Schizophrenia is a complex disorder with a wide range of symptoms. Many studies have found evidence of abnormal brain structure and function in patients with this disorder; however, most of the reported abnormalities only occur in a subset of cases. Therefore, one objective in psychiatric research is the identification of endophenotypes of schizophrenia. Reference Phillips1 Formal thought disorder is one of the main symptoms of schizophrenia, and there is increasing evidence that it can be traced to abnormalities in specific brain regions. Reference McGuire, Quested, Spence, Murray, Frith and Liddle2–Reference Shenton, Kikinis, Jolesz, Pollak, LeMay, Wible, Hokama, Martin, Metcalf and Coleman5 In particular, formal thought disorder is often interpreted as a complex dysfunction of the language system, Reference Stirling, Hellewell, Blakey and Deakin6 involving executive function, semantic memory and spreading activation in semantic memory. Reference Kerns and Berenbaum7 One robust finding in formal thought disorder is volume reduction in the left superior temporal gyrus (STG). Reference Shenton, Kikinis, Jolesz, Pollak, LeMay, Wible, Hokama, Martin, Metcalf and Coleman5,Reference Vita, Dieci, Giobbio, Caputo, Ghiringhelli, Comazzi, Garbarini, Mendini, Morganti and Tenconi8,Reference Subotnik, Bartzokis, Green and Nuechterlein9 The posterior part of this brain region has a major role in semantic memory. Reference Jung-Beeman10,Reference Heim11 None the less, volume loss alone cannot explain the often waxing and waning character of formal thought disorder. Presumably the heterogeneous presentation of this disorder is caused by additional, dynamic factors in terms of neuronal activity.
To date, few studies on resting metabolism have used psychopathology (i.e. symptoms or symptom patterns instead of diagnostic categories) to distinguish schizophrenia subgroups. Liddle et al investigated the disorganisation syndrome, which is characterised by formal thought disorder and inappropriate affect. They found decreased regional cerebral blood flow (rCBF) in the right Brodmann area (BA) 47/45, BA 44 and in the bilateral angular gyrus. Regional CBF was increased in the anterior cingulate cortex, BA 9/10, the dorsomedial thalamus and the left STG. Reference Liddle, Friston, Frith, Hirsch, Jones and Frackowiak12 In a single photon emission computed tomography (SPECT) study, Ebmeier et al showed increased tracer uptake in the anterior cingulate in the disorganisation syndrome. Reference Ebmeier, Blackwood, Murray, Souza, Walker, Dougall, Moffoot, O'Carroll and Goodwin13 In a study by Sabri et al, bifrontal, left parietal and anterior cingulate hyperperfusion was found in patients with formal thought disorder and grandiosity. Reference Sabri, Erkwoh, Schreckenberger, Owega, Sass and Buell14 Recently, Lahti et al demonstrated a positive correlation between disorganisation syndrome and rCBF in the left posterior inferior frontal gyrus (IFG) extending into the anterior insula. Reference Lahti, Weiler, Holcomb, Tamminga, Carpenter and McMahon15 Thus, resting metabolism in patients with formal thought disorder has been investigated only in combination with other schizophrenia symptoms, disregarding the severity of the thought disorder; this may explain the great variability of the findings reported above. Moreover, the studies mentioned measured relative values of metabolism or perfusion and are therefore dependent on the values of reference regions. The primary aim of our study was to clarify the specific relationship between the severity of formal thought disorder and resting perfusion on the one hand, and between resting perfusion and grey-matter volume on the other.
Method
Participants
We investigated 13 people with schizophrenia receiving in-patient treatment at the University Hospital of Psychiatry in Bern, Switzerland (5 women and 8 men; mean age 29.6 years, s.d.=11.2). Schizophrenia was diagnosed according to DSM–IV and ICD–10 criteria, 16,17 based on clinical interview and psychiatric history. We also recruited 13 healthy controls (5 women and 8 men; mean age 26.6 years, s.d.=4.6). The age of the patient group and that of the control group did not differ significantly (t (12)=–0.91). All participants were right-handed according to the Edinburgh Handedness Scale. Reference Oldfield18 Potential participants with medical disorders other than schizophrenia were excluded based on medical history and medical and neurological examination. None of the patients or controls reported substance misuse in the 4 weeks before or during the study. All patients except two were being treated with antipsychotic medication: the mean dosage in chlorpromazine equivalents was 556.2 mg (range 0–1850). Reference Woods19 The daily antipsychotic dose in chlorpromazine equivalents was not correlated with the severity of formal thought disorder. The mean duration of illness was 2.79 years (s.d.=2.60).
