THERMODYNAMICS
Leslie White and Betty Meggers proposed that human societies are thermodynamic systems and are subject to the laws of physics. Ilya Prigogine demonstrated, based on his Nobel Prize–winning theory of self-organization and dissipative structures, that all self-organized systems, including hierarchical human civilizations, require strong, consistent, stable flows of energy through them, enabling the systems to organize themselves. If the strong flow of energy is cut off, a system collapses to a level of hierarchical organization that can be supported by the available energy (Meggers Reference Meggers and Newman1955; Prigogine Reference Prigogine1980; White Reference White1959).
Prigogine showed that the history of all self-organized systems is characterized by alternating periods of stability interspersed with periods of catastrophic chaos. He also demonstrated that self-organized systems are in constant, two-way interaction with their physical environment. Not only do the systems affect their environment, but the environment will also affect the system, completely independently of any internal processes within the system and completely independently of the actions of human beings themselves (see Gill Reference Gill2000:39–71 for a more complete discussion).
CLIMATE AND DEMOGRAPHY
To what extent it is reasonable to conclude that independent climate changes directly affect the health and vitality of a population? Numerous studies have been done to try to answer this question using historical weather records and historical demographic data from preindustrial Europe during the seventeenth and eighteenth centuries. Some of the most dramatic work tying climate to the fate of human beings has been done by demographers. Studies performed in Sweden and in London, in particular—and in England as a whole—determined that a decrease in winter temperature is significantly correlated with an increase in mortality, independent of the harvest. The same studies in England and London found that cooler summers tend to reduce mortality. Furthermore, the studies showed that colder-than-average winters in Sweden reduced human fertility, and in England cooler winters and warmer summers also reduced fertility. These results are further corroborated by studies in Sweden and Croatia showing decreases in mortality associated with abundant harvests. Other studies have been carried out in Sweden, France, and Croatia that demonstrate similar relationships between weather and the increase or decrease in human populations (Galloway Reference Galloway1986:11).
The demographer Patrick Galloway therefore can now speak of “the striking synchrony in the long-term movements of temperature, agricultural yield, and population series across space and time. The results of this analysis suggest that an important driving force behind the long-term fluctuations in population may be long-term variations in climate and their effects on carrying capacity and vital rates” (Galloway Reference Galloway1986:20–21).
Enough evidence has now been developed that Ronald Lee can conclude that, in historical Europe, “Typically, a 1°C [1.8°F] warming would depress prices by about 10 percent through an unknown increase in output and thereby indirectly raise population growth rates by about 0.1 percent per year. So analysis of short-run variations suggests that climate did indeed affect human populations in the past, in roughly equal measure indirectly through agricultural productivity and directly through vital rates.” He also found that a 1°C warming of winter temperature would reduce annual mortality by about 2% and a 1°C cooling of summer temperature would reduce annual mortality by about 4%. In short, then, Lee found that mortality in England was increased by cold temperatures in winter and hot temperatures in summer, and fertility was decreased by the same conditions, a very clear and direct tie between climate and the fate of human populations (Ingram et al. Reference Ingram, Farmer, Wigley, Wigley, Ingram and Farmer1981:28; Lee Reference Lee, Wrigley and Schofield1981:398, Reference Lee1987:456).
Fairly small, independent changes in climate—which are not the fault of human beings or the result of human actions—can have discernible effects on the well-being of human populations. If that is the case, then it is reasonable to conclude that catastrophic climate changes can have catastrophic effects on human populations and can even rise to the level of causing the collapse of an advanced society.
CLIMATE AND HISTORY
Fernand Braudel sought answers in climate with regard to the global economic expansion and the increase in population in the eighteenth century. He asked:
Why did these phenomena occur at the same time throughout the world when space had always been available? The simultaneity is the problem. The international economy, effective but still so fragile, cannot assume sole responsibility for such a general and powerful movement. It too is as much consequence as cause.
One can only imagine one single general answer to this almost complete coincidence: changes in climate. Today they are no longer dismissed by academics as a joke. (Braudel Reference Braudel and Kochan1973:19)
Braudel has hit upon a critical point in the study of the ninth-century collapse in the Maya Lowlands: it was similarly regionally simultaneous, extending across hundreds of kilometers and covering many independent polities. Any solution to the mystery of the Maya Collapse must address the issue of simultaneity.
