Smithers and Lowe2021; Zhao et al., Reference Zhao, Yuan, Strokal, Wu, Liu, Murk, Kroeze and Osinga2021; Cooley et al., Reference Cooley, Schoeman, Bopp, Boyd, Donner, Ghebrehiwet, Ito, Kiessling, Martinetto, Ojea, Racault, Rost, Skern-Mauritzen, Pörtner, Roberts, Tignor, Poloczanska, Mintenbeck, Alegría, Craig, Langsdorf, Löschke, Möller, Okem and Rama2022). Importantly, both vulnerability to and recovery from the impacts of climate change are affected by local anthropogenic stressors (França et al., Reference França, Benkwitt, Peralta, Robinson, Graham, Tylianakis, Berenguer, Lees, Ferreira, Louzada and Barlow2020; Cramer et al., Reference Cramer, Donovan, Jackson, Greenstein, Korpanty, Cook and Pandolfi2021; Donovan et al., Reference Donovan, Burkepile, Kratochwill, Shlesinger, Sully, Oliver, Hodgson, Freiwald and van Woesik2021). This is a double-edged sword: where reefs are exposed to both climate change and other human impacts, consequences can be severe; but this also means that well-designed climate-smart conservation interventions in these places, which both alleviate non-climate human impacts and deploy complementary strategies, should reduce vulnerability to climate change (Mellin et al., Reference Mellin, Matthews, Anthony, Brown, Caley, Johns, Osborne, Puotinen, Thompson, Wolff, Fordham and MacNeil2019; França et al., Reference França, Benkwitt, Peralta, Robinson, Graham, Tylianakis, Berenguer, Lees, Ferreira, Louzada and Barlow2020; Dutra et al., Reference Dutra, Haywood, Singh, Ferreira, Johnson, Veitayaki, Kininmonth, Morris and Piovano2021; Kuempel et al., Reference Kuempel, Tulloch, Giffin, Simmons, Hagger, Phua and Hoegh-Guldberg2022), at least in the short to medium term. Many other coastal ecosystems are less well-studied but would benefit equally from climate-smart conservation planning approaches (Brown et al., Reference Brown, Morrison, Schulz, Cross, Püschel-Hoeneisen, Suresh and Eguren2022; Doxa et al., Reference Doxa, Almpanidou, Katsanevakis, Queirós, Kaschner, Garilao, Reyes and Mazaris2022; Buenafe et al., Reference Buenafe, Dunn, Everett, Brito-Morales, Schoeman, Hanson, Alvise Dabalà, Neubert, Cannicci, Kaschner and Richardson2023).
Projecting future risks of climate change in coastal ecosystems
Given the difficulties in detecting and attributing climate-change impacts in coastal ecosystems, it should be no surprise that projecting future risks comes with even greater uncertainties. In some instances, projected risks of climate change are inferred from magnitudes of projected change in climate-driven hazards (Table 1, Figure 2), combined with the same established (or inferred) sensitivities to these drivers as are used in attributing observed impacts (Figure 1). In such cases, only the direction of change can be projected with any confidence (e.g., Hughes et al., Reference Hughes, Alderdice, Cooney, Kühl, Pernice, Voolstra and Suggett2020; Friess et al., Reference Friess, Adame, Adams and Lovelock2022). In other cases, statistical models – including, but not limited to species distribution models – are used to map ecological responses against climate-driven hazards, and then this model is used to project the magnitude of future change in that response variable (e.g., Moltó et al., Reference Moltó, Palmer, Ospina-Álvarez, Pérez-Mayol, Benseddik, Gatt, Morales-Nin, Alemany and Catalán2021; Van der Stocken et al., Reference Van der Stocken, Vanschoenwinkel, Carroll, Cavanaugh and Koedam2022; Chaudhary et al., Reference Chaudhary, Alfaro-Lucas, Simões, Brandt and Saeedi2023). More sophisticated, still, are ecosystem models that couple multiple environmental drivers to multiple interacting ecological response variables; these models are then used to extrapolate those interacting relationships forward under projected future climates (e.g., Moullec et al., Reference Moullec, Barrier, Drira, Guilhaumon, Marsaleix, Somot, Ulses, Velez and Shin2019; Tittensor et al., Reference Tittensor, Novaglio, Harrison, Heneghan, Barrier, Bianchi, Bopp, Bryndum-Buchholz, Britten, Büchner, Cheung, Christensen, Coll, Dunne, Eddy, Everett, Fernandes-Salvador, Fulton, Galbraith, Gascuel, Guiet, John, Link, Lotze, Maury, Ortega-Cisneros, Palacios-Abrantes, Petrik, du Pontavice, Rault, Richardson, Shannon, Shin, Steenbeek, Stock and Blanchard2021). All of these methods assume that the underlying models are transferable in time (and sometimes space), despite known problems with this assumption (Yates et al., Reference Yates, Bouchet, Caley, Mengersen, Randin, Parnell, Fielding, Bamford, Ban, Barbosa, Dormann, Elith, Embling, Ervin, Fisher, Gould, Graf, Gregr, Halpin, Heikkinen, Heinänen, Jones, Krishnakumar, Lauria, Lozano-Montes, Mannocci, Mellin, Mesgaran, Moreno-Amat, Mormede, Novaczek, Oppel, Crespo, Peterson, Rapacciuolo, Roberts, Ross, Scales, Schoeman, Snelgrove, Sundblad, Thuiller, Torres, Verbruggen, Wang, Wenger, Whittingham, Zharikov, Zurell and Sequeira2018; Neupane et al., Reference Neupane, Zipkin, Saunders and Ries2022; Rousseau and Betts, Reference Rousseau and Betts2022). But as with the detection of climate impacts, confidence in their attribution – and therefore their utility as predictors of future responses – increases in the presence of multiple lines of evidence.