Less than 45 min prior to the participants undergoing magnetic resonance imaging (MRI) scanning, their psychopathological state was assessed with the Positive and Negative Syndrome Scale (PANSS) Reference Kay, Fiszbein and Opler20 and the Scale for the Assessment of Thought, Language and Communication (TLC). Reference Andreasen and Grove21 For the latter variable we used the sum of all TLC items for each individual; the TLC score is directly proportional to the severity of formal thought disorder. The ratings were performed by an experienced, specifically trained psychiatrist (H.H.). The study was approved by the local ethics committee; all participants gave written informed consent to take part.
Structural image acquisition
Structural images were acquired using a 1.5 T whole-body MRI system (Siemens Vision, Erlangen, Germany) with a standard radiofrequency head coil. During arterial spin labelling MRI we obtained one set of three-dimensional T 1-weighted, magnetisation prepared rapid acquisition gradient echo (MP-RAGE) images for each participant, providing 192 sagittal slices of 1.0 mm thickness, 256×256 mm2 field of view (FOV), matrix size 256×256. Further scan parameters were 2000 ms repetition time (TR), 4.4 ms echo time (TE) and a flip angle of 15°. These high-resolution images were used for voxel-based morphometry analysis to compare grey-matter volume and total intracranial volume between groups. The structural images for each participant were pre-processed according to the optimised VBM protocol, Reference Good, Johnsrude, Ashburner, Henson, Friston and Frackowiak22,Reference Honea, Crow, Passingham and Mackay23 using SPM5 (Wellcome Department of Imaging Neuroscience, London, UK; www.fil.ion.ucl.ac.uk). The spatially normalised segments of each individual's grey-matter images were modulated for volume analysis and then smoothed with 10 mm full width at half maximum (FWHM) kernel. Reference Honea, Crow, Passingham and Mackay23 Finally, we calculated the volumes of grey matter, white matter and cerebrospinal fluid, as well as the total intracranial volume.
Cerebral blood perfusion
Absolute cerebral blood perfusion was measured using the non-invasive MRI method of pulsed arterial spin labelling. This technique magnetically labels the endogenous water molecules in the blood flowing into the brain, thus providing a tracer to quantify perfusion of blood into brain regions. We used this method with quantitative imaging of perfusion using a single substraction, second version QUIPPS II and thin-slice inversion time 1 (TI1) periodic saturation, as has been described elsewhere. Reference Luh, Wong, Bandettini and Hyde24,Reference Wong, Buxton and Frank25 The pulsed arterial spin labelling parameters were as follows: the gap between the labelling slab and the proximal slice was 10 mm; TI1=700 ms; TI1 stop time 1300 ms; TI2=1400 ms; crusher bipolar gradients were switched between slice excitation and readout to reduce signal from large vessels. Further parameters were: TR=2500 ms, TE=15 ms, FOV= 224×224 mm2, matrix size 64×64; six slices at a distance of 1.5 mm; slice thickness 6.0 mm; partial Fourier 6/8; bandwidth 3.004 kHz per pixel; echo spacing 0.4 ms; number of measurements n=144. The brain volume covered by the arterial spin labelling perfusion measurement is shown in Fig. 1. We analysed the data using self-written Matlab programs Reference Federspiel, Muller, Horn, Kiefer and Strik26 (Matlab version 7, release 14; The MathWorks Inc., Natick, USA) and visualised with BrainVoyager QX 1.7.6 (Brain Innovation B.V., Maastricht, The Netherlands). All arterial spin labelling time series were first realigned to correct for motion artefacts, co-registered with each participant's anatomical MRI, and then spatially smoothed with a three-dimensional 12 mm FWHM Gaussian kernel. Reference Wang, Rao, Wetmore, Furlan, Korczykowski, Dinges and Detre27 We calculated flow-time series by simply subtracting the labelling images from the control images. This difference signal is proportional to CBF. Reference Luh, Wong, Bandettini and Hyde24 The quantification of CBF of the flow time series was performed using the equation:


Fig. 1 Brain volume covered by the arterial spin labelling perfusion measurement.
where ΔM is the difference signal (control – labelling), λ is the blood/water partition coefficient (0.9 ml/g) and M 0 is the equilibrium brain tissue magnetisation; the time constants TI1, TI2 were set to 700 ms, 1400 ms, and for 1.5 T the decay time for labelled blood TIb is 1200 ms and the labelling efficiency is α=0.95.