In 1946, in fact, the Maya archaeologist Tatiana Proskouriakoff recognized the dynamic of simultaneity, though not necessarily of climate, at work in the Maya Collapse. She wrote, “Though it is conceivable that the disappearance of the population may have been a gradual process, the catastrophically sudden extinction of the arts can be explained only in the terms of some widespread and unforeseen disaster that afflicted most Maya cities soon after a.d. 800” (Proskouriakoff Reference Proskouriakoff1946:n.p.).
DROUGHT AND COLLAPSE
If climate can affect human populations, to what extent has it been shown that climatic catastrophe, and in particular drought, has been tied to the collapse of complex civilizations?
The first steps in identifying the relationship between drought and demographic disaster in archaeology have been taken during the last two decades. Peter deMenocal reviewed on a worldwide basis the tie between the collapse of complex civilizations and environmental catastrophes, particularly drought. As a result of his review of numerous studies, he concluded:
For the examples discussed …, available paleoclimate and archaeological data show that societal collapse and prolonged drought were coincident within respective dating uncertainties. Coincidence alone cannot demonstrate causality; indeed each of the cultural collapses had been at one time interpreted solely in terms of human factors unrelated to natural climate variability, such as warfare, overpopulation, deforestation, and resource depletion. However, joint interpretation of the paleoclimatic and archaeological evidence now underscores the important role of persistent, longterm drought in the collapse of Akkadian, Maya, Mochica, and Tiwanaku civilization (deMenocal Reference deMenocal2001:672).
Harvey Weiss maintained, “Drought, wind and dust, not failing leadership or military conquest, brought destruction to the first of the ancient civilizations,” the Akkadian civilization in Mesopotamia (Weiss Reference Weiss1996:30). Weiss and Raymond Bradley analyzed the driving forces of societal collapse and concluded:
The archaeological and historical record is replete with evidence for prehistoric, ancient, and premodern societal collapse. These collapses occurred quite suddenly and frequently involved regional abandonment, replacement of one subsistence base by another (such as agriculture by pastoralism), or conversion to a lower energy sociopolitical organization (such as local state from interregional empire). Each of these collapse episodes has been discussed intensively within the archaeological community, commonly leading to the conclusion that combinations of social, political, and economic factors were their root causes.
That perspective is now changing with the accumulation of high-resolution paleoclimatic data that provide an independent measure of the timing, amplitude, and duration of past climate events. These climatic events were abrupt, involved new conditions that were unfamiliar to the inhabitants of the time, and persisted for decades to centuries. They were therefore, highly disruptive leading to societal collapse—an adaptive response to otherwise insurmountable stresses ….
Climate during the past 11,000 years was long believed to have been uneventful, but paleoclimatic records increasingly demonstrate climatic instability. Multidecadal to multicentury-length droughts started abruptly, were unprecedented in the experience of the existing societies, and were highly disruptive to their agricultural foundations because social and technological innovations were not available to counter the rapidity, amplitude, and duration of changing climatic conditions.
These past climatic changes were unrelated to human activities. (Weiss and Bradley Reference Weiss and Bradley2001:609–610)
David E. Stuart, associate provost and professor of anthropology at the University of New Mexico, has convincingly argued that drought was the major factor in the collapse of Chacoan Anasazi society. “I believe it was the drought of the 1090s that ripped apart the very fabric of the Chaco phenomenon,” he wrote. That was followed by a second drought—the coup de grace—beginning in 1130. Many farmers abandoned their Chaco farms during the droughts and moved into the uplands to elevations of 2000–2225 m. But the upland rains became erratic after 1250 and failed even more dramatically during the 1260s and 1270s. “After about 1275, the erratic and declining rainfall turned into a series of deep, localized droughts. These severe droughts finally drove people from the mesa tops” (Stuart Reference Stuart2000:120–121, 137–140).
Although in Maya archaeology the independent role of climate in past environmental disaster has not been widely accepted, in recent years other time-dependent disciplines such as evolutionary biology and geology have incorporated catastrophism as part of the alternating processes of stability and chaos in the evolution and development of self-organized systems. Major changes can occur in the environment that are completely independent of the actions of human beings, and those changes can devastate human societies.
The following questions are important for this study:
(1) What is the evidence for catastrophic, devastating drought in the Maya Lowlands?
(2) Is there any evidence suggesting a clear link between drought and societal collapse among the Maya?
