INTRODUCTION
Early estimates of the incidence of sports-related concussion by the Centers for Disease Control (CDC) approximated the prevalence at 300,000 cases per year in the United States (Guskiewicz, Weaver, Padua, & Garrett, Reference Guskiewicz, Weaver, Padua and Garrett2000). However, this figure is based on only sports-related concussions that resulted in a reported loss of consciousness (LOC), which only account for between approximately 8 and 20% of all cases of sports-related concussion (Langlois, Rutland-Brown, & Wald, Reference Langlois, Rutland-Brown and Wald2006). Given this, a more appropriate estimate for the rate of sports-related concussions is 1.6 million to 3.8 million cases annually (Langlois et al., Reference Langlois, Rutland-Brown and Wald2006). One difficulty with assessing the prevalence rates for concussions is the non-uniform nature of concussion; however, with increased awareness, there has also been a movement toward the development of guidelines for identifying symptoms of a concussion that distinguishes it from other more severe forms of traumatic brain injury (TBI), as well as what distinguishes it from a non-pathological reaction after a blow to the head (McCrory et al., Reference McCrory, Meeuwisse, Aubry, Cantu, Dvorák, Echemendia and Turner2013).
Although LOC or post-traumatic amnesia (PTA) are used in most concussion grading systems to signify concussion severity, more recent systems have elevated the importance of the severity and persistence of post-concussion symptoms. These include confusion, headache, dizziness, loss of balance, sleep disturbance, and visual disturbances, among others (Echemendia & Cantu, Reference Echemendia and Cantu2004). Given that factors such as headache, sleep disturbance, and affective problems are associated with cognitive problems in other populations (Basso, Miller, Estevis, & Combs, Reference Basso, Miller, Estevis and Combs2013; Engleman, Kingshott, Martin, & Douglas, Reference Engleman, Kingshott, Martin and Douglas2000; Moore, Keogh, & Eccleston, Reference Moore, Keogh and Eccleston2013), this may also be the case following sports-related concussion.
There are a small number of studies that have examined the relationship between total concussion symptoms and overall cognitive functioning post-concussion (Collie, Makdissi, Maruff, Bennell, & McCrory, Reference Collie, Makdissi, Maruff, Bennell and McCrory2006). However, to our knowledge, no study has explored whether particular symptom clusters are associated with different cognitive domains in concussed athletes. Cognitive dysfunction following a concussion may impair students’ academic performance and other life domains, so distinguishing any specific effects of symptomatology on cognition could better inform treatment plans. The present study was designed to address these issues in a sample of concussed collegiate athletes.
Symptomatology Post-concussion
One method of assessing symptomatology following concussion is the Post-Concussion Symptom Scale (PCSS), a self-report measure where examinees rate 22 symptoms on a severity scale of 0–6 (Lovell et al., Reference Lovell, Iverson, Collins, Podell, Johnston, Pardini and Maroon2006). Often the total sum of the scale is used as an outcome measure following concussion, but given the heterogeneity of presentation, the individual symptom items from the scale may provide unique information. Factor analyses of the PCSS in individuals at baseline and post-concussion have shown that PCSS items cluster together into four distinct factors (Merritt & Arnett, Reference Merritt and Arnett2014; Pardini et al., Reference Pardini, Stump, Lovell, Collins, Moritz and Fu2004).
Pardini and colleagues (2004) found cognitive, sleep, emotional, and somatic factors when examining symptoms post-concussion. Subsequently, Lau, Collins, and Lovell (Reference Lau, Collins and Lovell2011) then used Pardini’s symptom clusters to examine the role of symptom type and recovery time. Similarly, symptoms reported at baseline followed a four-factor structure, with cognitive, sleep, affective, and physical factors identified (Merritt & Arnett, Reference Merritt and Arnett2014). While the emotional/affective clusters included the same symptoms, there were some differences between the somatic/physical symptom factors between these two studies.