Statistical analysis
We commenced factor analysis by computing a correlation matrix for all PANSS items, including factor extraction and orthogonal rotation for the interpretation of the factors. The factors were extracted by principal component analysis in which the components were all uncorrelated. Factor loadings were used to interpret which PANSS item was included in each factor. Items with factor loadings greater than 0.4 were selected such that each explained at least 10% of the variance (eigenvalue >1). This principal components factor analysis revealed six PANSS items (N2, N3, N6, N1, P2 and N4) that explained 86.4% of the observed variance. In the following statistics we excluded item P2 because it is directly linked to formal thought disorder. None of the five remaining PANSS items showed any correlation with the severity of formal thought disorder as measured by the TLC: N2: r=–0.28, P=0.35; N3: r=–0.28, P=0.36; N6: r=0.02, P=0.95; N1: r=–0.19, P=0.53; N4: r=–0.18, P=0.54. Moreover, no correlation was found between severity of formal thought disorder and antipsychotic dosage in chlorpromazine equivalents (r=0.14, P=0.65), patient age (r=0.49, P=0.09) or patient gender (r=–0.48, P=0.1).
Voxel-based morphometry
We performed group comparisons of the morphometric data using a general linear model (t-test for matched pairs). Cortical areas showing significant differences (P<0.01, corrected for multiple comparisons; see below) in grey-matter volumes between the schizophrenia and control groups were defined as clusters. To evaluate the relationship between severity of formal thought disorder (TLC score) and grey-matter volume in the schizophrenia group, we used the non-parametric Spearman rank correlation coefficient (r s). Likewise, clusters were defined as brain regions with significant correlation coefficient (P<0.01, corrected for multiple comparisons).
Cerebral blood flow
Group comparisons of CBF data performed with a general linear model (t-test for matched pairs) defined cortical areas that showed significant differences (P<0.01, corrected for multiple comparisons) in CBF values between the schizophrenia group and the control group as clusters (Fig. 2). To evaluate the relationship between formal thought disorder severity and CBF in the schizophrenia group, we used non-parametric Spearman rank correlation coefficients (r s). Likewise, clusters were defined as brain regions with significant correlation coefficients (P<0.01, corrected for multiple comparisons). For the significant regions showing a correlation between formal thought disorder severity and CBF in the patient group, we additionally extracted the perfusion values in these regions for the control group.

Fig. 2 Maps of t-values (P<0.01, corrected for multiple comparisons) at z=2 and z=20 for the comparison between the schizophrenia and control groups. The upper row shows the t-values for grey-matter volume differences; only values above the indicated line are significant. The lower row shows the t-value differences in absolute cerebral blood flow (CBF); here no region reached significance. NS, not significant.
The correction for multiple comparisons (in order to control for type I errors) was performed using the method of cluster-size thresholding (implemented in BrainVoyager QX 1.7.6), which has been described in detail elsewhere. Reference Forman, Cohen, Fitzgerald, Eddy, Mintun and Noll28 In this correction procedure the smoothing kernel of the data was included. Additionally, the intersections of the significant locations (CBF and grey matter) were tested in a conjunction analysis using two statistical maps (threshold P<0.001): the map to evaluate the relationship between formal thought disorder severity and grey matter, and the map to evaluate the relationship between severity and CBF in the schizophrenia group. Finally, for the overlapping regions, we performed a linear regression with the pooled CBF and pooled grey-matter values to check for dependencies between both functional entities. Statistical testing was performed using Matlab routines glmfit, regstats and corr, with the option [Spearman] and the SPSS version 13.0 for Windows.
Results
The mean global PANSS score was 63.9 (s.d.=16.7) and the mean TLC score was 7.2 (range 0–28). To avoid the confounding effects of positive or negative psychopathological symptoms other than formal thought disorder, we evaluated the interaction between positive PANSS scores (13.8, s.d.=4.8), negative PANSS scores (15.5, s.d.=6.1) and the effects of formal thought disorder (TLC score). Adjusted for the effects of formal thought disorder (excluding PANSS item P2), positive and negative PANSS scores were not significantly correlated with the severity of formal thought disorder (TLC score). Also, duration of illness was not correlated with TLC score (r=0.33, P=0.35).
Grey-matter volume
Schizophrenia group v. controls
Whole-brain voxel-based morphometry revealed significantly reduced total grey-matter volume in the schizophrenia group compared with the control group: t (12)=–2.6, P=0.02 (controls=773.1, s.d.=54.8; patients 714.2, s.d.=76.2). However, we did not find any significant volumetric differences for total white matter (t (12)=–1.2, P=0.24; controls=512.1, s.d.=43.4; patients 500.1, s.d.=51.9), total cerebrospinal fluid (t (12)=1.5, P=0.16; controls=448.4, s.d.=80.6; patients 510.2, s.d.=133.5) or total intracranial volume (t (12)=–0.1, P=0.89; controls=1733.5, s.d.=100.9; patients 1724.5, s.d.=215.5).
