In bivalve molluscs the ligament joins both valves dorsally and, being flexible, provides the thrust for opening the shell, acting as the resistance in a lever system against which contraction of adductor muscles acts as effort (Owen et al. Reference Owen, Trueman and Yonge1953). The relative position of resistance, effort and fulcrum allows for the distinction of three classes of levers, only the first two being of interest here. In levers of class 1, the fulcrum is located between the two forces, which act in the same direction although at either side of the fulcrum (Fig. 1A). In levers of class 2, the resistance is located between the fulcrum and the effort, which acts in opposite direction to the resistance (Fig. 1B). Usually, in an external parivincular ligament, the outer lamellar layer is subjected to tensile stress, while the inner fibrous layer is exposed to compression, with the pivotal axis (representing the fulcrum of the lever system) located between them (Trueman Reference Trueman1949). The effort (i.e., the action of the adductor muscles) is always ventral to the pivotal axis; tensile stress develops dorsal to the pivotal axis and in the same direction as the effort, constituting the resistance of a class 1 lever, while compression develops ventral to the pivotal axis and in the opposite direction with respect to the effort, hence resulting in a class 2 lever (Fig. 1C). When a concave dorsal margin is developed, the pivotal axis is shifted dorsally to a line connecting the umbo and the elevated postero-dorsal angle of the shell, and so the tensile stress of the lamellar layer, now ventral to the pivotal axis, would prevent the valves from opening (Fig. 1D).
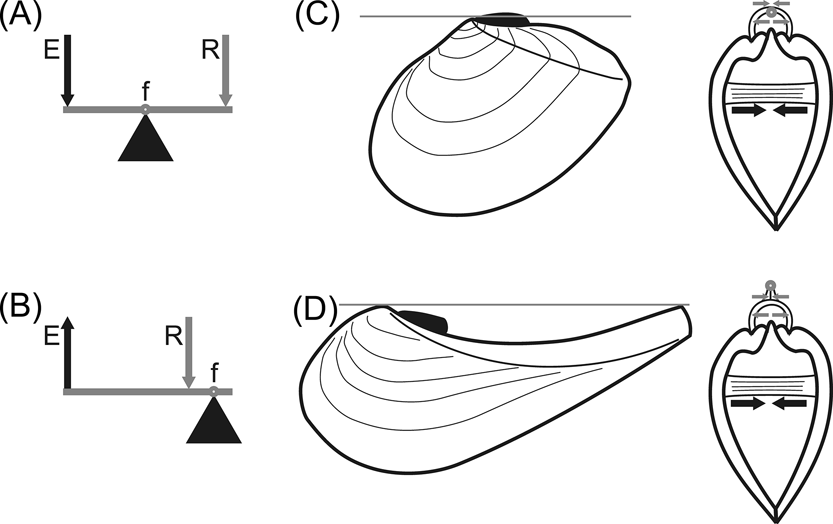
Figure 1 Bivalve shell as a lever system. (A–B) schematic drawings of class 1 (A) and class 2 (B) levers. Abbreviations: f = fulcrum; R = resistance; E = effort. (C–D) diagrams showing working of ligaments in prosogyrate (C) and opisthogyrate (D) shells. Grey line (on the left) and small grey circle (on the right) show the position of the pivotal axis; grey arrows indicate the direction of action of the resistance of both ligamental layers: lamellar (upper arrows, acting by tensile stress) and fibrous (lower arrows, acting by compression); black arrows indicate the direction of action of the effort, provided by the adductor muscles. Note in (D) that the resistance provided by the lamellar layer, being ventral to the pivotal axis (as is the effort) would prevent the valves from opening. Modified and extended from Yonge & Thompson (Reference Yonge and Thompson1976).
A prosogyrate shell shape, generally associated with the development of a lunule, is currently very frequent in shallow-burrowing bivalves, and is considered of adaptive value during burrowing (Stanley Reference Stanley1975a, Reference Stanleyb). On the other hand, an opisthogyrate shell shape is unusual amongst burrowing bivalves, with the main exception being the order Trigoniida (Paleoheterodonta), where this condition is very common (Stanley Reference Stanley1977, Reference Stanley1978). The most extreme cases are the crescentic shells of many Pterotrigoniinae. These frequently have a produced posterior margin generating a rostrum, resulting in a concave escutcheon. Thus, the umbo and the postero-dorsal angle of the shell project dorsally relative to the ligament, which then fails to act as a pivotal axis.
