INTRODUCTION
Holocene environmental changes and the interplay between natural conditions and human activities are intensely discussed research topics (Constante et al., Reference Constante, Peña, Muñoz and Picazo2010; Bellin et al., Reference Bellin, Vanacker and De Baets2013; Ackermann et al., Reference Ackermann, Greenbaum, Ayalon, Bar-Matthews, Boaretto, Bruins and Cabanes2014) that cover, for example, soil erosion, natural hazards, and settlement location decisions (Black et al., Reference Black, Adger, Arnell, Dercon, Geddes and Thomas2011). The complex causes of changing geomorphodynamics in Mediterranean landscapes have been debated for more than 50 years (Vita-Finzi, Reference Vita-Finzi1969; Butzer, Reference Butzer2005; Dusar et al., Reference Dusar, Verstraeten, Notebaert and Bakker2011; Walsh et al., Reference Walsh, Berger, Roberts, Vanniere, Ghilardi, Brown and Woodbridge2019; Jouffroy-Bapicot et al., Reference Jouffroy-Bapicot, Pedrotta, Debret, Field, Sulpizio, Zanchetta and Sabatier2021). The main issue is the degree to which geomorphological changes are triggered by environmental fluctuations (Avni et al., Reference Avni, Porat, Plakht and Avni2006; Jalut et al., Reference Jalut, Dedoubat, Fontugne and Otto2009; Roberts et al., Reference Roberts, Brayshaw, Kuzucuoğlu, Perez and Sadori2011), human decisions, and socioeconomic conditions (van Andel et al., Reference van Andel, Zangger and Demitrack1990; Remondo et al., Reference Remondo, Soto, González-Díez, Díaz de Terán and Cendrero2005; Roberts, Reference Roberts2019) or their complex interactions (Brisset et al., Reference Brisset, Miramont, Guiter, Anthony, Tachikawa, Poulenard and Arnaud2013; Verstraeten et al., Reference Verstraeten, Broothaerts, Van Loo, Notebaert, D'Haen, Dusar and De Brue2017; May et al., Reference May, Norpoth, Pint, Shumilovskikh, Raith, Brill and Rixhon2021). Hence, disentangling these related factors is not always straightforward (Fuchs, Reference Fuchs2007; Walsh et al., Reference Walsh, Berger, Roberts, Vanniere, Ghilardi, Brown and Woodbridge2019). Past human settlement strategies are, conversely, to some extent related to the geomorphic locations and processes (Kondolf and Piégay, Reference Kondolf, Piégay, Gregory and Goudie2011; Aucelli et al., Reference Aucelli, Cinque, Mattei, Pappone and Stefanile2018). River terraces and piedmonts, for example, are often preferred settlement locations (e.g., Kidder et al., Reference Kidder, Adelsberger, Arco and Schilling2008; Knitter, Reference Knitter2013; Lü et al., Reference Lü, Mo, Zhuang, Jiang, Liao, Lu, Ren and Feng2019).
In the eastern Mediterranean, the meta-analyses of Holocene terrestrial sediment archives indicate several phases of increased geomorphodynamics (Dusar et al., Reference Dusar, Verstraeten, Notebaert and Bakker2011; Walsh et al., Reference Walsh, Berger, Roberts, Vanniere, Ghilardi, Brown and Woodbridge2019), which especially occur during the periods when human impacts on the environment became more important. This general trend, however, might not be equally seen in all Mediterranean areas due to diverse settlement patterns and landscape sensitivities. A ground check in small valleys occupied by remote settlements and under the background of large urban centers could thus provide advanced and multilayered insights.
A suitable study area for such a ground check is the ancient city of Pergamon and its micro-region. As one of the most important and largest cities in Asia Minor (Pirson, Reference Pirson2017), Pergamon experienced a profound transformation from the Hellenistic Period to the Roman Imperial Period (300 BC–AD 300) (Pirson, Reference Pirson2020). During this time, the urban area of Pergamon doubled (Wulf, Reference Wulf1994) in tandem with a significant increase in population and an intensive public building program (Pirson, Reference Pirson2020). The population probably reached 180,000 people in the entirety of the Pergamon micro-region (Zimmermann, Reference Zimmermann2011). The contemporary demilitarization of Pergamon's hinterland increased the importance of rural settlements (Fig. 1), where possibly around 70% of the population lived (Pavúk and Horejs, Reference Pavúk and Horejs2018; Pirson, Reference Pirson2020; Laabs and Knitter, Reference Laabs and Knitter2021). In this micro-region, diverse archaeological sites (e.g., settlements, farms, and luxury agricultural estates) were located in different geomorphological settings, including plains, plain–mountain transitional areas, intra-mountain basins, and small tributary valleys (Horejs, Reference Horejs, Önen, Mutluer and Çetin2011a, Reference Horejs, Scholl and Pirson2014; Pirson, Reference Pirson2020, Reference Pirson2022). These landforms are potentially favorable for small settlements because of the proximity to fertile floodplains for agriculture and mountains for horticulture or pastoralism (Sommerey, Reference Sommerey, Pirson and Bachmann2008; Yang et al., Reference Yang, Becker, Knitter and Schütt2021). Rural areas were also highly influenced by the rising demand for agricultural products during the period of transformation (Knitter, Reference Knitter2013; Laabs and Knitter, Reference Laabs and Knitter2021). Hence, the investigation of rural catchments is integral to developing a complete picture of Pergamon's history on both very local and micro-regional scales.
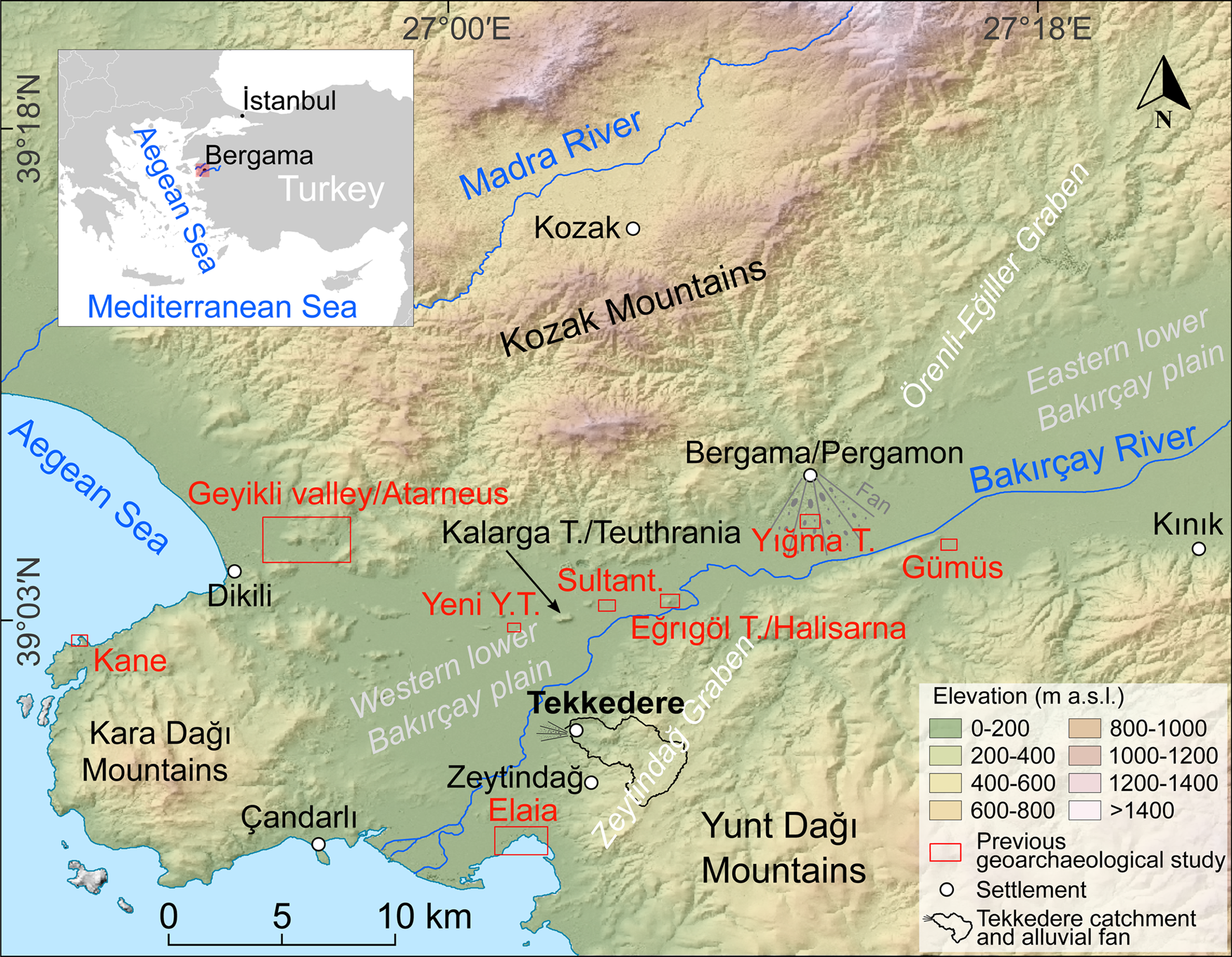
Figure 1. Topography and previous geoarchaeological study sites of the Pergamon micro-region. The inset map indicates the location of this study in the Aegean coastal region of western Turkey. Abbreviations: T., Tepe; Yeni Y.T., Yeni Yeldeğirmentepe; Sultant., Sultantepe.
Previous geoarchaeological studies in the micro-region have had different foci on selected archaeological sites, including the geomorphological investigation of prehistoric settlement locations in the Gümüş catchment (Knitter, Reference Knitter2013); the Geyikli valley (Schneider et al., Reference Schneider, Nykamp, Matthaei, Bebermeier and Schütt2013) and the landscape evolution around a hilltop settlement (Schneider et al., Reference Schneider, Matthaei, Bebermeier and Schütt2014); Bakırçay floodplain dynamics related to archaeological sites (Schneider et al., Reference Schneider, Matthaei, Schlöffel, Meyer, Kronwald, Pint and Schütt2015, Reference Schneider, Schlöffel, Schwall, Horejs and Schütt2017); the ancient harbor city of Elaia, with studies of its development and paleoenvironmental conditions (Seeliger et al., Reference Seeliger, Bartz, Erkul, Feuser, Kelterbaum, Klein, Pirson, Vött and Brückner2013, Reference Seeliger, Brill, Feuser, Bartz, Erkul, Kelterbaum, Vött, Klein, Pirson and Brückner2014, Reference Seeliger, Pint, Frenzel, Feuser, Pirson, Riedesel and Brückner2017, Reference Seeliger, Pint, Feuser, Riedesel, Marriner, Frenzel, Pirson, Bolten and Brückner2019; Pint et al., Reference Pint, Seeliger, Frenzel, Feuser, Erkul, Berndt, Klein, Pirson and Brückner2015; Shumilovskikh et al., Reference Shumilovskikh, Seeliger, Feuser, Novenko, Schlütz, Pint, Pirson and Brückner2016); the marine seismic investigation of the ancient harbor of Kane (Fediuk et al., Reference Fediuk, Wilken, Wunderlich, Rabbel, Seeliger, Laufer and Pirson2019); and geophysical investigations on a monumental tumulus (Mecking et al., Reference Mecking, Meinecke, Erkul, Driehaus, Bolten, Pirson and Rabbel2020).
Notwithstanding this relatively intensive research program, no study in the micro-region covers a continuously occupied settlement chamber that allows for the reconstruction of local geomorphodynamics in the longue durée. In addition, the micro-region shows remarkable diversities in relief, drainage network, and sediment accumulation in different landscape units (Yang et al., Reference Yang, Becker, Knitter and Schütt2021), but not all of these landforms have been geoarchaeologically studied. The metadata analyses of 14C ages from the western Pergamon micro-region suggest past geomorphodynamics are more or less associated with the general—but spatially more fragmented—settlement history of the region (Becker et al., Reference Becker, Knitter, Nykamp and Schütt2020a). Hence, additional research, especially in the Yunt Dağı Mountains (the southern part of the micro-region), is required to fill this gap and develop a deeper understanding of the whole area.
Tekkedere catchment was therefore selected as our study area to understand human–environment interactions in the Pergamon micro-region. Archaeological remains suggest the lower Tekkedere valley was continuously settled since the Middle Bronze Age (since ca. 1700 BC) (Ludwig, Reference Ludwig and Pirson2020a; Michalski, Reference Michalski2021). Its settlement history thus covers the main phases of intensified human impacts on Mediterranean landscapes during the Late Holocene (Dusar et al., Reference Dusar, Verstraeten, Notebaert and Bakker2011).
As the backbone of geoarchaeology, sediments and soils hold enormous amounts of information concerning landscape evolution and human usage history that can be accessed through various sediment analyses and chronological datings (Goldberg and Macphail, Reference Goldberg and Macphail2006). Different sediment archives might react separately to certain triggers on multiple temporal and spatial scales (e.g., Goldberg and Macphail, Reference Goldberg and Macphail2006; Dusar et al., Reference Dusar, Verstraeten, Notebaert and Bakker2011; Nykamp et al., Reference Nykamp, Becker, Braun, Pöllath, Knitter, Peters and Schütt2021). Among them, alluvial (and colluvial) deposits near ancient settlements in lower-order watersheds hold the benefit of a more direct interpretation of causal relationships between human disturbance and sediment accumulation (Dotterweich, Reference Dotterweich2008; Dusar et al., Reference Dusar, Verstraeten, Notebaert and Bakker2011). With the availability of archaeological data, climatic and anthropogenic influences on geomorphodynamics can be more easily differentiated (Pérez-Lambán et al., Reference Pérez-Lambán, Peña-Monné, Badía-Villas, Picazo Millán, Sampietro-Vattuone, Alcolea Gracia, Aranbarri, González-Sampériz and Fanlo Loras2018).