Voxel-wise statistical testing revealed that 12 brain regions showed significant reductions in grey-matter volume (P<0.01, corrected for multiple comparisons) in the schizophrenia group compared with controls (Table 1 and Fig. 2). To test for non-normality of the residuals, the Shapiro–Wilk test was computed for each voxel of each of the 12 brain regions. With the Shapiro–Wilk test the null hypothesis is that residuals follow a normal distribution, i.e. if the P-value is greater than the α-value of 0.05, then the null hypothesis will not be rejected. Reference Jones, Symms, Cercignani and Howard29 In all 12 brain regions the P-values were greater than 0.05.
Table 1 Significant clusters of grey matter reduction in the schizophrenia group compared with controls a

Talairach coordinates at centre of gravity, mean (s.d.) | General linear model | ||||||||
---|---|---|---|---|---|---|---|---|---|
Anatomical brain region | x | y | z | Voxels within cluster | Mean t-value | P b | |||
Left anterior STG | -47 (1.6) | -1 (2.8) | -6 (2.8) | 2969 | -4.0262 | 0.0017 | |||
Right middle frontal gyrus | 27 (2.5) | 13 (2.9) | 45 (2.9) | 541 | -3.8978 | 0.0021 | |||
Left parahippocampal gyrus | -23 (2.5) | 1 (1.6) | -23 (2.5) | 1484 | -3.8190 | 0.0024 | |||
Right anterior cingulate gyrus | 4 (2.6) | 33 (2.8) | 17 (2.8) | 1626 | -3.6557 | 0.0033 | |||
Right fusiform gyrus | 44 (2.1) | -23 (2.5) | -16 (3.1) | 447 | -3.6444 | 0.0034 | |||
Right insula | 33 (2.7) | 3 (2.6) | 4 (2.6) | 2681 | -3.5253 | 0.0042 | |||
Right medial frontal gyrus | 5 (1.1) | 5 (2.6) | 51 (2.3) | 1620 | -3.5222 | 0.0042 | |||
Right parahippocampal gyrus | 28 (3.1) | -1 (7.1) | -23 (4) | 1201 | -3.5175 | 0.0042 | |||
Left medial frontal gyrus | -6 (1.2) | 16 (2.6) | 47 (2.8) | 230 | -3.4475 | 0.0048 | |||
Left insula | -7 (2.6) | 3 (2.6) | -1 (2.6) | 2227 | -3.4176 | 0.0051 | |||
Left anterior cingulate gyrus | -5 (1.1) | 18 (2.3) | 39 (2.7) | 241 | -3.3100 | 0.0062 | |||
Right anterior STG | 47 (1.8) | -2 (4.4) | -7 (2.9) | 2402 | -3.2872 | 0.0065 |
STG, superior temporal gyrus
a. Clusters are displayed in the order of their t-values
b. P-values are corrected for multiple comparisons
Correlation with severity of thought disorder
We used a Spearman rank correlation analysis to study the relationship between severity of formal thought disorder (as measured by the TLC) and grey-matter volume. A total of seven brain regions showed significant correlations (P<0.01, corrected for multiple comparisons), indicating that grey-matter deficit increased along with the severity of thought disorder (Table 2 and Fig. 3).

Fig. 3 Maps of significant correlation (expressed as Spearman rank correlation coefficient r s) (P<0.01, corrected for multiple comparisons) at z=2 and z=20. These maps show a correlation between absolute grey-matter volume (upper row) and absolute cerebral blood flow (CBF; lower row) to the severity of formal thought disorder in the schizophrenia group.