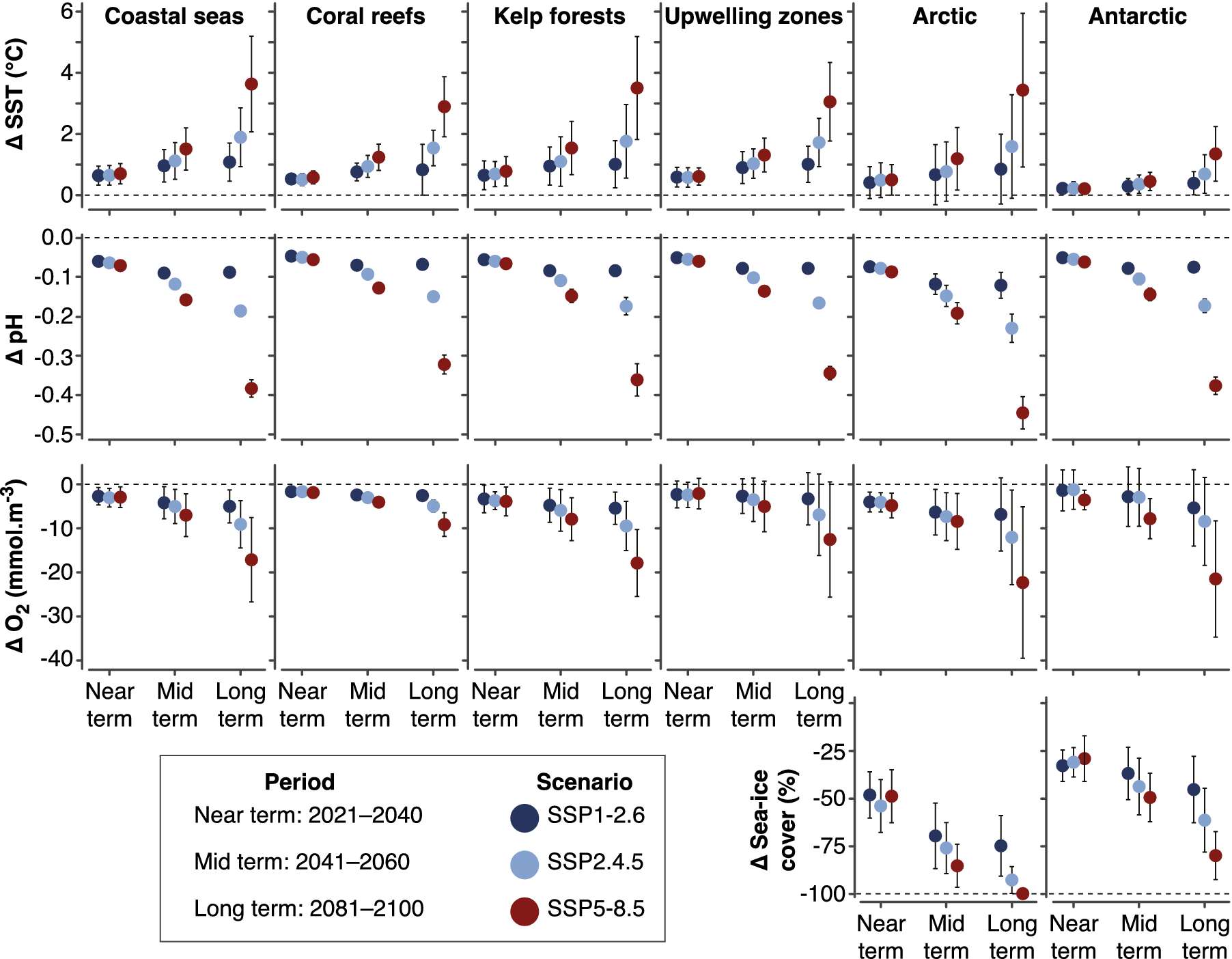
Figure 2. Projected changes in climate-induced drivers across coastal systems relative to the recent past (1985–2014), after Cooley et al. (Reference Cooley, Schoeman, Bopp, Boyd, Donner, Ghebrehiwet, Ito, Kiessling, Martinetto, Ojea, Racault, Rost, Skern-Mauritzen, Pörtner, Roberts, Tignor, Poloczanska, Mintenbeck, Alegría, Craig, Langsdorf, Löschke, Möller, Okem and Rama2022). Climate-induced drivers are arranged by row, while coastal systems are arranged by column. All measures are for the ocean surface, except changes in oxygen concentrations, which are subsurface (100–600 m) in upwelling and polar systems. Projections are derived from an ensemble of CMIP6 models interpolated to a 1° x 1° grid. Error bars represent 90% confidence.
An alternative approach that does not rely on projecting established relationships forward in time, involves using palaeodata to estimate the magnitude of ecological responses to past climate states analogous to projected future climates (Fordham et al., Reference Fordham, Jackson, Brown, Huntley, Brook, Dahl-Jensen, Gilbert, Otto-Bliesner, Svensson, Theodoridis, Wilmshurst, Buettel, Canteri, McDowell, Orlando, Pilowsky, Rahbek and Nogues-Bravo2020). Examples of such palaeodata for coastal ecosystems include reef and sediment cores (Jones et al., Reference Jones, Wingard, Stackhouse, Keller, Willard, Marot, Landacre and Bernhardt2019; Cohen et al., Reference Cohen, Figueiredo, Oliveira, Fontes, França, Pessenda, de Souza, Macario, Giannini, Bendassolli and Lima2020; Cramer et al., Reference Cramer, Donovan, Jackson, Greenstein, Korpanty, Cook and Pandolfi2021; Hesterberg et al., Reference Hesterberg, Jackson and Bell2022; Bograd et al., Reference Bograd, Jacox, Hazen, Lovecchio, Montes, Pozo Buil, Shannon, Sydeman and Rykaczewski2023). The advantages of palaeo-analogues of future climate are that they potentially account for natural adaptation in the taxa or systems impacted, and that human impacts are effectively eliminated (Kiessling et al., Reference Kiessling, Smith and Raja2023). But questions about the transferability of estimates remain.