Our main objectives are (1) reconstructing the geomorphodynamics of the Tekkedere valley and its alluvial fan using sediment analyses and radiocarbon dating and (2) elucidating the potential drivers of the increasing geomorphodynamics, including the regional climate change and the local human impact (changing settlement pattern), in relation to supraregional Holocene records. The term “geomorphodynamics” used in this study refers to the surface changes of the Earth and related processes (Sauer and Ries, Reference Sauer and Ries2008; Becker et al., Reference Becker, Knitter, Nykamp and Schütt2020a). In particular, it covers erosion and deposition in the terrestrial environments in our study.
Study area
Natural setting
Tekkedere catchment (ca. 13 km2 drainage basin area) is a mountainous sub-catchment (second order) of the western lower Bakırçay River catchment (Fig. 1). The Bakırçay catchment (3382 km2) is under the territory of the Pergamon micro-region (Yang et al., Reference Yang, Becker, Knitter and Schütt2021). It is one of the most fundamental river networks in Aegean Anatolia (Kayan, Reference Kayan1999; Yang et al., Reference Yang, Becker, Knitter and Schütt2021). The modern climate is a typical Mediterranean subhumid climate that is characterized by dry and hot summers and mild and wet winters (Peel et al., Reference Peel, Finlayson and McMahon2007). The average annual precipitation was 749 mm and the temperature was 14.7°C during 1981–2010 in Bergama (the modern name of Pergamon) (Yang et al., Reference Yang, Becker, Knitter and Schütt2021).
The Tekkedere catchment is adjacent to the major route network between the city of Pergamon and its ancient harbor of Elaia (Pirson, Reference Pirson and Jöchner2008; Ludwig, Reference Ludwig2020b) with short travel distances (around 18 km and 7 km, respectively) (Fig. 1). Modern Tekkedere village is also close to ancient settlements of Teuthrania (Kalarga Tepe, ca. 8 km in the north) (Gehrke, Reference Gehrke, Scholl and Pirson2014; Zimmermann et al., Reference Zimmermann, Matthaei, Ateş, Matthaei and Zimmermann2015; Williamson, Reference Williamson, McInerney and Sluiter2016), Halisarna (Eğrigöl Tepe, ca. 9 km in the northeast) (Pirson, Reference Pirson and Jöchner2008; Zimmermann et al., Reference Zimmermann, Matthaei, Ateş, Matthaei and Zimmermann2015; Pavúk and Horejs, Reference Pavúk and Horejs2018), and the ancient polis of Atarneus (16 km in the northwest) (Pirson and Zimmermann, Reference Pirson, Zimmermann, Scholl and Pirson2014; Zimmermann et al., Reference Zimmermann, Matthaei, Ateş, Matthaei and Zimmermann2015).
Geologically, the Pergamon micro-region is located in the western Extensional Province of Anatolia, which is regarded as one of the most rapidly extending regions on Earth (Özpolat et al., Reference Özpolat, Yıldırım, Görüm, Gosse, Şahiner, Şarıkaya and Owen2022). The NE-striking Miocene Zeytindağ and Örenli–Eğiller Grabens and the Plio-Pleistocene Bergama Graben formed under tectonic stress (Bozkurt, Reference Bozkurt2003; Yang et al., Reference Yang, Becker, Knitter and Schütt2021). Subsidence of the grabens of Zeytindağ and Bergama (Bakırçay) is ongoing, at a rate lower than 1 mm/yr, which has resulted in a steadily rising local sea level during the Holocene (Seeliger et al., Reference Seeliger, Pint, Frenzel, Feuser, Pirson, Riedesel and Brückner2017). Part of the Zeytindağ Graben (Yilmaz et al., Reference Yilmaz, Genç, Gürer, Bozcu, Yilmaz, Karacik, Altunkaynak, Elmas, Bozkurt, Winchester and Piper2000; Fig. 1), the upper Tekkedere catchment, is characterized by slightly rolling plains and shallow depressions (Yang et al., Reference Yang, Becker, Knitter and Schütt2021). Within the catchment, Pleistocene tectonics formed NE-trending strike-slip faults (Yilmaz et al., Reference Yilmaz, Genç, Gürer, Bozcu, Yilmaz, Karacik, Altunkaynak, Elmas, Bozkurt, Winchester and Piper2000; Emre et al., Reference Emre, Doğan and Özalp2011a, Reference Emre, Özalp and Duman2011b); more (unspecified or inferred) faults are reported with the NE or NW trend (modified after Yang et al. [Reference Yang, Becker, Knitter and Schütt2021] based on MTA [2002]; Fig. 2B). The bedrock in the catchment is composed of Miocene continental clastic rocks (ca. 61% of the catchment), Permian carbonate rocks with occasionally occurring clastic rocks (ca. 38% of the catchment), and pyroclastic rocks (ca. 1% of the catchment) (MTA, 2002; Fig. 2B). Reddish-brown and red Mediterranean soils develop on the clastic and pyroclastic rocks, whereas Rendzinas form on the carbonate rocks (Danacıoğlu and Tağıl, Reference Danacıoğlu and Tağıl2017).

Figure 2. Overview of the Tekkedere area. (A) Geomorphological map of the Tekkedere area with locations of the sediment profiles and settlements (Ludwig, Reference Ludwig and Pirson2019, Reference Ludwig and Pirson2020a; Michalski, Reference Michalski2021). (B) Geological map of the Tekkedere area (modified after Yang et al. [Reference Yang, Becker, Knitter and Schütt2021] based on MTA [2002] and Emre et al. [Reference Emre, Doğan and Özalp2011a, Reference Emre, Özalp and Duman2011b]). (C) Schematic cross-section through the Tekkedere creek B (located at the sediment profile Tek-3).
Tekkedere creek encompasses several intermittent drainage systems (Fig. 2A) with a torrential flow regime that are similar to the other small rivers along the Aegean coast (Kayan and Vardar, Reference Kayan, Vardar, Lambrianides and Spencer2007). There are two major creeks in the catchment. Creek A, the longest drainage way in the valley, flows from the intramountainous basin of the headwater area to the gorge, south of Kuyulu Kaya Tepe (summit ca. 160 m above sea level [m asl]). Creek B drains the northern part of the catchment (east of the Yıldız Tepe, summit ca. 210 m asl) and joins with creek A west of the Kuyulu Kaya Tepe. They continue westward as the Tekkedere creek. The creek is channelized on its alluvial fan and drains into the Bakırçay River just 12 km upstream of its mouth into the Aegean Sea.
The slopes of the lower valley (including the valley of creek B) are asymmetrical (Ludwig, Reference Ludwig and Pirson2019; Fig. 2C). The northern slopes are steeply inclined (16–37°, mean = 20°), whereas the southern slopes are gentler (10–30°, mean = 15°). Strong denudation and soil erosion are the prevailing geomorphological processes on the southern slopes, partly confined by agricultural terraces grown with olives (Becker et al., Reference Becker, Knitter, Yang, Schütt and Pirson2020b; Fig. 2A and C). Where soils are fully eroded, scattered bare rocks crop out, mainly on the southern slopes (Ludwig, Reference Ludwig and Pirson2019; Fig. 2A). The Kuyulu Kaya Tepe has large areas of outcropping bedrock with screes covering its southern footslopes.
Locally, the tributaries of Tekkedere creek are incised for more than 1 m into the valley infills (Fig. 2C). The valley bottom widens in its lower course where the modern Tekkedere village is built on its right riverbank (Fig. 2A). The Tekkedere alluvial fan forms at the opening area from the valley to the Bakırçay floodplain, covers an area of ca. 2 km2 (length: ca. 2 km; width: ca. 1.4 km), and is the largest alluvial fan in the foreland of the western Yunt Dağı Mountains. The altitude difference from the fan's apex to its toe amounts to 18 m, which is calculated based on TanDEM-X data (Wessel et al., Reference Wessel, Huber, Wohlfart, Marschalk, Kosmann and Roth2018). At present, the toe of the fan is located only ca. 500 m east of the current Bakırçay River channel (Fig. 2A).
While arable lands with vegetable and grain cropping dominate the land use in the headwater area of Tekkedere valley, coniferous forests, transitional woodland–shrubland covers, and olive groves on agricultural terraces characterize the vegetation cover on the slopes of the middle valley (Supplementary Fig. 1 based on Corine Land Cover data [Cole et al., Reference Cole, Smith and Balzter2018] and field observations). In the lower part, pine forests cover the northern slopes (Yıldız Tepe), whereas olives are mainly cultivated on the southern slopes and the valley bottom. Olive cultivation characterizes the upper part of the Tekkedere alluvial fan. Downslope, at the fan's apex, At the middle and distal fan, cotton and vegetable cropping predominates, and fig and citrus fruit trees occur sporadically.
A palynological study around Elaia indicates the vicinity of Tekkedere was dominated by natural deciduous oak forests between 7.6 and 3 ka BP (Shumilovskikh et al., Reference Shumilovskikh, Seeliger, Feuser, Novenko, Schlütz, Pint, Pirson and Brückner2016). Deforestation occurred as a consequence of the increased layout of olive groves and the development of macchia shrublands under rising grazing pressure since the Hellenistic Period, reaching a maximum during 2.1–1.8 ka BP. Alongside the hillslopes, semi-natural forests (mainly pines) developed during the past millennium (Shumilovskikh et al., Reference Shumilovskikh, Seeliger, Feuser, Novenko, Schlütz, Pint, Pirson and Brückner2016). The abundance of grains and the fertility of the area were described by ancient geographers and historians (Sommerey, Reference Sommerey, Pirson and Bachmann2008; Pirson and Zimmermann, Reference Pirson, Zimmermann, Scholl and Pirson2014). Recent studies, however, suggest that Pergamon's demand for grain, at least in the Roman Imperial Period, could not be covered without imports or environmental modifications (e.g., agricultural terracing) (Kobes, Reference Kobes1999; Günther et al., Reference Günther, Clemen, Duttmann, Schütt and Knitter2021; Laabs and Knitter, Reference Laabs and Knitter2021).
Settlement history
Since 2018, archaeological surveys have been conducted in the Tekkedere catchment by the German Archaeological Institute (Deutsches Archäologisches Institut [DAI]) (Ludwig, Reference Ludwig and Pirson2019, Reference Ludwig and Pirson2020a). Their findings provide evidence of at least six archaeological sites in the catchment (Fig. 2A), indicating almost uninterrupted settlement continuity and changing settlement patterns since the late Middle Bronze Age (ca. 1700 BC) (Ludwig, Reference Ludwig and Pirson2019, Reference Ludwig and Pirson2020a; Michalski, Reference Michalski2021).
Ceramic remains on and around the hill site of Kuyulu Kaya Tepe (Um19 Fst 02) (Fig. 2A) indicate settlement activities continued from the late Middle Bronze Age to the end of the Early Byzantine Period (ca. 3.7–1.2 ka BP) (Ludwig, Reference Ludwig and Pirson2019; Michalski, Reference Michalski2021). Building remains, including several cisterns, have been found on the rock plateau (Ludwig, Reference Ludwig and Pirson2019). A small rural settlement that probably reached its peak during Classical antiquity was situated at the footslopes of Kuyulu Kaya Tepe. Considering that the Kuyulu Kaya Tepe surmounts the surroundings by ca. 40 m and is characterized by steep slopes with outcropping bedrock toward creek A, the site, especially the hill site on the rock plateau, might have been used as a fortress to overlook or control the entire Tekkedere valley and large parts of the western lower Bakırçay plain (Ludwig, Reference Ludwig and Pirson2020a; Fig. 2A).
Two small hilltop sites (UM19 Fst 04 and 13), a few hundred meters to the north and west of the Kuyulu Kaya Tepe along creek B (Fig. 2A) with a high concentration of ceramics and bricks, suggest human activities took place during Classical antiquity (Ludwig, Reference Ludwig and Pirson2020a).
The predecessor settlement of the modern Tekkedere village was likely first established as the upper settlement (Yukarı Kışlak; UM18 Fst 01 or UM19 Fst 09), ca. 1 km north of the Kuyulu Kaya Tepe along creek B (Ludwig, Reference Ludwig and Pirson2019; Fig. 2A). Based on the archaeological results, the upper settlement duration is assumed to have been from late antiquity to the Byzantine Period (Ludwig, Reference Ludwig and Pirson2020a). The settlement was replaced by the smaller lower settlement (Aşağı Kışlak; UM19 Fst 14) which was situated in the middle of the lower valley (Ludwig, Reference Ludwig and Pirson2020a; Tozan, Reference Tozan and Pirson2022; Fig. 2A). When the lower settlement was abandoned, the old Tekkedere settlement (Eski Tekkedere) was built farther downstream (Tozan, Reference Tozan and Pirson2022). The old Tekkedere settlement was given up mostly due to an earthquake-triggered landslide, which was previously assumed to occur in the middle of the nineteenth century (Ludwig, Reference Ludwig and Pirson2020a). Based on the information provided by the locals, it was the earthquake that took place around Dikili, Zeytindağ, and Bergama in 1939 (Paradisopoulou et al., Reference Paradisopoulou, Papadimitriou, Karakostas, Taymaz, Kilias and Yolsal2010; Çelik et al., Reference Çelik, Karacakaya, Mete, Genç and Bal2019). Residents left and developed the modern Tekkedere village, which is built directly to the west of the old Tekkedere settlement (Ludwig, Reference Ludwig and Pirson2020a).
