CVD are responsible for 26 % of deaths in the UK, and healthcare costs related to CVD represent a £9 billion economic burden annually(1). In parallel, metabolic disorders such as type 2 diabetes (T2D), metabolic syndrome and non-alcoholic fatty liver disease are associated with increased risk of CVD in epidemiological studies(Reference Huxley, Barzi and Woodward2–Reference Lonardo, Ballestri and Guaraldi4). With 7 million people living with CVD and 4·6 million with T2D in the UK, prevention of these cardiometabolic diseases (CMD) is currently one of the biggest modern challenges for public health(1,5) . Whilst some CMD risk factors are not modifiable (e.g. age, sex or genetic makeup), modifying dietary behaviours may constitute an effective strategy for disease risk reduction. In particular, dietary intakes of saturated fat, Na and fruits and vegetables have been extensively studied in relation to CMD prevention and have been targeted by public health recommendations(6). In the UK, the first recommendation on dietary SFA (< 10 % total energy) intake was initially implemented by the National Advisory Committee on Nutritional Education (NACNE) in 1983(7,Reference Walker8) and was reiterated in the 2019 Scientific Advisory Committee in Nutrition report on saturated fat and health which recommended a replacement of SFA with poly-PUFA or MUFA for CVD prevention(9). Furthermore, recent epidemiological evidence suggested the potential different associations of individual SFA on CMD risk, which might reflect their different chain lengths or food matrices in which they are incorporated(Reference Forouhi, Koulman and Sharp10,Reference Khaw, Friesen and Riboli11) .
From a food perspective, dairy products are an interesting food group for the management of SFA entry in the food chain. Dairy products contribute up to 21 % of total SFA intake among British adults, and their consumption (particularly cheese and yogurt) seems to have beneficial associations with some risk markers for CMD such as blood pressure and arterial stiffness(12,Reference Lovegrove and Hobbs13) . However, most of the currently available evidence on the consumption of dairy foods and/or their fat content and CMD risk is limited to observational studies which often rely on imprecise methods of dietary assessment. Indeed, the reported intakes from FFQ, diet-diaries or dietary recalls may be subject to underestimation, recall bias and systematic errors from food composition tables, which may impact on the associations between dairy intakes and CMD risk in prospective cohort studies. However, validation studies of FFQ against dietary records or blood and urinary biomarkers, the use of repeated assessments of dietary intakes in longitudinal studies and the integration of novel technological tools have contributed to improving the accuracy of these traditional methods of dietary assessment(Reference Kroke, Klipstein-Grobusch and Voss14,Reference Naska, Lagiou and Lagiou15) . Limited precision when assessing dairy foods consumed within composite dishes (e.g. pizza, bakery items or coffee drinks), which might independently impact CMD risk, is a further shortcoming of these dietary assessment methods that may be overcome by deconstructing dairy-derived ingredients from composite dishes and re-allocating them to dairy food categories, as illustrated in the Prospective Urban Rural Epidemiology (PURE) study(Reference Dehghan, Mente and Rangarajan16). Whilst these methodological limitations are not restricted to dairy fat intakes and are commonplace in nutritional science, the integration of data from traditional methods of dietary assessment with more objective biomarkers of intake may improve the accuracy of dietary intake assessments and prediction of disease risk. In particular, reliable circulating biomarker candidates need to be (1) specific to the food source of interest; (2) easy to quantify in the organism; (3) exclusively derived from dietary intake rather than endogenous synthesis and (4) highly correlated to dietary intakes. For example, blood concentrations of vitamin C and carotenoids have been widely used as a proxy for fruit and vegetable intakes and linked with decreased risks of cancer in observational studies(Reference Jenab, Slimani and Bictash17). Therefore, the identification of biomarkers for the consumption of other food groups, such as fats derived from dairy foods, might provide useful insights into their role in cardiometabolic health by complementing the extensive evidence base already available(Reference Lovegrove, Hodson and Sharma18).
The following article will thus aim to review the evidence for a range of FA present in milk and dairy foods as biomarker candidates for dairy fat intake: odd-chain SFA (C15:0 and C17:0), which are traditionally used in nutritional epidemiology, and more novel biomarkers such as ruminant trans-FA (trans-palmitoleic acid C16:1 trans-9 and vaccenic acid C18:1 trans-11) and one branched-chain FA (phytanic acid). The physiological role of those FA in the context of cardiometabolic health and possible mechanisms of action will also be discussed.
Identification of individual fatty acids as biomarkers of dairy fat intake
Odd-chain fatty acids: pentadecanoic (C15:0) and heptadecanoic (C17:0) acids
Presence in dairy milk and other food group
In dairy cows and other ruminants, odd-chain FA are produced by bacteria in the rumen and by post-absorptive de novo lipogenesis using short-chain FA like propionic acid (C3:0) as a substrate, or by α-oxidation which converts C16:0 or C18:0 into C15:0 or C17:0, respectively via the elimination of the α-carbon(Reference French, Bertics and Armentano19–Reference Jansen and Wanders21) (Fig. 1). The produced odd-chain FA are then utilised in the mammary gland for the production of milk fat, although their contribution remains minor in comparison to even-chain FA which are produced via de novo lipogenesis at much higher levels(Reference Fulco22). Although there are small seasonal variations, SFA account for 67–72 % of total FA in milk and include mostly palmitic (C16:0, 30–33 % total FA), myristic (C14:0, 10–11 % total FA), stearic (C18:0, 9–10 % total FA) acids and short/medium-chain FA (C4:0–C12:0, < 4 % total FA), while odd-chain FA such as pentadecanoic (C15:0) and heptadecanoic (C17:0) represent 1 % and 0·5 % total FA, respectively(Reference Kliem, Shingfield and Livingstone23). Thus, one serving of 200 ml of semi-skimmed milk would provide 2 g SFA, of which 20 mg were from C15:0 and 10 mg from C17:0.