Beyond the approaches used to project future risks of climate change, it is important, also, to ensure that the future being assessed is plausible. Recent reviews (Burgess et al., Reference Burgess, Becker, Langendorf, Fredston and Brooks2023; Schoeman et al., Reference Schoeman, Gupta, Harrison, Everett, Brito-Morales, Hannah, Bopp, Roehrdanz and Richardson2023) provide detailed analysis of the use of future scenarios (described in Table 3), as used in climate-change ecology. Results reveal that the practice of focusing exclusively on RCP8.5/SSP5-8.5 – often designated a “high-emissions scenario” – is common. But even when complemented by RCP2.6/SSP1-2.6 – commonly designated a “low-emissions scenario” – the use of this extreme scenario is problematic for projections out to 2100: while SSP1-2.6 is still attainable over this timeframe, SSP5-8.5 is highly unlikely (Hausfather and Peters, Reference Hausfather and Peters2020), despite being potentially useful over the near- to mid-term (Schwalm et al., Reference Schwalm, Glendon and Duffy2020). Instead, SSP2-4.5 (Table 3) is believed to be the most plausible of the common long-term scenarios, arguably along with SSP1-2.6, and while SSP3-7.0 is a better “business-as-usual” scenario than SSP5-8.5, especially in the long term, it is also reasonably implausible in some regards (Burgess et al., Reference Burgess, Becker, Langendorf, Fredston and Brooks2023). It is important to note, however, that the plausibility of future emissions scenarios depends heavily on whether countries’ ambitions to reduce such emissions will be matched with strong action (Rogelj et al., Reference Rogelj, Fransen, Den Elzen, Lamboll, Schumer, Kuramochi, Hans, Mooldijk and Portugal-Pereira2023). Irrespective, since much of the literature on projected impacts in the coastal zone by 2100 relies on RCP8.5/SSP5-8.5, significant caution is warranted when interpreting these projections.
Table 3. Policy context of common future climate scenarios (Meinshausen et al., Reference Meinshausen, Nicholls, Lewis, Gidden, Vogel, Freund, Beyerle, Gessner, Nauels, Bauer, Canadell, Daniel, John, Krummel, Luderer, Meinshausen, Montzka, Rayner, Reimann, Smith, van den Berg, Velders, Vollmer and Wang2020; Chen et al., Reference Chen, Rojas, Samset, Cobb, Diongue Niang, Edwards, Emori, Faria, Hawkins, Hope, Huybrechts, Meinshausen, Mustafa, Plattner, Tréguier, Masson-Delmotte, Zhai, Pirani, Connors, Péan, Berger, Caud, Chen, Goldfarb, Gomis, Huang, Leitzell, Lonnoy, Matthews, Maycock, Waterfield, Yelekçl, Yu and Zhou2021). Scenarios are named by Shared Socioeconomic Pathway (SSPs) and radiative forcing level in 2100, approximating Representative Concentration Pathways (RCPs; W m−2).
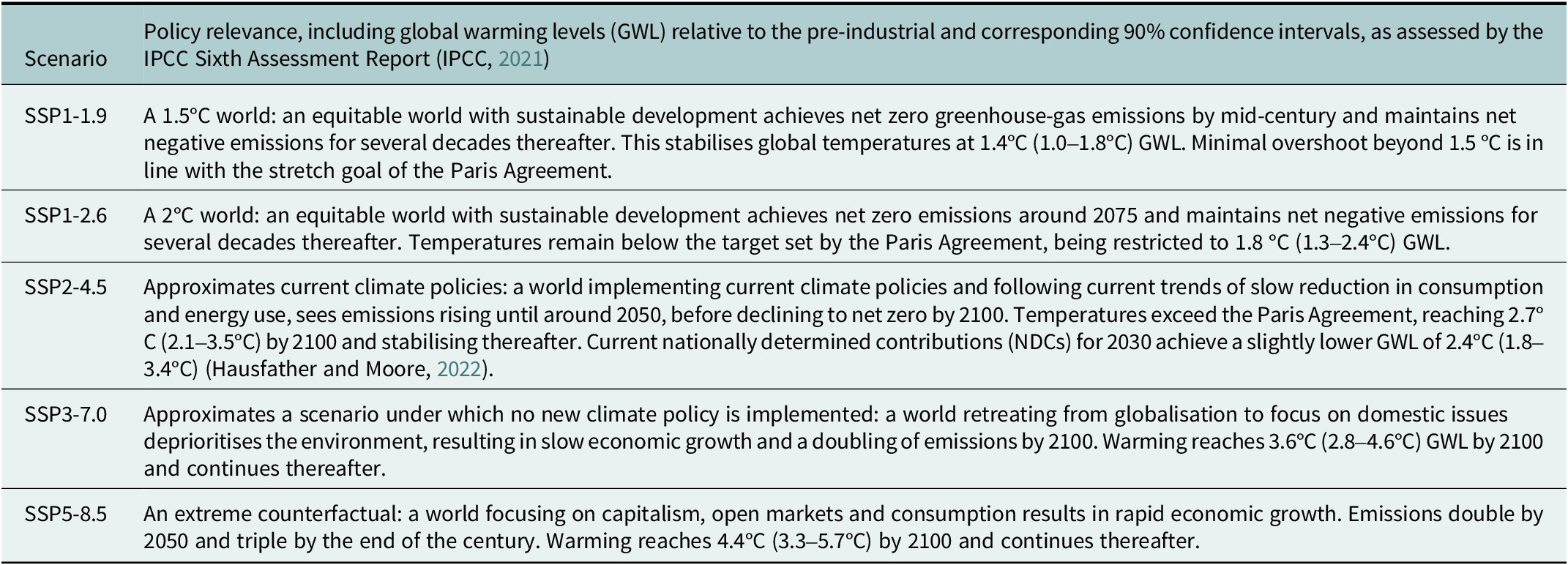
Projected future ocean conditions increase risks to ocean and coastal systems, including elevated risk of regional extirpations and global extinctions