METHODS
Fieldwork
Three sediment outcrops along the banks of creek B were described and sampled in September 2019 (Becker et al., Reference Becker, Knitter, Yang, Schütt and Pirson2020b), and five sediment cores were extracted and brought to the surface along a transect from the apex to the toe of the alluvial fan by vibracoring (Wacker BHF 30 S, with 5-cm-diameter open cores) in October 2020 (Becker et al., Reference Becker, Yang, Nykamp, Doğan, Schütt and Pirson2022; Fig. 2A, Table 1). None of the profiles reached the underlying bedrock.
Table 1. Metadata of sediment outcrops in the valley (n = 3) and drilling cores on the alluvial fan (n = 5) of the Tekkedere catchment (WGS 84).

Lithostratigraphy was documented as comprising sediment color (Munsell soil color), unit thickness, sediment depositional structure (Stow, Reference Stow2005), sediment texture and fabric (Blair and McPherson, Reference Blair and McPherson1999), hydromorphic feature, biological modification, and occurrence of artifacts (Jahn et al., Reference Jahn, Blume, Asio, Spaargaren and Schad2006). The degree of reaction to 9.9% HCl was applied as an indicator for the amount of calcium carbonate (CaCO3) (Jahn et al., Reference Jahn, Blume, Asio, Spaargaren and Schad2006). A summary classification of the sediment description can be found in Yang et al. (Reference Yang, Becker, Nykamp and Schütt2022). Sediment samples (3- to 5-cm-thick pieces, n = 191) for geochemical analyses were collected according to the unit thickness and their position to unit. Sediment profiles were carefully checked for charcoal pieces and bulk samples containing organic matter for radiocarbon dating.
Laboratory work
Sediment analyses
Geochemical analyses of sediments can effectively assist in distinguishing the occurrence of past soil formation and the difference in natural and cultural processes (Terry, Reference Terry and Gilbert2017). Sediment samples were analyzed in the laboratory of the Bergama archaeological excavation house. Before the measurement, samples were air-dried for more than 48 h, and the aggregates were crushed in a porcelain mortar. For subsequent analyses, the fine fraction (Ø < 2 mm) was separated from the coarse component by dry sieving.
The pH and electrical conductivity (EC) values were obtained in the water-saturated sediments (1:2.5 solutions of 5 g of sediment and 12.5 mL of distilled water) with a TDS/EC/pH/TEMP meter (EZ9908; resolution: 0.01 pH, 1 μS/cm EC). Reaction class terms and the pH range are based on the soil survey manual (Soil Science Division Staff, 2017; Table 2). The pH value in sediment solutions is highly related to the buffering process of organic acids and is indicative of paleosol occurrence (Nykamp et al., Reference Nykamp, Knitter and Schütt2020) and human activity (Terry, Reference Terry and Gilbert2017). The EC value provides a hint of readily soluble salts that crystallize from chemical weathering or soil water evaporites (Nykamp et al., Reference Nykamp, Knitter and Schütt2020).
Table 2. The equal interval classification of the sediment proxies (except pH; Soil Science Division Staff, 2017).a

aEC, electrical conductivity; LOI550, loss on ignition at 550°C; XLF, mass-specific susceptibility at the low frequency.
Approximately 10 g of oven-dried (105°C) sediments were measured using loss on ignition at 550°C (LOI550) (Dean, Reference Dean1974; Heiri et al., Reference Heiri, Lotter and Lemcke2001). LOI550 was calculated as the weight difference of a sample treated at both temperatures and is used for the estimation of organic matter content (Heiri et al., Reference Heiri, Lotter and Lemcke2001; Santisteban et al., Reference Santisteban, Mediavilla, López-Pamo, Dabrio, Zapata, García, Castaño and Martínez-Alfaro2004). The in-lab reference material was measured after every 20 sample batch to control variability, which is reproducible with a median LOI550 value of 5.6 mass% (median absolute deviation [MAD]: 0.2 mass%). Often, a higher LOI550 value is associated with oxidation accumulation, pointing to soil horizons, the stability of land surface, and the intensity of human activity (Goldberg and Macphail, Reference Goldberg and Macphail2006; Panin et al., Reference Panin, Filippova, Bukhonov, Karpukhina, Kalinin and Ruchkin2021).
The magnetic susceptibility of sediments was measured with a Bartington magnetic susceptibility system (MS3 meter and MS2B dual-frequency sensor; measuring resolution: 2 × 10-6 SI; range: 26 SI) using the Bartsoft software. The volume-specific magnetic susceptibility (κ) at low frequency (LF, 0.46 kHz) of samples weighed using 12 cm3 plastic pots was determined with triple repetition. The mass-specific susceptibility at the low frequency (XLF, 10−8 m3/kg) was calculated according to Dearing (Dearing, Reference Dearing1994; Dearing et al., Reference Dearing, Dann, Hay, Lees, Loveland, Maher and O'Grady1996). The confidence intervals of sample median XLF values were estimated by simple quantile bootstrapping (B = 1000) in R v. 3.6 (R Core Team, 2021). In addition, we used the MS2B meter to measure the Tek-5 samples (n = 48) five times. The results are highly comparable to 88% of samples used via the MS3 meter (correlation of the sediment measured in both meters: median = 0.97). The median of the volume-specific susceptibility of the 10 mL calibration sample is 2620 × 10−5 SI (MAD: 0.25× 10−5 SI; accuracy: 1%). Higher XLF values are generally related to the formation of clay minerals under a higher weathering process (Maher and Thompson, Reference Maher and Thompson1991; Blundell et al., Reference Blundell, Dearing, Boyle and Hannam2009; Sherriff, Reference Sherriff, Cook, Clarke and Nield2014).
The elemental composition of sediment samples from the profiles of Tek-5, Tek-5-2, and Tek-6 was analyzed using a portable energy-dispersive X-ray fluorescence spectrometer (p-ED-XRF; Thermo Fisher Scientific NITON XL3t) measuring each sample for 120 s in the manufacturer's Mining Cu/Zn mode with main, high, low, and light filters (50, 50, 15/20, and 8 kV, respectively). The certified reference material (LKSD-4) (Lynch, Reference Lynch1990) and the acid-purified silica sand were taken as standards to ensure measurement quality. The reproducibility of selected elements (Zr, Rb, Ti, and Ca) for LKSD-4 and silica sand are in ranges of 22–34% and 6–11%, respectively. Two element ratios were calculated for sediment characterization. The Zr/Rb ratio is used as a proxy for grain-size variability (Dinelli et al., Reference Dinelli, Tateo and Summa2007) and the Ca/Ti ratio as an indicator for synsedimentary weathering as well for the precipitation of secondary carbonates (Ülgen et al., Reference Ülgen, Franz, Biltekin, Çagatay, Roeser, Doner and Thein2012).
The sediment proxies in this study are reported in median and MAD (in parentheses) values and are grouped using an equal interval classification of the data (Table 2). Raw/processed data and R code (R Core Team, 2021) can be found in the data set in Pangaea (Yang et al., Reference Yang, Becker, Nykamp and Schütt2022).
Radiocarbon dating
In our study, radiocarbon dating would be the optimum solution for the development of a chronological framework due to the scarcity of available materials for alternative methods. Accelerator mass spectrometry (AMS) radiocarbon dating (14C) of samples was conducted at the TÜBİTAK National 1 MV AMS Laboratory (Doğan et al., Reference Doğan, İlkmen and Kulak2021). Dated materials (n = 20) include charcoals, the mixture of mainly charcoal with adjacent sediments (if %C of the macroscopically separatable charcoal is <1–2 mg), organic-rich bulk samples, and one terrestrial snail shell (Table 3).
Table 3. The AMS-14C dating samples from the Tekkedere valley (n = 6) and Tekkedere alluvial fan (n = 14), pretreatment procedure, sediment facies of the sample location and their dating results and calibration (calibration curve: IntCal20; Reimer et al., Reference Reimer, Austin, Bard, Bayliss, Blackwell, Bronk Ramsey and Butzin2020).

Macroscopic plants and rootlets were removed from the samples before the standard pretreatment procedure (acid-base-acid [ABA] protocol) was applied (Fowler et al., Reference Fowler, Gillespie and Hedges1986; Bronk Ramsey, Reference Bronk Ramsey2008; Brock et al., Reference Brock, Higham, Ditchfield and Ramsey2010). Fragile charcoal remnants with an amount of charcoal below ca. 10 mg were only treated with an acid wash (Fowler et al., Reference Fowler, Gillespie and Hedges1986; Brock et al., Reference Brock, Higham, Ditchfield and Ramsey2010). The radiocarbon ages were calibrated via the IntCal20 calibration curve (Reimer et al., Reference Reimer, Austin, Bard, Bayliss, Blackwell, Bronk Ramsey and Butzin2020) and the rcarbon package (Crema and Bevan, Reference Crema and Bevan2021) in R v. 3.6 (R Core Team, 2021). We report a 95% probability range for the calibrated ages.
SEDIMENT CHARACTERISTICS
The analyzed sediment profiles are located in two different landforms: the valley infill of the tributary creek B and the Tekkedere alluvial fan (Table 1). Profile Tek-2 from the valley infill and profiles Tek-5 and Tek-6 from the fan are taken as key profiles and are presented in detail. Additional profiles provided in the Supplementary Material show comparable sediment characteristics. The calculation of the median value of sediment proxies from different profiles’ sedimentary units is provided in the data set (Yang et al., Reference Yang, Becker, Nykamp and Schütt2022). Taken together, the samples’ ages range between ca. 6.6 and 0.3 cal ka BP (Table 3).
Tekkedere valley infill: key profile Tek-2
Profile Tek-2 has a total thickness of 158 cm, and its six lithostratigraphic units are dominated by alternating units of silts/sands and pebbles (Fig. 3). Except for the upper two units (units 5 and 6), lithostratigraphic units 1–4 show sharp contacts. Roots occur commonly above 100 cm below surface (cm bs). The sediment matrix is strongly calcareous and moderately alkaline (pH: 8.1 (0.1)). EC values (EC: 95 (10) μS/cm) are very low (class 1; Table 2) and only slightly increased in unit 4. XLF (24 (9) × 10−8 m3/kg, class 1) and LOI550 (4.1 (0.6) mass%, class 3) peak in the same unit.

Figure 3. The lithostratigraphy, radiocarbon dates, and sedimentological analyses of sediment outcrop Tek-2. The color of the sediment texture column represents the color observed in the field; the shaded band represents the different units and the dashed line refers to the depth in every meter. cm bs, cm below surface; EC, electrical conductivity; LOI550, loss on ignition at 550°C; XLF, mass-specific susceptibility at the low frequency.
Basal unit 1 (158–135 cm bs) is characterized by yellowish-brown, highly compacted silty sand with few embedded medium pebbles (Ø < 2 cm). Some pebbles show a grayish weathering rind.
Unit 2 (135–116 cm bs) shows clast-supported fabric. The clasts consist of yellowish-brown, disorganized pebbles with few fine cobbles (Ø < 11 cm). The matrix is sandy silt with adhesions of secondary precipitated carbonates and very few fine roots.
Unit 3 (116–84 cm bs) is characterized by normally graded fabric. The sediment texture changes from strong yellowish silty sand with few angular, disorganized fine to coarse pebbles (Ø < 3 cm) at the base of the unit, to light gray silty sand at the top. Clasts are partly carbonated and slightly weathered. Very few fine to coarse roots occur throughout the unit. The analytical values of sediment samples (n = 4) from units 1–3 remain roughly constant. Sediments are moderately alkaline (pH: 8.2 (0)). EC (94 (4) μS/cm, class 1) and XLF (14 (3) × 10−8 m3/kg, class 1) are very low. LOI550 (3.4 (0.2) mass%, class 2) is low.
Unit 4 (84–65 cm bs) is composed of light grayish, slightly sandy silt with few fine pebbles. Fine roots and burrows are abundant. Sediments are moderately alkaline and enhanced in solutes (EC: 128 (9) μS/cm, class 1, n = 3). XLF amounts to 38 (0) × 10−8 m3/kg (class 2, n = 3), and LOI550 totals to 4.9 (0.5) mass% (class 4, n = 3). Two charcoal pieces (at 65 cm bs and 75–80 cm bs) date unit 4 to around 908–732 cal yr BP (Fig. 3, Table 3).
Unit 5 (65–32 cm bs) is characterized by light grayish, silty sand with embedded pebbles to fine cobbles (Ø < 7 cm). A thin rooted clast-supported layer of fine pebbles is interbedded at 65–60 cm bs A shell of a land snail occurs at 55 cm bs EC, XLF, and LOI550 values (n = 2) widely correspond to the values of units 1–3.
Unit 6 (32–0 cm bs) corresponds to the modern plow horizon. It consists of dark to light brown sandy silt with very few fine to coarse pebbles (Ø < 3 cm). The unit has abundant roots and common burrows. The samples (n = 2) show slightly decreased pH values (7.9 (0.1)) and increased XLF (37 (13) × 10−8 m3/kg, class 2) and LOI550 (5.5 (0.8) mass%, class 4) values.
Tekkedere mid-fan: key profile Tek-5
Profile Tek-5 was obtained from the middle position of the Tekkedere alluvial fan (Fig. 2A). The extracted 786-cm-thick sediment sequence is divided into eight units (Fig. 4). The lower part of the profile (below 426 cm bs, units 1–4) is mainly characterized by layers of dark grayish or brownish silts with very few embedded fine pebbles, whereas units of brownish silts and sands with variable contents of pebbles prevail in the upper half of the profile (units 5–8). Concentrations of secondary precipitated carbonates and manganese mottles or small nodules occur almost throughout the whole profile.

Figure 4. The lithostratigraphy, radiocarbon dates, and sediment analyses of sediment core Tek-5. The confidence intervals of the sample median mass-specific susceptibility at the low frequency (XLF) values are estimated by simple quantile bootstrapping (B = 1,000). cm bs, cm below surface; EC, electrical conductivity; LOI550, loss on ignition at 550°C.