Table 2 Significant clusters of linear correlation between grey-matter volume and severity of thought disorder in the schizophrenia group a

Talairach coordinates at centre of gravity, mean | Correlation of grey-matter volume and severity of formal thought disorder b | ||||||||
---|---|---|---|---|---|---|---|---|---|
Anatomical brain region | x | y | z | Voxels within cluster | Correlation coefficient c | P d | |||
Left superior temporal sulcus | -45 | -27 | 2 | 4190 | -0.8516 | 0.0002 | |||
Left anterior cingulate gyrus | -8 | 40 | 4 | 3345 | -0.8294 | 0.0005 | |||
Left angular gyrus | -45 | -62 | 25 | 3027 | -0.8211 | 0.0006 | |||
Left precuneus | -4 | -35 | 49 | 1420 | -0.8100 | 0.0008 | |||
Left posterior STG | -61 | -45 | 16 | 1591 | -0.7934 | 0.0012 | |||
Right anterior cingulate gyrus | 13 | 43 | 11 | 2910 | -0.7767 | 0.0018 | |||
Right precuneus | 8 | -31 | 45 | 1504 | -0.7767 | 0.0018 |
STG, superior temporal gyrus
a. Clusters are displayed in the order of their correlation coefficient value; results of the Spearman rank correlation analysis
b. Formal thought disorder measured with the Scale for the Assessment of Thought, Language and Communication
c. Spearman rank correlation coefficient r s
d. Corrected for multiple comparisons
Cerebral blood flow
Schizophrenia group v. controls
Voxel-wise statistical testing of CBF values in the schizophrenia and control groups revealed no significant difference (P<0.05) between the two groups after correcting for multiple comparisons. The global CBF value for the schizophrenia group was 57.2 ml/100 g per min (s.d.=25.1) and for the control group it was 58.2 ml/100 g per min (s.d.=17.7).
Correlation with severity of thought disorder
We performed a Spearman rank correlation analysis to search for brain regions showing an association between formal thought disorder severity (as measured by the TLC) and absolute CBF values. The hypothesis was that CBF would be correlated with behavioural state, as manifested in formal thought disorder. We found three brain regions with significant positive correlations (P<0.01, corrected for multiple comparisons), indicating that CBF increased along with the severity of formal thought disorder (Table 3 and Fig. 3). The individual scatter plots for TLC score and CBF for all three regions are presented in Fig. 4. We found no region with a negative correlation.
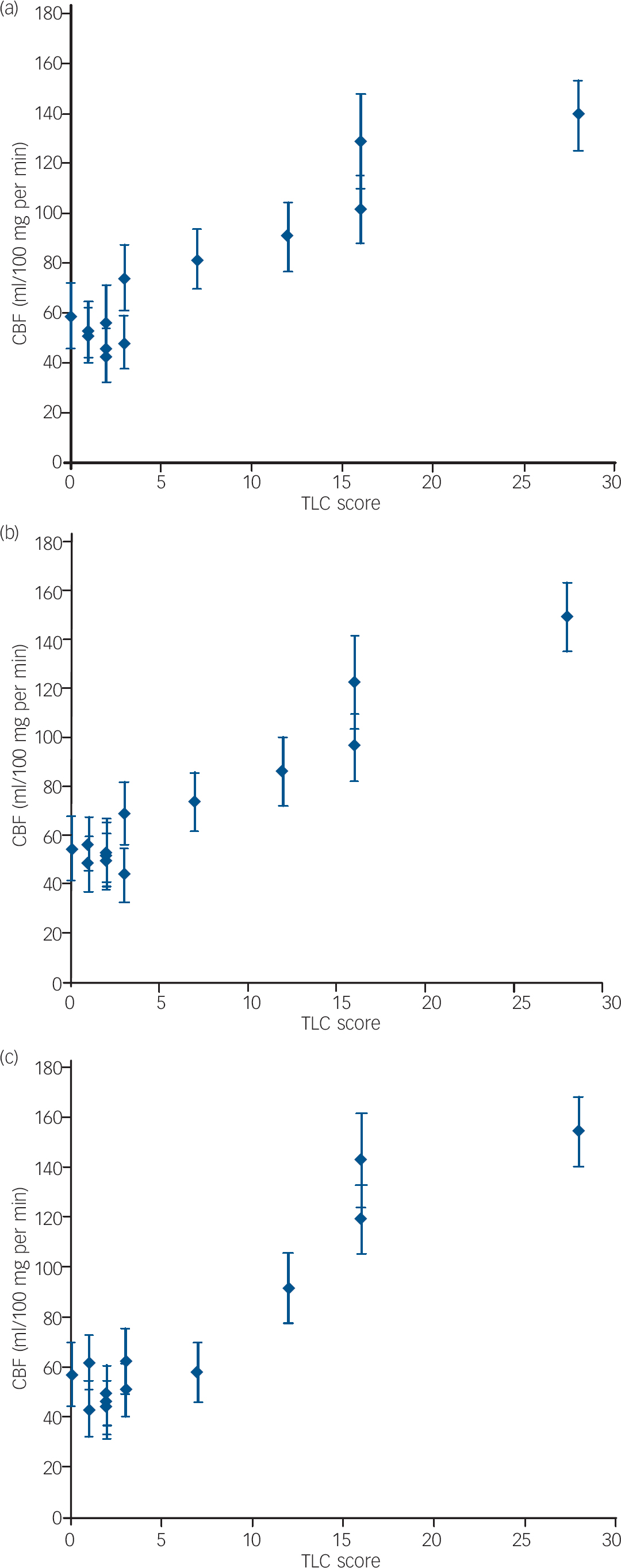
Fig. 4 Scatter plots of formal thought disorder severity, measured using the Scale for the Assessment of Thought, Language and Communication (TLC) and absolute cerebral blood flow (CBF) values (means plus standard deviations, measured using arterial spin labelling) for the three significant clusters presented in Table 3: (a) left posterior superior temporal gyrus, x=–50, y=–58, z=22; (b) left anterior insula, x=–35, y=23, z=2; (c) left inferior frontal gyrus, pars orbitalis, x=–33, y=36, z=3.