A clear message from the IPCC Sixth Assessment Report cycle, including its Special Reports, is that risks from climate change escalate with the magnitude and duration of warming caused by greenhouse-gas emissions (Magnan et al., Reference Magnan, Pörtner, Duvat, Garschagen, Guinder, Zommers, Hoegh-Guldberg and Gattuso2021; IPCC, Reference Lee and Romero2023): every increment beyond 1.5°C of warming matters. Nowhere on Earth is this message more pertinent than along the world’s coasts, where warming is faster than for the global ocean as a whole (Figure 2; Lima and Wethey, Reference Lima and Wethey2012; Varela et al., Reference Varela, de Castro, Dias and Gómez-Gesteira2023). The thermal inertia of the ocean, together with the acknowledgement that sea levels will continue to rise for centuries and remain elevated for millennia (Fox-Kemper et al., Reference Fox-Kemper, Hewitt, Xiao, Aðalgeirsdóttir, Drijfhout, Edwards, Golledge, Hemer, Kopp, Krinner, Mix, Notz, Nowicki, Nurhati, Ruiz, Sallée, ABA, Yu, Masson-Delmotte, Zhai, Pirani, Connors, Péan, Berger, Caud, Chen, Goldfarb, Gomis, Huang, Leitzell, Lonnoy, JBR, Maycock, Waterfield, Yelekçl, Yu and Zhou2021), means that there are few easy ways to reduce risks of climate change to coastal ecosystems over the remainder of this century. But rapidly reducing emissions, alleviating non-climate anthropogenic stressors, and attempting to restore damaged habitats will provide more operating room for the full adaptation toolkit. This includes revising institutions related to ocean and coastal activities and users, developing new technologies and innovative built infrastructure, and employing marine and coastal nature-based solutions while also carefully managing existing and future coastal development (Duarte et al., Reference Duarte, Agusti, Barbier, Britten, Castilla, Gattuso and Worm2020; Frazão Santos et al., Reference Santos, Agardy, Andrade, Calado, Crowder, Ehler, García-Morales, Gissi, Halpern, Orbach, H-O and Rosa2020; Cooley et al., Reference Cooley, Schoeman, Bopp, Boyd, Donner, Ghebrehiwet, Ito, Kiessling, Martinetto, Ojea, Racault, Rost, Skern-Mauritzen, Pörtner, Roberts, Tignor, Poloczanska, Mintenbeck, Alegría, Craig, Langsdorf, Löschke, Möller, Okem and Rama2022; Shin et al., Reference Shin, Midgley, Archer, Arneth, Barnes, Chan, Hashimoto, Hoegh‐Guldberg, Insarov, Leadley, Levin, Ngo, Pandit, Pires, Pörtner, Rogers, Scholes, Settele and Smith2022; van Woesik et al., Reference Woesik, Shlesinger, Grottoli, Toonen, Thurber, Warner, Hulver, Chapron, McLachlan, Albright, Crandall, DeCarlo, Donovan, Eirin‐Lopez, Harrison, Heron, Huang, Humanes, Krueger, Madin, Manzello, McManus, Matz, Muller, Rodriguez‐Lanetty, Vega‐Rodriguez, Voolstra and Zaneveld2022; Pörtner et al., Reference Pörtner, Scholes, Arneth, Barnes, Burrows, Diamond, Duarte, Kiessling, Leadley, Managi, McElwee, Midgley, Ngo, Obura, Pascual, Sankaran, Shin and Val2023; Rossbach et al., Reference Rossbach, Steckbauer, Klein, Arossa, Geraldi, Lim, Martin, Rossbach, Shellard, Valluzzi and Duarte2023).
Given this reality, together with the assessment that anthropogenic climate change has already exposed coastal ecosystems to conditions unprecedented over millennia, it can be projected with some confidence that the impacts already caused by climate change will become worse and more extensive (Cooley et al., Reference Cooley, Schoeman, Bopp, Boyd, Donner, Ghebrehiwet, Ito, Kiessling, Martinetto, Ojea, Racault, Rost, Skern-Mauritzen, Pörtner, Roberts, Tignor, Poloczanska, Mintenbeck, Alegría, Craig, Langsdorf, Löschke, Möller, Okem and Rama2022). Here, we present a selection of the most robust projections of future risk, focusing on habitat-forming species due to their importance in ecological structure and functioning.
