INTRODUCTION
Considered as two ‘colliding epidemics’, tuberculosis (TB) and tobacco lead to 1·8 and 5·4 million deaths per year, respectively [1]. Active smoking increases the risk of acquiring TB infection and its progression to TB disease [Reference Lin, Ezzati and Murray2–4]. TB patients who smoke have an increased risk of treatment failure, relapse, and mortality than those who do not [Reference Batista5–7]. Approximately 20% of the TB disease burden is attributable to smoking [Reference Brands8]; based on current smoking trends, it is predicted to lead to an extra 18 million TB cases and 40 million TB deaths between 2010 and 2050 [Reference Basu9]. Earlier reviews also point towards a plausible association between exposures to second-hand smoke (SHS) and TB [Reference Lin, Ezzati and Murray2, Reference Slama3]. Tobacco smoke impairs the ciliary function of the airways leading to altered bacterial clearance, decreases T-cell immunity and alters the phagocytic ability of the pulmonary alveolar macrophages disabling the early defence mechanism of the lungs to clear the invading mycobacteria [Reference Di10, Reference Van Zyl-Smit11].
SHS exposure leads to cardiovascular diseases, chronic respiratory diseases, lung cancer in non-smoking adults [Reference Coultas12, Reference Rushton13], and asthma, lower respiratory infections, chronic middle ear disease, sudden infant death syndrome and meningitis in children [Reference Murray, Britton and Leonardi-Bee14, Reference Cook and Strachan15]. Recognizing SHS as a public health threat, most countries have introduced comprehensive smoking bans in enclosed public and work places. In countries where these bans are strictly enforced, this has significantly reduced exposure to SHS and its associated morbidity and mortality [Reference Been16]. However, for most non-smoking women and children, homes and cars remain the more important sources of SHS exposure. In countries, with high TB burden, particularly those where unrestricted smoking in homes is commonplace; non-smoking women and children are extremely vulnerable to any TB-related risks associated with SHS. Despite this potential threat, the role of SHS exposure in TB is not well documented.
In 2007, a systematic review examined associations between TB, smoking and indoor air pollution and found a handful of small studies indicating that SHS exposure increases the risk of TB disease [Reference Lin, Ezzati and Murray2]. Another systematic review, in 2007, indicates a moderate association between SHS and TB disease, although there was limited evidence to support any association between SHS and TB infection [Reference Slama3]. Since then, more evidence has emerged on this topic, prompting us to conduct a dedicated systematic review on SHS and TB. We were interested in two questions: (a) What is the effect of SHS exposure in households, on the risk of acquiring TB infection and developing disease in non-smokers, both with and without the presence of a TB patient in their household? (b) What is the effect of SHS exposure in households, in non-smoker TB patients on their disease/treatment outcomes such as sputum conversion, treatment success (both cured and completed), treatment default, death, relapse, and recurrence?
METHODS
Search
The databases searched for relevant publications between January 1991 and May 2014 included Medline, EMBASE and PsycINFO by K.S. (last author/investigator) with the key words: ‘tobacco smoke pollution’; ‘passive, second-hand’; ‘second hand’; ‘involuntary, parent*’; ‘maternal, mother*’; ‘paternal’; ‘spouse’; ‘wife’; ‘husband’; ‘household*’; ‘smok*’; ‘tobacco*’; ‘cigarette*’; ‘hookah’; ‘huqqa’; ‘shisha’; ‘sheesha’; ‘bidi’; ‘water pipe’; ‘waterpipe’; ‘cigar’; ‘tuberculosis’; ‘TB’; ‘pulmonary tuberculosis’; ‘pulmonary TB’; ‘acid fast bacilli’; ‘Mycobacterium tuberculosis’; ‘Koch's disease’. Furthermore, we scanned reference lists of the included studies, searched for citations in the International Journal of Tuberculosis and Lung Diseases, Tobacco Control, Nicotine and Tobacco Research, and Addiction published in the last 10 years, and used the Conference Proceedings Citation Index: Science (ISI) on Web of Knowledge (May 2014) to identify relevant conference abstracts. We imposed no language restrictions. Abstracts of the articles identified in languages other than English were translated using Google Translate.
Selection criteria
Study design
All comparative epidemiological studies (case-control, cross-sectional, repeat cross-sectional, and cohort designs) were included.
Exposure and comparators
All SHS exposures (from parents, carers, siblings, spouses, partners, etc.) either self-reported or measured by biochemical markers (cotinine levels in saliva, blood, urine, hair or PM2·5 measurements in households) were included. Papers reporting on indoor air pollution were excluded.