Lau and colleagues (2011) label the physical symptom cluster as “Migraine,” which included both the headache symptom and numbness/tingling symptom. Neither the headache nor the numbness symptom loaded onto any of the four factors in Merritt and Arnett’s (Reference Merritt and Arnett2014) study. The fact that headache did not load onto this factor is interesting, given its intuitive relationship with other symptoms encompassed by the physical factor, including dizziness and sensitivity to light.
Headache appears to be a symptom of particular significance following concussion. It is one of the most common symptoms post-concussion, and individuals who report immediate headache symptoms following injury also have more symptom reporting in the first week following concussion (Guskiewicz et al., Reference Guskiewicz, Weaver, Padua and Garrett2000; Merritt, Rabinowitz, & Arnett, Reference Merritt, Rabinowitz and Arnett2015). Other research has shown that headache lasting more than 60 hours following injury is related to delayed return-to-play (Makdissi et al., Reference Makdissi, Darby, Maruff, Ugoni, Brukner and McCrory2010). While the research specifically linking post-concussion headache to neurocognitive performance seems limited, one study on high school athletes did show that headache was related to slower reaction time and lower memory scores on the ImPACT (Collins et al., Reference Collins, Field, Lovell, Iverson, Johnston, Maroon and Fu2003).
Cognitive Impairment Following Concussion
The research on cognitive functioning post-concussion has revealed particular patterns of cognitive impairment. Because cognitive problems are common post-concussion, cognitive evaluations have been shown to provide critical information to clinicians post-injury, and are often part of return-to-play decisions (Grindel, Lovell, & Collins, Reference Grindel, Lovell and Collins2001; McCrory et al., Reference McCrory, Johnston, Meeuwisse, Aubry, Cantu, Dvorak and Schamasch2005). A meta-analysis by Broglio and Puetz (Reference Broglio and Puetz2008) found that sports-related concussion showed a large negative effect on general cognitive performance. A review of 11 meta-analyses explored the specific domains of cognition that appear to be affected by concussion (Karr, Areshenkoff, & Garcia-Barrera, Reference Karr, Areshenkoff and Garcia-Barrera2014). These meta-analyses showed differences between domains, with one meta-analysis examining mixed-mechanism mild TBI (mTBI) showing the greatest effects for verbal fluency and delayed memory, and the smallest effects for global abilities and memory generally (Karr et al., Reference Karr, Areshenkoff and Garcia-Barrera2014).
Another meta-analysis examining sports concussion specifically found the largest effects for global abilities and memory. Karr and colleagues (2014) posit that these discrepancies may be related to how the authors operationalized the cognitive domains. In particular, the effect sizes for executive functioning were variable across the different meta-analyses. The authors attribute this to how the studies defined executive function. For example, some studies included measures of verbal fluency in the executive functioning category while some did not, and this could contribute to the discrepant results (Karr et al., Reference Karr, Areshenkoff and Garcia-Barrera2014). Despite the variability among cognitive domains explored, overall neurocognitive performance appears to be negatively affected by concussion (Broglio & Puetz, Reference Broglio and Puetz2008; Grindel et al., Reference Grindel, Lovell and Collins2001; Karr et al., Reference Karr, Areshenkoff and Garcia-Barrera2014; McCrory et al., Reference McCrory, Johnston, Meeuwisse, Aubry, Cantu, Dvorak and Schamasch2005).
Present Study
Research has also shown a link between symptoms following concussion and neurocognitive impairments. A study by Collie and colleagues (2006) examined the cognitive performance of concussed athletes compared with non-injured athlete controls. They further explored the differences between injured athletes who were symptomatic versus asymptomatic. Compared to baseline performance, symptomatic athletes showed cognitive declines in multiple domains while asymptomatic athletes remained mostly stable, except for declines on divided attention measures. The non-injured controls showed no significant changes from baseline. More specifically, symptomatic athletes showed large and significant declines on attention and motor functioning tasks. A similar result was replicated with Fazio, Lovell, Pardini, and Collins (Reference Fazio, Lovell, Pardini and Collins2007) who found that, compared with asymptomatic concussed athletes, symptomatic athletes performed worse on computerized measures of cognitive functioning. These findings support the idea that the symptoms following concussion are related to neurocognitive functioning.