A constructional morphology approach was employed in order to understand the mechanical operation of the shell–ligament system in the Pterotrigoniinae. Ligament structure and evolution have been extensively treated in the literature and their detailed discussion is beyond the scope of this contribution. Neotrigonia Cossmann, Reference Cossmann1912 is the only extant trigoniides genus but, unfortunately, it strongly differs from Pterotrigonia v. Hoepen, Reference Hoepen1929 in shell shape, as it is orthogyrate and triangular-shaped (Stanley Reference Stanley1978; Morton Reference Morton1987a). Two other opisthogyrate rostrate extant genera of unrelated lineages were also analysed: Nuculana Link, Reference Link1807 (Palaeotaxodonta) and Cuspidaria Nardo, Reference Nardo1840 (Anomalodesmata). The rostrate, crescentic shape is infrequent in both Palaeotaxodonta and Anomalodesmata, suggesting a convergent development of this shell morphology in both lineages and in the trigoniides. These three taxa have few other common features: they differ in feeding habits, hinge and ctenidia, but they are all shallow burrowers. The particular shell morphology developed by all these taxa is probably related to this life habit: opisthogyrate growth favours an upward orientation of the posterior margin, making it easier for the animal to reach the water–sediment interface. The rostrum would thus allow the animal to reach the water column while most of the body remains below the sediment surface (Stanley Reference Stanley1977), in the same way as a snorkel allows a diver to maintain contact with the atmosphere while the body is underwater. Within the septibranchs, the rostrum is probably an adaptation for rapid eversion of the raptorial siphon (Morton Reference Morton1981, Reference Morton1987b). Also, the reduced size of the posterior end of the shell would reduce scour around it (Stanley Reference Stanley1977). Interestingly, both Nuculana and Cuspidaria are siphonate species, unlike Pterotrigonia, and they both generate water currents by pumping action, due to muscular contractions of the ctenidia (Yonge Reference Yonge1928, Reference Yonge1939).
1. Material and methods
The following trigoniide samples were analysed:
Scabrotrigonia thoracica (Morton, Reference Morton1834): USNM-PAL 636024, USNM-PAL 636032, USNM-PAL 129702, USNM-PAL 130844, USNM-PAL 386648.
Scabrotrigonia eufaulensis (Gabb, Reference Gabb1860): USNM-PAL 636026, USNM-PAL 27923, USNM-PAL 28435, USNM-PAL 305123.
Myophorella garatei Leanza, Reference Leanza1981: MCF-PIPH 427–429, MOZ-PI 930, MOZ-PI 933, MOZ-PI 7192–7194.
Myophorella coihuicoensis (Weaver, Reference Weaver1931): MCF-PIPH 484, MCF-PIPH 486, MCF-PIPH 488–489, MCF-PIPH 491–492, MOZ-PI 1687, MOZ-PI 7186–7188, MOZ-PI 8974–8975, MOZ-PI 8977– 8978.
Myophorella volkheimeri Leanza & Garate, Reference Leanza, Garate Zubillaga and Volkheimer1987: MCF-PIPH 459, MOZ-PI 1752, MOZ-PI 4173, MOZ-PI 7197–7198, MOZ-PI 7201–7204, MOZ-PI 8979.
Pterotrigonia coheni Leanza, Reference Leanza1993: MCF-PIPH 385, MCF-PIPH 389.
Pterotrigonia transatlantica (Behrendsen, Reference Behrendsen1892): MOZ-PIPH 2953, MOZ-PIPH 3072, MOZ-PIPH 3189, MOZ-PIPH 4397, MOZ-PIPH 4400.
Steinmanella caicayensis Lazo & Luci, Reference Lazo and Luci2013: MCF-PIPH 410, MCF-PIPH 412, MLP-PI 34629–34630.
Neotrigonia spp.: USNM 41485, USNM 88769, USNM 162080, USNM 465397, USNM 637181, USNM 76059, USNM 703373, USNM 162081, USNM 681695, USNM 846264, USNM 198491, USNM 75120, USNM 126852.
In each case, nymph length was measured with callipers and was compared to shell height (less affected by rostration than length); given the strong similarity in shell morphology amongst extant species of Neotrigonia, they were pooled together for the purpose of this analysis. Both variables were log-transformed and a standardised major axis regression (SMA) was fitted to the dataset; the slope of that regression is the allometric coefficient. An allometric coefficient of 1 implies isometry, whilst greater or lower values imply positive allometry (i.e., relative ligament increase) or negative allometry (i.e., relative ligament decrease), respectively. For a discussion of line-fitting in the context of allometry, see Warton et al. (Reference Warton, Wright, Falster and Westoby2006). Allometry analyses were performed using PAST 2.17 (Hammer et al. Reference Hammer, Harper and Ryan2001).