Outcome measures
For TB infection, Mantoux test [tuberculin skin test (TST)] with a specified cut-off of induration size or more specific tests [like interferon gamma release assays (IGRAs)] were considered acceptable. For TB disease, sputum smear positive for acid-fast bacilli and/or a culture positive for Mycobacterium tuberculosis were considered. An acceptable measure included clinical, radiological, molecular (Xpert) or histological diagnosis in addition to expected response to anti-TB treatment or notification in TB registers. A number of other outcomes were included, sputum conversion, culture conversion, treatment success (cured plus treatment completed), treatment default, death, relapse and recurrence.
Participants
For TB risk, we included studies on non-smoking people of all ages with no history of TB. For TB outcomes, we included studies on non-smoking people of all ages with pulmonary TB. Active smokers were excluded from the analysis, as this would have confounded any association between SHS exposure and risk of TB and its outcomes. Participants with extra-pulmonary TB were also excluded.
Data extraction
All citations were assessed for eligibility by first screening the titles and abstracts by two authors (K.S., R.Z.) independently, yielding 19 eligible publications based on the selection criteria described. The full texts of the eligible papers were then reviewed by four authors (K.S., N.P., N.S., R.Z.) independently; two authors (K.S. and N.S.) reviewed studies related to SHS and TB infection, while the other two authors (N.P. and R.Z.) reviewed studies related to SHS and TB disease. Disagreements were resolved with other authors (O.D., S.S.). Data were extracted by two authors (K.S., N.P.), using a pre-structured tool. This included: study location, research design, participants, settings, exposure, outcomes, adjusted variables, and study findings.
Risk of bias (quality) assessment
All selected papers were critically appraised by three authors (N.P., K.S., R.Z.) and were scored for methodological quality using the Newcastle–Ottawa Assessment Scale (maximum score 9). This assessment included how the authors handled confounders either in the design or in the analysis. This scale uses a ‘star system’ in which a study is judged on three broad perspectives: the selection of the study groups; the comparability of the groups; and the ascertainment of either the exposure or outcome of interest for case-control or cohort studies, respectively. For the scope of our investigation, a score of ⩾7 was taken as the threshold for a good quality study. As we had <10 studies for each outcome of interest, we assessed publication bias by using Begg's funnel plot by visual inspection for asymmetry [Reference Higgins and Green17, Reference Begg and Mazumdar18].
Data analysis
Analyses were performed in general accordance with MOOSE (Meta-analysis of Observational Studies in Epidemiology) guidelines [Reference Stroup19]. All analyses were performed using RevMan (Review manager) program (version 5.3), developed for Cochrane meta-analyses. We calculated risk ratios (RRs) for each outcome, summarizing the individual study estimates and the overall estimate, using random-effects models [Reference Mosteller and Colditz20]. Further, subgroups were defined by the presence of a TB contact and study population (children or adults). Poor overlap of confidence intervals (CIs) between individual studies and statistically significant Cochran's Q (a χ 2 test) were considered as evidence of heterogeneity. To quantify the degree of inconsistency between the studies’ results, the I 2 statistic (I 2 = 100% × (Q – df)/Q, where Q is Cochran's heterogeneity statistic and df, the degrees of freedom) was also computed for each outcome [Reference Higgins21]. To further explore these sources of heterogeneity, we took an informative approach and performed sensitivity analyses by study characteristics relating suspected sources of bias and variability to the study findings based on the I 2 statistic [Reference Stroup19, Reference Higgins and Green22].
FINDINGS
Overview
Out of 428 citations found through the literature search (Fig. 1), only 12 met the inclusion criteria (Table 1). Out of these, six examined the association between SHS and risk of TB infection and six risk of TB disease. Five of the eligible studies included participants that lived with a TB patient, three for TB infection and two for TB disease. We did not find any studies examining associations between SHS exposure and TB treatment-related outcomes.
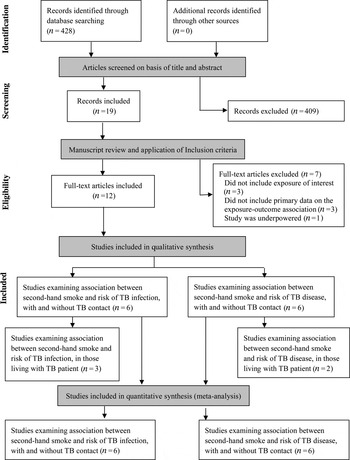
Fig. 1. Flow diagram of study selection.