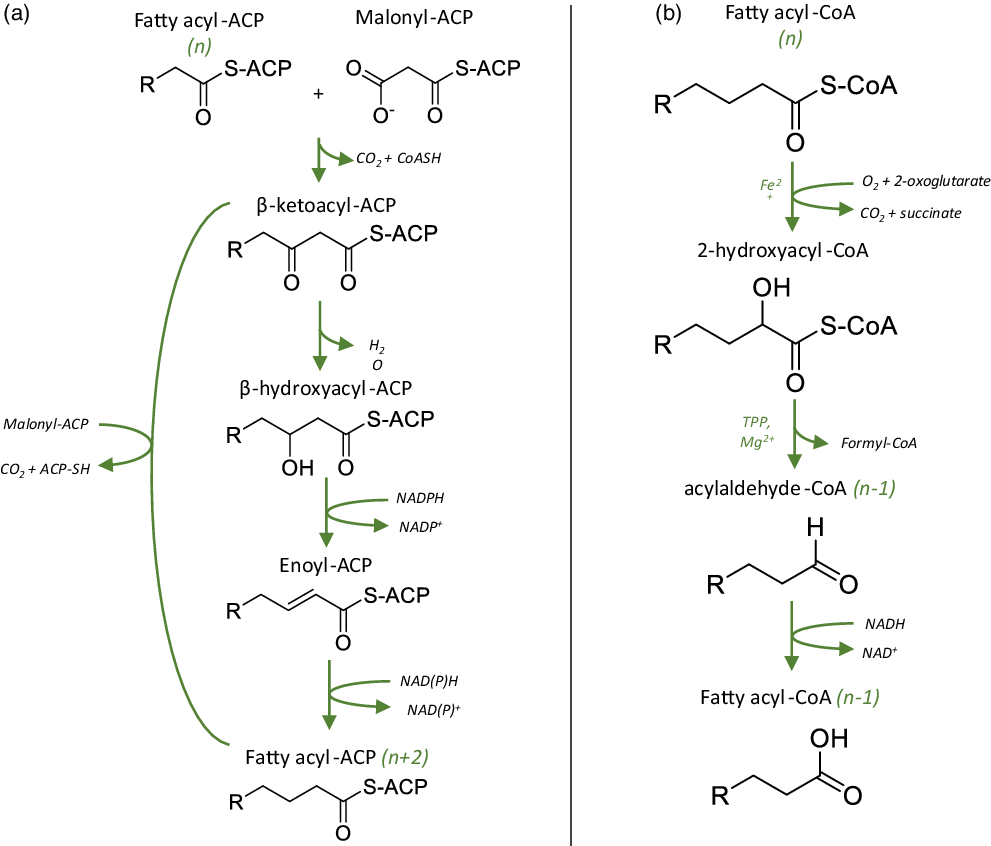
Fig. 1. Possible mechanisms for the synthesis of odd-chain SFA. Adapted from Jansen (2006) and Jenkins (2015)(Reference Jansen and Wanders21,Reference Jenkins, West and Koulman32) . (a) Metabolic pathway for the synthesis and elongation of even-chain FA (e.g. 16:0, 18:0), starting with the condensation of malonyl-CoA with a fatty acyl-ACP of n carbons. Odd-chain SFA may be produced via the same route, using propionyl-CoA as a precursor instead of malonyl-CoA. (b) Main steps of the α-oxidation of odd-chain fatty acids, involving the decarboxylation of the α-carbon to allow further oxidation of the acyl chain. Even-chain SFA may undergo the same decarboxylation reaction, leading to the formation of odd-chain SFA.
Non-dairy dietary sources of C15:0 and C17:0 include ruminant meats and fish, although the proportions of C15:0 from these sources are lower than of C17:0(Reference Risérus and Marklund24). With meat and meat products contributing to as much dietary SFA as dairy foods in British adults (about 21 % of total dietary SFA)(12), the sole use of odd-chain SFA as biomarkers of dairy fat consumption may be questionable especially in the context of diets rich in ruminant meat. However, very limited evidence from the European Prospective Investigation into Cancer and Nutrition-Potsdam(Reference Prada, Wittenbecher and Eichelmann25) and European Prospective Investigation into Cancer and Nutrition-E3N(Reference Thiébaut, Rotival and Gauthier26) prospective studies has suggested that circulating C15:0 or C17:0 and red meat consumption might be inversely correlated or not correlated, respectively. Moreover, the analysis of the FA content of twenty-seven freshwater fish species revealed that C15:0 and C17:0 represented 0·4 % and 0·6 % total FA, respectively(Reference Wang, Jackson and Twining27), which was also reflected in the analysis of nineteen different European brands of fish oil supplements(Reference Kolanowski28). Thus, further concerns could be raised on the validity of odd-chain SFA as biomarkers of dairy fats in populations consuming high-fish, low-dairy diets. This is not the case for most Western populations, including British adults, among which fish consumption is relatively low (22 g/d on average or 3 % total energy for British adults aged between 19 and 64 years old)(12), but does not account for the intake of fish oil supplements consumed by an estimated 11 % of British adults in 2012(29).
Correlations between circulating levels and dietary intakes
Despite their low contribution to total dietary SFA, odd-chain SFA are detectable in low concentrations in human plasma samples but were traditionally used as internal standards in GC analytical methods which masked any possible quantitative assessment(Reference Firl, Kienberger and Hauser30,Reference Burdge, Wright and Jones31) . However, with the growing interest in their utility as biomarkers of dairy fat intake, circulating levels are more routinely measured in a number of plasma FA fractions, such as non-esterified FA or phospholipids(Reference Jenkins, West and Koulman32).