Materials of the extant species Nuculana inaequisculpta (Lamy, Reference Lamy1906) (MLP-Ma 12389), Nuculana sulculata (Gould, Reference Gould1852) (MLP-Ma 12386, MLP-Ma 12391, MLP-Ma 12399), Nuculana sp. (MLP-Ma 9120), Cuspidaria tenella Smith, Reference Smith1907 (MLP-Ma 7382) and Cuspidaria sp. (MLP-Ma 7381) were analysed, paying special attention to the dorsal margin, the ligament and shell alignment during opening of the valves. Comparisons with examples from the literature were also made.
Institutional repositories. MCF-PIPH, Museo Paleontológico Carmen Funes, Plaza Huincul, Argentina; MLP-Ma, Malacology, Invertebrate Zoology Collection, La Plata Natural Sciences Museum, Argentina; MLP-PI, Invertebrate Palaeontology Collection, La Plata Natural Sciences Museum, Argentina; MOZ-PI, Museo Provincial de Ciencias Naturales “Dr. Prof. Juan A. Olsacher”, Zapala, Argentina; USNM, United States National Museum of Natural History (NMNH), Department of Invertebrate Zoology, Smithsonian Institution, Washington DC, USA; USNM-PAL, Department of Paleobiology, Smithsonian Institution, Washington DC, USA.
2. Results
2.1. Shell/ligament system in Nuculana
Both layers in the ligament of Nuculana differ in position and relative development. The fibrous layer is strongly developed ventrally, placed in a resilifer (Fig. 2A–B, res.) and acting as an internal ligament, while the lamellar layer is still external but strongly reduced (Owen Reference Owen1959) and, hence, does not disturb shell opening. Also, part of the lamellar layer develops anteriorly to the umbo, following the displacement of the pivotal axis from the dorsal margin; in fact, protobranchs (with the exception of the Solemyoida) are the only bivalves in which the ligament grows anterior-ward (Waller Reference Waller and Morton1990). All resistance results from compression only, ventral to the pivotal axis, so this is a class 2 lever.
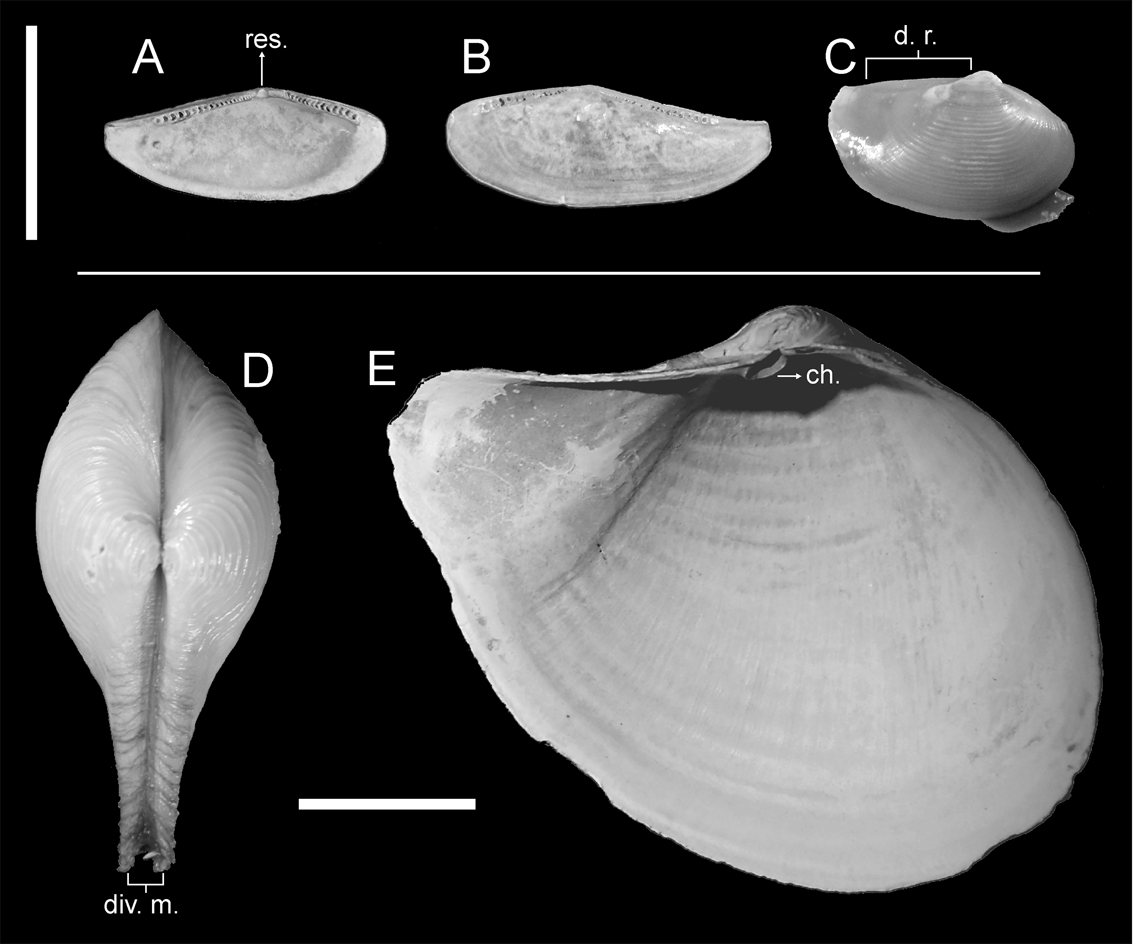
Figure 2 Part of the analysed material for extant species of nuculanids and cuspidariids. (A–B) MLP-Ma 9120, internal view of Nuculana sp.: (A) left valve; (B) right valve. (C) MLP-Ma 12389, right lateral view of N. inaequisculpta. (D) MLP-Ma 7381, dorsal view of Cuspidaria sp. (E) MLP-Ma 7382, internal view of left valve of C. tenella. Abbreviations: ch. = chondrophore; d. r. = dorsal ridge; div. m. = divergent margins; res. = resilifer. Scale bars = 1cm.