Table 3 Significant clusters of linear correlation between absolute cerebral blood flow at rest and the severity of formal thought disorder in the schizophrenia group a

Talairach coordinates at centre of gravity, mean | Correlation of CBF at rest and severity of formal thought disorder b | ||||||||
---|---|---|---|---|---|---|---|---|---|
Anatomical brain region | x | y | z | Voxels within cluster | Correlation coefficient c | p d | |||
Left anterior insula | -35 | 23 | 2 | 464 | 0.7656 | 0.0023 | |||
Left posterior STG/angular gyrus | -50 | -58 | 22 | 488 | 0.7462 | 0.0034 | |||
Left IFG pars orbitalis | -33 | 36 | 3 | 214 | 0.7407 | 0.0038 |
CBF, cerebral blood flow; IFG, inferior frontal gyrus; STG, superior temporal gyrus
a. Clusters are displayed in the order of their correlation coefficient value; results of the Spearman rank correlation analysis
b. Formal thought disorder measured with the Scale for the Assessment of Thought, Language and Communication
c. Spearman rank correlation coefficient r s
d. P-values are corrected for multiple comparisons
The CBF values for the control group in the same three brain regions were as follows: left IFG pars orbitalis (x=–33, y=36, z=3), 50.4 ml/100 g per min (s.d.=10.5); left posterior STG/angular gyrus (x=–50, y=–58, z=22), 52.3 ml/100 g per min (s.d.=10.3); left anterior insula (x=–35, y=23, z=2), 54.6 ml/100 g per min (s.d.=12.1).
Overlapping regions between CBF and grey-matter clusters
Results of the conjunction analysis of the relation of thought disorder to grey matter and to CBF respectively in the schizophrenia group showed overlapping regions located in the left posterior STG and in the left angular gyrus: x=–48 (s.d.=3), y=–68 (s.d.=3), z=22 (s.d.=1).
CBF correlation with grey-matter volume within overlapping regions
We performed regression analyses with the pooled CBF and the pooled grey-matter values and found a significant negative correlation between both entities: r=–0.71, P=0.0088.
Discussion
Our findings concerning overall grey-matter differences between the schizophrenia and control groups are consistent with previous voxel-based morphometry studies in schizophrenia (for review see Honea et al Reference Honea, Crow, Passingham and Mackay23 ), and are not further discussed.
Grey-matter deficit and thought disorder severity
Our data show that deficits in grey-matter volume in different brain regions are positively correlated with severity of formal thought disorder measured using the TLC. Specifically, we observed bilateral deficits in grey matter in the anterior cingulate gyrus and the precuneus. In addition, we identified deficits in the posterior STG, the posterior temporal sulcus and the angular gyrus in the left hemisphere. The volume deficit observed in the left STG is consistent with conventional volumetric studies demonstrating reduction of this region in patients with formal thought disorder. Reference Shenton, Kikinis, Jolesz, Pollak, LeMay, Wible, Hokama, Martin, Metcalf and Coleman5,Reference Vita, Dieci, Giobbio, Caputo, Ghiringhelli, Comazzi, Garbarini, Mendini, Morganti and Tenconi8,Reference Subotnik, Bartzokis, Green and Nuechterlein9 Further, we observed grey-matter deficits in the left angular gyrus, anterior cingulate gyrus and the precuneus.