Table 1. Studies included in the systematic review
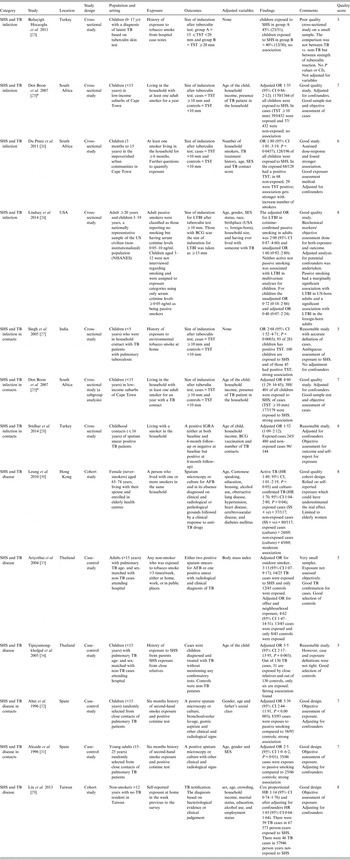
AFB, Acid-fast bacilli; BCG, bacillus Calmette-Guérin; CI, confidence interval; HR, hazard ratio; IGRA, interferon-gamma release assay; LTBI, Latent tuberculosis infection; OR, odds ratio; SES, socioeconomic status; SHS, second-hand smoke; TST, tuberculin skin test.
* Den Boon et al. [Reference Den25]; both studies are in the same paper but the latter one is a subgroup analysis of the original study.
For TB infection, all six studies used cross-sectional design and investigated the effects of SHS in children except one (Lindsay et al. [Reference Lindsay24]) that included both adults and children. For TB disease, two studies used a prospective cohort design, the remainder (n = 4) used case-control designs; one study investigated the effect of SHS in young people (15–24 years), two in adults (one >15 years, one >12 years), two in children and one in older women (65–74 years). All studies examining TB infection used the Mantoux (TST) test with acceptable but varying [one study (20 mm), three studies (10 mm), one study (15 mm for bacillus Calmette-Guérin (BCG) scar, 10 mm otherwise)] cut-offs for induration size except for a study by Sridhar et al. [Reference Sridhar28], which used IGRA positivity for diagnosis. For TB disease, two studies used sputum smear microscopy, while three used either sputum smear microscopy or culture to confirm TB; one study did not specify measures used for diagnosis [Reference Tipayamongkholgul34]. Only three studies [Reference Lindsay24, Reference Alcaide31, Reference Altet32] confirmed exposure to SHS using biochemical measures (cotinine testing). The rest relied on self-reports, using a range of definitions.
The methodological quality was mixed. Newcastle–Ottawa score (NOS) ranged from 3 to 8 (median 7). Eight studies scored ⩾7; the highest score of 8 was given to the two cohort studies for TB disease and two cross-sectional studies from 2014 for TB infection. The key methodological issues included weak study designs (cross-sectional) for establishing causality and temporality, non-standardized case definitions of TB and self-reported exposure to SHS.
SHS and TB infection
Children exposed to SHS had greater risk of acquiring TB infection (RR 1·19, 95% CI 0·90–1·57) compared to the non-exposed but this association did not reach statistical significance (Fig. 2). To reduce variability in the meta-analysis, we did not include adults’ data, which was reported in only one study [Reference Lindsay24]. Despite this exclusion, test for heterogeneity was statistically significant (I 2 = 74%, P = 0·0008) indicating marked variability between studies’ results. Two of the six studies [Reference Babayigit23, Reference Lindsay24] showed no association, probably because the researchers used an induration cut-off >10 mm (indicative of TB infection); this has been explored further in sensitivity analyses (Supplementary Figs S1 and S2). The study by Babayigit Hocaoglu et al. [Reference Babayigit23] scoring very low on quality, used a case-note analysis on a small number of children (n = 81) in an urban hospital in Turkey [Reference Babayigit23]. On the other hand, Lindsay et al.'s [Reference Lindsay24] study, scoring high on quality, used a national survey dataset with biochemically verified exposure and outcome measures [Reference Lindsay24].
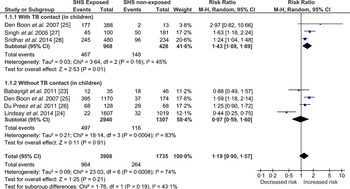
Fig. 2. Forest plot of studies that examined second-hand smoke and tuberculosis (TB) infection (with and without TB contact).