Although the genus is usually characterised as having a rostrate posterior end, it shows a high interspecific variability of this character; whilst some species bear a short pointed rostrum (associated with an almost straight dorsal margin), others have a long and recurved one (Coan et al. Reference Coan, Valentich-Scott and Bernard2000; Coan & Valentich-Scott Reference Coan and Valentich-Scott2012). In the latter case, the dorsal margin is concave, as in N. pernula (Müller, Reference Müller1779), N. navisa (Dall, Reference Dall1916) or N. hamata (Carpenter, Reference Carpenter1864); sometimes the rostrum can be extremely long, as in N. extenuata (Dall, Reference Dall1897) (Coan et al. Reference Coan, Valentich-Scott and Bernard2000). Some species may also bear heavy, widely-spaced commarginal ribs (Coan et al. Reference Coan, Valentich-Scott and Bernard2000), recalling somewhat the strong ornamentation of trigoniides; and N. cordyla (Dall, Reference Dall1908) even has transverse ribs on the rostrum (Coan & Valentich-Scott Reference Coan and Valentich-Scott2012). Nevertheless, unlike trigoniides, most species of Nuculana are usually small, rarely exceeding 2 cm in length. Nuculana inaequisculpta also has a dorsal ridge parallel to the commissural plane, thus resulting in a straight dorsal margin levelled with, or even surpassing, the rostrum and acting as pivotal axis (Fig. 2C, d.r.).
Other genera amongst the Nuculanoidea may also develop a rostrum, though rarely associated with a concave dorsal margin; but Yoldia cooperii Gabb, Reference Gabb1865 and Scaeoleda illepida Iredale, Reference Iredale1929 are exceptions (Abbott & Dance Reference Abbot and Dance1982; Coan et al. Reference Coan, Valentich-Scott and Bernard2000). Many species of Saccella Woodring, Reference Woodring1925 may also bear a concave dorsal margin (Coan & Valentich-Scott Reference Coan and Valentich-Scott2012). Another interesting rostrate Nuculanoidea is the fossil genus Ryderia Wilton, Reference Wilton1830, which not only has a very long rostrum (with a concave dorsal margin), but also frequently shows divaricate ornamentation (Hodges Reference Hodges2000).
2.2. Shell/ligament system in Cuspidaria
Before describing the shell/ligament system, it should be noted that most cuspidariids are relatively small bivalves (<5 mm long); sizes at which shells can be relatively flexible, so that valve opening would not pose major problems. Something similar occurs in Nuculana, although in this genus shells are usually more heavily calcified. Since Pterotrigoniinae are generally medium-sized bivalves, large shells of Cuspidaria were preferred for the analysis.