PHYSICAL EVIDENCE
Meteorology
The Maya Lowlands participate in the Atlantic weather system. Very little rainfall arrives from the Pacific. Only the occasional norte in winter brings a storm that may have originated in the Pacific. Most of the precipitation in the lowlands rides the trade winds in off the Atlantic; in at least one climatic regime, it can fail.
It is important, at this point, to emphasize that there are no doubt multiple possible causes of drought in Mesoamerica. What follows is an explanation of one very important scenario. The Yucatan Peninsula lies at the northern limit of the tropical rainfall zone. Precipitation levels range from as high as 4,000 mm at Ilusión in the Peten to as little as 440 mm at the port of Progreso on the northern coast and to below 330 mm on Cayo Arenas, an offshore island 150 km to the north. There is a twelve-fold difference in precipitation across the peninsula. A southward extension or displacement of the northern zones of semiaridity and aridity would devastate those areas accustomed to regular, heavy rainfall.
Carole Crumley has done extensive paleoclimatic research in Western Europe. She determined that the ecotone dividing the Mediterranean climatic regime from the continental regime lay along the North Sea coast of Western Europe at the beginning of the first millennium and that, by the ninth century a.d., it had migrated south to the northern coast of Africa, a distance of some 880 km (Figure 1) (Crumley Reference Crumley and Crumley1994:192–195).

Figure 1. The ecotone dividing the continental and Mediterranean climatic regimes in western Europe has ranged from 36°N latitude along the North African coast to 48°N in northern Burgundy and Germany, a distance of some 880 km (adapted from Crumley Reference Crumley and Crumley1994:195).
It is possible, therefore, for climatic zones to migrate over relatively large distances as atmospheric circulation patterns change. Similar migrations have been noted in recent decades in the Sahara's southern boundary, which moved southward, bringing drought to the Sahel, and then retreated to the north, allowing the rains to resume. A southward migration of the northern Yucatecan zone of aridity in the lowlands would have been devastating for the 95% of Maya cities that relied on dependable annual rainfall for their drinking water and for their crops.
The Earth's atmosphere is made up of three principal convection cells, which are essential for understanding the migration of the ecotonal boundaries between climatic regimes. The first, and for our purposes the most important, is the Hadley Cell, named for the British meteorologist George Hadley, who first proposed the existence of a thermodynamically driven circulation cell in 1735. It covers the region of the Earth's surface between the equator and about 30° latitude. It is the primary thermodynamically driven convection cell in the atmosphere (Figure 2).

Figure 2. A vertical cross-section along a line running north to south illustrates the main global regions of rising and sinking air and how each region influences precipitation. A small shift of the circulation patterns to the south, especially in Mesoamerica, could move the boundary between wet summer and dry winter and all seasons dry to the south, causing severe problems to cultures dependent on wet summers to provide them with water. In modern times, the boundary between wet summers and dry winters and all seasons dry passes through northern Yucatan. The ITCZ is the intertropical convergence zone (adapted from Ahrens Reference Ahrens1988:480).
In the Hadley Cell, air is heated at the equator and rises. In the lower atmosphere, or troposphere, air at higher elevations is colder, so warm, heated air rises until it reaches the boundary between the troposphere and the stratosphere, the tropopause, where the atmosphere stops cooling and begins to heat up as the altitude increases. At the tropopause, around 15 km near the equator and lower toward the poles, the warm air can rise no farther, so it flows poleward in both hemispheres. It descends to the surface at around 30°, between 20° and 35°, and flows back to the equator. Because of the Earth's rotation, the air aloft travels toward the northeast, and the return flow at the surface is toward the southwest. At the surface, the returning air forms the trade winds, the “river of air” on which Christopher Columbus relied to carry him westward to the New World.
Where the air rises near the equator is known as the Inter-Tropical Convergence Zone (ITCZ). As it ascends, it cools, and the water vapor it carries condenses, forming very active thunderstorms. The ITCZ, then, is the area of tropical rainfall.
The air that flows poleward just below the tropopause is very dry, having lost its moisture on the way up. The descending air therefore has very little water vapor left. The area of the Earth under the descending branch of the Hadley Cell circulation is the region of most of the world's important deserts, between 20° and 35° latitude, including the Mojave, Chihuahuan, Sonoran, Sahara, Negev, Great Indian, Kalahari, and Atacama deserts and the deserts of Australia. The position and strength of the Hadley circulation and the annual migration of the ITCZ are very important factors in determining the annual rainfall at locations such as the Maya Lowlands, which lie at the northern limit of tropical rainfall.