To date, C15:0 and C17:0 have formed the basis for much of the research on biomarkers of dairy fat and specific dairy food group consumption. In particular, findings from two cross-sectional studies indicated a better correlation between circulating C15:0 and high-fat dairy products or total dairy fat compared with lower fat dairy product foods(Reference Smedman, Gustafsson and Berglund33,Reference Albani, Celis-Morales and O’Donovan34) . In a cross-sectional study of n seventy-two participants, C15:0 in plasma phospholipids was positively correlated with fat from both milk and cream (r = 0·34) and total dairy fat (r = 0·34), but not with butter, ice cream or fat from either(Reference Smedman, Gustafsson and Berglund33). More recently, findings from the Food4Me study (n 1054 participants) suggested stronger correlations between high-fat dairy food consumptions and C15:0 in dried blood spots compared with C17:0(Reference Albani, Celis-Morales and O’Donovan34). The authors reported positive associations between C15:0 (% change in blood) and consumed daily portions of total dairy (regression coefficient β = 1·02, 95 % CI (0·14, 1·91)), high-fat dairy (β = 0·32, 95 % CI (0·05, 0·58)), cheese (β = 1·77, 95 % CI (0·52, 3·02)) and butter (β = 3·34, 95 % CI (1·34, 5·35)). In contrast, C17:0 was associated with intakes of cream (β = 9·42, 95 % CI (3·43, 15·4)) and high-fat dairy albeit to a lesser extent than C15:0 (β = 0·27, 95 % CI (0·1, 0·45)), but not with total dairy, cheese or butter. Finally, the authors did not report any associations between C15:0 or C17:0 and consumption of low-fat dairy foods, milk or yogurts(Reference Albani, Celis-Morales and O’Donovan34). The discrepancy observed between C15:0 and C17:0 might be partly explained by the biological matrix used for analysis, as suggested by findings from the LifeLines Cohort study (n 864 participants) where total dairy product intakes were positively related with both C15:0 and C17:0 in plasma phospholipids, but only C15:0 in plasma TAG)(Reference Pranger, Muskiet and Kema35). Overall, findings from observational studies tend to support consistent, albeit weak, correlations between dairy product fat intakes and plasma/serum C15:0 (r = 0·33, 95 % CI (0·27, 0·39)) and to a lesser extent C17:0 (r = 0·19, 95 % CI (0·14, 0·25)), as summarised in a 2019 meta-analysis of eighteen cross-sectional and prospective studies(Reference Pranger, Joustra and Corpeleijn36). However, these results need to be interpreted in the light of their observational and cross-sectional nature, which prevent causal links between dairy fat productconsumption and circulating C15:0 or C17:0 from being inferred(Reference Wang and Cheng37).
In line with findings from observational studies, findings from RCT suggest that circulating concentrations of C15:0 are more responsive to dairy fat consumption than concentrations of C17:0(Reference Abdullah, Cyr and Lépine38–Reference O’Connor, Greffard and Leclercq40) (Table 1). However, the correlations between total dairy product intake and circulating levels of C15:0 and C17:0 are generally weak, as observed in a 12-month randomised controlled trial (RCT) in seventy-six adolescents which reported modest correlations between total dairy product consumptions (in servings/d) and C15:0 (r = 0·27) or C17:0 (r = 0·25) measured in erythrocytes(Reference Slim, Ha and Vanstone41). Importantly, the designs of the RCT included in this review suggest that most often the hypothesis being tested is whether specific FA, such as C15:0 and C17:0, are biomarkers of dairy food consumption, rather than dairy fat. As suggested by the poor or null correlations observed between C15:0 or C17:0 and intakes of low-fat dairy foods(Reference Smedman, Gustafsson and Berglund33,Reference Albani, Celis-Morales and O’Donovan34) , this approximation seems misplaced, as the circulating levels of FA would not accurately capture the consumption of dairy foods with a minimal fat content and thus would not reflect the consumption of total dairy accurately.
Table 1. Human randomised control trails investigating the correlations between dairy consumption and circulating levels of odd-chain, trans and/or branched-chain fatty acids
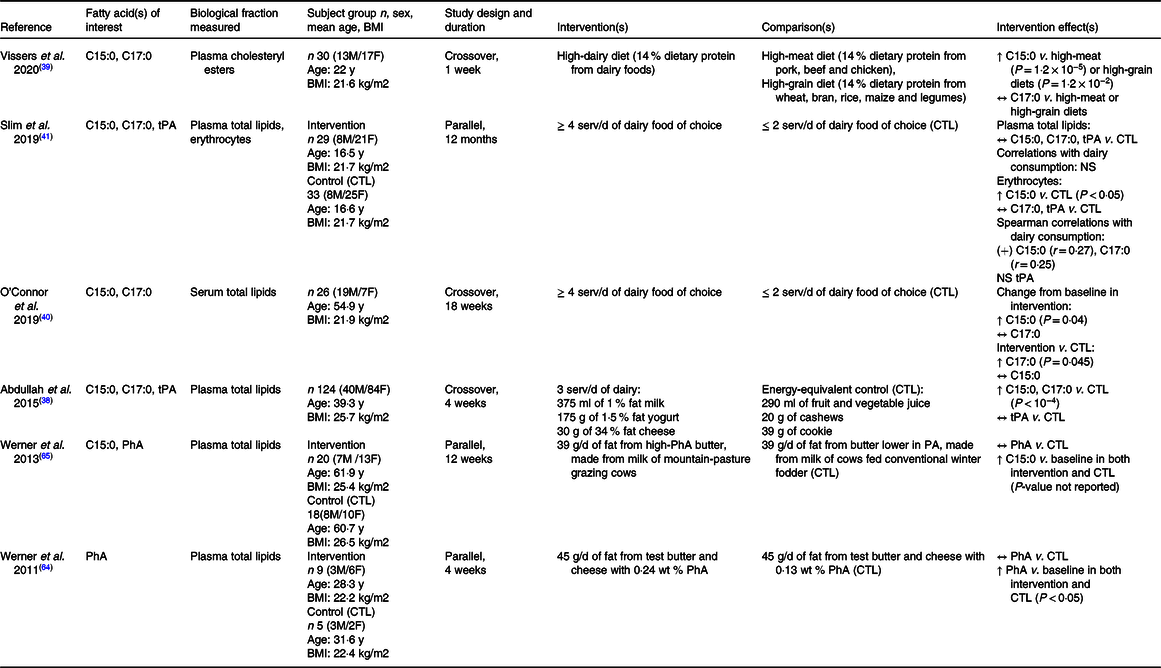
C15:0, pentadecanoic acid; C17:0, heptadecanoic acid; tPA, trans-palmitoleic acid; PhA phytanic acid; M, male; F, female; y, year; serv/d, serving per day; g/d, gram per day; % wt, weight percent; CTL, control; ↑, increase; ↔, no change; NS, not significant; (+), positive.
Potential limitations for use of C15:0 and C17:0 as biomarkers of dairy fat consumption
In phospholipid FA, levels of C15:0 and C17:0 range from 0·15 to 0·23 % (n 4 studies) and 0·33–0·41 % total phospholipid FA (n 3 studies), respectively(Reference Jenkins, West and Koulman32). Therefore, although C15:0 is more abundant in milk than C17:0, plasma phospholipid levels seem to indicate an inverse abundance of C15:0 and C17:0 in human plasma, which raises the question of possible alternative pathways for endogenous synthesis and/or oxidation of odd-chain SFA in humans. Proposed mechanisms suggest the human gut microbiota might be involved in the elongation of short-chain FA and/or decarboxylation of even-numbered very-long chain Fas(Reference Jenkins, West and Koulman32,Reference Pfeuffer and Jaudszus42) .