Ligament in Cuspidaria is completely internal, with both lamellar and fibrous layers settled in a chondrophore (Fig. 2E, ch.); a lithodesma placed ventral to the ligament avoids expansion of the ligament during valve closure (Yonge Reference Yonge1978; Yonge & Morton Reference Yonge and Morton1980). Thus, the ligament can act only by compression, both layers acting ventrally to the pivotal axis and in an opposite direction to the effort, resulting effectively in a class 2 lever. But there is another peculiarity in Cuspidaria, which is the development of a thickened periostracum extending across the entire dorsal margin of the shell (Yonge Reference Yonge1978; Yonge & Morton Reference Yonge and Morton1980). This structure results from the fusion of the periostracum of both valves and, as a consequence of its development, the dorsal margin has to act as pivotal axis. To avoid obstruction during shell aperture, dorsal margins diverge and gape from the sagittal plane on the portion of the rostrum which projects dorsally (Fig. 2D, div. m.). This feature also allows for a more efficient aperture of the valves, since the closeness of the pivotal axis to the resistance (i.e., the ligament) renders maximum advantage of its thrust.
Just as in nuculanids, the rostrum within septibranchs is not restricted to the genus Cuspidaria. Acreuciroa Thiele in Thiele & Jaeckel, Reference Thiele and Jaeckel1931, Austroneaera Powell, Reference Powell1937, Luzonia Dall & Smith, in Dall Reference Dall1889, Myonera Dall & Smith, in Dall Reference Dall1886, Plectodon Carpenter, Reference Carpenter1864, Bathyneaera Scarlato & Starobogatov, Reference Scarlato, Starobogatov and Likharev1983 and Cardiomya Adams, Reference Adams1864 also have a produced posterior margin, although only in the last three it is accompanied by a concave dorsal margin (Coan et al. Reference Coan, Valentich-Scott and Bernard2000). Within the Cuspidarioidea, it is noteworthy that the shells of members of the family Spheniopsidae Gardner, Reference Gardner1928, slightly prosogyrate, lack a rostrum or at most, are modestly produced (Coan & Valentich-Scott Reference Coan and Valentich-Scott2012; Machado & Passos Reference Machado and Passos2015; Morton et al. Reference Morton, Machado and Passos2016).
2.3. Shell/ligament system in trigoniides
As an important difference from previously described groups, ligament in trigoniides is always external and parivincular (Newell & Boyd Reference Newell and Boyd1975; see also Fig. 3), hence it cannot act as a class 2 lever, because its lamellar layer will be always subjected to tensile stress during valve closure.
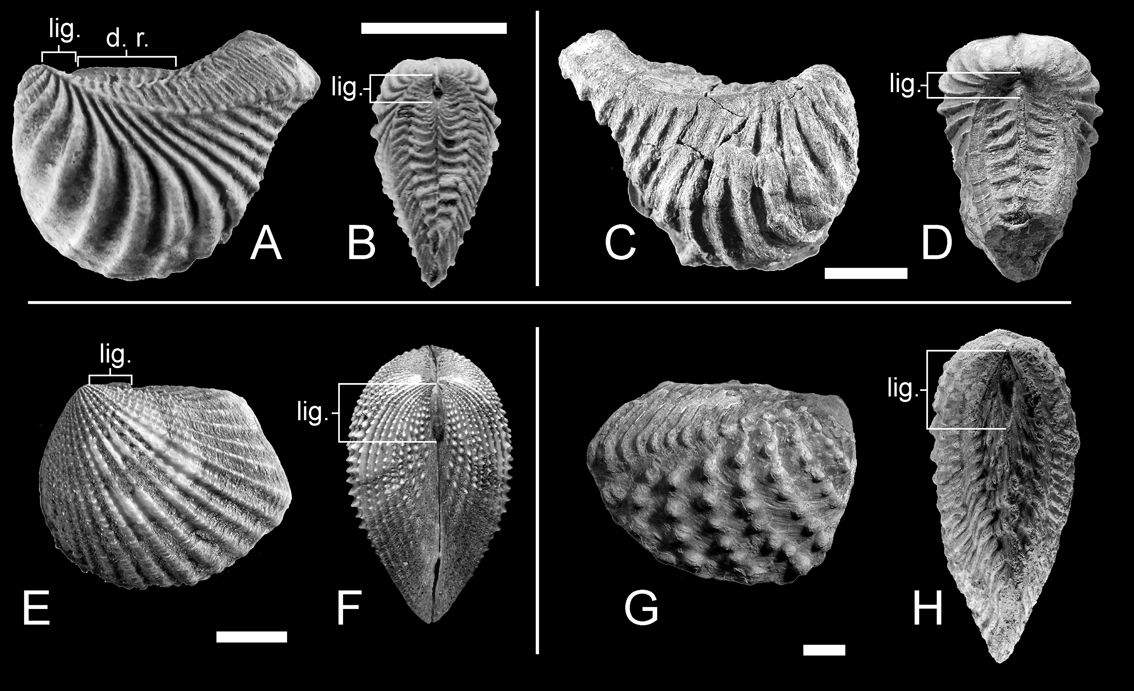
Figure 3 Part of the analysed material of trigoniides. (A–B) Myophorella garatei, from the Valanginian–lower Hauterivian of Argentina: (A) MCF-PIPH 428, left lateral view; (B) MCF-PIPH 427, dorsal view. (C–D) Pterotrigonia coheni, from the Tithonian–Valanginian of Argentina: (C) MCF-PIPH 389, right lateral view; (D) MCF-PIPH 385, dorsal view. (E–F) USNM 465397, Neotrigonia margaritacea (Lamarck, Reference Lamarck1804), from Australia: (E) left lateral view; (F) dorsal view. (G–H) MCF-PIPH 410, Steinmanella caicayensis, from the Valanginian of Argentina: (G) right lateral view; (H) dorsal view. Abbreviations: d. r. = dorsal ridge; lig. = ligament/nymph. Scale bars = 1 cm.