The Hadley circulation is best developed over the oceans where there are no local topographical features such as mountain chains to interfere with its flow. Every ocean in the world, therefore, has a high-pressure cell in it at around 30° latitude. This high-pressure cell is the descending branch of the Hadley Cell circulation. To sailors, this area was known as the doldrums or the horse latitudes.
In the North Atlantic Ocean, the high-pressure cell is known as the North Atlantic High, although some meteorologists refer to it as the Bermuda High or the Azores High. Various meteorologists, such as Stefan Hastenrath, Pedro Mosiño, and Enriqueta García have related rainfall in Mexico and the circum-Caribbean region to the position and the equatorward extension of the North Atlantic High (Armillas Reference Armillas1964:77; García Reference García1974:41; Hastenrath Reference Hastenrath1978; Mosiño and García Reference Mosiño and García1966).
Research indicates that the center of the high-pressure cell travels from year to year in a southwest–northeast direction. When the High is displaced towards the northeast, it lies closer to Europe and warm temperatures prevail over the continent. When it moves to the southwest towards the Caribbean and the Maya Lowlands, cold weather moves in over the European continent and drought comes to Mesoamerica (Gill Reference Gill1994:221–232).
The southwest–northeast travel of the North Atlantic High would indicate that its movement is a response to the expansion and contraction of the Hadley Cell circulation. Since the Hadley Cell is thermodynamically driven, it is logical to believe, although it has not been definitively established yet, that its areal extent responds to changes in the energy driving the system. In warm periods, then, when ample energy is available, the cell expands, and the High moves toward Europe. In cold periods, when there is less energy, it contracts, bringing the High closer to Mesoamerica, diminishing rainfall in the region.
Anthony Broccoli of Princeton University has run climate simulations on his general circulation model that demonstrate that during periods of cold in the Northern Hemisphere, the ITCZ stays far to the south and the summer rains do not develop over Central America (British Broadcasting Corporation Reference Page and Harris2001). The failure of the summer rains would have been devastating for the Maya.
Sea-Surface Salinity
Additional confirmation of Broccoli's simulation is found in a record of sea-surface-salinity (SSS) fluctuations obtained from marine sediments in the northeastern Caribbean Sea (Figure 3). The SSS record is derived from a combination of oxygen-isotope ratios of a shell-bearing plankton, the foraminifera Globigerinoides ruber, and of estimated past sea-surface temperatures using an artificial neural network on 26 species of planktonic foraminifera (Nyberg et al. Reference Nyberg, Malmgren, Kuijpers and Winter2002). It shows that SSSs in the Caribbean were markedly lower around a.d. 200, 550–600, 850, 1000, 1400, and 1600 (all ± 50 years), periods that coincide with repeated demographic devastation in the Maya Lowlands—specifically, the Preclassic Abandonment (a.d. 150–200), the Hiatus (a.d. 536–595), the Collapse (a.d. 760–930), the Postclassic Abandonment (a.d. 1450–1454), and the Colonial droughts and famines of the Little Ice Age.

Figure 3. Locations of the marine sediment core sites discussed in the text. SSS = sea-surface salinity (map adapted from Higuera-Gundy et al. Reference Higuera-Gundy, Brenner, Hodell, Curtis, Leyden and Binford1999:160).
The Postclassic Abandonment coincides with the beginning of the Little Ice Age (LIA) around a.d. 1400–1450. The beginning of the LIA is characterized by a rapid increase in north–south atmospheric circulation in the North Atlantic and South Pacific, as seen in annually dated ice cores from Siple Dome, West Antarctica, and the GISP2 core from central Greenland (Kreutz et al. Reference Kreutz, Mayewski, Meeker, Twickler, Whitlow and Pittalwala1997). The episode around 1600 corresponds as well to the LIA, a period of repeated drought and famine in Mesoamerica known from Colonial historical records.