Sediment samples (n = 48) in the profile Tek-5 are slightly to strongly alkaline (pH: 8.0 (0.1)). EC indicates an overall very low amount of solutes (195 (43) μS/cm, class 1, n = 48) with slightly increased values in units 1–4 (237 (35) μS/cm, class 2, n = 25). XLF shows a median value of 27 (7) × 10−8 m3/kg (class 1, n = 48) with relatively low results in units 1–4 (20 (8) × 10−8 m3/kg, class 1, n = 25) and relatively high results in units 5–8 (32 (7) × 10−8 m3/kg, class 2, n = 23). LOI550 has a median value of 3.5 (0.9) mass% (class 3, n = 48) in the profile, with slightly higher values in units 1–4 (4.4 (0.7) mass%, class 3, n = 25) than in units 5–8 (3.2 (0.4) mass%, class 2, n = 23). Ca/Ti and Zr/Rb ratios also display marked differences between the upper and lower halves of the profile. Variabilities of both Ca/Ti (14.70 (2.1), n = 25) and Zr/Rb (1.50 (0.2), n = 25) in units 1–4 are slightly smaller than in units 5–8, where Ca/Ti has a median value of 19.99 (4.4, n = 23) and Zr/Rb has a median value of 1.71 (0.2, n = 23) (Fig. 4). In addition to sedimentological differences between the two parts of the profile, differences are also identified among the units. Dating results in Tek-5 (n = 7, from ca. 6.6 cal ka BP at 221 cm bs to 3.6 cal ka BP at 363 cm bs) show markedly repeated age inversions (Fig. 4, Table 3).
Unit 1 (786–693 cm bs) consists of very dark gray (10YR 3/1), moderately to strongly calcareous sandy silt. An intermediate sandy layer occurs at 751–740 cm bs Common carbonate nodules and few manganese mottles exist. Fine pieces of snail shells were found around 765 cm bs The charcoal sample from the bottom of the profile (781 cm bs) dates to 5301–5045 cal yr BP (Table 3). The transition from unit 1 to 2 takes place gradually.
Unit 2 (693–607 cm bs) contains two layers of dark yellowish-brown (10YR 4/3) sandy silt with an intercalated layer of very dark grayish-brown (10YR 3/2) sandy silt at 658–662 cm bs Sediments are strongly calcareous. Few blackish manganese and reddish iron nodules (Ø < 1 cm) and common carbonate nodules occur in the lower third of the unit. The sediment boundary between units 2 and 3 is gradual.
Unit 3 (607–477 cm bs) consists of strongly calcareous sandy silt with very few fine pebbles. The color changes from dark brown (7.5 YR 3/2) at the bottom to brown (7.5 YR 4/3) at the top. Few fine carbonate nodules and manganese mottles are incorporated. One vertical greenish mottled structure occurs at 530–520 cm bs.
Bulk parameters of LOI550 and XLF display strong oscillations in median values for the three units (Fig. 4). In unit 1, XLF has a median value of 32 (2) × 10−8 m3/kg (class 2, n = 5), and the median value of LOI550 ranges into class 3 (4.4 (0.2) mass%). With decreasing depth in unit 2, LOI550 values slightly increase, while values for XLF slightly decrease (Fig. 4). Small peaks in XLF and LOI550 occur at the intermediate layer of unit 2. In unit 3, XLF values (n = 9) peak at 570 cm bs (29 × 10−8 m3/kg, class 1) and gradually decrease toward the top, reaching a minimum value (10 × 10−8 m3/kg, class 1) at 480 cm bs LOI550 values gradually increase from 5 to 5.8 mass% (class 4) with decreasing depth. At the top of unit 3 (478 cm bs), the organic-rich bulk sample dates to 6270–6002 cal yr BP. The boundary between unit 3 and overlying unit 4 is sharp.
Unit 4 (477–426 cm bs) is composed of brown (7.5 YR 5/4), poorly compacted and strongly calcareous, slightly fine pebbly, silty sand in the lower part (477–463 cm bs) and dark yellowish-brown (10YR 4/4), heavily compacted, moderately calcareous sandy silt in the upper part (463–426 cm bs). Some pebbles are carbonated. Few fine manganese nodules and very few fine carbonate concretions were found.
The median pH value of water-saturated sediments in unit 4 is around 8.1 (n = 6) and is in the same range as the underlying units (moderately alkaline). Also, EC values are comparable to those in the underlying sediments but exhibit remarkable oscillations (245 (32) μS/cm, class 2, n = 6). LOI550 is low in the lower layer of unit 4 (2.7 mass%, class 2, at 477–463 cm bs), and it increases toward the unit top, peaking at 444 cm bs (4.1 mass%, class 3). XLF has a median value of around 13 (3) × 10−8 m3/kg (class 1, n = 6) and constantly increases from 10 × 10−8 m3/kg (class 1) at the bottom to 33 × 10−8 m3/kg (class 2) at the top. Graphs of element ratios (Ca/Ti and Zr/Rb) widely run in parallel, both showing low values in the lower strata (units 1–4; Fig. 4). At the top of unit 4, a bulk sample from 427 cm bs dates to 4958–4841 cal yr BP, and a mixed charcoal/sediment sample from 435 cm bs dates to 5462–5309 cal yr BP. The boundary with overlying unit 5 is sharp.
Unit 5 (426–358 cm bs) consists of dark yellowish-brown (10YR 4/6), normally graded sediments. Sediment texture changes from very soft, subangular to subrounded medium pebbly (Ø < 2 cm) sand at the base to silty sand at the top. A snail fragment occurs at 420 cm bs The sediments are moderately to strongly calcareous and contain very few blackish manganese mottles. The pH peaks at the top of unit 5 (pH: 8.6, strongly alkaline, at 363 cm bs). Within unit 5 (n = 4), EC (118 (17) μS/cm, class 1) and LOI550 (1.9 (0.4) mass%, class 1) drop to their lowest values, whereas XLF (37 (4) × 10−8 m3/kg, class 2) shows high values in comparison with the underlying and overlying units. Element ratios of Ca/Ti (19.2 (3.4)) and Zr/Rb (1.7 (0.4)) also slightly increase compared with the lower units. The mixed charcoal/sediment sample at 363 cm bs dates to 3812–3566 cal yr BP. The boundary with overlying unit 6 is sharp.
Unit 6 (358–169 cm bs) consists of soft, moderately calcareous, and normally graded sediments. The texture changes from strongly brown (7.5 YR 4/4) sandy silt at the bottom to brown (7.5 YR 5/6) slightly sandy silt at the top. Few fine pebbles (Ø < 1 cm) occur and very few of them are carbonated. Grayish to blackish manganese mottles commonly exist throughout the unit, particularly at 358–313 cm bs Fine brick/ceramic fragments were found at 180 and 273 cm bs.
The measured bulk parameters (n = 10) remain constant in this unit and show clear differences with the underlying unit 5 and the overlying unit 7. Sediments are moderately alkaline (pH: 8.1 (0.1)). EC shows alternating, but overall increased values (186 (33) μS/cm, class 1). XLF values display a distinct trough (24 (4) × 10−8 m3/kg, class 2) compared with the underlying unit 5 (37 (4) × 10−8 m3/kg, class 2, n = 4) and overlying unit 7 (44 (2) × 10−8 m3/kg, class 2, n = 6). LOI550 (3.2 (0.3) mass%, class 2) remains relatively stable and is higher than in the underlying (1.9 (0.4) mass%, class 1, n = 4) and overlying unit (2.2 (0.3) mass%, class 2, n = 6). Element ratios (Ca/Ti and Zr/Rb) remain roughly the same and show relatively lower values compared with the underlying and overlying units. The two datings of mixed charcoal/sediment samples show distinct age inversions. The sample from 260–265 cm bs dates to 5575–5325 cal yr BP. The sample from 221 cm bs dates to 6556–6396 cal yr BP and, thus, is older than the underlying age samples. The transition between units 6 and 7 is sharp.
Unit 7 (169–58 cm bs) is characterized by yellowish-brown (10YR 5/4), slightly compacted, and inversely graded sediments. Sediment texture changes from fine pebbly silty sand at the bottom to sandy, subangular subrounded medium pebbles at the top. The pebbly sediments at the top are matrix-supported and poorly sorted. Sediments in this unit are strongly calcareous and contain very few carbonate nodules. A ceramic piece occurs at 149–140 cm bs and a small brick fragment at 150–147 cm bs Geochemical samples (n = 6) indicate relatively low EC (150 (15) μS/cm, class 1) and LOI550 values (2.2 (0.3) mass%, class 2) compared with those in the overlying and underlying units. Overall, LOI550 values gradually increase from the bottom (1.8 mass%, class 1) to the top (3.3 mass%, class 2). Along with the whole extracted profile, values of XLF (44 (2) × 10−8 m3/kg, class 2) and the Ca/Ti ratio (40.05 (6.9)) reach their maximum in unit 7. Also, the Zr/Rb has a peak of 2.55 at 130 cm bs The boundary with overlying unit 8 is gradual.
Unit 8 (58–0 cm bs) corresponds to the present-day plow horizon and contains dark yellowish-brown (10YR 4/4), moderately calcareous, slightly sandy silt with very few embedded fine pebbles and roots. The pH values remain stable all over units 6–8 (moderately alkaline). EC values steadily increase from the bottom to the top of unit 8, reaching 271 μS/cm (class 2) at the surface. LOI550 contents have a median value of 5.1 (0.1) mass% (class 4, n = 3). XLF values (37 (0) × 10-8 m3/kg, class 2, n = 3) are slightly lower than in the underlying unit 7. Element ratios (n = 3) of Ca/Ti (18.57 (1.3)) and Zr/Rb (1.50 (0.1)) are in the same range as unit 6 and are distinctly lower than unit 7.
Tekkedere fan toe: key profile Tek-6
Profile Tek-6 was extracted from the toe of the Tekkedere alluvial fan. It has a total thickness of 800 cm and includes seven units (Fig. 5). This profile is characterized by a thick (2.5 m) unit of blackish-grayish clayey silt (unit 2) in its lower part, overlain by normally graded sediments (units 3 and 4) in the middle part and brownish fine-textured sediments (units 5–7) in the upper 2 m. Sediments of Tek-6 are moderately to strongly calcareous.

Figure 5. The lithostratigraphy, radiocarbon dates, and sediment analyses of sediment core Tek-6. cm bs, cm below surface; EC, electrical conductivity; LOI550, loss on ignition at 550°C; XLF, mass-specific susceptibility at the low frequency.
The pH values in the lower half of the profile (below 427 cm bs, units 1 and 2) are constant at 8.4 (0.1, n = 30), corresponding to a moderately alkaline environment. Sediments from 400 cm bs to the profile surface gradually change to slightly alkaline (pH is 7.9 at 33 cm bs). The graphs of EC (343 (108) μS/cm, class 2, n = 50) and LOI550 values (3.9 (2.1) mass%, class 3, n = 50) run inversely to the graph of pH values. The lowest EC and LOI550 values are observed at the middle profile (427–224 cm bs). The graph of XLF values (56 (29) × 10−8 m3/kg, class 2, n = 50) roughly shows the same trend as pH and peaks at 109 (10) × 10−8 m3/kg (class 4) in the middle part of the profile (427–224 cm bs, n = 11). The element ratio of Ca/Ti has a median value of 12.94 (3.9) and Zr/Rb has a median value of 1.20 (0.2), with a distinct Ca/Ti peak at 677 cm bs and a distinct Zr/Rb peak at 400 cm bs.
Unit 1 (800–677 cm bs) is composed of four thin layers of sandy to silty sediments varying in color (Fig. 5). Brown sediments at 747–725 cm bs are normally graded, with texture gradually changing from coarse sand with few medium pebbles (Ø < 3 cm, angular to subrounded) at the bottom to silty sand at the top. The uppermost layer (725–677 cm bs) consists of brown to yellowish-gray sandy silt with few weathered carbonate concretions. The yellowish-gray color of the sediment likely results from the weathered carbonate. At 770–725 cm bs, sediments are strongly calcareous. The graphs of measured bulk parameters have comparable trends with a slight decrease of pH, EC, and LOI550 values occurring around 770 cm bs The Ca/Ti ratio has a median value of 17.75 (4.2, n = 10) and a peak of 21 at the top of this unit (around 673 cm bs). The ratio of Zr/Rb has a median value of 1.45 (0.2, n = 10). The boundaries among the layers in this unit are gradual, and unit 1 sharply transitions to unit 2.
Unit 2 (677–427 cm bs) is characterized by poorly compacted, blackish-grayish clayey silt. Color varies slightly between dark greenish gray (Gley 1 3/5GY, 600–500 cm bs) and very dark gray (Gley 1 3/N, 500–436 cm bs). The sediments are moderately calcareous at 657–628 cm bs and slightly calcareous at 600–436 cm bs Other than a single carbonate nest around 585 cm bs and few carbonate concretions at 677–657 cm bs, very few carbonate concretions occur in this unit. Sediments at 677–657 cm bs are clayey sandy silt, slightly coarser than the upper part (657–436 cm bs). The latter is characterized by a very strong emanation of an H2S smell after adding 9.9% HCl, indicating the presence of Fe sulfides under reducing conditions (Jahn et al., Reference Jahn, Blume, Asio, Spaargaren and Schad2006). At 436–427 cm bs, sediments are moderately calcareous, poor in clay content, and contain very few fine reddish iron mottles.