While there is evidence from prior work for the effect of general symptomatology on cognitive performance, there have been mixed results for the domains of cognition that are most strongly affected. Also, as far as we know, there are no published studies exploring how differences in the type of post-concussion symptoms may be related to different domains of cognition. We explore these issues in the current study.
Specific aim 1a
Examine the distinct relationship between each of the different post-concussion symptom factors (Cognitive, Physical, Affect, Sleep, and Headache) and neurocognitive performance globally.
Specific aim 1b
Given that previous research has demonstrated that specific domains of cognition may be differentially affected by concussion (Collie et al., Reference Collie, Makdissi, Maruff, Bennell and McCrory2006; Karr et al., Reference Karr, Areshenkoff and Garcia-Barrera2014), this study will also examine the distinct relationship between each of the different post-concussion symptom factors (Cognitive, Physical, Affect, Sleep, and Headache) and neurocognitive performance in memory and attention/processing speed domains.
Specific aim 2a
Examine the distinct relationship between each of the different post-concussion symptom factors and neurocognitive impairment.
Specific aim 2b
Examine the distinct relationship between each of the different post-concussion symptom factors and impairment in memory and attention/processing speed domains.
METHODS
Participants
This is an archival study involving 122 Division I college athletes (100 men and 22 women). The sports included football, soccer, wrestling, lacrosse, ice hockey, basketball, baseball, softball, rugby, swimming and diving, and golf. The mean age of the participants was 18.7 years (SD=1.04) with a range from 17 to 22 years old, and the majority of the participants were Caucasian (67.2%). Participants were involved in the concussion program at the university and were referred for testing after experiencing an mTBI/concussion as determined by posttraumatic amnesia lasting less than 24 hr, a loss of consciousness lasting 30 min or less, or any change in mental status and/or post-concussion symptoms following the injury (Ruff, Iverson, Barth, Bush, & Broshek, Reference Ruff, Iverson, Barth, Bush and Broshek2009).
Following the concussion, the student athletes were referred to our program for testing by an athletic trainer or physician, and they completed a series of questionnaires and neuropsychological tests. The participants gave written consent for their de-identified data to be used in this research. The study sample was restricted to those individuals who were tested less than 30 days post-concussion. A majority of the individuals were tested within 4 days of sustaining their concussion (N=78) and almost all were tested within the first 2 weeks (N=109). The average number of days between concussion and the testing was 5.67 (SD=5.56). Some participants were missing measures and thus, in some analyses, there were fewer participants included due to missing data.
Measures
The participants completed an extensive neuropsychological battery of tests that assessed various domains of cognitive functioning including memory, attention, and processing speed. The participants also completed questionnaires assessing their symptomatology following their concussion. The neuropsychological battery consisted of the Brief Visuospatial Memory Test–Revised (BVMT-R; Benedict, Reference Benedict1997) and the Hopkins Verbal Learning Test–Revised (HVLT-R; Brandt & Benedict, Reference Brandt and Benedict2001), which test visual and verbal memory, respectively. The Symbol Digit Modalities Test (SDMT; Smith, Reference Smith1991), the Vigil/W Continuous Performance Test (Cegalis & Cegalis, Reference Cegalis and Cegalis1994), and the Stroop Color–Word Test: Times for Trial 1 and 2 (Stroop; Trenerry, Crosson, DeBoe, & Leber, Reference Trenerry, Crosson, DeBoe and Leber1989) were all used as measures of attention and processing speed.
Additionally, the ImPACT (Immediate Post-Concussion Assessment and Cognitive Testing; Lovell, Collins, Podell, Powell, & Maroon, Reference Lovell, Collins, Podell, Powell and Maroon2000), a test with multiple modules designed to measure outcomes following concussion, was also administered for measures of verbal memory, visual memory, attention and processing speed. The Wechsler Test of Adult Reading (WTAR; Wechsler, Reference Wechsler2001) was given to athletes at their baseline assessment (pre-concussion) as a measure of premorbid IQ. The BDI-FS (Beck Depression Inventory – Fast Screen; Beck, Steer, & Brown, Reference Beck, Steer and Brown2000) is an abbreviated 7-item depression questionnaire given to athletes at both baseline and post-concussion assessments. The participants also completed the Post-Concussion Symptom Scale (PCSS), rating their experience of 22 common post-concussion symptoms on a scale of 0 (None) to 6 (Severe) (Lovell et al., Reference Lovell, Iverson, Collins, Podell, Johnston, Pardini and Maroon2006).