The lowering of SSS appears to be associated with cooler sea-surface temperatures in the northeastern Caribbean (Nyberg et al. Reference Nyberg, Malmgren, Kuijpers and Winter2002), changes in the North Atlantic Ocean circulation (Bianchi and McCave Reference Bianchi and McCave1999), and stronger trade winds as recorded by planktonic foraminifera in the Cariaco Basin, in the southern Caribbean Sea (Black et al. Reference Black, Peterson, Overpeck, Kaplan, Evans and Kashgarian1999). The cooler Caribbean temperatures may have resulted from troughs of cold air penetrating farther south than present into the Caribbean, as well as a reduced northward transport of equatorial warm water to the North Atlantic (Nyberg et al. Reference Nyberg, Malmgren, Kuijpers and Winter2002). A cooler tropical North Atlantic relative to the South Atlantic is associated with increased surface pressure over the North Atlantic, a southward shift of the ITCZ (Hastenrath and Greischar Reference Hastenrath and Greischar1993), and a pronounced enhancement of north–south versus east–west atmospheric circulation, at least during the periods discussed (i.e., Kreutz et al. Reference Kreutz, Mayewski, Meeker, Twickler, Whitlow and Pittalwala1997). The southward shift in the ITCZ would have meant a failure of the summer rains in Central America, resulting in severe drought, as modeled by Broccoli.
There is a link between the tropical Atlantic and Pacific oceans involving anomalous freshwater fluxes (Latif Reference Latif2001; Schmittner et al. Reference Schmittner, Appenzeller and Stocker2000). Most probably, when the lower SSSs appeared, an enhanced freshwater influx from the Pacific to the tropical Atlantic occurred. This enhanced freshwater import and resulting SSS anomalies in the Atlantic may have reduced the convection and strength of the thermohaline circulation. A weaker thermohaline circulation transports less heat poleward, which results in an enhanced north–south atmospheric circulation that places the position of the ITCZ southward and the North Atlantic High to the southwest, closer to the Yucatan Peninsula, where its effects would be more strongly felt.
The lowering of SSSs may thus be associated with a southward shift in the ITCZ, which resulted, specifically, in more rainfall over the Amazon Basin area, causing an increased discharge of freshwater from the Amazon River into the tropical Atlantic Ocean, which in turn was transported by the mean ocean circulation northward into the Caribbean Sea. In addition, temperate and subpolar waters entering the tropical Atlantic Ocean may have played a role in lowering Caribbean SSSs (Nyberg et al. Reference Nyberg, Malmgren, Kuijpers and Winter2002).
There appears to have been a specific atmospheric/oceanic circulation pattern that established itself each time there was serious demographic disaster among the Maya—most important, the very circulation pattern that appeared during the Collapse in the eighth to tenth centuries.
The record of trade-wind variability from the Cariaco Basin, as well as climate models, suggests that small changes in solar output influence Hadley circulation and, thus, trade-wind variability (Black et al. Reference Black, Peterson, Overpeck, Kaplan, Evans and Kashgarian1999; Rind and Overpeck Reference Rind and Overpeck1993). Reduced solar output correlates well with stronger trade winds in the southern Caribbean Sea. Also, the SSS record from the northeastern Caribbean and climate models (Rind and Overpeck Reference Rind and Overpeck1993) indicate that changes in solar output may affect the salinity balance and, hence, the circulation, northward heat transport, and sea-surface temperature fields of the North Atlantic Ocean.
Rainfall in the Maya Lowlands can be strongly affected by the position of the North Atlantic High and the ITCZ. When the High is displaced far to the southwest, conditions are propitious for drought in Mesoamerica, and cold weather moves in over Europe. When it moves to the northeast, warm weather prevails in Europe, and ample rainfall returns to Mesoamerica. The effect appears to be stepwise in nature. When the High has moved off to the northeast, its exact location does not matter. Its effect is felt only when it moves within range of Mesoamerica. When the High is far to the southwest, the ITCZ remains far to the south, the summer rains fail over Central America and Mexico, and devastating drought ensues.
Our model has been confirmed during the twentieth century from meteorological records. A severe drought—severe for the twentieth century but not in terms of the Classic-period droughts—was reported in Mérida between 1902 and 1904 following three major volcanic eruptions in 1902. Arctic temperatures were the coldest of the century at that time, and the North Atlantic High was displaced far to the southwest. The drought that affected Yucatan was bad. The governor dispatched representatives to foreign cities to buy grain and formally petitioned the federal government for relief from import duties because of the seriousness of the situation. As bad as it was, however, it does not show up in the paleoclimatic records and was nowhere near as devastating as the Classic droughts.