The pH values (n = 20) gradually decrease from 8.5 (strongly alkaline) at the base to 8.2 (moderately alkaline) at the top. EC values (n = 20) gradually increase from ca. 300 μS/cm (class 2) at the bottom to 500 μS/cm (class 3) at the top. XLF values remain low (35 (3) × 10−8 m3/kg, class 2, n = 14) at 600–440 cm bs, where LOI550 values reach the maximum (6.7 (0.5) mass%, class 5, n = 20) of the whole profile. Element ratios of Ca/Ti (8.65 (2.0), n = 20) and Zr/Rb (0.94 (0.1), n = 20) remain low. Two organic-rich bulk samples from 665 cm bs and 460 cm bs date to 6187–5947 cal yr BP and 4959–4833 cal yr BP, respectively. The sediment boundary between units 2 and 3 is sharp.
Unit 3 (427–320 cm bs) shows slightly calcareous and normally graded sediments. The texture gradually changes from light olive brown (2.5 Y 5/3) pebbly coarse sand at the base, to medium–coarse sand in the middle, to dark yellowish-brown (10YR 4/6) fine sand at the top. Very few manganese mottles and few micas occur in the upper part of the unit (320–315 cm bs). Sediments are strongly alkaline (pH: 8.7 (0.1), n = 5). Median values (n = 5) of EC (112 (11) μS/cm, class 1) and LOI550 (0.8 (0.1) mass%, class 1) are the lowest of the whole profile. XLF values (114 (19) × 10−8 m3/kg, class 4, n = 5) peak at ca. 400 cm bs (143 × 10−8 m3/kg, class 5) and gradually decrease to 80 × 10−8 m3/kg (class 3) at the top. Ca/Ti values (14.68 (2.0), n = 5) are slightly higher than in unit 2. The Zr/Rb ratio peaks around 400 cm bs (3.91) and decreases toward the top. The boundary between unit 3 and the overlying unit 4 is sharp.
Unit 4 (320–224 cm bs) continues the trend of decreasing grain size with decreasing depth. The moderately calcareous sediments consist of brown (7.5 YR 5/4) coarse sand at the bottom, sandy silt at the middle, and dark reddish-gray (5 YR 4/2) sandy clayey silt at the top. Weathered fragments of carbonate rocks occur at 239–232 cm bs and very few manganese mottles at 244–224 cm bs Sediments are strongly alkaline (pH: 8.5 (0.1), n = 6). EC values gradually increase from 140 μS/cm (class 1) at the base to 487 μS/cm (class 3) at the top. Median XLF values (106 (7) × 10−8 m3/kg, class 4, n = 6) remain in the same range as in the underlying unit 3. Also, the LOI550 median value is around 1.5 (0.7) mass% (class 1, n = 6) and shows the same range as in unit 3. The Ca/Ti ratio (12.63 (1.9), n = 6) and Zr/Rb ratio (1.40 (0.1), n = 6) exhibit a slight increase from the bottom to the top and display small peaks in the upper part. The boundary with overlying unit 5 is gradual.
Unit 5 (224–107 cm bs) shows reddish-brown (5 YR 4/4), strongly compacted clayey silt with very few embedded fine pebbles. Manganese mottles commonly exist in this unit with slightly decreasing abundance from the bottom to the top. Sediments are slightly calcareous and very few fine to medium carbonate fragments occur at 200–150 cm bs The boundary with overlying unit 6 is a gradual contact.
Unit 6 (107–29 cm bs) consists of brown (7.5 YR 5/4), slightly calcareous clayey silt. The compactness and carbonate content decrease with decreasing depth. Sediments from units 5 and 6 are moderately alkaline with decreasing pH values from the bottom to the top. EC values gradually decrease from ca. 730 μS/cm (class 5) in unit 4 to 330 μS/cm (class 2) in unit 6. Median XLF values in units 5 and 6 are low without remarkable oscillations (24 (1) × 10−8 m3/kg, class 1, n = 9). LOI550 values in both units are relatively high (5.2 (0.2) mass%, class 4, n = 9) compared with the underlying units 3 and 4 (1.1 (0.4) mass%, class 1, n = 11). The Ca/Ti ratio (17.58 (1.7), n = 9) is high and the Zr/Rb ratio (1.22 (0.1), n = 9) is low in units 5 and 6. Unit 6 gradually transitions to unit 7.
Unit 7 (29–0 cm bs) corresponds to the present-day plow horizon, which consists of brown (7.5 YR 4/2), heavily compacted clayey silt.
SEDIMENT FACIES
Similar to previous studies in the Pergamon micro-region (Schneider et al., Reference Schneider, Matthaei, Bebermeier and Schütt2014, Reference Schneider, Matthaei, Schlöffel, Meyer, Kronwald, Pint and Schütt2015), sediment facies in the eight Tekkedere profiles are identified based on their geomorphological setting, field observation, sediment texture, and measured sediment characteristics (Blair and McPherson, Reference Blair and McPherson1994; Brown, Reference Brown1997; Goldberg and Macphail, Reference Goldberg and Macphail2006; Miall, Reference Miall2006, Reference Miall2014).
Facies A: Tekkedere valley bottom sediments
Sediments that infilled the Tekkedere valley, that is, profiles Tek-1 (Supplementary Fig. 2), Tek-2 (Fig. 3), and Tek-3 (Supplementary Fig. 3), are classified as facies A (Tekkedere valley bottom sediments). Facies A is further subdivided into fine-textured (facies Aa) and coarse-textured (facies Ab) deposits (Table 4). They were predominantly deposited by fluvial processes of the Tekkedere creek (Fig. 2A and C), as evidenced by the sediment lithology and topographical location (Goldberg and Macphail, Reference Goldberg and Macphail2006). Fluvial sediments are partly interfingering with colluvial deposits characterized by massive bedding and poor sorting (Mills, Reference Mills1979; Brown, Reference Brown1997; Miall, Reference Miall2014). The development of fine- and coarse-textured valley bottom sediments in Tekkedere is different from the formation of sediments in the Gümüş valley, where fine-grained sediments dominate (Knitter, Reference Knitter2013).
Table 4. The features of different sediment facies in the Tekkedere catchment.

aEC, electrical conductivity; LOI550, loss on ignition at 550°C; XLF, mass-specific susceptibility at the low frequency.
Facies Aa (fine-textured sediments) contains lower coarse fraction content (grains <2 mm are less than 10%), whereas facies Ab (coarse-textured) shows distinctively higher coarse fraction content (reaching 60%) (Fig. 3). Compared with facies Aa, facies Ab is mostly more alkaline and shows lower EC and LOI550 values. Parts of the fine-textured sediments present typical post-sedimentary and pedogenic features, including common animal burrows and roots, relatively dark color, low pH, high EC, and increased LOI550 values. They document processes of humification and consequently are classified as buried topsoil horizons (pedofacies sAa in Table 4; Goldberg and Macphail, Reference Goldberg and Macphail2006), such as the buried soil horizon of unit 4 in Tek-2 (Fig. 3). This facies indicates a poorly developed soil horizon, pointing to a short period of reduced land erosion activity (Dusar et al., Reference Dusar, Verstraeten, Notebaert and Bakker2011).
Facies B: Tekkedere alluvial fan sediments
The deposition of the Tekkedere alluvial fan is predominantly formed by brownish fan sediments (facies B). Facies B is also differentiated into fine-textured (facies Ba) and coarse-textured (facies Bb) deposits (Table 4) with distinct characteristics of alluvial fan deposits (Blair and McPherson, Reference Blair, McPherson, Parsons and Abrahams2009; Bowman, Reference Bowman2019). Facies Ba is inferred from the generally fine composition of grain size (clayey to silty sediments with a low proportion of pebbles), often increased EC and LOI550 values, and very low results for XLF values and Ca/Ti and Zr/Rb ratios. In contrast, facies Bb contains more coarse components (sands with fine to medium pebbles) and fewer Fe–Mn mottles than facies Ba, for example, unit 1 in Tek-4 (Supplementary Fig. 4) and units 5 and 7 in Tek-5 (Fig. 4). Facies Bb usually shows very low EC and LOI550 values, but increased XLF and element ratios.
Following the alluvial fan's longitudinal profile (the transect from the fan apex to its toe; Fig. 6), two major cycles of fine- and coarse-textured fan sediments can be identified in the mid-fan position, that is, profiles Tek-5 and Tek-4-2. In general, the texture of facies Ba and Bb fines from the apex to the toe of the alluvial fan, and the thickness of layers declines in parallel (Fig. 6), which is also reported on the alluvial fans in western Turkey (Özpolat et al., Reference Özpolat, Yıldırım and Görüm2020). In addition, the thin layers of facies Ba, which only vertically discontinuously occur in the lower part of Tek-5, can be linked with the fine-textured sediments below 463 cm bs in Tek-4-2 (Fig. 6).

Figure 6. Longitudinal profile along with the Tekkedere alluvial fan (91 × vertical exaggerations) with sediment cores. Display of the thickness of the different units corresponds to height scale, and sediment architectures are based on the sediment units of the different cores. Embedded calibrated radiocarbon ages provide an overview of sediment ages and age inversions. m asl, m above sea level.
Increased LOI550 values in units 2 and 5 of Tek-4-2 (Supplementary Fig. 5) indicate the evidence of buried topsoil horizons developed on the fine-textured alluvial fan deposits, reaching a thickness up to 30 cm (facies sBa in Fig. 6 and Table 4; Soil Survey Staff, 1999; Kapur et al., Reference Kapur, Akça and Günal2017; Macphail and Goldberg, Reference Macphail and Goldberg2017).
Facies C: Bakırçay River sediments
Given that the position of the fan toe is proximal to the Bakırçay River, the dark-colored and organic-rich sediments in the lower part of the profiles extracted at the middle–distal part of the fan (profiles Tek-5, Tek-5-2, and Tek-6) are interpreted as Bakırçay River deposits (facies C in Fig. 6; cf. Goldberg and Macphail, Reference Goldberg and Macphail2006; Miall, Reference Miall2006). Most of these sediments correspond to sandy silts, whereas coarse sands and angular pebbles are nearly absent. The Ca/Ti ratio in facies C is generally lower than in facies B, likely associated with the differences in the occurrence and distribution of carbonate rocks in the drainage basins of Bakırçay and Tekkedere (MTA, 2002; Yang et al., Reference Yang, Becker, Knitter and Schütt2021; Fig. 2B).
Field observation in the present-day Bakırçay floodplain indicates subenvironments of the abandoned channel, paleo-oxbow, and overbank, which are comparable to those of the Büyük Menderes floodplain in western Turkey (Özpolat et al., Reference Özpolat, Yıldırım and Görüm2020). Based on sediment characters and their occurrence in proximity to the present-day floodplain, the sandy-silty sediments are interpreted as Bakırçay River floodplain deposits (facies Cf in Table 4; cf. Miall, Reference Miall2006). These floodplain deposits can be observed in units 1 and 3 of Tek-5 (Fig. 4), unit 1 of Tek-5-2 (Supplementary Fig. 6), and units 1 and 4 of Tek-6 (Fig. 5). In the lower part of profile Tek-5 (mid-fan position), the floodplain deposits (facies Cf) interfingered with layers of fine-textured alluvial fan sediments (facies Ba), that is, units 2 and 4 in Tek-5 (Figs. 4 and 6). None of the alluvial fan sediments (facies B) occur in the lower parts of Tek-5-2 and Tek-6 (the toe of the Tekkedere fan).
The 2.5-m-thick unit of dark gray silty clay with very high LOI550 values (unit 2 in Tek-6; Fig. 5) is interpreted as still water sediments (facies Cs; Table 4) of an oxbow lake or a (secondary) channel (Miall, Reference Miall2006, Reference Miall2014; Charlton, Reference Charlton2007). Sediments overlying facies Cs in Tek-6 show a distinct fining-upward feature, from pebbly sand (unit 3) to sandy silt (unit 4) (Fig. 5). This gradual change in grain size reflects a reduction in the sediment transportation ability of the Bakırçay River. The decreasing fluvial activity points out a high-energy channel-related environment (facies Cc) transferred to a low-energy floodplain (facies Cf) (Miall, Reference Miall2006). In the middle part of unit 1 in Tek-6, a similar feature of abandoned channel-related deposits (facies Cc) can be observed, but it is less thick and distinct (Fig. 6). In general, the gradual change in vertical lithostratigraphy indicates a meandering pattern (Miall, Reference Miall2006). This is supported by Aksu et al. (Reference Aksu, Piper and Konuk1987) who suggest a strongly meandering channel pattern of the Bakırçay in its lower course during the Late Quaternary.
Redoximorphic features of Mn–Fe nodules as well as secondary precipitated carbonates prevailing in the Bakırçay floodplain deposits reflect the effect of a post-sedimentary seasonally fluctuating groundwater table (Goldberg and Macphail, Reference Goldberg and Macphail2006). These postdepositional processes have also been reported in several locations in the lower Bakırçay basin (Schneider et al., Reference Schneider, Matthaei, Bebermeier and Schütt2014, Reference Schneider, Matthaei, Schlöffel, Meyer, Kronwald, Pint and Schütt2015, Reference Schneider, Schlöffel, Schwall, Horejs and Schütt2017).
Boundaries between the facies of the Bakırçay River floodplain (facies Cf) and the overlying facies of the Tekkedere alluvial fan (facies B) in profiles Tek-5, Tek-5-2, and Tek-6 are roughly at the same absolute elevation (ca. 4–5 m asl; Fig. 6). At these boundaries, the floodplain deposits frequently show weakly developed thin fossil soil horizons (facies sCf; Fig. 6, Table 4), that is, the buried paleosols in the upper part of units 3 and 4 in Tek-5 (Fig. 4), unit 1 in Tek-5-2 (Supplementary Fig. 6), and unit 4 in Tek-6 (Fig. 5). Comparable to soil facies sAa in the Tekkedere valley bottom, this pedofacies sCf with its immature A horizon documents past soil formation and a stable surface on the floodplain.