Resting perfusion and thought disorder
A specific pattern of grey-matter atrophy alone cannot explain the transient character of formal thought disorder in schizophrenia. Therefore, we measured absolute resting perfusion as a potential state marker of formal thought disorder. The results show that its severity was positively correlated with resting perfusion in the left IFG (pars orbitalis), left posterior STG/angular gyrus and the left anterior insula. There was no negative correlation. Patients with severe formal thought disorder showed hyperperfusion in the mentioned regions compared with patients with schizophrenia who had mild or no formal thought disorder, and with healthy controls. This is consistent with previous data showing increased rCBF in the left STG Reference Liddle, Friston, Frith, Hirsch, Jones and Frackowiak12 and IFG, extending into the anterior insula Reference Lahti, Weiler, Holcomb, Tamminga, Carpenter and McMahon15 in patients with disorganisation syndrome. Moreover, in a SPECT study, the combination of formal thought disorder and grandiosity was associated with increased rCBF values in frontal and left parietal regions. Reference Sabri, Erkwoh, Schreckenberger, Owega, Sass and Buell14 None the less, the observation of hyperperfusion in the left angular gyrus is not consistent with findings of Liddle et al, who showed decreased perfusion in the bilateral angular gyrus among patients with disorganisation syndrome. Reference Liddle, Friston, Frith, Hirsch, Jones and Frackowiak12 This difference may, however, result from the syndrome approach of these authors in contrast to the single symptom severity approach of this study.
The interpretation of regional hyperperfusion is not trivial, since it can be functional or dysfunctional in terms of psychological performance. Studies of other clinical conditions, however, indicate that pronounced hyperperfusion is linked to dysfunction. In migraine, for example, clinical symptoms such as hemiplegia and transient aphasia occurred during initial cortical hyperperfusion. Reference May30 A second example of such dysfunction is postictal hyperperfusion, which is related to dysfunction of the affected brain regions in local epilepsy. Reference Leonhardt, de Greiff, Weber, Ludwig, Wiedemayer, Forsting and Hufnagel31 In line with these examples, we propose that the hyperperfusion in patients with schizophrenia and formal thought disorder in our study indicates local dysfunction.
Understanding the physiological function of the hyperperfused regions is essential to recognise their contribution to the dysfunctions in formal thought disorder. The most posterior part of the left STG and the left angular gyrus have a key role in providing access to semantic information. Reference Heim11 Lesions in the left posterior STG can cause informational disconnection between verbal and non-verbal domains of knowledge. Reference Saygin, Dick, Wilson, Dronkers and Bates32 The left angular gyrus is involved in higher-order conceptual knowledge and semantic processing (for review see Vigneau et al Reference Vigneau, Beaucousin, Herve, Duffau, Crivello, Houde, Mazoyer and Tzourio-Mazoyer33 ). Lesions of this region have been related to severe semantic – but not phonological – impairments. Reference Dronkers, Wilkins, Van, Redfern and Jaeger34 Dysfunction of the left posterior STG and angular gyrus may, therefore, result in disorders of semantic processing, as found in formal thought disorder. Recently, using event-related potentials, Kreher et al demonstrated increased spreading activation in semantic memory with increasing severity of formal thought disorder. Reference Kreher, Holcomb, Goff and Kuperberg35 These results are consistent with our findings of increasing perfusion in semantic areas with increasing severity of thought disorder. Both results may depend on enhanced neuronal activity in the semantic areas and, therefore, support the model of decreased cortical inhibition via a disturbance of gamma-aminobutyric acid (GABA) interneurons. Reference Lewis and Moghaddam36,Reference Daskalakis, Fitzgerald and Christensen37 These inhibitory interneurons play a part in shaping receptive fields and are important for organising cortical processes such as spreading activation. Reference Daskalakis, Fitzgerald and Christensen37,Reference Jones38 A selective loss of GABA interneurons may explain our finding of reduced grey matter. However, the issue of grey-matter volume loss in schizophrenia is controversial, owing to the multitude of possible causes. One could speculate that GABA interneuron loss in posterior temporal regions could ‘lock in’ local activation states that are subsequently propagated to other cortical regions to produce more widespread disruptions in generation of conversational language.
The posterior STG and angular gyrus are connected to the IFG by an intrahemispheric fibre bundle, the arcuate fasciculus. Reference Catani, Howard, Pajevic and Jones39 Therefore, structural and functional temporal impairments may involve frontal regions, including the left IFG. This could explain our finding that the temporal hyperperfusion was related to the severity of formal thought disorder in the left IFG (pars orbitalis). The finding is of particular interest in this context, since the left IFG is supposed to have a key role in selection and unification at different levels of language processing. Reference Hagoort40
In our study, the regions with hyperperfusion and grey-matter deficits that correlated with formal thought disorder overlapped in the left posterior STG and the left angular gyrus. In these regions we observed a negative correlation between CBF and grey matter – i.e. reduced grey matter was related to increased perfusion. In contrast, the left IFG showed hyperperfusion, but no grey-matter deficit correlated with formal thought disorder. Therefore, volume deficit may be a risk factor for decompensation of semantic processing in the left posterior STG and angular gyrus leading to a state-related hyperactivity in the controlling regions of the IFG via the arcuate fasciculus.