Joel Gunn, William Folan, and Hubert Robichaux have produced another, independent line of research that confirms the relationship between cold temperatures and lowland precipitation. They determined that periods of global cold, as indicated by the global energy balance, result in reduced precipitation in the Yucatan Peninsula, as measured by Candelaria River discharge data. The global energy balance is the difference between the total influx of solar radiation to the earth's surface and the loss of this energy via terrestrial radiation, evaporation, and the dissipation of sensible heat into the ground. In other words, they found that cold global temperatures reduce rainfall in the Maya Lowlands, at least in the Candelaria River drainage basin (Answers.com 2007; Gunn et al. Reference Gunn, Folan and Robichaux1995:19–20).
A Stalagmite from Belize
Paleoenvironmental data from a stalagmite taken from Macal Chasm in the Vaca Plateau of western Belize provides further confirmation of the tie between drought and demographic disaster in the Maya Lowlands. During the 3,300-year record contained in the stalagmite, extending from 1225 b.c. to the present, there were four periods of significant drought that coincided with the Preclassic Abandonment, the Hiatus, the Classic Collapse, and the Postclassic Abandonment. The stalagmite indicates that a series of droughts, which collectively make up the most prolonged dry interval of the 3,300-year record, lasted from a.d. 700 to 1135, thus coinciding with the Classic Collapse. During this time, peak droughts occurred around 754–798, 871, and 893–922, with successive droughts increasing in severity. According to the researchers, these droughts are “the most extreme droughts in the entire 3,300-year record” (Webster et al. Reference Webster, Brook, Bruce Railsback, Cheng, Lawrence Edwards, Alexander and Roeder2007).
The Early Postclassic period saw additional serious droughts centered in 1074 and 1139. These droughts were likely the reason that the Classic culture never succeeded in restoring itself. It should be noted that, according to this record, the droughts persisted for four centuries before returning to Early Classic levels of rainfall, and the period was drier than at any time in the preceding 1,200 years.
The Random Effects of Drought
The effects of drought, however, are rarely uniform over large areas. The effects on neighboring regions can be quite varied. During the late 1980s and early 1990s, for example, San Antonio and South Texas suffered drought, while Houston and Southeast Texas endured very heavy rainfall. Over the past century, recurring drought has afflicted the Great Plains, the Southwest, and northern Mexico repeatedly. In the 1930s, the focus of intense drought was northern Texas, Oklahoma, and parts of Kansas; in the 1950s, it was central and East Texas; and in the 1990s, the worst hit areas were Chihuahua, Coahuila, and West Texas, although the total area affected by drought was much more extensive in each case.
During the terrible drought year of 1955–1956 in Texas, areas of moderate drought were distributed through areas of severe drought in a very random fashion (Figure 4). Thus, the apparent pattern that we will see in the Maya Lowlands during the Collapse of droughts affecting certain areas more at one time and less at another, even though the region as a whole was experiencing drought, is quite normal (Hatfield Reference Hatfield1964).

Figure 4. The severity of drought during the years 1955–1956 for the State of Texas as a whole. The areas of severe drought are indicated in gray; areas of moderate drought are in white. Note the random distribution of severe drought over the state, with areas of moderate drought within the areas of severe drought (adapted from Hatfield Reference Hatfield1964).
Drought and the Maya
Each of the four periods of major demographic disaster in the Maya Lowlands between a.d. 1 and 1500 coincides with episodes of cold in the Northern Hemisphere; each has been associated with drought reported from one or more sites in the Maya Lowlands, as can be seen from Table 1; and each is associated with specific climatic regimes, as indicated by deep-sea SSS cores. Note in Table 1 that every major demographic disaster has been associated by researchers with a drought at some place in the Maya Lowlands (Gill Reference Gill2000:313–314).
Table 1. Collapse, abandonment, and evidence of drought

Note: SSS = sea-surface salinity. Every severe population decline among the Maya is accompanied by drought in the lowlands and lowered SSS in the northeastern Caribbean. The four SSS lowerings are the only ones reported for a.d. 1–1500.