Projected climate risks for habitat-forming coastal taxa
Evidence from the palaeorecord and from species-environment relationships suggests that ocean warming and acidification will result in declining coral reef extent and species richness (Pandolfi et al., Reference Pandolfi, Connolly, Marshall and Cohen2011; Pandolfi and Kiessling, Reference Pandolfi and Kiessling2014; Hoegh-Guldberg et al., Reference Hoegh-Guldberg, Jacob, Taylor, Bindi, Brown, Camilloni, Diedhiou, Djalante, Ebi, Engelbrecht, Guiot, Hijioka, Mehrotra, Payne, Seneviratne, Thomas, Warren, Zhou, Masson-Delmotte, Zhai, Pörtner, Roberts, Skea, Shukla, Pirani, Moufouma-Okia, Péan, Pidcock, Connors, Matthews, Chen, Zhou, Gomis, Lonnoy, Maycock, Tignor and Waterfield2018a; Hoegh-Guldberg et al., Reference Hoegh-Guldberg, Pendleton and Kaup2019; van der Zande et al., Reference van der Zande, Achlatis, Bender-Champ, Kubicek, Dove and Hoegh-Guldberg2020; Chaudhary et al., Reference Chaudhary, Alfaro-Lucas, Simões, Brandt and Saeedi2023). Recent assessments that exclude consideration of natural adaptive capacity project declines in reef extent by >70% at 1.5°C of warming, and by >99% at 2°C (Hoegh-Guldberg et al., Reference Hoegh-Guldberg, Kennedy, Beyer, McClennen and Possingham2018b; Kalmus et al., Reference Kalmus, Ekanayaka, Kang, Baird and Gierach2022). Considering return times of severe bleaching-level heat events provides an even more pessimistic outlook (Kalmus et al., Reference Kalmus, Ekanayaka, Kang, Baird and Gierach2022). However, on the basis of results from a coral-symbiont eco-evolutionary model, Logan et al. (Reference Logan, Dunne, Ryan, Baskett and Donner2021) concluded that natural adaptation – including evolution and other processes – could allow 70–80% of coral to survive the century with ~2°C of warming, but with warming beyond 3.5°C by 2100, <10% of coral cover would remain. The relatively high levels of trait heritability among corals (Bairos-Novak et al., Reference Bairos-Novak, Hoogenboom, van Oppen and Connolly2021) further emphasise the potential for future adaptation. However, limits remain, and resilience is spatially variable, even when accounting for adaptive capacity (Cornwall et al., Reference Cornwall, Comeau, Donner, Perry, Dunne, Hooidonk, Ryan and Logan2023).
Kelps and seagrasses are also at risk from future warming (Cooley et al., Reference Cooley, Schoeman, Bopp, Boyd, Donner, Ghebrehiwet, Ito, Kiessling, Martinetto, Ojea, Racault, Rost, Skern-Mauritzen, Pörtner, Roberts, Tignor, Poloczanska, Mintenbeck, Alegría, Craig, Langsdorf, Löschke, Möller, Okem and Rama2022). For both taxa, warming trends commonly underlie projections of extirpations at warm range edges, with poleward range extensions at cool range edges (Wilson and Lotze, Reference Wilson and Lotze2019; Assis et al., Reference Assis, Serrão, Duarte, Fragkopoulou and Krause-Jensen2022; Davis et al., Reference Davis, Champion and Coleman2022; Pecquet et al., Reference Pecquet, Mouchet, Campagne, Raybaud, Baulaz, Gevaert and Lasram2022; Daru and Rock, Reference Daru and Rock2023). However, there are exceptions to this pattern (e.g., Goldsmit et al., Reference Goldsmit, Schlegel, Filbee-Dexter, MacGregor, Johnson, Mundy, Savoie, McKindsey, Howland and Archambault2021), especially for invasive seagrasses (Wesselmann et al., Reference Wesselmann, Chefaoui, Marbà, Serrao and Duarte2021). Some species might even find refuge from warming at depth and boost their overall biomass due to increasing productivity in the cooler parts of their ranges (Davis et al., Reference Davis, Champion and Coleman2022). The potential effects of projected changes in marine heatwaves remain qualitative (e.g., Starko et al., Reference Starko, Neufeld, Gendall, Timmer, Campbell, Yakimishyn, Druehl and Baum2022), although progress is being made towards more quantitative projections (e.g., Pruckner et al., Reference Pruckner, Bedford, Murphy, Turner and Mills2022; Li and Donner, Reference Li and Donner2023).