A dorsal ridge, such as the one present in Nuculana inaequisculpta, is commonly present in rostrate trigoniides (Fig. 3A, d.r.). Nevertheless, it is not as well developed as in the protobranch species and, in most cases, the rostrum projects dorsally beyond the ridge, still hindering shell opening. Shell edges, unlike those in Cuspidaria, remain parallel and in contact throughout the whole dorsal margin (Fig. 3B), so the straight margin of the dorsal ridge cannot act as pivotal axis.
When compared to shell height, the ligament seems to be shorter in rostrate opisthogyrate species (Fig. 4A–C) than in orthogyrate ones (Fig. 4D). Ontogenetic development shows a negative allometric relationship for these characters in Pterotrigonia coheni and a positive one for M. garatei, M. coihuicoensis, Steinmanella caicayensis and Neotrigonia spp., and possibly for S. thoracica as well (Table 1).
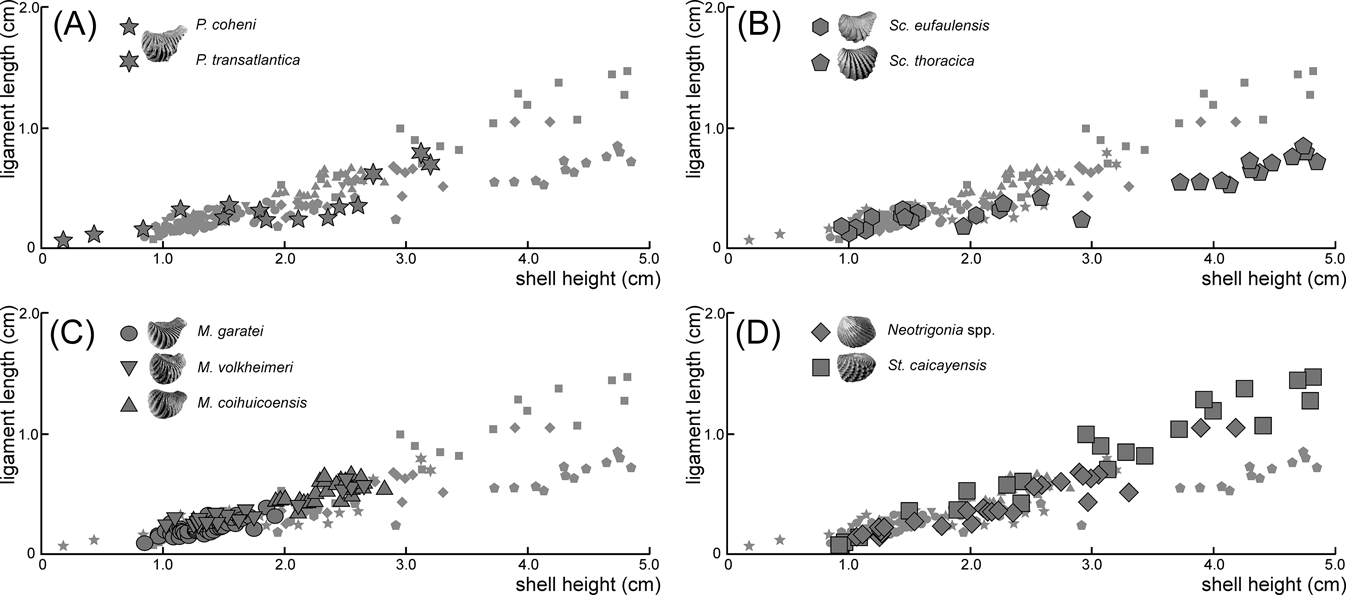
Figure 4 Dispersion plots showing the length of ligament (y-axis) against height of shell (x-axis) for the analysed species of trigoniides: (A) Pterotrigonia species; (B) Scabrotrigonia species; (C) Myophorella species; (D) orthogyrate species. Plots of the other species are shown in all graphs as small grey symbols for easier comparison.