Changes in resting perfusion might alter the ability of functional activation of the described regions. McGuire et al showed a decrease of activation in the IFG and the left STG during a language production task in patients with thought disorder, Reference McGuire, Quested, Spence, Murray, Frith and Liddle2 and Kircher et al found decreased language-related blood oxygen level dependent (BOLD) response in the left posterior STG in patients with formal thought disorder. Reference Kircher, Liddle, Brammer, Williams, Murray and McGuire3 The location of Kircher's findings of reduced activation corresponds to the region of resting hyperperfusion in our patient group.
All hyperperfused regions that correlated with formal thought disorder were located in the left hemisphere. To understand this asymmetry and its possible implications for the aetiology of this disorder, the hemispheric differences in the language system must be addressed. Although language-related brain regions have been found in both hemispheres, language-related information is processed differently in the left- and right-sided language areas. Reference Jung-Beeman10 In particular, the temporoparietal junction has been found to be asymmetric in function and structure. Reference Coulson and Williams41 The left posterior STG is supposed to focus quickly on the dominant semantic context; at the same time, it inhibits irrelevant meanings. In contrast, the right posterior STG maintains weak, diffuse semantic activation, including distant and unusual semantic features that may be irrelevant to the context. The semantic fields in the right hemisphere thus provide only an approximate interpretation of the semantic information. Reference Jung-Beeman10 This view is supported by studies on the microstructure of language areas. The dendrites of pyramidal cells are longer and split into more branches in the right temporal lobe than in the left. Reference Hutsler and Galuske42 The microarchitecture in the right STG is, therefore, supposed to be amenable to more distant and ‘creative’ associations than its left-sided counterpart. Dysfunctions in the left-sided language system in schizophrenia in patients with formal thought disorder may lead to a compensatory use of right-sided language areas, resulting in more diffuse and coarse processing in language-related tasks, as has been suggested by Kircher et al. Reference Kircher, Liddle, Brammer, Williams, Murray and McGuire4 This may account for thought disorder symptoms such as loose associations, loss of goal, derailment and incoherence. Our study investigated formal thought disorder only in schizophrenia and therefore the findings cannot be generalised to this condition in other psychiatric disorders. If schizophrenic thought disorders can be related to the left hemispheric language system, however, they can be considered to be an expression of a phylogenetically recent and exclusively human pathology, which is consistent with the idea of schizophrenia being the price humans pay for language. Reference Crow43
Finally, we found two additional regions (anterior cingulate gyrus and the precuneus), that showed reduced grey-matter volume with increasing formal thought disorder but no irregularities in perfusion. The anterior cingulate gyrus is essential in error detection, Reference Van Veen and Carter44 a function that is disturbed in formal thought disorder. The precuneus is involved in visuospatial imagery, episodic memory and self-consciousness. Reference Cavanna and Trimble45 These functions are not directly related to the deficits observed in formal thought disorder, therefore the relevance of this finding for formal thought disorder remains open.
Limitations of the study
The correlation of the age distribution and the severity of formal thought disorder in the schizophrenia group was not significant but showed a trend (r=0.49, P=0.09). However, perfusion is expected to decrease and not increase with age. Reference Buijs, Krabbe-Hartkamp, Bakker, de Lange, Ramos, Breteler and Mali46 Therefore, our perfusion results cannot be attributed to an age effect. Grey-matter volumes also decrease with age; this has been previously described in normal ageing, especially in the frontal lobe. Reference Raz and Rodrigue47 The pattern of grey-matter alterations related to formal thought disorder presented here is not consistent with these ageing effects. However, Good et al showed grey-matter decreases with ageing in the left angular gyrus and anterior cingulate gyrus. Reference Good, Johnsrude, Ashburner, Henson, Friston and Frackowiak22 Thus, ageing might influence grey matter results in these regions.
Implications
Our data show that grey-matter deficits and resting hyperperfusion overlapped in the left posterior STG and the left angular gyrus. In addition, we found resting hyperperfusion in the left IFG. Local grey-matter deficit in the temporal language regions may be a risk factor for a state-related, dysfunctional hyperactivity of the entire left-sided language system, leading to formal thought disorder.
Acknowledgements
This study was funded by the Swiss National Science Foundation, grant 3200B0-100823.
eLetters
No eLetters have been published for this article.