Procedures
The Pennsylvania State University’s Sports-Concussion Program is based on the “Sports as a Laboratory Assessment Model (SLAM)” model (Bailey, Barth, & Bender, Reference Bailey, Barth and Bender2009). All participants were referred to our program from their team physician following a sports-related concussion and were administered the neuropsychological measures, as well as the background information and neurobehavioral questionnaires. The neuropsychological tests were administered by clinical psychology graduate students and undergraduate research assistants, all under the supervision of a clinical neuropsychologist. This study was approved by the University’s Institutional Review Board and informed consent was collected from all participants.
Analyses
Approach to data analysis
All analyses were conducted with the Statistical Package for the Social Sciences (SPSS), Version 24.0 (IBM Corp, 2016).
Main data analyses
The aim of this study was to explore the relationship between specific post-concussion symptoms and cognitive outcomes. Previous research using factor analysis has shown that the items on the PCSS load into four main factors: Cognitive, Physical, Affective, and Sleep (Merritt & Arnett, Reference Merritt and Arnett2014). New variables were created using the sum of the participants’ scores from the questions that comprise the various symptom factors. Each symptom is rated on a scale of 0 (not experiencing that symptom) to 6 (experiencing that symptom severely). Therefore, higher values on these factor variables can indicate either more symptoms or greater severity for each type of symptom factor (see Figure 1). Given the evidence that headache is an important symptom following concussion, and because it did not load on any of the other factors, it was also examined in the same manner as other symptom factors.
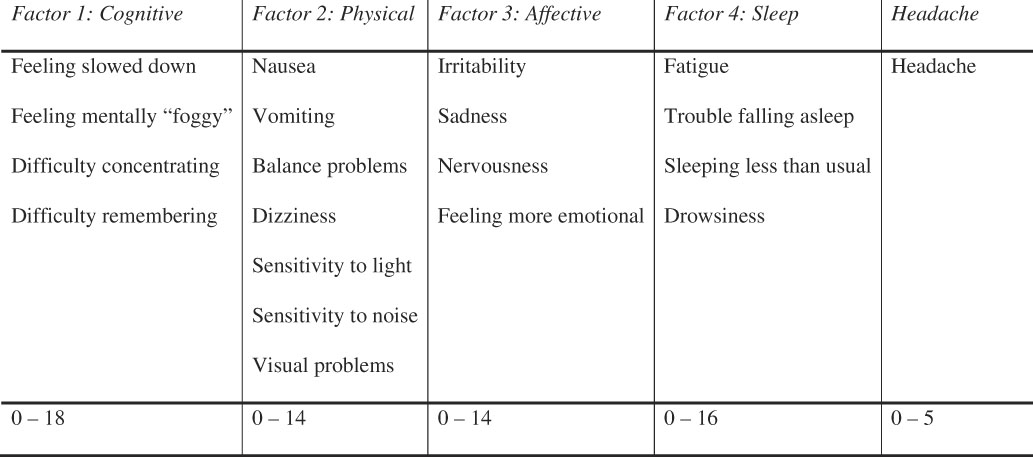
Fig. 1 The symptoms from the PCSS for each factor and headache are listed, and the range of scores that participants reported are noted in the bottom row.
To determine the best approach for analyzing the data, these factor variables were checked for skewness. All of the distributions for the symptom factor variables were positively skewed. Due to these skewed distributions, each factor variable and the headache variable were dichotomized. Given the large number of individuals who reported no symptoms at the time of testing following their concussion, “no symptoms” and “symptoms” groups were used which separated the individuals into a group with scores of 0 and a group with scores of 1 or more.