Table 1 Results of the allometry analyses of ligament length (y-variable) vs. shell height (x-variable) for the trigoniide species considered. Asterisks indicate values significantly departing from isometry; values lower than one indicate negative allometric trend (i.e., relative ligament length reduction). Abbreviations: al.coef. = allometric coefficient; p(al.coef.=1) = probability of the allometric coefficient being one; N = number of specimens.
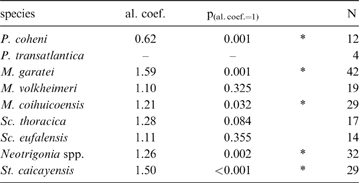
3. Discussion
3.1. Internal ligament as a solution to the shell opening problem
In rostrate opisthogyrate bivalves, the pivotal axis of the shell is shifted dorsally. If the lamellar layer of the ligament were to remain ventral to the pivotal axis, tensile stress would prevent shell opening (Fig. 1D). The most efficient solution to this problem would be the development of an internal ligament. In this way, the resilifer would press on the ligament, which would then act only by compression, the whole system acting effectively as a class 2 lever.
Nevertheless, the presence of an internal ligament in Nuculana and Cuspidaria should not be considered as an adaptation resulting from their particular morphology, but most likely as an exaptation (sensu Gould & Vrba Reference Gould and Vrba1982). Non-rostrate Nuculanidae and Cuspidariidae also bear internal ligaments, suggesting that this condition developed earlier, allowing for the acquisition of a crescentic shell shape.
3.2. Shell opening in rostrate trigoniides
Despite the general variability in ligament length and development (Fig. 4; Table 1), it is clear that more rostrate trigoniides have shorter ligaments than less rostrate ones. This is true whether comparing genera (i.e., Pterotrigonia (Fig. 4A) and Scabrotrigonia (Fig. 4B) vs. Steinmanella and Neotrigonia (Fig. 4D)) or species within a genus (i.e., M. garatei vs. M. volheimeri and M. coihuicoensis (Fig. 4C)). Also, it is noteworthy that species having longer rostra tend to show isometric or even negative allometric relationships to size (with the main exception of M. garatei).
On functional grounds, this could have several meanings. Stanley (Reference Stanley1977, Reference Stanley1978) suggested that in Neotrigonia, the muscular foot aids the ligament during shell opening, due to the large amount of friction in the hinge system. It is possible, then, that in rostrate trigoniides, the foot was mainly responsible for shell opening, whilst ligament reduction would reduce the disturbance of tensile stress. On the other hand, the ligament is not only reduced, but is also very close to the umbo (though always opisthodetic; Fig. 3). Although the antero-posterior axis of the ligament does not coincide with the pivotal axis of the shell, they are closer to each other on the anteriormost part of the dorsal margin, thanks to the development of the dorsal ridge. As a consequence, part of the ligament would develop dorsally to the pivotal axis, especially its anterior part (Fig. 5); this will be of particular importance if we consider the observation by Trueman (Reference Trueman1949) that the anterior part of the ligament is the most effective for shell opening. As the ligament grows longer (posteriorly), most of it would remain ventral to the pivotal axis. So a short ligament would ensure that the functional part of the lamellar layer is raised dorsal to the pivotal axis, still assisting in shell opening.
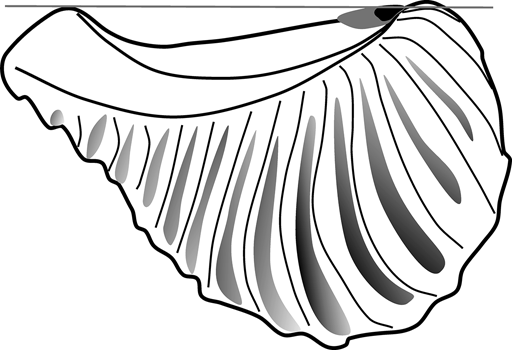
Figure 5 Sketch of Pterotrigonia showing the relationship between the pivotal axis of the shell (grey line) and reconstructed ligaments of different lengths (short ligament in black against long ligament in grey).