Other climate-sensitive coastal taxa that contribute to habitat structure include mangroves and saltmarshes. But even for the well-studied mangrove forests, quantitative projections are challenged by difficulties in disentangling the impacts of recent climate change from those of other non-climate anthropogenic stressors, and by the mix of positive (e.g., through enhanced productivity due to CO2 enrichment and warming) and negative (e.g., due to sea-level rise and drought) effects of projected climate change (Friess et al., Reference Friess, Adame, Adams and Lovelock2022). Further complications are introduced by uncertainties surrounding future trajectories of socioeconomic development in the coastal zone, which can have effects on projected gains or losses of coastal wetland habitat that at least equal those of climate change (Ouyang et al., Reference Ouyang, Connolly and Lee2022; Liang et al., Reference Liang, Hu, Liu, Su, Chen, Chen, Xie, Du, Liu and Chen2023). Central to the future of these systems is the availability of accommodation space, which regulates their ability to accrete sediment and move inland in response to sea-level rise (Krauss, Reference Krauss2021; Rogers, Reference Rogers2021). In this sense, coastal development that restricts accommodation space and traps wetlands in a coastal squeeze is arguably the largest threat to their resilience (Cooley et al., Reference Cooley, Schoeman, Bopp, Boyd, Donner, Ghebrehiwet, Ito, Kiessling, Martinetto, Ojea, Racault, Rost, Skern-Mauritzen, Pörtner, Roberts, Tignor, Poloczanska, Mintenbeck, Alegría, Craig, Langsdorf, Löschke, Möller, Okem and Rama2022). Irrespective, analysis of reconstructed palaeorecords suggests projected rates of sea-level rise (Table 1) will overwhelm the ability of mangroves to keep pace with rising water levels by mid-century, even with ambitious mitigation of greenhouse-gas emissions, and that saltmarshes face the same fate by the end of the century (Horton et al., Reference Horton, Shennan, Bradley, Cahill, Kirwan, Kopp and Shaw2018; Saintilan et al., Reference Saintilan, Khan, Ashe, Kelleway, Rogers, Woodroffe and Horton2020; Törnqvist et al., Reference Törnqvist, Jankowski, Li and González2020). Although the vulnerability of coastal wetlands decreases with increasing sediment availability, greater elevation on the shore, and increasing tidal range (Schuerch et al., Reference Schuerch, Spencer, Temmerman, Kirwan, Wolff, Lincke, McOwen, Pickering, Reef, Vafeidis, Hinkel, Nicholls and Brown2018; Saintilan et al., Reference Saintilan, Khan, Ashe, Kelleway, Rogers, Woodroffe and Horton2020; Friess et al., Reference Friess, Adame, Adams and Lovelock2022), efforts at restoration and protection appear to be the key to the future resilience of these systems. Quantitative projections remain contentious for other coastal systems, such as sandy beaches (e.g., Cooper et al., Reference Cooper, Masselink, Coco, Short, Castelle, Rogers, Anthony, Green, Kelley, Pilkey and Jackson2020; Vousdoukas et al., Reference Vousdoukas, Ranasinghe, Mentaschi, Plomaritis, Athanasiou, Luijendijk and Feyen2020a; Vousdoukas et al., Reference Vousdoukas, Ranasinghe, Mentaschi, Plomaritis, Athanasiou, Luijendijk and Feyen2020b).
Broader projections of climate risks for marine taxa and regions
When considering quantitative projections of processes such as range shifts (e.g., García Molinos et al., Reference Molinos, Halpern, Schoeman, Brown, Kiessling, Moore, Pandolfi, Poloczanska, Richardson and Burrows2016; Gokturk et al., Reference Gokturk, Bartlett, Erisman, Heyman and Asch2022) and phenology (e.g., Asch et al., Reference Asch, Stock and Sarmiento2019; Gokturk et al., Reference Gokturk, Bartlett, Erisman, Heyman and Asch2022; Yamaguchi et al., Reference Yamaguchi, Rodgers, Timmermann, Stein, Schlunegger, Bianchi, Dunne and Slater2022), analyses are often either taxon-specific or global, and not focused specifically on coastal ecosystems. The same is true for quantitative projections of future risk. Yet results are instructive for coastal ecosystems. For example, Trisos et al. (Reference Trisos, Merow and Pigot2020) project not only that temperatures across entire species’ ranges will transition to levels unprecedented in those species’ recent (1850–2005) experience, but that this will occur abruptly, especially for marine species such as seagrasses, corals, cephalopods, marine reptiles and marine mammals. This phenomenon is projected to manifest in tropical oceans before 2030 under the highest emissions scenarios and escalate with duration and the magnitude of emissions. Pigot et al. (Reference Pigot, Merow, Wilson and Trisos2023) confirm this trend towards abrupt thermal exposure across species’ ranges. Further evidence comes from a recent analysis of future climate risk across ~25,000 marine species (Boyce et al., Reference Boyce, Tittensor, Garilao, Henson, Kaschner, Kesner-Reyes, Pigot, RodolfoReyes, Gabriel Reygondeau, Schleit, Shackell, Sorongon-Yap and Worm2022), which found that by the end of the century, risk was substantially reduced for ~1.8°C relative to ~4.4°C of global warming, with 1.3% vs 2.7% of assessed species being at critical risk and 54% vs 84% at high risk, respectively. Since many of the taxa in these analyses occur primarily in coastal waters, these generic risks may be assumed to hold there, too.
Moving beyond projections of climate risk for individual taxa becomes more difficult, but climate analogues can help. For example, on the basis of data from the palaeorecord, Reddin et al. (Reference Reddin, Aberhan, Raja and Kocsis2022) project that if warming levels approach those anticipated under the highest emissions scenarios (Table 3), taxa with thermal optima beyond ~21°C will experience elevated risk of extinction, as will those with thermal optima below ~11°C. This pattern is mirrored by projections from an ecophysiological model validated on spatial patterns of extinction from the fossil record, which projects extirpations at the tropics and extinctions at the poles, but with substantial reductions in risk from immediate and strong mitigation (Penn and Deutsch, Reference Penn and Deutsch2022). Again, however, these are generic projections, and coastal taxa must be assumed to comply with reported patterns.
The emergence of ecosystem and global models for projecting of climate risks in the ocean
The growing need for policy advice in the face of these projected climate-change risks has challenged the scientific community to develop models that go beyond exploring risks to biodiversity, such as those discussed above, to instead assess risks to ecosystem functioning and service provision (Weiskopf et al., Reference Weiskopf, Myers, Arce-Plata, Blanchard, Ferrier, Fulton, Harfoot, Isbell, Johnson, Mori, Weng, HarmáČková, Londoño-Murcia, Miller, Pereira and Rosa2022). This requires modellers to build from familiar outputs of Earth System models (ESMs), such as changes in temperature, pH and salinity, and, more recently, nutrient availability, phytoplankton and even zooplankton biomass (Canadell et al., Reference Canadell, Monteiro, Costa, Cotrim da Cunha, Cox, Eliseev, Henson, Ishii, Jaccard, Koven, Lohila, Patra, Piao, Rogelj, Syampungani, Zaehle, Zickfeld, Masson-Delmotte, Zhai, Pirani, Connors, Péan, Berger, Caud, Chen, Goldfarb, Gomis, Huang, Leitzell, Lonnoy, JBR, Maycock, Waterfield, Yelekçl, Yu and Zhou2021), to project changes in primary and secondary production.