In vivo studies in rats suggest the need for a distinction between circulating levels of C15:0, which would be mostly of dietary origin and inversely correlated with total dietary fat, and circulating levels of C17:0, which seem unaffected by total dietary fat and may be endogenously synthesised(Reference Jenkins, Aoun and Feillet-Coudray43). This hypothesis is in line with recent in vitro findings that suggested that the main human elongation of very long-chain fatty acids (ELOVL) enzymes could not only elongate even-chain SFA but could also act on odd-chain FA by catalysing the elongation of C13:0 to C15:0 and C15:0 to C17:0(Reference Wang, Wang and Goykhman44). To date, the metabolic mechanisms related to the elongation of odd-chain SFA in humans are not fully elucidated and their contribution to circulating levels have not been precisely quantified.
In addition to potential endogenous synthesis and gut microbiome fermentation, the interactions between odd-chain SFA and other dietary nutrients such as fibre are unclear(Reference Pertiwi, Küpers and Wanders45). In particular, in a human RCT of sixteen healthy participants, the consumption of 30 g/d of inulin or 6 g/d of propionate for 7 d increased plasma phospholipid concentrations of C15:0 by 17 % and 13 % and concentrations of C17:0 by 11 % and 13 %(Reference Weitkunat, Schumann and Nickel46). The authors suggested that these results may be explained by the activation of odd-chain SFA metabolic pathways in the gut microbiota(Reference Weitkunat, Schumann and Nickel46). A potential endogenous synthesis of odd-chain SFA in response to dietary fibre and in the absence of dairy fat consumption would be in line with observations from an Austrian cross-sectional study which reported similar circulating levels of C17:0 in vegan (n 37), omnivore (n 23), semi-omnivore (n 13) and vegetarian (n 25) participants(Reference Kornsteiner, Singer and Elmadfa47).
Trans-fatty acids of ruminant origins: trans-palmitoleic acid (C16:1 trans-9) and vaccenic acid (C18:1 trans-11)
Presence in dairy milk and other food groups
In ruminant animals, dietary FA, such as oleic, linoleic or α-linolenic acids, are utilised by rumen microbiota and undergo a series of biohydrogenation reactions leading to the synthesis of trans-FA (Fig. 2)(Reference Enjalbert, Troegeler-Meynadier, Destaillats, Sébédio and Dionisi48). Trans-FA represent 4·5–6·5 % total FA in retail milk, with trans-vaccenic acid (tVA, C18:1 trans-11) being the major contributor (0·8–1·5 % total FA), followed by trans-palmitoleic acid (tPA, C16:1 trans-9) representing 0·35–0·45 % total Fas(Reference Kliem, Shingfield and Livingstone23). Thus, one serving of 200 ml semi-skimmed milk would provide low doses of tVA and tPA – up to 84 mg total trans-FA, including 27 mg tVA and 8·1 mg tPA(Reference Kliem, Shingfield and Livingstone23). Similar to odd-chain SFA, tPA and tVA are not entirely specific to dairy foods. However, they belong to the distinct category of ruminant trans-FA, as opposed to industrially processed trans-FA that derive from the partial hydrogenation of vegetable oils such as elaidic acid (C18:1 trans-9).
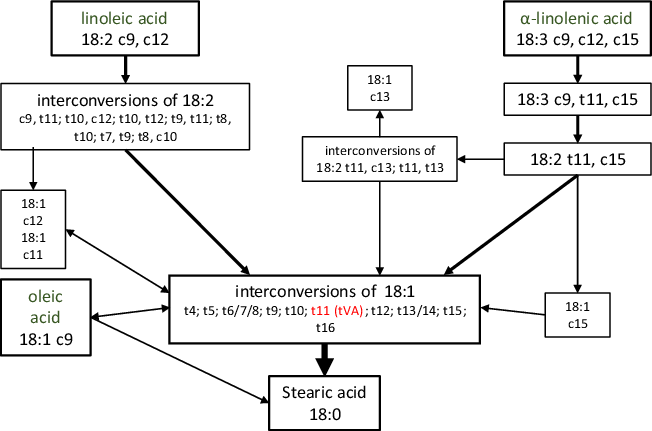
Fig. 2. Major biohydrogenation pathways of oleic, linoleic and α-linolenic acids into trans-fatty acids in ruminants. Adapted from Enjalbert (2012)(Reference Enjalbert, Troegeler-Meynadier, Destaillats, Sébédio and Dionisi48).
Correlations between circulating levels and dietary intakes
Circulating tPA measured by GC represents < 1 % total phospholipid FA (0·18 ± 0·05 % total FA)(Reference Mozaffarian, Cao and King49), although most studies only tend to report concentrations of total trans-FA or elaidic acid(Reference Imamura, Sharp and Koulman50,Reference Markey, Vasilopoulou and Kliem51) . However, one cross-sectional study of 210 healthy Canadian adults reported that tPA and tVA each represented < 0·20 % of total plasma phospholipid FA(Reference Da Silva, Julien and Couture52). Circulating tPA levels in this study were weakly correlated with dairy product intakes estimated by an FFQ (r = 0·15, P = 0·04) but tVA levels were not(Reference Da Silva, Julien and Couture52). In contrast, a more recent cross-sectional study from the Netherlands described that circulating tVA in plasma phospholipids had a stronger correlation with dairy fat consumption than odd-chain SFA(Reference Pranger, Muskiet and Kema35). Overall, very few well-controlled RCT have investigated the associations between dairy fat consumption and circulating ruminant trans-FA, and the few that have only reported results on tPA, but not tVA (Table 1).
Several epidemiological studies, which date from the 1990s, observed significant correlations between circulating levels of tPA and non-dairy fat dietary sources such as cakes, cookies and pies rather than dairy fat(Reference Mozaffarian, de Oliveira Otto and Lemaitre53,Reference Santaren, Watkins and Liese54) . At the time, such products contained hidden sources of dairy fat such as milk powder, butter and chocolate, but also partially hydrogenated oils. Since then, trans-fat has mostly been eliminated from the European and American food chains due to the significant link with increased CVD risk(55). Therefore, dietary tPA and tVA now almost entirely originate from dairy foods and ruminant fats, as described by a wide market basket analysis of French foods(Reference Guillocheau, Penhoat and Drouin56).