MID- TO LATE HOLOCENE GEOMORPHODYNAMICS
The lithostratigraphic analyses and the radiocarbon ages indicate four major geomorphodynamic phases from the Middle to Late Holocene (Figs. 6 and 7).

Figure 7. The calibrated radiocarbon ages of the samples from the Tekkedere area and the natural and cultural variables in the Pergamon micro-region. Data set: 1) Tekkedere: sediment facies (this study) and preliminary results of the human occupation (Ludwig, Reference Ludwig and Pirson2020a; Michalski, Reference Michalski2021; Tozan, Reference Tozan and Pirson2022) and earthquake in 1939 (Paradisopoulou et al., Reference Paradisopoulou, Papadimitriou, Karakostas, Taymaz, Kilias and Yolsal2010; Çelik et al., Reference Çelik, Karacakaya, Mete, Genç and Bal2019; Pirson, Reference Pirson2020; Tozan, Reference Tozan and Pirson2022); 2) preliminary results of occupation in Pergamon city (Pirson, Reference Pirson2017); 3) Elaia: preliminary results of occupation (Pirson, Reference Pirson, Ladstätter, Pirson and Schmidts2014; Pirson et al., Reference Pirson, Ateş, Bartz, Brückner, Feuser, Mania, Meier, Seeliger, Matthaei and Zimmermann2015; Feuser et al., Reference Feuser, Mania, Pirson, Mohr, Rheidt and Arslan2020) and landscape and forest cover (Shumilovskikh et al., Reference Shumilovskikh, Seeliger, Feuser, Novenko, Schlütz, Pint, Pirson and Brückner2016; Seeliger et al., Reference Seeliger, Pint, Feuser, Riedesel, Marriner, Frenzel, Pirson, Bolten and Brückner2019); 4) Geyikli: preliminary results of occupation in Atarneus hill settlement (Schneider et al., Reference Schneider, Nykamp, Matthaei, Bebermeier and Schütt2013, 2014; Zimmermann et al., Reference Zimmermann, Matthaei, Ateş, Matthaei and Zimmermann2015); 5) western lower Bakırçay plain: preliminary results of occupation in Yeni Yeldeğirmentepe (Horejs, Reference Horejs and Pirson2010b, Reference Horejs and Pirson2011b; Schneider et al., Reference Schneider, Schlöffel, Schwall, Horejs and Schütt2017) and Teuthrania/Teult114 (Schneider et al., Reference Schneider, Matthaei, Schlöffel, Meyer, Kronwald, Pint and Schütt2015; Williamson, Reference Williamson, McInerney and Sluiter2016) and modeled sedimentation (Becker et al., Reference Becker, Knitter, Nykamp and Schütt2020a); 6) preliminary results of occupation in the Pergamon micro-region (only valid for its western part) (Horejs, Reference Horejs, Horejs and Kienlin2010a, Reference Horejs, Önen, Mutluer and Çetin2011a, Reference Horejs, Scholl and Pirson2014; Pirson and Zimmermann, Reference Pirson, Zimmermann, Scholl and Pirson2014); 7) Beyşehir Occupation phase (Eastwood et al., Reference Eastwood, Roberts and Lamb1998); 8) rainfall in Turkey (Finné et al., Reference Finné, Woodbridge, Labuhn and Roberts2019). Abbreviations: E, Early; M, Middle; L, Late; Hell., Hellenistic Period; Rom., Roman Imperial Period; pre-Otto., pre-Ottoman; Ottom., Ottoman.
Phase 1 (ca. 6.2 to 5–4 ka): aggradation of the Bakırçay floodplain
Sediment dynamics during the first phase are mainly related to the deposition of Bakırçay River sediments (facies C), for example, the floodplain aggradation. Proximal to the current Bakırçay channel, the paleo-floodplain (facies Cf) at the Tek-6 location was first dissected by a (secondary) channel (facies Cc) (Fig. 6). The channel was then filled by sediments with the fining upward feature. Hereafter, a still water environment (facies Cs) existed for more than a millennium (from 6.1 to 4.9 ka; Table 3). It was then dissected by a younger channel (facies Cc) and successively covered with silty floodplain deposits (facies Cf). The upper floodplain layers (facies Cf) in profile Tek-6 are linked to the floodplain sediments in Tek-5-2 based on the same absolute elevation at ca. 4–5 m asl (Fig. 6). More distal to the current Bakırçay River, floodplain deposits (facies Cf) in Tek-5 interfinger with the sediments originating from the Tekkedere catchment (facies Ba), which indicates the location of Tek-5 marks a former overlapping zone of the peripheral Bakırçay plain and the Tekkedere fan.
The beginning of the Bakırçay floodplain aggradation in the area of the present-day Tekkedere fan predates ca. 6.2 ka. This is revealed by the radiocarbon sample from the bottom of the still water sediment in Tek-6 (6187–5947 cal yr BP at 665 cm bs; Table 3). Accordingly, the underlying floodplain (facies Cf) and the secondary channel (facies Cc) deposits were older than 6.2 cal ka BP. The Bakırçay floodplain sediments continued to deposit in the distal part of the current Tekkedere alluvial fan at least until 5301–5045 cal yr BP, which is indicated by the charcoal sample at 781 cm bs in Tek-5 (Table 3). Although it has to be considered that radiocarbon dates of charcoals are often interpreted as the maximum depositional age due to the possible reworking of the samples before their last deposition (Lang and Hönscheidt, Reference Lang and Hönscheidt1999; Chiverrell et al., Reference Chiverrell, Harvey and Foster2007; Nykamp et al., Reference Nykamp, Knitter and Schütt2020), the aforementioned charcoal, because of its considerable size (Ø = ca. 0.5 cm, larger than other charcoal pieces) and its deposition in fine floodplain sediments, is considered to have been deposited a rather short time after being burned. Hence, it is acceptable to use the charcoal date as the depositional age of the surrounding sediments.
The termination of floodplain aggradation in the first phase is marked by the formation of soil horizons (Fig. 6). The dating of the end of phase 1 is precarious due to several age inversions and the specific characteristics of different dated materials. In Tek-5, the dating results of the two samples from 478 cm bs (6270–6002 cal yr BP) and 435 cm bs (5462–5309 cal yr BP) are most likely overestimated (Fig. 6), because the charcoal sample taken from the bottom of the profile (781 cm bs, 5301–5045 cal yr BP) represents a maximum depositional age of the overlying deposits. We therefore suggest the dating samples from the soil horizons (around 430 cm bs in Tek-5) incorporated older materials, such as reworked and washed terrestrial soils and organics (Fowler et al., Reference Fowler, Gillespie and Hedges1986; Strunk et al., Reference Strunk, Olsen, Sanei, Rudra and Larsen2020). However, an alternative interpretation of dating results from bulk soil samples is possible. Based on the open system of soil formation (Scharpenseel and Schiffmann, Reference Scharpenseel and Schiffmann1977; Wang et al., Reference Wang, Amundson and Trumbore1996; Kovda et al., Reference Kovda, Lynn, Williams and Chichagova2001), radiocarbon ages of organic-rich bulk samples are considered to be minimum ages since the initiation of soil formations (Scharpenseel and Schiffmann, Reference Scharpenseel and Schiffmann1977; Wang et al., Reference Wang, Amundson and Trumbore1996). In this sense, the time of the soil formation and the deposition of the parent material should be even older than the dating result of the bulk sample at 478 cm bs of Tek-5 (6270–6002 cal yr BP). Nonetheless, this is inconsistent with the charcoal dating result.
The date of the bulk sample at 427 cm bs (4968–4841 cal yr BP) in Tek-5 (Fig. 6) indicates soil formation continued after ca. 5.0 ka. The bulk sample from the buried surface of the floodplain (facies sCf, 4224–3982 cal yr BP at 285 cm bs) in Tek-5-2 suggests the floodplain aggradation occurred before 4 ka. In Tek-6, the uppermost layer of still water sediments dated to 4959–4833 cal yr BP represents the maximum burial age of the floodplain at this location. Thus, based on the dating results from the three profiles, the depositional termination of the Bakırçay River sediments in the area of the recent Tekkedere fan started before ca. 6.2 ka and ended between ca. 5 and 4 ka. Concurrently, the fine-textured Tekkedere alluvial fan deposits (facies Ba) accumulated at least to the location of Tek-4-2 and interfingered with Bakırçay River sediments at the location of Tek-5.
The dominant deposition of Bakırçay River sediments over Tekkedere fan deposits was mainly controlled by supraregional factors. It is assumed that the extensive aggradation of the Bakırçay River is associated with the rising sea level around the Elaia Bay in the Aegean part of Turkey that slightly slowed down but continued after 6 ka (Kayan, Reference Kayan1999; Seeliger et al., Reference Seeliger, Pint, Frenzel, Feuser, Pirson, Riedesel and Brückner2017, Reference Seeliger, Pint, Feuser, Riedesel, Marriner, Frenzel, Pirson, Bolten and Brückner2019), resulting in the rise of the erosional base level (Robustelli et al., Reference Robustelli, Lucà, Corbi, Pelle, Dramis, Fubelli, Scarciglia, Muto and Cugliari2009). In addition, during the Middle Holocene, the prevailing wetter-than-today humid climate in Anatolia and the Balkans (Eastwood et al., Reference Eastwood, Leng, Roberts and Davis2007; Finné et al., Reference Finné, Woodbridge, Labuhn and Roberts2019; Fig. 7, rainfall in Turkey) fostered fluvial dynamics (Walsh et al., Reference Walsh, Berger, Roberts, Vanniere, Ghilardi, Brown and Woodbridge2019). Evidence for the increased sediment load and discharge of the Bakırçay River in the Tekkedere area (i.e., maximum floodplain extension, a 1000-year-long still water environment, and shifted secondary channels) coincides with this Middle Holocene humid period. These fluvial dynamics are not only observed in Tekkedere but also in other areas in the Pergamon micro-region (Schneider et al., Reference Schneider, Matthaei, Schlöffel, Meyer, Kronwald, Pint and Schütt2015, Reference Schneider, Schlöffel, Schwall, Horejs and Schütt2017; Becker et al., Reference Becker, Knitter, Nykamp and Schütt2020a) and the eastern Mediterranean region (Dusar et al., Reference Dusar, Verstraeten, Notebaert and Bakker2011; Benito et al., Reference Benito, Macklin, Zielhofer, Jones and Machado2015; Glais et al., Reference Glais, Lespez, Vannière and López-Sáez2017; Bulkan et al., Reference Bulkan, Yalçın and Wilkes2018; Ocakoğlu et al., Reference Ocakoğlu, Çilingiroğlu, Erkara, Ünan, Dinçer and Akkiraz2019).
Simultaneously, we could assume that under the Middle Holocene humid climate and high forest cover (Shumilovskikh et al., Reference Shumilovskikh, Seeliger, Feuser, Novenko, Schlütz, Pint, Pirson and Brückner2016; Fig. 7), erosion sensitivity and sediment dynamics were low, which explains the middle Tekkedere alluvial fan (Tek-4-2 and Tek-5) during this phase only consisted of fine-textured sediments and had a low spatial extent (Fig. 6). The interfingering layers of floodplain and alluvial fan deposits in profile Tek-5 are attributed to torrential runoff events in the Tekkedere valley, possibly corresponding to short-term relatively arid events, as also recorded in Anatolia, for example, at Gediz Graben during 5.7–4.2 ka (Bulkan et al., Reference Bulkan, Yalçın and Wilkes2018), the Kureyşler area at ca. 7.0–5.3 ka (Ocakoğlu et al., Reference Ocakoğlu, Çilingiroğlu, Erkara, Ünan, Dinçer and Akkiraz2019), and Tecer Lake at ca. 5.3–5 ka (Kuzucuoğlu et al., Reference Kuzucuoğlu, Dörfler, Kunesch and Goupille2011).
Human impacts on geomorphodynamics in the Tekkedere drainage basin during phase 1 might be negligible. Settlements may have existed in the Pergamon micro-region since the Neolithic, for example, Yeni Yeldeğirmentepe (Schneider et al., Reference Schneider, Schlöffel, Schwall, Horejs and Schütt2017) and many sites in the western lower Bakırçay catchment (Horejs, Reference Horejs, Horejs and Kienlin2010a, Reference Horejs, Önen, Mutluer and Çetin2011a, Reference Horejs, Scholl and Pirson2014; Pirson and Zimmermann, Reference Pirson, Zimmermann, Scholl and Pirson2014; Pirson, Reference Pirson2022), but there was likely no occupation or land use in Tekkedere during this time (Michalski, Reference Michalski2021; Fig. 7).
Phase 2 (between ca. 5 and 4 ka): formation of floodplain soils
In all three sediment profiles where Bakırçay River sediments were extracted, that is, Tek-5, Tek-5-2, and Tek-6, organic-rich topsoil horizons (facies sCf) developed on the floodplain deposits (facies Cf) (Fig. 6). The formation of the floodplain soil horizons is marked as the second phase of geomorphodynamics, which indicates no or negligible accumulation of materials and thus a phase of relative stability. Phase 2 is suggested to have occurred between ca. 5 and 4 cal ka BP, as dated by the bulk samples at 427 cm bs (4968–4841 cal yr BP) in Tek-5 and 285 cm bs (4224–3982 cal yr BP) in Tek-5-2 (Fig. 6).