Specific aim 1a and 1b
To examine cognitive functioning, standard score performance on the aforementioned neuropsychological tests was used to create a composite neuropsychological outcome score. Each of the symptom factor groups’ (i.e., “no symptoms” and “symptoms” for each factor) performance on this neuropsychological composite was compared using an independent samples t test. The same analysis was performed with a composite of all the memory tests (Impact Verbal and Visual Memory Composites, HVLT-R Immediate and Delay Trials, and BVMT-R Immediate and Delay Trials) as the outcome variable. A principal components analysis was conducted for the tests in this composite, and all of the variables loaded above .600. A comparable analysis of a composite of the attention/processing speed tests (Impact Visuomotor Speed and Reaction Time Composites, SDMT total correct, and Stroop 1 time) as the outcome variable was carried out. A principal components analysis was conducted for the tests in this composite, and again, all of the variables loaded above .600 (see Table 1 for more information on the neurocognitive composites).
Table 1 Neurocognitive composites

Specific aim 2a & 2b
Impairment scores were calculated by converting all the neuropsychological scores into standard score units, then any score below 80 (performance below the 10th percentile) was considered an impaired score. Based on normative data from the WAIS-III, performance below the 10th percentile is considered within the impaired range, and WAIS classifications are commonly accepted cutoffs of cognitive functioning (Strauss, Sherman, & Spreen, Reference Strauss, Sherman and Spreen2006). To examine general cognitive impairment, individuals were grouped based on the overall number of impaired scores. A cutoff for this was determined by using the median split score from baseline data from athletes in our program (median=1.00). Thus, individuals with two or more impaired scores were placed in the impaired groups, and individuals with one or no impaired scores were placed in the non-impaired group.
Chi-Square analysis on each of the symptom factor groups (i.e., “no symptoms” vs. “symptoms”) and the impairment groups (i.e., “impaired” vs. “not impaired”) was performed to determine if belonging to the “symptoms” group for any particular factor resulted in an individual being more likely to be in the cognitively “impaired” group. A composite variable was created that includes the total number of impairment scores on only memory tests and again the median split score from our baseline data was used to create the “memory-impaired” and “not memory-impaired” groups with a cutoff of two or more impaired tests. The same analyses were also conducted with the tests of attention/processing speed and the cutoff was again two tests. A chi-square analysis was performed on each of the symptom factors (i.e., “no symptoms” vs. “symptoms”) and the impairment groups (i.e., “memory impaired” vs. “not memory impaired”) to determine whether belonging to the “symptoms” group for any particular factor resulted in that an individual being more likely to be in the cognitively “impaired” group for either of these two domains.
RESULTS
The study included six outcome variables (three neurocognitive composite scores and three neurocognitive impairment scores); therefore, a Bonferroni correction was applied to account for multiple comparisons. Thus the following results are considered significant at p<.008 (.05/6=.008).
Specific Aim 1a
Individuals who reported physical symptoms did not show significantly worse performance (M=92.74; SD=16.00) on the overall neurocognitive composite compared with individuals who did not report physical symptoms (M=98.88; SD=9.95), t(105)=2.45, p=.016, d=.46. There was no significant effect of sleep symptom group, t(105)=2.22, p=.029, d=.43, cognitive symptom group, t(105)=0.65, p=.515, d=.13, or of the affective symptom group, t(105)=0.68, p=.501, d=.14 on overall neurocognitive performance. Finally, individuals who reported headache symptoms had significantly worse performance on the overall neurocognitive composite (M=92.91; SD=14.53) compared to the no headache symptom group (M=100.27; SD=9.54), t(87)=3.11, p=.003, d=.60.