Potential limitations for use as biomarkers of dairy fat consumption
Findings from a human parallel RCT, which showed a significant increase in tPA circulating levels following dietary intake of tVA (2·9 g/d for 6 weeks), suggested that dietary tVA could be converted into tPA endogenously, with a conversion rate of approximately 17 %(Reference Jaudszus, Kramer and Pfeuffer57). These results were in line with in vitro studies, suggesting that tVA could be converted into tPA via peroxisomal β-oxidation pathways(Reference Guillocheau, Garcia and Drouin58). Of note, these findings were challenged in an editorial from Mozaffarian, on the basis that one of the genome-wide association studies his group led failed to reveal significant genetic determinants for the synthesis of tPA, suggesting that this endogenous synthesis would be negligible(Reference Mozaffarian59,Reference Mozaffarian, Kabagambe and Johnson60) . Overall, the limited evidence on potential endogenous synthesis of tPA and tVA raises questions about their suitability as biomarkers of dairy fat consumption.
Branched-chain fatty acids: phytanic acid
Presence in dairy milk and other food groups
Phytanic acid (PhA) is a branched-chain FA with a main chain of sixteen carbons and four methyl groups (3,7,11,15-tetramethylhexadecanoic acid). As with previous FA described in this review, it is found in relatively low quantities in milk (200 ml semi-skimmed milk provides approximately 9·8 mg of PhA), with levels found to be ten times higher in hard cheese (98·9 mg/100 g cheese)(Reference Roca-Saavedra, Mariño-Lorenzo and Miranda61). However, PhA is also present in relatively high quantities in beef (up to 326 mg/100 g food) and fish (100 mg/100 g halibut or capelin)(Reference Roca-Saavedra, Mariño-Lorenzo and Miranda61). Furthermore, PhA is the degradation product of phytol, which derives from chlorophyll. Thus, its content in animal-derived products widely varies according to the farming method and animal diet, with a diet rich in grass leading to a higher PhA content in meat and dairy foods. In humans, PhA cannot be endogenously synthesised de novo but can be produced in the liver via the conversion of pristanic acid, a branched-chain FA involved in peroxisomal metabolism, although this pathway seems to be minimal(Reference Wanders, Jansen and Lloyd62).
Correlations between circulating levels and dietary intakes
A 2010 prospective study from the European Prospective Investigation into Cancer and Nutrition cohort revealed that although PhA levels correlated with dairy product intakes (r = 0·49, P < 10−4), this correlation was influenced by external factors such as age, country of residence and duration of fasting at blood collection(Reference Price, Allen and Appleby63). To date, very few RCT investigated the correlations between dairy fat intake and circulating PhA levels (Table 1). In 2011, a dietary RCT in humans revealed significant increases in PhA levels after consumption of milk, butter and cheese(Reference Werner, Hellgren and Raff64). The authors also reported a significant correlation between the consumption of butter from mountain-pasture grazing cows or cows fed conventional winter fodder and circulating PhA levels(Reference Werner, Hellgren and Raff65).
Summary of findings
Most of the evidence available to identify individual FA as biomarkers of dairy fat intake focused on odd-chain SFA, while findings on tPA, tVA and PhA are much more limited. The lack of well-controlled dose–response studies designed to validate biomarkers of dairy consumption represents an important limitation to their use in epidemiological studies.
Relevance to cardiometabolic disease risk and potential mechanisms
Circulating FA are often used as proxies for assessing the associations between dairy consumption and cardiometabolic health in observational epidemiological studies, although their physiological role in metabolism and cardiometabolic health is still unclear.
Odd-chain fattyacids: C15:0 and C17:0
Associations with markers of cardiometabolic health
At least six cross-sectional studies observed significant inverse associations between circulating odd-chain SFA, particularly C17:0, and lipid profiles, markers of inflammation or glucose metabolism, although these correlations seem to be observed mostly in overweight and obese participants(Reference Pranger, Joustra and Corpeleijn36,Reference Bongard, Yung Kai and Simon66–Reference Warensjö, Jansson and Cederholm70) . Two cross-sectional studies also suggested potential influences of other nutrients such as alcohol or different dietary patterns on the relationship between circulating odd-chain SFA and lipid profiles(Reference Zheng, Sharp and Imamura69,Reference Laguzzi, Risérus and Marklund71) . Stronger evidence from well-controlled intervention studies investigating the specific role of odd-chain SFA is still very limited. One recent dairy-feeding RCT reported concomitant increases in odd-chain SFA levels and LDL-cholesterol concentrations after the consumption of three servings of dairy/d for 4 weeks compared with an energy-matching control (290 ml of fruit and vegetable juice, 20 g of cashew nuts and a 39 g of cookie)(Reference Abdullah, Cyr and Lépine38).
Associations with CMD risk and mortality
So far, meta-analyses of prospective observational studies on the associations between circulating odd-chain SFA and CVD risk and mortality have yielded contrasting results, suggesting either neutral or inverse associations. Chowdury and colleagues observed a 23 % CHD risk reduction (relative risk (RR) = 0·77; 95 % CI (0·63, 0·93)), n 2283 cases/5490 participants) associated with higher circulating levels of C17:0. This association was consistent, albeit not statistically significant, when adding circulating C15:0 (RR = 0·81; 95 % CI (0·62, 1·06)), n 2283 cases/5490 participants)(Reference Chowdhury, Warnakula and Kunutsor72). However, these findings were not supported by data from a more recent meta-analysis of twelve European and North American prospective studies which included 7680 CVD cases(Reference Liang, Zhou and Kwame Amakye73). The authors failed to observe a significant association between circulating odd-chain SFA and overall CVD or subtypes of CVD risks, but did reveal a 28 % heart failure risk reduction associated with circulating C15:0 (RR = 0·72; 95 % CI (0·55, 0·95)), n 983 cases from two studies)(Reference Liang, Zhou and Kwame Amakye73). Further prospective human studies that investigated these associations but were not included in the above meta-analyses are summarised in Table 2. Briefly, findings from the prospective Cardiovascular Health Study revealed no changes in CVD risk, but a 33 % reduction in CVD mortality risk (hazard ratio HR = 0·77; 95 % CI (0·61, 0·98)), n 833 CVD deaths/1595 non-CVD deaths) associated with the highest quintile of circulating C17:0 compared with the lowest quintile (median concentration 0·48 % v. 0·31 % total plasma FA, respectively) after a 22-year follow-up. However, circulating C15:0 was not associated with CVD risk or mortality in this cohort(Reference de Oliveira Otto, Lemaitre and Song74). In contrast, three prospective studies failed to observe associations between circulating C17:0 and CVD risk but did report inverse associations between C15:0 and risks of myocardial infarction and stroke(Reference Warensjö, Jansson and Cederholm70,Reference de Oliveira Otto, Lemaitre and Song74,Reference Laursen, Dahm and Johnsen75) .