One response has been the development of the Fisheries and Marine Ecosystem Model Intercomparison Project (FishMIP), which specifies sets of common ESM forcings and model outputs for a diverse suite of marine fisheries and ecosystem models (Tittensor et al., Reference Tittensor, Eddy, Lotze, Galbraith, Cheung, Barange, Blanchard, Bopp, Bryndum-Buchholz, Büchner, Bulman, Carozza, Christensen, Coll, Dunne, Fernandes, Fulton, Hobday, Huber, Jennings, Jones, Lehodey, Link, Mackinson, Maury, Niiranen, Oliveros-Ramos, Roy, Schewe, Shin, Silva, Stock, Steenbeek, Underwood, Volkholz, Watson and Walker2018). The advantage of the “modelling intercomparison” approach is that it yields comparable projections from each model, allowing assessment of the range of plausible outcomes, given our current understanding and computing capacity (Heymans et al., Reference Heymans, Bundy, Christensen, Coll, de Mutsert, Fulton, Piroddi, Shin, Steenbeek and Travers-Trolet2020). This is important because marine ecosystem models still largely lack formal approaches to validation, calibration, and quantification of uncertainty (Steenbeek et al., Reference Steenbeek, Buszowski, Chagaris, Christensen, Coll, Fulton, Katsanevakis, Lewis, Mazaris, Macias, de, Oldford, Pennino, Piroddi, Romagnoni, Serpetti, Shin, Spence and Stelzenmüller2021).
In terms of uncertainty, scenario uncertainty can be assessed by comparing model outputs generated under different future scenarios, such as those in Table 3. But parametric uncertainty – the uncertainty around the parameters within individual models – is far more difficult to address for models with any level of complexity, given current computational capacity (Steenbeek et al., Reference Steenbeek, Buszowski, Chagaris, Christensen, Coll, Fulton, Katsanevakis, Lewis, Mazaris, Macias, de, Oldford, Pennino, Piroddi, Romagnoni, Serpetti, Shin, Spence and Stelzenmüller2021). Finally, intercomparison can help to quantify structural – inter-model – uncertainty, but attempts to do so reveal that the projected responses to two of the most fundamental inputs to the models – magnitude of warming and productivity of lower trophic levels – are inconsistent in both direction and magnitude amongst models (Heneghan et al., Reference Heneghan, Galbraith, Blanchard, Harrison, Barrier, Bulman, Cheung, Coll, Eddy, Erauskin-Extramiana, Everett, Fernandes-Salvador, Gascuel, Guiet, Maury, Palacios-Abrantes, Petrik, du Pontavice, Richardson, Steenbeek, Tai, Volkholz, Woodworth-Jefcoats and Tittensor2021). This disparity emphasises that understanding of how ecosystem-level effects emerge from individual-level processes remains incomplete. Despite limitations in individual ecosystem models, ensembles of models – such as those in FishMIP – provide projections of global decline in total biomass of marine animals that worsen with increasing emissions (Tittensor et al., Reference Tittensor, Novaglio, Harrison, Heneghan, Barrier, Bianchi, Bopp, Bryndum-Buchholz, Britten, Büchner, Cheung, Christensen, Coll, Dunne, Eddy, Everett, Fernandes-Salvador, Fulton, Galbraith, Gascuel, Guiet, John, Link, Lotze, Maury, Ortega-Cisneros, Palacios-Abrantes, Petrik, du Pontavice, Rault, Richardson, Shannon, Shin, Steenbeek, Stock and Blanchard2021), with reasonable agreement in the direction of change in coastal systems, but little agreement on the magnitude of change (Cooley et al., Reference Cooley, Schoeman, Bopp, Boyd, Donner, Ghebrehiwet, Ito, Kiessling, Martinetto, Ojea, Racault, Rost, Skern-Mauritzen, Pörtner, Roberts, Tignor, Poloczanska, Mintenbeck, Alegría, Craig, Langsdorf, Löschke, Möller, Okem and Rama2022).