Specific Aim 1b, Memory Composite
Individuals who reported physical symptoms did not perform significantly worse on the memory composite than individuals who did not report physical symptoms, t(112)=2.21, p=.029. d=.43. There was also no significant difference in performance on the memory composite between the cognitive symptom groups, t(112)=1.54, p=.127, d=.29, or affective symptom groups, t(112)=1.18, p=.243, d=.24. However, individuals who reported sleep symptoms did perform significantly worse on the memory composite (M=94.56; SD=13.95), t(107)=−2.80, p=.006, d=.53, than those who did not report sleep symptoms (M=101.00; SD=10.62). Additionally, compared with the no headache symptom group (M=101.31; SD=10.45), the headache symptom group displayed significantly worse performance on the memory composite (M=93.50; SD=13.65), t(112)= −3.45, p=.001, d=.64.
Specific Aim 1b, Attention/Processing Speed Composite
Individuals who reported physical symptoms did not perform significantly worse on the attention/processing speed composite than individuals who did not report physical symptoms, t(109)=−1.63, p=.106, d=.31. There was also no significant difference in performance on the attention/processing speed composite between the sleep symptom groups, t(109)= −0.84, p=.403, d=.16, cognitive symptom groups, t(109)= 0.26, p=.799, d=.05, or affective symptom groups, t(109)=−0.41, p=.685, d=.08. However, the headache symptom group showed worse performance on the attention/processing speed composite (M=92.96; SD=14.87) compared to the no headache symptom group (M=100.47; SD=10.56), t(95)=−3.05, p=.003, d=.54.
Specific Aim 2a
Regarding overall neurocognitive impairment, the following symptom/no symptom groups were not significantly different: Affective, Cognitive, Physical, and Sleep. In contrast, a significantly greater proportion of individuals with headache symptoms showed overall neurocognitive impairment (64.1%) compared to those without any headache symptoms (35.9%), χ2 (1, N=122)=7.65, p=.006, φ=.25.
Specific Aim 2b, Memory Impairment
For memory impairment, there were no significant differences between the physical symptom groups, χ2 (1, N=122)= .062, p=.803, φ=.02, cognitive symptom groups, χ2 (1, N= 122)=1.68, p=.195, φ=.12, or affective symptom groups, χ2 (1, N=122)=1.66, p=.20, φ=.12. Among the individuals demonstrating memory impairment, significantly more individuals reported sleep symptoms (76%) than did not (24%), χ2 (1, N=122)=8.50, p=.004, φ=.26. There were also significantly more individuals reporting headache symptoms, with 72% who reported headache symptoms and 28% who reported none, respectively, χ2 (1, N=122)=8.63, p=.003, φ=.27.
Specific Aim 2b, Attention/Processing Speed Impairment
For attention/processing speed impairment, there were no significant differences between the physical symptom groups, χ2 (1, N=122)=4.42, p=.036, φ=.19, sleep symptom groups, χ2 (1, N=122)=.062, p=.803, φ=.02, cognitive symptom groups, χ2 (1, N=122) = .000, p=.987, φ=.00, or affective symptom groups, χ2 (1, N=122)=.046, p=.829, φ=.02. However, in the attention/processing speed impaired group, significantly more individuals reported headache symptoms (78.9%) than those who reported none (21.1%) (N=122, p=.002, Fisher’s Exact Test, φ=.29) (see Figure 2).

Fig. 2 The percentage of total participants in each of the symptom and neurocognitive impairment groups are presented by the bars in the figure. The numbers within the bars note the number of participants in that cell. a: Overall neurocognitive impairment groups and headache symptom groups are illustrated. b: Memory impairment, headache symptom, and sleep symptom groups are illustrated. c: Attention/processing speed impairment groups and headache symptom groups are illustrated. *p<.008.
Supplementary Analyses
For each of the five factors, there were no significant differences between the symptom and no symptom groups on days since concussion. An additional analysis also examined whether there was a difference of gender in symptom reporting. There were no significant differences between genders for physical, sleep, cognitive, or affective symptom groups. However, a significantly higher percentage of women reported headache symptoms (77.3%) compared to men (39%), χ2 (1, N=122)=10.64, p=.001, φ=.295.
DISCUSSION
Results and Contributions From the Current Study
Symptoms and cognitive impairment are both common outcomes following a concussion and have an impact on an individual’s life and daily functioning. Recent research has shown a general relationship between symptoms following a concussion and cognitive functioning. However, it has been less clear how specific symptoms may be differentially associated with cognitive outcome generally as well as specific domains of cognition.