In another study in South Africa, researchers asked parents about the history of tobacco smoking in homes of children with a positive Mantoux test [Reference Den25]. This study, embedded within a TB contact tracing programme, also found significant association between TB infection and SHS but when adjusted for age, income and TB contact, the significance was not maintained. Another well-conducted cross-sectional study, also in Cape Town, found an association between SHS and TB infection that became intensified with increasing exposure [Reference Du26]. The researchers gathered detailed history of exposure and adjusted their analysis for number of household smokers, TB treatment history, age, socioeconomic status and TB contact; controlling for confounders did not affect the association.
Two studies [Reference Singh27, Reference Sridhar28] were conducted in TB contacts, while one study [Reference Den25] reported additionally on cases that had TB contact. The study by Singh et al. [Reference Singh27] followed up on TB contacts (children) and found that these children were 2–3 times more likely to become infected with TB if exposed to SHS. The study was ambiguous on the methods and definitions for assessing SHS. A recently published study [Reference Sridhar28] using data from a Turkish cohort of child contacts of TB patients scored high on quality characteristics and used IGRA (a more specific test than TST) for indication of TB infection. The study showed a positive association between SHS and TB infection in child contacts of TB patients; adjusting for age of the child, BCG vaccination, household income and number of TB contacts did not affect the magnitude or direction of the association. One of the above-mentioned South African studies also conducted a post-hoc analysis on a TB contacts’ (children) subgroup and found a significant and strong association between TB infection and SHS [Reference Den25].
Two types of sensitivity analysis were conducted (Supplementary Figs S1 and S2), to explore the observed heterogeneity. Supplementary Figure S1 shows the pooled estimates of studies grouped by the quality assessed using NOS. Heterogeneity remained high (I 2 = 84%, P = 0·0003) in the good-quality studies, most likely attributed to the Lindsay et al. study [Reference Lindsay24] where TST >15 was used for patients with BCG scar. Based on this definition there were four children who had induration between 10 mm and 15 mm but were not categorized as having TB infection [Reference Lindsay24], which changes the case-definition from the rest of the included studies. To explore this heterogeneity further, Supplementary Figure S2 shows the pooled estimates of studies grouped by the TB diagnostic criteria; about 68% (I 2 = 68%, P = 0·17) of the heterogeneity was contributed by the two studies [Reference Babayigit23, Reference Lindsay24] that used TST induration cut-offs >15 mm. The between-group (test type/measurement) differences accounted for 72% (I 2 = 71·8%, P = 0·03) of variation, meaning the observed variation between the studies might be due to the differences in the quantification or measurement (dependent on the diagnostic criteria or test used) for TB infection. The study by Babayigit Hocaoglu et al. [Reference Babayigit23] used a TST induration cut-off of 20 mm, which could potentially underestimate the actual number of TB-infected cases. This study also rated low on quality assessment. A statistically significant positive association (RR 1·39, 95% CI 1·19–1·62) between SHS and TB infection was found when these two studies [Reference Babayigit23, Reference Lindsay24] were excluded from analysis.
The evidence presented in the above studies is relatively scanty and weak. Moreover, it is not possible to infer causality from the findings of cross-sectional studies. However, our findings do indicate a plausible association between SHS exposure and acquisition of TB infection that needs to be explored further with longitudinal studies to establish causality. Further, assessing exposure to SHS based on self-reports could be subject to bias as only one study (recently published) measured exposure to SHS using biochemical tests. A funnel plot (Fig. 3) appears symmetrical, suggesting little possibility of a reporting bias.
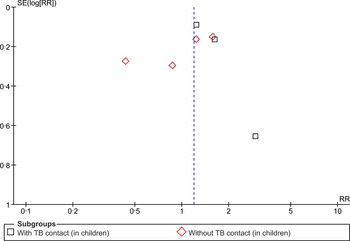
Fig. 3. Begg funnel plot for studies that examined second-hand smoke and tuberculosis infection.
SHS and TB disease
In this category, we present the meta-analysis (Fig. 4) of the included studies – two cohort [Reference Lin29, Reference Leung30] and four case-control [Reference Alcaide31–Reference Tipayamongkholgul34] studies. Those exposed to SHS were found to have greater risk (RR 1·59, 95% CI 1·11–2·27) of developing TB disease. The test for heterogeneity was statistically significant (I 2 = 77%, P = 0·0006) indicating high inconsistency between studies’ results that cannot otherwise be explained by chance. This might be due to substantial heterogeneity in the study design, participants (children vs. adult), study quality and/or outcome measures (sputum smear microscopy vs. culture confirmed). This has been further explored in the sensitivity analyses (Supplementary Figs S3–S5).