Table 2. Prospective human studies investigating the associations between circulating levels of odd-chain or trans-fatty acids and incident CVD, CVD mortality or incident type 2 diabetes (T2D)
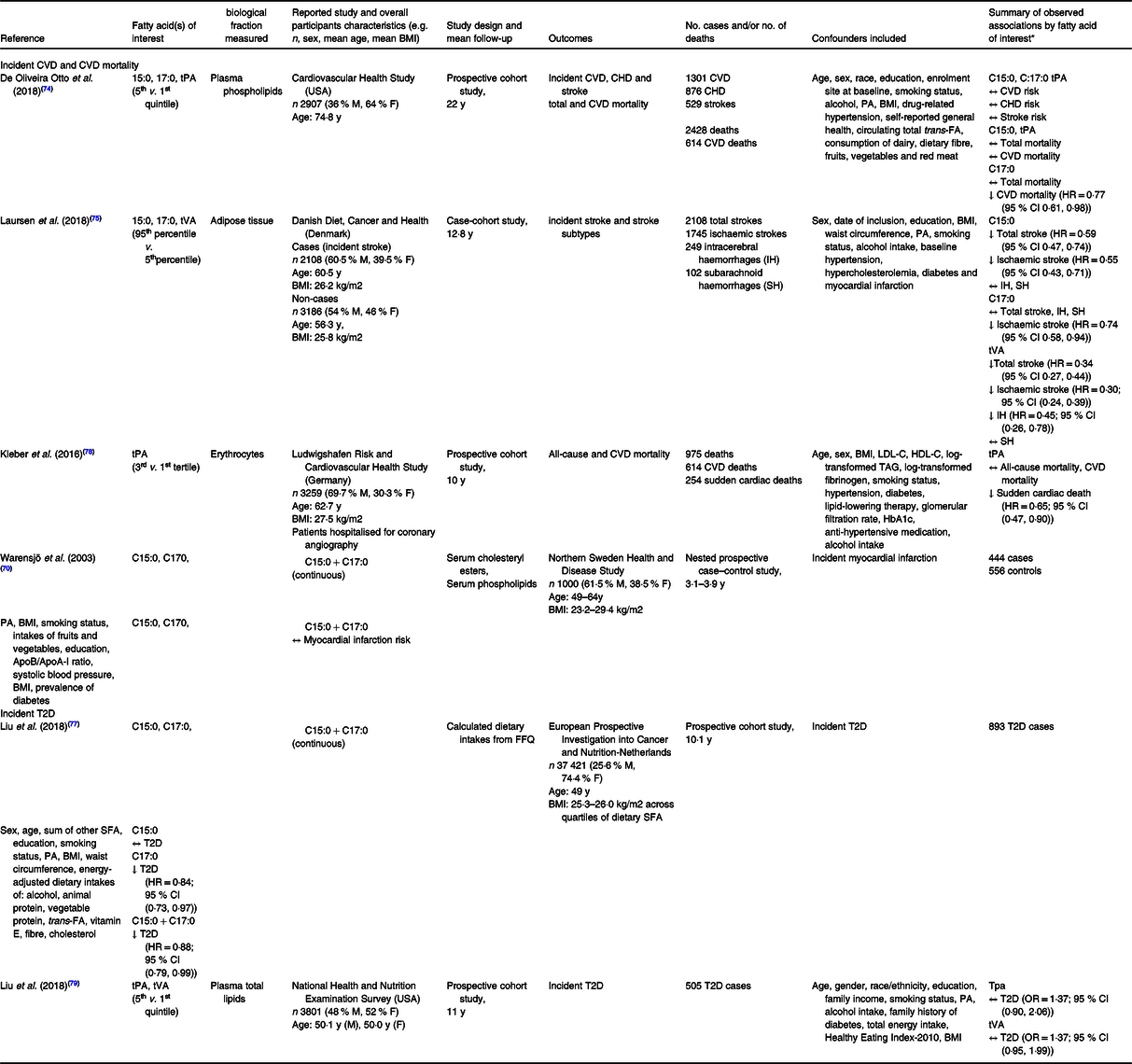
C15:0, pentadecanoic acid; C17:0, heptadecanoic acid; tPA, trans-palmitoleic acid; M, male; F, female; y, year; T2D, type 2 diabetes; IH, intracerebral haemorrhage; SH, subarachnoid haemorrhage; Apo, apolipoprotein; FA, fatty acids; PA, physical activity; ↑, direct association; ↓, inverse association; ↔, no association.
* HR and OR presented as estimate (95 % confidence interval).
Many prospective cohorts have assessed the relationships between circulating odd-chain SFA and risk of T2D, as summarised in a large meta-analysis of sixteen prospective cohorts and which included > 60 000 participants(Reference Imamura, Fretts and Marklund76). In this pooled analysis, the authors observed inverse associations between T2D risk and both circulating C15:0 (HR = 0·80; 95 % CI (0·73, 0·87)) and C17:0 (HR = 0·65; 95 % CI (0·59, 0·72)). These findings were in line with one prospective cohort published since then that was based on dietary intakes of odd-chain SFA rather than circulating levels, which observed a 12 % T2D risk reduction associated with every additional 0·11 % total energy from odd-chain SFA(Reference Liu, van der Schouw and Soedamah-Muthu77).
Potential physiological role(s) in cardiometabolic health
As previously described, odd-chain SFA metabolism in humans may involve gut microbiota and interactions with other dietary components such as fibre(Reference Weitkunat, Schumann and Nickel46). In addition, it has been suggested that they may contribute to mitochondrial metabolism and to the synthesis of very-long-chain SFA(Reference Pfeuffer and Jaudszus42). Thus, the action of odd-chain SFA might have an indirect effect on CMD risk.