Reduced flooding activities, as well as low accumulation of fan sediments, are the direct reasons for the soil formation, similar to the case study in the Central Ebro Basin (NE Spain) (Pérez-Lambán et al., Reference Pérez-Lambán, Peña-Monné, Badía-Villas, Picazo Millán, Sampietro-Vattuone, Alcolea Gracia, Aranbarri, González-Sampériz and Fanlo Loras2018) and in the wider Mediterranean region (Collins et al., Reference Collins, Rust, Salih Bayraktutan and Turner2005; Vött et al., Reference Vött, Brückner, Handl and Schriever2006; Dusar et al., Reference Dusar, Verstraeten, Notebaert and Bakker2011). The trend to floodplain stabilization or slow aggradation is in accordance with climatic drying since 5.2 ka, particularly when drier conditions dominated after 4500 yr BP (Finné et al., Reference Finné, Woodbridge, Labuhn and Roberts2019). The climatic erosion sensitivity, hence, became relatively low (Dusar et al., Reference Dusar, Verstraeten, Notebaert and Bakker2011). In addition, the human disturbance in the Tekkedere catchment and the immediate vicinity might also be negligible in the second phase (Fig. 7), which altogether, promotes the development of the soil horizons.
Phase 3 (ca. 4–3.8 ka): transition from floodplain to fan environment and the onset of human impacts
The third depositional phase is characterized by the predominant deposition on the Tekkedere alluvial fan (facies B) that covered the floodplain soils (facies sCf; phase 2) and a terminal accumulation of the Bakırçay floodplain (facies C; phase 1). The mixed charcoal/sediment sample (3812–3566 cal yr BP, 363 cm bs) from the alluvial fan deposit overlying the buried soil in Tek-5 suggests the maximum depositional age of the fan sediment at this location (Fig. 6). Therefore, the transition from the floodplain (and floodplain soils) environment to the Tekkedere alluvial fan presumably took place between ca. 4 and 3.8 cal ka BP. The charcoal sample taken from the overlying fan sediments dates much later (2743–2499 cal yr BP, 273 cm bs) in Tek-5-2 which reveals a younger coverage of fan sediments at the distal location.
This remarkable transition corresponds to the Mid-/Late Holocene boundary and marks a substantial geomorphodynamic change in the area. Factors that trigger this transition from phases 1 and 2 to phase 3 likely include (1) the 4.2 ka BP drought event (Bini et al., Reference Bini, Zanchetta, Perşoiu, Cartier, Català, Cacho and Dean2019; Finné et al., Reference Finné, Woodbridge, Labuhn and Roberts2019); (2) the onset of settlement activities in the catchment area (Ludwig, Reference Ludwig and Pirson2020a; Michalski, Reference Michalski2021; Fig. 7); and (3) the internal behavior of the fluvial system (Schumm, Reference Schumm1977), for example, the (secondary) channels of the Bakırçay River shifted to the west.
The 4.2 ka BP drought event, generally corresponding to the period from 4.3 to 3.8 ka BP, shows drier conditions throughout the Mediterranean winters, in addition to already dry summers (Bini et al., Reference Bini, Zanchetta, Perşoiu, Cartier, Català, Cacho and Dean2019). Because of its global significance, it defines a formal boundary between the Mid- and Late Holocene (Walker et al., Reference Walker, Head, Lowe, Berkelhammer, Björck, Cheng and Cwynar2019). More studies suggest this climate oscillation is not just one single dry event, but a highly dynamic succession of dry and wet events (Bini et al., Reference Bini, Zanchetta, Perşoiu, Cartier, Català, Cacho and Dean2019) affecting the worldwide development of seasonal rainfalls, vegetation cover change (Shumilovskikh et al., Reference Shumilovskikh, Seeliger, Feuser, Novenko, Schlütz, Pint, Pirson and Brückner2016; Di Rita et al., Reference Di Rita, Michelangeli, Celant and Magri2022), and ensuing torrential runoff and high-frequency environmental instabilities (Türkeş and Erlat, Reference Türkeş and Erlat2005; Kuzucuoğlu et al., Reference Kuzucuoğlu, Dörfler, Kunesch and Goupille2011; Dreibrodt et al., Reference Dreibrodt, Lubos, Lomax, Sipos, Schroedter and Nelle2014; Ocakoğlu et al., Reference Ocakoğlu, Çilingiroğlu, Erkara, Ünan, Dinçer and Akkiraz2019; Lawrence et al., Reference Lawrence, Palmisano and de Gruchy2021). The change from dominating floodplain aggradation to enlarged fan development as observed in Tekkedere, therefore, probably corresponds to the global effect of the 4.2 ka drought event.
In addition to a general change in climate, after the onset of the Late Holocene, human activities started at the Kuyulu Kaya Tepe in the Tekkedere valley (Ludwig, Reference Ludwig and Pirson2020a; Michalski, Reference Michalski2021; Figs. 2A and 7). The subsequent continuous occupation and agricultural and olive cultivation in the Tekkedere drainage basin (Ludwig, Reference Ludwig and Pirson2019, Reference Ludwig and Pirson2020a; Michalski, Reference Michalski2021; Tozan, Reference Tozan and Pirson2022) accompanied by a generally dry climate caused the reduction of forest cover, as is evident from the past vegetation change in Elaia (Shumilovskikh et al., Reference Shumilovskikh, Seeliger, Feuser, Novenko, Schlütz, Pint, Pirson and Brückner2016; Fig. 7), which in turn accelerated the soil erosion processes and hillslope dynamics in the Tekkedere area.
In the Pergamon micro-region, the meta-analyses of previous studies suggest the modeled sediment facies and reconstructed geomorphodynamics in the western lower Bakırçay plain changed remarkably at ca. 4 ka, in relation to both triggers of climate change and continuous human settlements (Becker et al., Reference Becker, Knitter, Nykamp and Schütt2020a). In Elaia, terrestrial input is for the first time observed to cover marine sediments after ca. 4 ka, which is associated with human-induced catchment erosion (Pint et al., Reference Pint, Seeliger, Frenzel, Feuser, Erkul, Berndt, Klein, Pirson and Brückner2015). An analogous environmental change is marked at 4.2 ka in Mediterranean France, where soil development was buried by rainfall-induced and ongoing human-exacerbated erosion (Brisset et al., Reference Brisset, Miramont, Guiter, Anthony, Tachikawa, Poulenard and Arnaud2013). In our study, it is still a challenge to distinguish the dominating trigger between the climate and humans on the increased geomorphodynamics during this period.
Notwithstanding the climatic drying and intensifying human activities on both Tekkedere and Bakırçay catchments, the important contribution of the Tekkedere alluvial fan sediments over the Bakırçay floodplains is assumed to be due to different landscape sensitivities (Yang et al., Reference Yang, Becker, Knitter and Schütt2021), sediment (dis)connectivities, and reaction times (Goldberg and Macphail, Reference Goldberg and Macphail2006; Fryirs et al., Reference Fryirs, Brierley, Preston and Kasai2007; Fryirs, Reference Fryirs2013; Becker et al., Reference Becker, Knitter, Nykamp and Schütt2020a). In addition, the internal shift in channel patterns is observed in the Bakırçay plain (Schneider et al., Reference Schneider, Matthaei, Schlöffel, Meyer, Kronwald, Pint and Schütt2015; Becker et al., Reference Becker, Knitter, Yang, Schütt and Pirson2020b, Reference Becker, Yang, Nykamp, Doğan, Schütt and Pirson2022) and the Büyük Menderes plain (western Turkey) (Özpolat et al., Reference Özpolat, Yıldırım and Görüm2020), which cannot be excluded for the location of the recent Tekkedere fan. However, no direct evidence is available.
Phase 4 (after ca. 3.8 ka BP): alluvial fan dynamics and changing human settlement pattern
During the ensuing Late Holocene, the geomorphodynamics in the Tekkedere catchment generally increased and varied spatiotemporally compared with the former phases. The increased geomorphodynamics are evident in the overall spatial enlargement of the alluvial fan coverage, the recurring coarse-textured fan deposits, age inversions (Fig. 6), and the lack of older valley bottom sediments. The alternating fine- and coarse-textured sediments (facies Ba and Bb) with occasionally developed soils (facies sBa) correspond to event layers and temporary landscape stability.
During the last phase, the alluvial fan sediments, particularly the coarse-textured materials, were for the first time deposited at the mid–toe part of the current alluvial fan (Fig. 6). This reveals that more sediments were produced at the unstable land surface and transported along the valley bottom. Radiocarbon dating results of the alluvial fan sediments (facies B) show several age inversions (Tek-5 and Tek-4-2; Fig. 6), reflecting the reworking of older deposits stored in temporal sinks due to the intensified sediment erosion in the catchment. This corresponds to the sediment cascade model (Lang and Hönscheidt, Reference Lang and Hönscheidt1999), which has been reported in other studies in the Mediterranean region (Vita-Finzi, Reference Vita-Finzi1969; van Andel et al., Reference van Andel, Zangger and Demitrack1990; Nykamp et al., Reference Nykamp, Becker, Braun, Pöllath, Knitter, Peters and Schütt2021; Cartelle et al., Reference Cartelle, García-Moreiras, Martínez-Carreño, Muñoz Sobrino and García-Gil2022).
A comparison of local, regional, and supraregional records indicates that a general trend of aridization, rapid climate change (RCC) events, and human activities were the major triggers for the Late Holocene changing geomorphodynamics (Constante et al., Reference Constante, Peña, Muñoz and Picazo2010; Dusar et al., Reference Dusar, Verstraeten, Notebaert and Bakker2011, Reference Dusar, Verstraeten, D'Haen, Bakker, Kaptijn and Waelkens2012; Shumilovskikh et al., Reference Shumilovskikh, Seeliger, Feuser, Novenko, Schlütz, Pint, Pirson and Brückner2016; Finné et al., Reference Finné, Woodbridge, Labuhn and Roberts2019; Roberts et al., Reference Roberts, Woodbridge, Palmisano, Bevan, Fyfe and Shennan2019a, Reference Roberts, Allcock, Barnett, Mather, Eastwood, Jones, Primmer, Yiğitbașıoğlu and Vannière2019b; Becker et al., Reference Becker, Knitter, Nykamp and Schütt2020a; Fig. 7). In general, the Late Holocene hydroclimate becomes drier than the Early–Mid-Holocene (Eastwood et al., Reference Eastwood, Leng, Roberts and Davis2007; Finné et al., Reference Finné, Woodbridge, Labuhn and Roberts2019; Bozyiğit et al., Reference Bozyiğit, Eriş, Sicre, Çağatay, Uçarkuş, Klein and Gasperini2022). It shows various fluctuations in different Mediterranean regions (Dusar et al., Reference Dusar, Verstraeten, Notebaert and Bakker2011; Finné et al., Reference Finné, Woodbridge, Labuhn and Roberts2019). Centennial-scale climate oscillations between aridity and humidity during the Late Holocene have been widely reported in Anatolia, where the Earth's surface responded to the rapid events accordingly both in coastal and hinterland areas (Kuzucuoğlu et al., Reference Kuzucuoğlu, Dörfler, Kunesch and Goupille2011; Bulkan et al., Reference Bulkan, Yalçın and Wilkes2018; Bassukas et al., Reference Bassukas, Emmanouilidis and Avramidis2021; Bozyiğit et al., Reference Bozyiğit, Eriş, Sicre, Çağatay, Uçarkuş, Klein and Gasperini2022). A study in central Turkey indicates alternating humid (ca. 3.9–3.8, 3.65–3.55, 3.4–3.4 ka) and dry (3.8–3.65, 3.5–3.4, 3.25–3.0 ka) episodes, followed by an erosion crisis in 2.8–2 ka and a drying episode in the Roman climatic optimum (ca. 2–1.5 ka) (Kuzucuoğlu et al., Reference Kuzucuoğlu, Dörfler, Kunesch and Goupille2011). In southwest Turkey, stable isotope analyses further suggest wetter conditions during Classical and Early Byzantine times (Eastwood et al., Reference Eastwood, Leng, Roberts and Davis2007). The climatic drying trend, amplified by the RCC events in the wider eastern Mediterranean region, presumably triggered the repetition of the sediment dynamics in the Tekkedere catchment.
Settlement activities in the Tekkedere valley continued from the hilltop of the Kuyulu Kaya Tepe since the late Middle Bronze Age (Michalski, Reference Michalski2021). The location of settlement sites changed to flatter areas in the lower valley (Ludwig, Reference Ludwig and Pirson2020a; Tozan, Reference Tozan and Pirson2022; Figs. 2A and 7). Strategies for settlement occupation and drivers of settlement abandonment (or the changing settlement pattern) vary in different geographic locations and societies throughout time and are hence controlled by environmental and societal factors (Butzer, Reference Butzer2005, Reference Butzer2012; Boyer et al., Reference Boyer, Roberts and Baird2006; McLeman, Reference McLeman2011). Environmental triggers encompass various types of natural hazards such as RCC events, (flash) floods, earthquakes, landslides, volcanic eruptions, and the ensuing geomorphodynamics (soil erosion and mass movements) (Black et al., Reference Black, Adger, Arnell, Dercon, Geddes and Thomas2011; Dusar et al., Reference Dusar, Verstraeten, Notebaert and Bakker2011; Palmisano et al., Reference Palmisano, Lawrence, de Gruchy, Bevan and Shennan2021; Zhang et al., Reference Zhang, Cheng, Sinha, Spötl, Cai, Liu and Kathayat2021; Kennett et al., Reference Kennett, Masson, Lope, Serafin, George, Spencer and Hoggarth2022). This last factor might be important in the Tekkedere catchment.
The recurring cycles of increased geomorphodynamics during the Late Holocene were presumably triggered by past settlement locations around steep slopes in the mountainous part of the Tekkedere catchment (Fig. 2A). These settlement areas are geomorphologically sensitive to climate change and intensified land use. Hence, soil erosion and sediment dynamics may have occurred rapidly in the most sensitive areas, in particular, during the recent two millennia (Table 5). Conceivably, erosion on the land surface further results in unfavorable areas for agricultural, arboricultural, and settlement use. For this reason, a concomitant change to a more suitable settlement location, for example, the fertile and less landscape-sensitive Tekkedere valley bottom and Bakırçay floodplain, is understandable. The nearest analogous studies have been reported in Elaia (Pint et al., Reference Pint, Seeliger, Frenzel, Feuser, Erkul, Berndt, Klein, Pirson and Brückner2015; Seeliger et al., Reference Seeliger, Pint, Feuser, Riedesel, Marriner, Frenzel, Pirson, Bolten and Brückner2019) and suggest the harbor was abandoned in the Late Roman period due to progressive erosional processes and the resultant siltation.