3.3. Constructional aspects of the ligament in opisthogyrate shells
The relative shortening of the ligament in rostrate opisthogyrate trigoniides can also be explained on morphogenetic grounds. In orthogyrate bivalves, the direction of growth at any region of the mantle edge can be resolved into a radial component, radiating from the umbo and within the commissural plane (Fig. 6A, R), and a transverse component, normal to the commissural plane (Fig. 6B, N); both of them will be greatest mid-ventrally and least mid-dorsally (Wilbur & Owen Reference Wilbur, Owen, Wilbur and Yonge1964). In prosogyrate and opisthogyrate shells, a third component is added (Fig. 6C, T), acting tangentially to, and in the plane of, the commissure (Wilbur & Owen Reference Wilbur, Owen, Wilbur and Yonge1964). In opisthogyrate shells, tangential and radial components will augment one another anterior to the umbos, with a larger resultant growth; whilst posterior to them, they will oppose (Fig. 6C). As a consequence, an allometric reduction of the dorsal margin (and of the ligament associated with it) would be expected, as opisthogyrate growth becomes stronger.
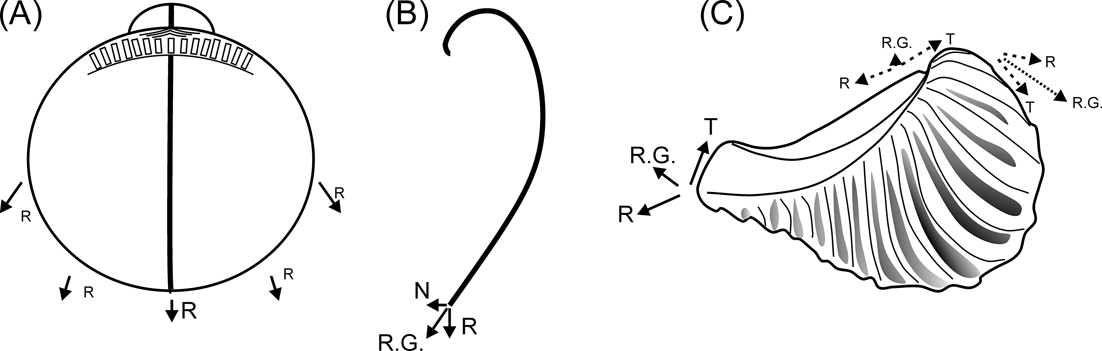
Figure 6 Diagrams showing growth components of bivalve shells. (A–B) growth on orthogyrate shells (modified from Wilbur & Owen Reference Wilbur, Owen, Wilbur and Yonge1964): (A) lateral view; (B) transverse section of one valve. (C) growth on an opisthogyrate shell exemplified on a right valve of Pterotrigonia. Abbreviations: N = transverse component, R = radial component, R.G. = resultant growth, T = tangential component.
Thus, the reduction of the ligament in rostrate, crescentic shells can be interpreted as a constructional consequence of opisthogyrate shell growth and, given its functional value as discussed above, it should also be considered as an exaptation.
4. Conclusions
– In rostrate opisthogyrate bivalves, the umbo and the postero-dorsal angle of the shell project dorsally relative to the ligament, which then fails to act as a pivotal axis. This challenge, present in several bivalve groups, has been functionally solved in different, and sometimes complementary, ways.
– The presence of an internal ligament, acting by compression, results in a class 2 lever, which allows for the development of a rostrate shell morphology with a concave dorsal margin (exaptation in Nuculanidae and Cuspidariidae).The development of a dorsal ridge parallel to the commissural plane (in Nuculanidae), and the development of divergent margins on the posterior part of the dorsal margin (in Cuspidariidae), also allow for shell opening in opisthogyrate rostrate bivalves.
– When the ligament is external, shell growth promotes the allometric reduction of the ligament; this fact, together with the development of a dorsal ridge, allowed the Pterotrigoniinae and other rostrate trigoniides to maintain the functionality of the ligament, although shell opening was most likely aided by a strong muscular foot.
5. Acknowledgments
The authors wish to express their gratitude to the organisers of the Symposium ‘Form, Function and Palaeobiology’, S. F. Vizcaíno, E. N. K. Clarkson and B. E. Schoenemann, for their invitation to participate. We also thank the staff of the visited institutions for their assistance: Departments of Invertebrate Zoology and of Paleobiology, NMNH, Washington DC; Servicio Nacional de Geología y Minería de Chile (SERNAGEOMIN), Santiago, Chile; Museo Olsacher, Zapala, Argentina; Museo Carmen Funes, Plaza Huincul, Argentina; División Zoología de Invertebrados, Museo de La Plata, Buenos Aires, Argentina. Comments from two anonymous reviewers helped to improve the manuscript.