The current study explored the relationship between different symptom factors and multiple neurocognitive outcomes. First, the neurocognitive outcomes were examined at the mean level and this explored how reporting of symptoms for the five different symptom factors was related to outcomes. We found that reports of headache symptoms were significantly related to poorer overall neurocognitive performance. More specifically, we found that reports of sleep symptoms were significantly associated with poorer performance on memory tests. Individuals reporting headache symptoms had significantly worse performance on both the memory and attention/processing tests compared to those not reporting any headache symptoms.
Another way of examining neuropsychological outcomes is through impairment scores, which can be more clinically meaningful as they are often used in making return to play decisions. The results of the present study showed that there was no significant relationship between reports of affective, cognitive, physical, or sleep symptoms and overall neurocognitive impairment. However, individuals reporting headache symptoms were more likely to show general neurocognitive impairment than those not reporting headache symptoms. When examining the specific domains of cognition, comparable to the mean level data there was a relationship between sleep and memory. Individuals reporting sleep symptoms were more likely to be impaired on the memory tests than individuals without sleep symptoms. Again, individuals with headache were more likely to be impaired on both tests of memory and attention/processing speed than those without headache symptoms.
Previous research has indicated that headache is an important symptom following concussion. Immediate headache has been related to greater symptoms a week following a concussion and it has been linked to longer time before return-to-play decisions (Guskiewicz et al., Reference Guskiewicz, Weaver, Padua and Garrett2000; Makdissi et al., Reference Makdissi, Darby, Maruff, Ugoni, Brukner and McCrory2010; Merritt et al., Reference Merritt, Rabinowitz and Arnett2015). While most of the previous concussion literature has focused on the relationship between general symptoms and cognitive impairment, this study indicates the potential for a more specific relationship between headache and cognitive impairment.
This was also demonstrated by Collins and colleagues (2003) who showed that high school athletes who reported headache symptoms following concussion had worse cognitive performance than athletes who did not report headache. However, that study did not examine other symptom types, which our present study included. Our study provides further evidence that headache may be a particularly important symptom for cognitive functioning following a sports-related concussion. This could be due to the salience of head-related pain when individuals are attempting to focus on cognitively demanding tasks.
These results also indicated a specific relationship between sleep symptoms and both mean performance and impairment on a composite of memory tests. While there has been little research in the concussion literature examining the specific relationship between sleep problems and memory impairments, that link has previously been observed in other literatures. Multiple studies of adults and children with sleep apnea have demonstrated memory impairment in these populations (Rhodes et al., Reference Rhodes, Shimoda, Waid, O’Neil, Oexmann, Collop and Willi1995; Salorio, White, Piccirillo, Duntley, & Uhles, Reference Salorio, White, Piccirillo, Duntley and Uhles2002). Other research has also explored the mechanisms through which sleep may disrupt memory function. In one study using a rodent model, sleep disturbance resulted in neuroinflammation in hippocampal areas which was associated with impairments in memory performance (Zhu et al., Reference Zhu, Dong, Xu, Gompf, Ward, Xue and Xie2012). Although the exact mechanism is not entirely clear in the present study’s post-concussion sample, the specific relationship between sleep symptoms and memory impairment is consistent with other research literature.
Given the importance of cognition in daily functioning, particularly to student-athletes who suffer from concussions, this work could help raise awareness around specific cognitive vulnerabilities that may be associated with distinct symptom clusters. The knowledge that experiencing sleep symptoms following a concussion could be more related to memory difficulties and physical symptoms more to attention/processing speed difficulties could help clinicians better tailor treatment recommendations and accommodations to suit the needs of the individuals affected.
Also, knowing that headache appears to be most strongly related to all the aforementioned types of cognitive difficulties could help clinicians more readily identify athletes who may be at particular risk of overall negative cognitive outcomes and make recommendations accordingly. It may be that post-concussion headache can serve as a marker for athletes who are also at high risk for cognitive problems.