Trans-fatty acids: trans-palmitoleic and vaccenic acids
Associations with markers of cardiometabolic health
Cross-sectional studies have observed inverse correlations between circulating levels of tPA and systolic blood pressure(Reference Da Silva, Julien and Couture52), fasting glucose(Reference Kratz, Marcovina and Nelson68) and plasma TAG(Reference Pranger, Muskiet and Kema35). These studies also suggested inverse correlations between tVA and BMI (in men only)(Reference Da Silva, Julien and Couture52), fasting glucose and C-reactive protein concentrations(Reference Pranger, Muskiet and Kema35). In addition, four cohort studies determined cross-sectional associations between baseline levels of tPA and CMD risk biomarkers. Higher circulating tPA levels were associated in three of these studies with elevated LDL-cholesterol and HDL-cholesterol concentrations, but lower TAG and biomarkers of T2D risk (i.e. fasting glucose, fasting insulin and insulin resistance index)(Reference Mozaffarian, Cao and King49,Reference Mozaffarian, de Oliveira Otto and Lemaitre53,Reference Kleber, Delgado and Lorkowski78) . In contrast, a more recent prospective cohort study observed direct cross-sectional relationships between higher circulating tPA and tVA and markers of glycaemic control (i.e. fasting glucose and insulin, HOMA-IR and HbA1c)(Reference Liu, Sun and Snetselaar79). Overall, most of these observations suggest inverse correlations between tPA and TAG together with direct correlations with LDL-cholesterol and HDL-cholesterol. To date, only one crossover RCT (n 106) reported that replacing dietary stearic acid with ruminant tVA or industrial trans-FA led to a similar increase in atherogenic lipids such as LDL-cholesterol, apolipoprotein B and lipoprotein (a)(Reference Gebauer, Destaillats and Dionisi80).
Associations with CMD risk and mortality
A 2018 cross-sectional study (n 3504) reported an inverse correlation between CVD risk and circulating tPA, but not tVA(Reference Zhang, Yang and Hu81). Prior to this, stronger observational evidence from a meta-analysis of prospective studies observed a trend for inverse associations between tPA levels and overall CVD risk (RR = 0·82; 95 % CI (0·67, 1·02)) and significant inverse associations with T2D risk (RR = 0·58; 95 % CI (0·46, 0·74), P < 0·001)(Reference de Souza, Mente and Maroleanu82). The latter observation was in line with results from a more recent larger meta-analysis of sixteen prospective cohort studies, which found that circulating tPA was associated with an 18 % reduced risk of T2D (HR = 0·82; 95 % CI (0·70, 0·96))(Reference Imamura, Fretts and Marklund76).
Since then, two prospective cohort studies observed inverse associations between circulating tVA and risk of strokes(Reference Laursen, Dahm and Johnsen75), or between tPA and sudden cardiac mortality(Reference Kleber, Delgado and Lorkowski78), but other studies failed to observe associations between tPA and CVD risk(Reference de Oliveira Otto, Lemaitre and Song74) or between tVA and tPA and risk of T2D(Reference Liu, Sun and Snetselaar79) (Table 2).
Potential physiological role(s) in cardiometabolic health
A number of mechanisms have been proposed from in vitro and animal studies to explain a potential impact of tPA and tVA on health outcomes. In vitro treatment of human endothelial cells with tPA and tVA (50 μmol/l for 24 h) was reported to significantly decrease TNFα-induced prostaglandin excretion and inflammatory gene expression, thus potentially limiting low-grade inflammation in blood vessels(Reference Da Silva, Bilodeau and Larose83). Second, tPA may have similar functions as its cis-isomer palmitoleic acid, which has been shown to protect animals from diabetes via the improvement of insulin sensitivity and reduction of hepatic de novo lipogenesis(Reference Mozaffarian, de Oliveira Otto and Lemaitre53,Reference Cao, Gerhold and Mayers84) . Similar improvements in markers of glycaemic control have been observed in obese and diabetic rats supplemented with tVA(Reference Wang, Gupta and Kerslake85,Reference Wang, Jacome-Sosa and Ruth86) .
Phytanic acid
To our knowledge, there is no epidemiological data on the associations between dietary or circulating PhA and CMD risk markers or clinical events. A small double-blind, parallel trial (n 5 in control group, n 9 in intervention group) failed to reveal any significant changes in blood lipids, C-reactive protein, insulin or glucose levels after chronic consumption of PhA-enriched milk, butter and cheese for 4 weeks(Reference Werner, Hellgren and Raff64).
Hypotheses regarding PhA in human metabolism and health have been proposed in a number of narrative reviews and mostly derive from in vitro and animal studies(Reference Roca-Saavedra, Mariño-Lorenzo and Miranda61,Reference Hellgren Lars87) . For example, diets enriched in phytol, a precursor for PhA, led to significant weight loss and reductions in serum total lipids, TAG and cholesterol esters in male mice(Reference Atshaves, McIntosh and Payne88). Similarly, obese mice fed with high-fat diets enriched in phytol showed reduced plasma TAG, hepatic TAG accumulation and obesity-induced fatty liver via the activation of peroxisome proliferator-activated receptor-α (PPARα)(Reference An, Jheng and Nagai89). Recent in vitro findings have suggested that the activation of PPARα may also reduce deleterious adiposity by promoting the differentiation of preadipocyte cells into beige adipocytes(Reference Wang, Mao and Du90).
Summary of findings
Overall, the evidence linking circulating odd-chain SFA, tPA and tVA with cardiometabolic health status or CMD events is mostly based on observational studies that reported contrasting results, while only one RCT investigated the effect of PhA on markers of CMD risk. In addition, the physiological role of these FA and their potential importance in CMD aetiology have not been previously investigated in humans, warranting further research on this topic.
Discussion
Dairy food consumption has often been associated with minimal or decreased CMD risk in epidemiological studies, but current methods of dietary assessment do not provide objective and reliable measures of intake. Thus, the identification of valid and reliable biomarkers of dairy fat intake is an important challenge to best determine the relationship between dairy fat consumption and cardiometabolic health. This review investigated potential biomarker candidates of dairy fat, such as odd-chain, trans- and branched-chain FA.