The first great florescence of Maya culture burst forth during the Preclassic period, when true Maya urbanism developed. Then catastrophe set in. Between a.d. 150 and 200, major cities from the Gulf Coast in the north to the Pacific Coast in the south were staggered or emptied during a drought at the time of the Preclassic Abandonment. The major city of the time, El Mirador, was abandoned and saw no significant reoccupation until the Late Classic period. To the east, populations in the Three Rivers region plunged by more than 70% (Figure 5). Drought has been identified as a major factor in the collapse of El Mirador and, given the simultaneous demographic disaster that occurred over the Maya Lowlands as a whole, may be implicated throughout much of the lowlands, as Bruce Dahlin has stated (Dahlin Reference Dahlin1983:261; Hansen Reference Hansen1990).

Figure 5. Population history of the Three Rivers region—Río Azul, Río Bravo, and Booth's River along the Belizean–Guatemalan border. Note the demographic disasters at the time of the Preclassic Abandonment, the Hiatus, and the Classic Collapse. On three occasions, the area lost 70% or more of its population. Clearly, demographic disasters were recurring, devastating phenomena, and each coincides with known periods of drought in the Maya Lowlands. The chart is plotted on a logarithmic scale (Adams et al. Reference Adams, Robichaux, Valdez, Houk, Mathews and Adams1999:196).
Populations then recovered, and most of the cities were rebuilt and boomed in the ensuing 300 years. During the Hiatus, lasting from about a.d. 536 to about 590, social turbulence erupted, with rebellion, war, and demographic collapse halting construction in large areas of the Maya Lowlands. Río Azul, in fact, was abandoned and population in the surrounding countryside dropped 70%. Construction was suspended at Tikal and in the Río de la Pasión–Usumacinta corridor. A serious drought at this time has been identified by Jason Curtis, David Hodell, and Mark Brenner (Reference Curtis, Hodell and Brenner1996) from a lake sediment core taken at Punta Laguna in Yucatan (Adams Reference Adams1999:186; Adams et al. Reference Adams, Robichaux, Valdez, Houk, Mathews and Adams1999:196; Willey Reference Willey and Adams1977:72–73).
The rains returned, and during the next 200 years or so the Maya Lowlands boomed in a way never seen before or since. Then, devastation on a scale rarely suffered in world history destroyed Classic Maya civilization between a.d. 760 and 930 as a brutal drought struck the Yucatan Peninsula. The Southern Lowlands were largely wiped out, but small Postclassic populations hung on in the north, the east, and central Peten, around stable sources of drinking water, and slowly began to rebuild Maya culture.
Around 1200, populations had grown large enough to allow some degree of political integration to occur. A northern state was formed, with its capital at Mayapan. Its hegemony lasted until about 1450 when, during a period of severe drought and cold, its vassals revolted and destroyed the city.
THE CHRONOLOGY OF THE MAYA COLLAPSE
Signor-Lipps Effect
If we can correlate the cessation of monument building, as indicated by the last dates at Maya cities, with the death of the city, then we can examine the pattern of collapse. To do that, it is necessary to turn, for a moment, to paleontology, where researchers have long wrestled with the problem of trying to tie the evidence of the fossil record to a chronological sequence of events.
Luis Alvarez, Walter Alvarez, Frank Asaro, and Helen Michel proposed in 1980 that the extinction of the dinosaurs and over half of the rest of life on Earth at the end of the Cretaceous era was due to the impact of a huge asteroid that caused a massive climatic catastrophe, first dropping then raising temperatures around the globe. They proposed that the extinction was catastrophically sudden, not gradual. Interestingly enough for Mayanists, the asteroid's or comet's impact point is the northern coast of Yucatan, centered on the village of Chicxulub, 17 km east of the port of Progreso (Alvarez et al Reference Alvarez, Alvarez, Asaro and Michel1980:1095–1108).
Critics of the theory argued that they saw in the fossil record much evidence of a gradual extinction of numerous taxa, the best known being the dinosaurs, ammonites, and certain bivalves. The criticism lodged against the Alvarezes' theory was: given the gradual extinction apparent in the fossil record, how could an impact, or any catastrophe, cause extinctions prior to the actual event (Gould Reference Gould1992:10–12)?