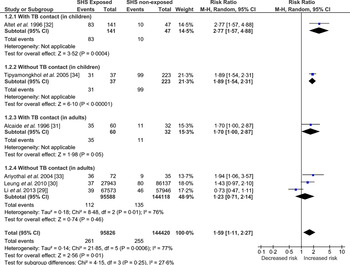
Fig. 4. Forest plot of studies that examined second-hand smoke and tuberculosis (TB) disease (with and without TB contact).
One case-control study, conducted in Thailand, found that the odds of exposure to SHS in children with TB disease were almost nine times that in non-TB controls, when adjusted for age, average number of persons per room and the frequency of illness [Reference Tipayamongkholgul34]. However, the SHS exposure was not assessed biochemically and the test for the diagnosis of TB disease was also not apparent in text; the study scored low on quality criterion. Another case-control study [Reference Ariyothai33] conducted in Thailand, in adults (>15 years) with TB and age- and sex-matched non-TB cases as controls, found that those exposed to SHS were 4·6 times more likely to develop TB disease. However, the study scored low on quality assessment.
A cohort study conducted in a group of >15 000 elderly women in Hong Kong found that those women who lived with a smoker spouse were 1½ times more likely to develop TB compared to those living with a non-smoker spouse [Reference Leung30]. Due to its prospective design, large sample size and laboratory-confirmed TB cases this study has high validity. Another cohort study [Reference Lin29], published recently did not find a statistically significant association between SHS and TB disease. The study was conducted in Taiwan by prospectively following participants of the National Health Interview Survey and scored high on quality assessment.
Two Spanish-linked case-control studies in TB contacts, one in children (<15 years) [Reference Altet32] and the other in young people (15–25 years) [Reference Alcaide31] found that those exposed to SHS were five and three times more likely to develop TB disease, respectively. Cases were confirmed using laboratory diagnosis and SHS exposure was assessed using cotinine. The findings of these two well-conducted studies are not definitive but highly suggestive of an association between SHS exposure and TB disease in TB contacts.
Three types of sensitivity analysis were conducted (Supplementary Figs S3–S5) to explore the observed heterogeneity. Supplementary Figure S3 shows the pooled estimates of studies grouped by quality, assessed using NOS. Heterogeneity remained high in the good-quality studies, most likely attributable to the two cohort studies as they differed considerably in the characteristics of the target population. The Leung et al. [Reference Leung30] study was conducted in older (65–74 years) females and the Lin et al. [Reference Lin29] study was carried out in the population aged ⩾12 years, where data could not be separated out for children and adults. To explore this heterogeneity further, Supplementary Figure S4 shows the pooled estimates of studies grouped by study design (case-control vs. cohort); about 81% (I 2 = 81%, P = 0·02) of the heterogeneity was contributed by these two cohort studies [Reference Lin29, Reference Leung30]. Between-group differences (case-control vs. cohort design) accounted for 70% (I 2 = 70·2%, P = 0·07) of variation; however, the strength of evidence for heterogeneity (based on the P value) was weak, meaning the observed variation between the studies was due to the internal factors of the two cohort studies and not because of the pooling of case-control with cohort study designs. The test for subgroup differences was not statistically significant for the sensitivity analysis grouped by diagnostic test for TB (Supplementary Fig. S5).
The asymmetry of the distribution in a funnel plot (Fig. 5) suggests potential for publication bias.
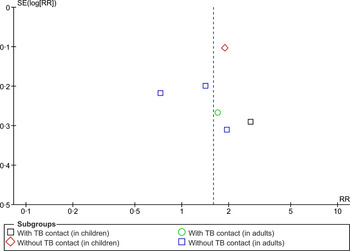
Fig. 5. Begg funnel plot for studies that examined second-hand smoke and tuberculosis disease.
DISCUSSION
This review found moderate evidence to suggest an association between SHS exposure and the risk of developing TB disease. The risk might be higher in children and TB contacts. The dose-response relationship indicates that the association is likely to be causal. However, given that most studies were cross-sectional, it is almost impossible to infer causality on that basis. We also found some evidence for a possible association between SHS exposure and the risk of TB infection. However, due to the weak study designs this evidence remains rather limited and statistically insignificant, although highly plausible. Evidence on any association between SHS exposure and TB outcomes remains largely unknown.