The supplementary analyses revealed that symptom reporting was not simply a reflection of time since concussion. Additionally, we found a substantial gender difference in headache symptom reporting such that women reported more headache symptoms than men. Despite the small sample of female participants (n=22), the effect size was medium (φ=.285). Other research within the concussion and traumatic head injury literature has also shown that women tend to report more symptoms than men following a concussion (Broshek et al., Reference Broshek, Kaushik, Freeman, David, Webbe and Barth2005; Farace & Alves, Reference Farace and Alves2000). Preiss-Farzanegan, Chapman, Wong, Wu, and Bazarian (Reference Preiss-Farzanegan, Chapman, Wong, Wu and Bazarian2009) found this gender effect as well, specifically for headache, dizziness, fatigue, and trouble concentrating. This result is also consistent with studies conducted in a general population, which found that women are more likely to report experiencing headache, greater pain with headache, and were more likely to use health care services for headaches (Burch, Loder, Loder, & Smitherman, Reference Burch, Loder, Loder and Smitherman2015; Stewart, Lipton, Celentano, & Reed, Reference Stewart, Lipton, Celentano and Reed1992).
Together, these results indicate that women could be more prone to experiencing headache symptoms than men, and this effect may simply be captured in concussion assessments. It is also possible that baseline proneness to headaches in women leads to an increased susceptibility following a concussion. Finally, women may simply be more likely to report these symptoms compared to their male counterparts.
Limitations
One limitation of the current study is that the grouping method does not fully isolate specific symptoms. For example, individuals who are included in the headache group almost always also reported symptoms from other factors, but the individuals in the no headache group also reported symptoms from other factors. Given the extremely small number of individuals reporting symptoms from only one factor, it was not feasible to create meaningful pure symptom groups. Additionally, given that it is rare for individuals to report only one type of symptom, it may not be clinically meaningful to examine the data in this way because such a conceptualization does not reflect the actual reality of most symptom reports.
It should also be noted that for one of the attention/processing speed analyses, Fisher’s Exact Test was used because one of the cell counts fell below 5. Given this, further exploration with a larger sample size may better elucidate the strength of the relationship between headache and attention/processing speed impairment following sports-related concussion.
Other limitations of the study involve the generalizability of the findings given that the sample consisted solely of college athletes who had suffered mTBIs. It is possible that these results might differ in older or more severely injured samples. Our sample also included a majority of male participants (n=82%), and given that our results indicated a potential increased risk or vulnerability for headache symptoms in women, this could be an important gender difference to study further with a larger sample of female athletes.
Another limitation of the current study is the use of self-reported symptoms, which may be misrepresented by athletes due to return-to-play motivations. However, the athletes included in this sample all demonstrated sufficient effort and motivation based on clinician ratings and objective neuropsychological testing measures. Given the importance of self-reported symptoms in making return-to-play decisions, it is worthwhile to continue to examine such measures and their relationship to other outcomes following concussion.
CONCLUSIONS
When examining the relationship between specific types of symptoms and cognitive outcomes following concussion, headache symptoms are related to overall cognitive impairment as well as memory and attention/processing speed impairment. Sleep symptoms appear to be specifically related to impairments in memory. As previous research has established, the overall number of reported symptoms is related to outcomes following concussion. However, the present results highlight the importance of identifying the particular types of symptoms, as this could provide more knowledge about specific cognitive impairment and allow for more tailored recommendations for treatment and accommodations following injury.
ACKNOWLEDGMENTS
Special thanks to Jessica Meyer, Victoria Merritt, Margaret Cadden, Dr. Frank Hillary, and Dr. Michele Diaz for their help with various aspects of the project. We also thank Dr. Wayne Sebastianelli, MD, Director of Penn State Sports Medicine, and the Penn State Sports Medicine team, for their ongoing support of our research. This project received financial support from the Penn State Psychological Clinic and Penn State Sports Medicine. Ms. Guty does not have any conflicts of interest to report. Dr. Arnett has served as part of a paid speaker series sponsored by EMD Serono. He has also served as a consultant for Biogen IDEC.