According to the available literature, circulating levels of odd-chain SFA seem to be modestly associated with consumptions of high-fat dairy foods in most populations. Limitations include potential endogenous synthesis and contributions of other dietary sources of these FA, which crucially need to be further assessed and ideally quantified. Moreover, the reliability of odd-chain SFA as biomarkers of dietary dairy fat in the context of different dietary patterns (e.g. low-dairy, high-fish, high-meat, high-fibre or vegan diets) is still unclear. While associations between odd-chain SFA and CVD risk are still unknown, potential associations are more likely to be driven by circulating C17:0 than C15:0. Meanwhile, the evidence on T2D appears to be more in agreement and suggests an inverse association between circulating odd-chain SFA and risk of T2D. In general, findings from circulating odd-chain SFA, which cannot discriminate specific types of dairy foods consumed, are broadly supportive of those from observational studies based on traditional dietary assessment methods(Reference Jakobsen, Trolle and Outzen91). However, these studies highlight that differential associations may exist between specific dairy foods consumed in the diet and CVD risk and may also be reflective of their overall fat content(Reference Guo, Astrup and Lovegrove94,Reference Soedamah-Muthu and de Goede95) . Importantly, although attempts have been made to quantify dairy fat consumption using traditional dietary assessment methods such as FFQ in prospective cohort studies(Reference Ardisson Korat, Li and Sacks92,Reference Chen, Li and Sun93) , future systematic literature reviews and pooled meta-analyses are warranted to assess the consistency between biomarker and dietary-based approaches to investigate the impact of dairy fat on CMD risk.
Interestingly, prospective cohort studies have reported significant correlations between circulating levels of C15:0, C17:0 and tPA (ranging from r = 0·3 to 0·8), suggesting the utility of tPA and potentially other ruminant trans-FA as novel biomarkers of dairy fat intake(Reference Imamura, Fretts and Marklund76). However, with mostly outdated results from observational studies on ruminant trans-FA, there is a striking need for new investigations on the validity of tPA and tVA as biomarkers of dairy fat intakes, especially since circulating levels of tPA are gaining interest in relation to cardiometabolic health(Reference Mozaffarian59). Conversion pathways between the two FA may also lead to confounding if used as biomarkers of dairy fat consumption separately, although the topic seems to be contentious among experts in the field. In the light of the current evidence, no conclusions can be drawn on the relevance of these FA to be used as proxies for dairy fat consumption until more well-controlled dose–response research in humans is conducted. Moreover, the observational evidence on circulating ruminant tPA and tVA in relation to cardiometabolic health does not provide a clear picture, with scarcer evidence on tVA than on tPA. Updated meta-analyses are warranted to confirm the inverse associations observed between circulating tPA levels and T2D risk.
Similar to trans-FA, the correlation between circulating concentrations of PhA and consumption of dairy products or dairy fat is still mostly unknown and has not been compared with other potential dietary sources such as fish and meat. While, to our current knowledge, PhA might not represent the most accurate biomarker of dairy fat, its utility might lay in the reflection of different cow feeding regimes (pasture grazing v. conventional fodder) and ultimately be used as a more refined biomarker of dairy food quality in combination with more robust biomarkers of intake. Besides, the current lack of data on the direct role of PhA in human cardiometabolic health highlights an important need for research in this direction.
Overall, it is still unclear whether these FA exert a direct effect on cardiometabolic health, or if they only reflect other beneficial components of dairy foods such as bioactive peptides(Reference Jauhiainen and Korpela96). It may also be difficult to disentangle the potential physiological roles of FA from those of the rest of the dairy food matrix, which is highly variable among dairy food groups such as cheese and butter(Reference Drouin-Chartier, Tremblay and Maltais-Giguère97,Reference Brassard, Tessier-Grenier and Allaire98) . In addition, it has been proposed that fermented dairy foods such as yogurts may help prevent obesity via beneficial effects on the gut microbiota, the intestinal barrier function and the hormonal regulation of appetite(Reference Pei, Martin and DiMarco99) and may be associated with lower risks of cerebrovascular diseases(Reference Sellem, Srour and Jackson100).
Conclusion
The evidence reviewed here indicates that FA are often considered as biomarkers of dairy consumption by researchers, rather than biomarkers of dairy fat intakes. This may contribute to an important confounding in the interpretation of the results, since FA, if validated as reliable biomarkers, may only accurately estimate the consumption of full-fat dairy products. With current public health guidelines often promoting the consumption of low-fat dairy, and prospective cohorts relying on FFQ that may not always distinguish dairy foods according to their fat content, it is crucial to describe FA biomarkers precisely in research papers to avoid confusion within and outside the scientific community. Importantly, the utility of FA as biomarkers of dairy fat intake needs to be interpreted in the context of overall dietary patterns and potential interactions with other food groups or nutrients.
Overall, well-controlled intervention studies would help strengthen the evidence base on the impact of dairy FA on traditional biomarkers of CMD risk (such as fasting lipid profiles and markers of glycaemic control), which are easily measured and responsive to dietary changes in a matter of a few weeks. Furthermore, the use of combinations rather than individual FA, and insights from -omics methods such as lipidomic, proteomic and metabolomics analyses, may be helpful to identify and validate new biomarkers of dairy fat and total dairy intakes(Reference González-Domínguez, Jáuregui and Mena101). Finally, research in precision nutrition and nutrigenetics have so far showed mixed associations between dairy foods, lactase persistence and cardiometabolic health(Reference Comerford and Pasin102), and further studies which take into account phenotypical traits (e.g. sex, age, adiposity or physical activity levels) may improve the overall understanding of the relationship between dairy and cardiometabolic health. In the future, validated approaches to identify new functional biomarkers of dairy fat consumption may improve our understanding of the relationship between dairy fat intake and cardiometabolic health and contribute to a better assessment of adherence to public health dietary guidelines.
Acknowledgements
Laury Sellem was funded by the Biotechnology and Biological Sciences Research Council (BBSRC) Joint Programme Initiatives (JPI) ‘HDHL Biomarkers: Fatty Acid Metabolism -Interlinking Diet with Cardiometabolic Health (FAME)’ (Project Reference: BB/P028217/1).
L. S. and L. P. conducted the literature searches. L. S. interpreted the findings and drafted the manuscript. L. P., I. D. G., K. G. J. and J. A. L. contributed to the conception of the manuscript and revised each draft for important intellectual content. K. G. J. and J. A. L. had primary responsibility for the final content of the manuscript. All authors read and approved the final manuscript.
JAL is Deputy Chair of the UK Scientific Advisory Committee for Nutrition and was an expert on SACN’s saturated fats working group. JAL (Chair), LS and KGJ are part of the International Life Science Institute (ILSI) Europe expert group on ‘Update on health effects of different dietary saturated fats’.