A view of the way ahead
Despite the recent advances in our understanding of the impacts of anthropogenic climate change on coastal ecosystems described in Cooley et al. (Reference Cooley, Schoeman, Bopp, Boyd, Donner, Ghebrehiwet, Ito, Kiessling, Martinetto, Ojea, Racault, Rost, Skern-Mauritzen, Pörtner, Roberts, Tignor, Poloczanska, Mintenbeck, Alegría, Craig, Langsdorf, Löschke, Möller, Okem and Rama2022) and updated above, projections of future climate risk have not progressed much beyond the notion that risks escalate with cumulative emissions (i.e., with warming). Part of the problem is that our detailed understanding of processes at the single-organism level seldom adequately addresses interactions among multiple drivers, so does not scale intuitively to predict integrated responses at the levels of populations or ecosystems (Boyd et al., Reference Boyd, Collins, Dupont, Fabricius, Gattuso, Havenhand, Hutchins, Riebesell, Rintoul, Vichi, Biswas, Ciotti, Gao, Gehlen, Hurd, Kurihara, McGraw, Navarro, Nilsson, Passow and Pörtner2018; Collins et al., Reference Collins, Whittaker and Thomas2022). Theoretical progress is being made in this regard (Orr et al., Reference Orr, Vinebrooke, Jackson, Kroeker, Kordas, Mantyka-Pringle, den, Laender, Stoks, Holmstrup, Matthaei, Monk, Penk, Leuzinger, Schäfer and Piggott2020; Pirotta et al., Reference Pirotta, Thomas, Costa, Hall, Harris, Harwood, Kraus, Miller, Moore, Photopoulou, Rolland, Schwacke, Simmons, Southall and Tyack2022), but proposed solutions are not yet commonly implemented. This problem is exacerbated for coastal ecosystems by the interactions among numerous climate-induced and non-climate anthropogenic drivers (Table 2; Gissi et al., Reference Gissi, Manea, Mazaris, Fraschetti, Almpanidou, Bevilacqua, Coll, Guarnieri, Lloret-Lloret, Pascual, Petza, Rilov, Schonwald, Stelzenmüller and Katsanevakis2021). Not only do these interactions complicate the parameterisation of models, but they also complicate the scenario space that must be explored: what humans do in the coastal zone can often have a larger ecological effect than that of climate change. For example, under ambitious mitigation, conservation and coastal-zone planning can ameliorate climate impacts on coastal ecosystems, but climate-uninformed coastal development can condemn those same ecosystems (Cooley et al., Reference Cooley, Schoeman, Bopp, Boyd, Donner, Ghebrehiwet, Ito, Kiessling, Martinetto, Ojea, Racault, Rost, Skern-Mauritzen, Pörtner, Roberts, Tignor, Poloczanska, Mintenbeck, Alegría, Craig, Langsdorf, Löschke, Möller, Okem and Rama2022). The utility of advice to policymakers therefore requires more purposeful selection and articulation of scenarios to be considered – simply making projections for SSP1-2.6 and SSP5-8.5 cannot remain the norm. In this context, one solution might be to consider warming levels (e.g., 1.5°C, 2°C and 3°C relative to pre-industrial), each in combination with alternative coastal development scenarios. This is impractical with current CMIP6 model outputs because each scenario subsumes a shared socioeconomic pathway (Table 3), but the increasingly prominent use of climate-model emulators (Nicholls et al., Reference Nicholls, Meinshausen, Lewis, Gieseke, Dommenget, Dorheim, Fan, Fuglestvedt, Gasser, Golüke, Goodwin, Hartin, Hope, Kriegler, Leach, Marchegiani, McBride, Quilcaille, Rogelj, Salawitch, Samset, Sandstad, Shiklomanov, Skeie, Smith, Smith, Tanaka, Tsutsui and Xie2020; IPCC, Reference Masson-Delmotte, Zhai, Pirani, Connors, Péan, Berger, Caud, Chen, Goldfarb, Gomis, Huang, Leitzell, Lonnoy, Matthews, Maycock, Waterfield, Yelekçl, Yu and Zhou2021) might provide a solution.
There are several other areas where progress is urgently needed. But one requires particular attention because we have not addressed it elsewhere in this review: the need to expand the knowledge systems on which our assessments depend by ensuring that Indigenous Knowledge and perspectives are more adequately represented in our assessments (Fischer et al., Reference Fischer, Maxwell, Nuunoq, Pedersen, Greeno, Jingwas, Blair, Hugu, Mustonen, Murtomäki and Mustonen2022; Schipper et al., Reference Schipper, Revi, Preston, Carr, Eriksen, Fernandez-Carril, Glavovic, Hilmi, Ley, Mukerji, Muylaert de Araujo, Perez, Rose, Singh, Pörtner, Roberts, Tignor, Poloczanska, Mintenbeck, Alegría, Craig, Langsdorf, Löschke, Möller, Okem and Rama2022). Silent cores of sediment and coral have taught us so much about the past and the future; how much more could we learn from the rich oral histories held by Indigenous Peoples around the world?
Open peer review
To view the open peer review materials for this article, please visit http://doi.org/10.1017/cft.2023.27.
Acknowledgements
We acknowledge the contributions of Laurent Bopp, Philip Boyd, Simon Donner, Shin-Ichi Ito, Wolfgang Kiessling, Paulina Martinetto, Elena Ojea, Marie-Fanny Racault, Björn Rost, Mette Skern-Mauritzen, Dawit Yemane Ghebrehiwet, Lisa Levin and Karim Hilmi, who were Lead Authors or Review Editors on Chapter 3 of the IPCC Sixth Assessment Report: Oceans and Coastal Ecosystems and Their Services. Without their work, neither that assessment nor this paper could have been completed. We also acknowledge the many Contributing Authors and Expert Reviewers for the IPCC Chapter, especially Olivier Torres, who provided the data for Figure 2.
Author contribution
All authors contributed to all aspects of the development of this manuscript.
Financial support
D.S.S. was funded by Australian Research Council Discovery Project DP230102359. J.A.B. was funded by an Australian Government Research Training Program Scholarship and a Commonwealth Scientific and Industrial Research Organisation ResearchPlus Scholarship.
Competing interest
The authors declare no competing interests exist.
Comments
Please find enclosed the files comprising our submission for our invited Cambridge Prisms review. We found the subject matter more challenging to condense than we had anticipated, despite our attempts to remain high-level and focus on key advances in knowledge since the recent IPCC Report. We have therefore exceeded the word limit in this initial submission but hope that you will allow us the leeway. We have provided the names of several potential candidate referees, who are all subject specialists. We hope that these prove useful.
We have attempted to match Cambridge Prisms requirements as closely as possible, but if there are any problems, these should be easy to resolve, so please contact me directly by email, should this be the case.
Thank you for your support so far and for your ongoing consideration.
Sincerely,
David Schoeman (on behalf of the authorship team)