Table 5. The summary of settlements and natural–social relationships in the Tekkedere valley.

In addition to soil erosion, earthquakes would also be an important trigger for the shifting occupation of settlements in the Tekkedere catchment. In the Pergamon micro-region, at least nine earthquakes have been documented during the last two millennia (Nalbant et al., Reference Nalbant, Hubert and King1998; Emre et al., Reference Emre, Özalp, Dogan, Özaksoy, Yildirim and Göktas2005; McHugh et al., Reference McHugh, Seeber, Cormier, Dutton, Cagatay, Polonia, Ryan and Gorur2006; Paradisopoulou et al., Reference Paradisopoulou, Papadimitriou, Karakostas, Taymaz, Kilias and Yolsal2010; Schneider et al., Reference Schneider, Matthaei, Bebermeier and Schütt2014; Seeliger et al., Reference Seeliger, Pint, Frenzel, Feuser, Pirson, Riedesel and Brückner2017). Of particular note, the abandonment of the old Tekkedere settlement (Eski Tekkedere) and its relocation to the modern village took place due to the earthquake-triggered landslide in September–November 1939 (Paradisopoulou et al., Reference Paradisopoulou, Papadimitriou, Karakostas, Taymaz, Kilias and Yolsal2010; Çelik et al., Reference Çelik, Karacakaya, Mete, Genç and Bal2019; Fig. 7, Table 5).
In addition to the geomorphodynamics, rapid cold and dry climate events or heavy and unstable rainfall have been reported to cause settlement abandonment (Weiss, Reference Weiss2016; Bini et al., Reference Bini, Zanchetta, Perşoiu, Cartier, Català, Cacho and Dean2019). Due to flood threat, rural settlements in the Pergamon micro-region were built on elevated locations (Knitter, Reference Knitter2013; Schneider et al., Reference Schneider, Matthaei, Schlöffel, Meyer, Kronwald, Pint and Schütt2015). In general, these extreme natural hazards led to relatively short-distance settlement relocation (Black et al., Reference Black, Adger, Arnell, Dercon, Geddes and Thomas2011), which explains the settlement mobility in Tekkedere.
Notwithstanding potential natural triggers, sociocultural factors also influenced settlement dynamics in Tekkedere. In the case of Kuyulu Kaya Tepe, political-administrative reasons in the Late Hellenistic–Early Roman Period (third to second centuries BC) probably led to the razing of the fortified rock plateau (Table 5). The demilitarization also happened in the neighboring hilltop settlements of Teuthrania and Eğrigöl Tepe (in the lower Bakırçay plain) and Atarneus (the Geyikli river area in Fig. 1; Schneider et al., Reference Schneider, Matthaei, Bebermeier and Schütt2014; Zimmermann et al., Reference Zimmermann, Matthaei, Ateş, Matthaei and Zimmermann2015; Williamson, Reference Williamson, McInerney and Sluiter2016; Pirson, Reference Pirson2017; Ludwig, Reference Ludwig and Pirson2020a). The reasons for the final abandonment of the Kuyulu Kaya Tepe settlement in Byzantine times are obscure, and a combination of human impacts and changing environmental conditions may have played a role. The gradual settlement relocation towards the downstream of the Tekkedere valley may have been influenced by the accessibility of major traffic routes and agricultural areas (Table 5), although other studies have attributed settlement abandonment and migration solely to human causes such as military strategies and sociocultural dynamics (Butzer, Reference Butzer2012; Schneider et al., Reference Schneider, Matthaei, Bebermeier and Schütt2014; Weiberg et al., Reference Weiberg, Hughes, Finné, Bonnier and Kaplan2019) or at most societal and environmental factors in combination (Black et al., Reference Black, Adger, Arnell, Dercon, Geddes and Thomas2011; Brown and Walsh, Reference Brown and Walsh2017).
Beyond Tekkedere, intensified sediment dynamics have been indicated from several alluvial fans and colluvium in the western lower Bakırçay plain since the Early Iron Age (Becker et al., Reference Becker, Knitter, Nykamp and Schütt2020a; Fig. 7). Since then, large settlements such as Pergamon, Elaia, Teuthrania, Halisarna, and Atarneus gradually arose and the population increased (Pirson, Reference Pirson and Jöchner2008; Pirson and Zimmermann, Reference Pirson, Zimmermann, Scholl and Pirson2014; Zimmermann et al., Reference Zimmermann, Matthaei, Ateş, Matthaei and Zimmermann2015; Pavúk and Horejs, Reference Pavúk and Horejs2018; Feuser et al., Reference Feuser, Mania, Pirson, Mohr, Rheidt and Arslan2020; Fig. 7). Among them, the transformation of Pergamon from the Hellenistic Period to the Roman Imperial Period is of greatest significance (Pirson, Reference Pirson2020). To meet the increasing demand for cultivated crops and wood as food and construction materials (Kobes, Reference Kobes1999; Laabs and Knitter, Reference Laabs and Knitter2021), Pergamon had remarkable impact on land use in rural areas, such as Tekkedere. Likewise, the ancient harbor cities (Elaia and Kane) and the monumental tumulus encountered human reshaping and construction of the land surface (Fediuk et al., Reference Fediuk, Wilken, Wunderlich, Rabbel, Seeliger, Laufer and Pirson2019; Seeliger et al., Reference Seeliger, Pint, Feuser, Riedesel, Marriner, Frenzel, Pirson, Bolten and Brückner2019; Mecking et al., Reference Mecking, Meinecke, Erkul, Driehaus, Bolten, Pirson and Rabbel2020).
Increasing landscape instability after land abandonment due to the concomitant absence of landscape management (cf. Schneider et al., Reference Schneider, Matthaei, Bebermeier and Schütt2014; Weiberg et al., Reference Weiberg, Bonnier and Finné2021) is negligible for Tekkedere valley, because continuous human occupation is recorded here for roughly the last four millennia. The same reason may also apply to the difference in geomorphodynamics between the Tekkedere and Geyikli valleys. The depositional system in Geyikli is reported to have remained generally stable during the last 4000 years (Schneider et al., Reference Schneider, Matthaei, Bebermeier and Schütt2014), which is presumably related to the limited human impacts during ca. 2.3–1.9 ka BP (Schneider et al., Reference Schneider, Nykamp, Matthaei, Bebermeier and Schütt2013).
Throughout western Anatolia, particularly during the Beyşehir occupation phase (Classical and Early Byzantine times, ca. 3.2–1.3 ka BP; Kaniewski et al., Reference Kaniewski, Paulissen, De Laet, Dossche and Waelkens2007; Fig. 7), human impacts (forest clearance, fire activities, and grazing) have had a remarkable influence on the local landscape (Eastwood et al., Reference Eastwood, Roberts and Lamb1998; Knipping et al., Reference Knipping, Müllenhoff and Brückner2007; Dusar et al., Reference Dusar, Verstraeten, Notebaert and Bakker2011; Finné et al., Reference Finné, Holmgren, Sundqvist, Weiberg and Lindblom2011; Schneider et al., Reference Schneider, Nykamp, Matthaei, Bebermeier and Schütt2013; Stock et al., Reference Stock, Laermanns, Pint, Knipping, Wulf, Hassl and Heiss2020). Likewise, continuous human activities since the Bronze Age (ca. 3.7 ka BP) may have strongly disturbed the landscape stability in Tekkedere and contributed to the ensuing colluviation, valley infill, and alluvial fan deposition in the western Yunt Dağı Mountains. The palynological archives from Elaia (Shumilovskikh et al., Reference Shumilovskikh, Seeliger, Feuser, Novenko, Schlütz, Pint, Pirson and Brückner2016), the olive cultivation (Tozan, Reference Tozan and Pirson2022) accompanied by deforestation, and the occurrence of ceramic fragments in profile Tek-5 (Fig. 4) point to increased human activities in the Tekkedere catchment, though the duration and intensity of activities in different locations of the valley are currently unclear (Ludwig, Reference Ludwig and Pirson2020a; Michalski, Reference Michalski2021; Tozan, Reference Tozan and Pirson2022; Fig. 7).
The infills of the Tekkedere valley bottom (facies A) date younger than ca. 1300 yr BP, which again reveals the removal of old materials by former increased geomorphodynamics was followed by ongoing sediment accumulation and a corresponding phase of increased erosion. The dynamics of fine- and coarse-textured sediments (facies Aa and Ab) and the local initial soil formation (facies sAa) in the Tekkedere valley bottom also suggest the erosion and stability repeated and varied spatiotemporally in the catchment during the last 1300 years. A similar process that resulted in substantial siltation is reported in Elaia, which continued during ca. 1.8–1.1 ka (Seeliger et al., Reference Seeliger, Bartz, Erkul, Feuser, Kelterbaum, Klein, Pirson, Vött and Brückner2013, Reference Seeliger, Pint, Feuser, Riedesel, Marriner, Frenzel, Pirson, Bolten and Brückner2019; Shumilovskikh et al., Reference Shumilovskikh, Seeliger, Feuser, Novenko, Schlütz, Pint, Pirson and Brückner2016). The onset of the modern trend toward aridity about 1300 years ago in southwest Turkey (Eastwood et al., Reference Eastwood, Leng, Roberts and Davis2007), the transition to the Little Ice Age (Kuzucuoğlu et al., Reference Kuzucuoğlu, Dörfler, Kunesch and Goupille2011), and the various land use around the local settlements in Tekkedere (Fig. 7) together have the potential to have influenced the geomorphodynamics during the last millennium.
CONCLUSIONS
The geoarchaeological investigation of the Tekkedere alluvial fan and its catchment is the first detailed study that focuses on the Mid- to Late Holocene geomorphodynamics through alluvial sediments from a long-term settled valley in the hinterland of a major ancient city in western Turkey, the city of Pergamon. Sediment analyses, facies interpretation, and radiocarbon dating results suggest four principal phases of changing geomorphodynamics after 6.2 cal ka BP. In the first phase, before ca. 5–4 ka, the aggradation of the Bakırçay plain, that is, the deposition of still water sediments, channel beds, and floodplain sediments, dominated local geomorphodynamics. This period is generally characterized by rising sea level and the relatively humid Middle Holocene climate in western Anatolia. Alluvial soils that formed on the Bakırçay floodplain during phase 2 reflect a decline of the Bakırçay floodplain aggradation and low sediment dynamics in the Tekkedere catchment during a climatic phase of decreasing humidity between ca. 5 and 4 ka. The deposition of alluvial fan sediments from the Tekkedere valley was of low significance before ca. 4 ka. In the transition from the Middle to Late Holocene, the floodplain soils were buried by Tekkedere alluvial fan deposits (phase 3), pointing to the notably intensified geomorphodynamics in the Tekkedere catchment in comparison to the first two phases. This notable change is attributed to the supraregional trend of aridization and RCC events between 5 and 3 ka, superimposed by the onset of local human activities from the late Middle Bronze Age (ca. 3.7 ka) in the catchment. In the last phase, the indications of the enlarged fan coverage, the repetition of fine- and coarse-textured fan deposits, and the reworking of older sediments suggested by age inversions and young valley infills reveal increased geomorphodynamics during the Late Holocene. This increase is associated with the varying environmental conditions and natural hazards, soil erosion, and earthquake-triggered landslides, which largely affected local geomorphodynamics and consequent settlement activities.
A clear relationship between the climate variations, the changing settlement patterns, and geomorphodynamics, especially during the Late Holocene, remains unclear due to several age inversions that make the timing of erosion events challenging. Nonetheless, the evidence from Tekkedere suggests that the transformation of large settlements (e.g., Pergamon and Elaia) from the Hellenistic Period to the Roman Imperial Period contributed to landscape change in rural areas, though the small catchment of Tekkedere reacted more sensitively to human activities than the higher-order catchment of the Bakırçay River. The ongoing pressure from local land use promotes the accumulation of the relatively young valley infills.
Acknowledgments
The research was carried out as part of the project “The Transformation of the Pergamon Micro-Region between the Hellenistic Period and the Roman Imperial Period” (https://www.dainst.blog/transpergmikro, accessed March 2022). The fieldwork was conducted as part of the Pergamon Excavation of the Istanbul Department of the German Archeological Institute (DAI), for which Felix Pirson holds the license. We would like to express our special thanks for the kind permission and support from the Ministry of Culture and Tourism of the Republic of Turkey and Ayşe Tok (Directorate Museum Demre) who took part in the works as a government representative. Murat Tozan and geography students from Ege University Izmir (Nami Yurtseven, Minel Korkmaz, and Elif Gültekin) and Freie Universität Berlin (Lena Schimmel) are acknowledged for their help during fieldwork and laboratory analyses. We also appreciate the help of colleagues from the DAI (Felix Pirson, Ulrich Mania, Paula Michalski, and Nicole Neuenfeld), Manisa Celal Bayar Üniversitesi (Güler Ateş), and the Bergama Museum. The authors declare that they have no known competing financial interests or personal relationships that could have appeared to influence the work reported in this paper.
Financial Support
We would like to acknowledge financial support from the China Scholarship Council (grant no. 201906190216) and Deutsche Forschungsgemeinschaft (German Research Foundation; grant number 419349690).
Supplementary Material
The supplementary material for this article can be found at https://doi.org/10.1017/qua.2022.73.