Strengths and limitations
We systematically reviewed literature on an area of global public health importance. Our choice of key words, both for TB and SHS, were consistent with those used in published systematic reviews. In line with the standard review methods [35], we included 2–3 researchers at each step. We consider our findings an advance from the previous reviews on this topic [Reference Slama3, Reference Brands8] as we found seven additional studies (five cross-sectional studies for TB infection and two cohort studies for TB disease) on the topic. However, our conclusions are consistent with and provide further strength to those drawn by the previous reviewers. Our findings are also consistent with the evidence on associations between TB and active smoking [Reference Siddiqi and Lee36].
Our review included only a limited number of studies, nearly half of which did not meet the quality threshold. With the exception of two, no cohort designs were used making it difficult to infer causality. In addition, most studies had relatively small sample sizes, increasing their probability of missing an actual association purely by chance. If there were more studies or larger sample sizes of the included studies it would have given more precise estimates with more confidence in the findings presented. Most studies relied on self-reported SHS exposure, using a variety of definitions. Similarly, the diagnostic measurement criteria differed between studies included in TB infection, making it more likely to miss potential TB-infected cases in some studies with higher diagnostic cut-offs then recommended. This could lead to misclassification bias. It has been found that only one third of parents report their children being exposed to SHS [Reference Eriksen37]; the underreporting by parents might be more if children are diagnosed with TB, due to any perceived guilt in admitting responsibility. On the other hand, recall bias could have led to an overestimation of an association in case-control studies, as people are generally more likely to recall an exposure when ill than when healthy. Most case-control studies used hospital-based controls or relatives as controls, these are more likely to be exposed to SHS biasing the results toward the null [Reference Wacholder38]. A few studies did not provide information on the potential confounders and any potential adjustments required in the analysis.
While our review could not identify any studies that examined an association between SHS and TB treatment outcomes, it is plausible that such an association does exist based on the literature on active smoking. Tobacco smoking not only contributes to acquisition of TB infection and its progression to active disease but also has profound effects on TB treatment outcomes, such as death, default (non-adherence to treatment), and treatment failure or relapse [Reference Doll39, Reference Peto, Chen and Boreham40]. Smoking by TB patients also causes more cavitary lesions [Reference Altet-Gomez41], increasing the duration of conversion of positive sputum culture to negative [Reference Onyebujoh42].
Policy and research implications
Our findings are of particular relevance to those in low- and middle-income countries, where TB is endemic, and tobacco smoking is also increasingly becoming common, visible and socially acceptable. Due to limited awareness of the harms of SHS, adults in such countries often do not implement any smoking restrictions at home [Reference Siddiqi43]. Children, who are mostly exposed to SHS in their homes, are at particular risk. Policy-makers need to acknowledge this risk and in addition to the other legislative initiatives to restrict smoking in public places, should also run awareness-raising campaigns on the harms of smoking at home. Our review provides additional evidence to support such campaigns. Health professionals can make parents aware of the risks of SHS when dealing with sick children. Such messages should be integrated within TB programmes due to their ability to reach large populations. Avoiding SHS exposure should be a part of any TB control plan.
While our review provides evidence to support an association between SHS and TB, it also highlights widespread inconsistencies in assessing SHS exposure both in self-reports and biochemical measures. Like Russell's Standard for active smoking [Reference West44], there needs to be an agreement on a set of standards for assessing outcomes in SHS studies. We also need more longitudinal studies to confirm causality between SHS exposure and TB infection, disease and outcomes. However, such studies are usually expensive and time consuming. On the other hand, TB programmes in many countries have a well-organized data collection system both for patients and contact tracing and it should be possible to design longitudinal studies.
Innovations such as Electronic Nicotine Delivery Systems (ENDS) pose new challenges for researchers and policy-makers. The use of ENDS, which vaporizes tobacco smoke-free nicotine, could benefit smokers by reducing their personal harm related to combustion of tobacco and non-smokers who would otherwise be exposed to SHS generated by burning tobacco [4]. However, there may be additional harms related to nicotine itself as provided by ENDS, as it is an immunomodulator in the lungs. Therefore, this potential of reduced harm by switching from burned tobacco to ENDS needs more research and careful consideration before making substantive recommendations. We also need research on educational interventions encouraging people to implement voluntary smoking restrictions in the home.
SUPPLEMENTARY MATERIAL
For supplementary material accompanying this paper visit http://dx.doi.org/10.1017/S0950268815001235.
ACKNOWLEDGEMENTS
There was no funding source for this paper.
DECLARATION OF INTEREST
None.