Philip Signor and Jere Lipps analyzed the evidence for gradual declines to determine whether the data actually supported a gradual decline, in contrast to a catastrophic extinction, or whether they might be consistent with an abrupt event. Their analysis provides the mathematical foundation necessary for understanding the data of the fossil record. Their conclusions are known as the Signor-Lipps effect:
Catastrophic hypotheses for mass extinctions are commonly criticized because many taxa gradually disappear from the fossil record prior to the extinction. Presumably, a geologically instantaneous catastrophe would not cause a reduction in diversity or a series of minor extinctions before the actual mass extinction. Two types of sampling effects, however, could cause taxa to appear to decline before their actual biotic extinction. The first of these is reduced sample size provided in the sedimentary record and the second, which we examine in greater detail, is artificial range truncation. The fossil record is discontinuous in time and the recorded ranges of species or of higher taxa can only extend to their last known occurrence in the fossil record. If the distribution of last occurrences is random with respect to actual biotic extinction, then apparent extinctions will begin well before a mass extinction and will gradually increase in frequency until the mass extinction event, thus giving the appearance of a gradual extinction. Other factors, such as regressions, can exacerbate the bias toward gradual disappearance of taxa from the fossil record. Hence, gradual extinction patterns prior to a mass extinction do not necessarily eliminate catastrophic extinction hypotheses….
The apparent decline of taxa prior to a mass extinction may simply reflect sampling effects and not actual diversity trends. Therefore, the apparent record of decline in diversity of various taxa prior to the Cretaceous-Tertiary extinction need not be considered evidence for a gradual extinction mechanism. (Signor and Lipps Reference Signor, Philip, Lipps, Silver and Schultz1982:291)
Signor and Lipps framed their hypothesis in mathematical terms that can be paraphrased as: animals die randomly; their remains are preserved randomly; and paleontologists dig randomly. The result is an apparent gradual die-off, even though the actual extinction could have been totally abrupt.
Three Phases of Collapse
The concept can also be applied to archaeology—in particular, to the last dates of monuments in the Maya Lowlands. Many sites have badly eroded, illegible stelae and inscriptions, some of which may well have been erected as katun-ending markers, maybe even in the final years of the Classic period. Other stelae and inscriptions have been defaced, reused, looted, lost, or not yet discovered—reasons that are random in nature. A site may have failed to erect a marker for reasons unrelated to its demise. The presence of dated monuments and their discovery in legible condition represent the random-sampling effects described by Signor and Lipps. An apparent gradual cessation of monument building, therefore, is not necessarily evidence of a gradual collapse. An abrupt end could also produce a record of apparent gradual decline.
One can approach the possibility of abrupt disasters in the Maya Lowlands from two perspectives. The first is to assume that all the sites collapsed together at one time. This approach requires one to accept that at sites that have a large number of known, legible stelae, erected over hundreds of years, as many as five katun-ending markers are missing, which is probably unlikely.
The second approach is to assume that there was more than one episode of collapse and to try to break down the last dates into groups, so that no more than two katun-ending markers are missing at any particular site. If one uses dates from all known sites, however, there is a further problem. Some small sites erected only one or two stelae during their lifetimes. It is difficult in those cases to claim that the absence of stelae during a particular period is indicative of anything meaningful. If we limit the analysis to those cities with a courtyard count greater than 10 in Richard Adams and Richard Jones's rank-ordering scheme—a way of measuring the size of a city—then most of the remaining large cities erected a number of stelae during their lifetimes, and the absence of any further monuments may well be indicative of the death of the site (Adams and Jones Reference Adams and Jones1981). By limiting the possible missing katun markers to no more than two, a scheme consistent with the Signor-Lipps effect, the last dates can be grouped into four 50-year periods: a.d. 761, 762–810, 811–860, and 861–910 (Table 2). When these cities are located on a map, they are grouped not only temporally but also geographically (Figure 6). Furthermore, attempts to shift the cutoff dates earlier or later result in meaningless groups.

Figure 6. Three phases of collapse: a map of the last monument dates at sites of rank order 10 or greater in Adams and Jones's scheme. The lines have been drawn to include some sites whose last dates are known but that have a rank order lower than 10. In addition to the three phases shown, Naachtun's last date was a.d. 761, indicating an earlier phase of its own. All dates from the cities listed in Table 2 are incorporated in this map, except Naachtun (Adams and Jones Reference Adams and Jones1981; Gill Reference Gill1994:405; Lowe Reference Lowe1985:213–216; Schele and Freidel Reference Schele and Freidel1990:381).
Table 2. Starting and ending hieroglyphic dates in the Maya Lowlands

Note: L = Lowe, M = Marcus, SF = Schele and Friedel. Last dates on Maya monuments for sites of rank order 10 or greater, according to Adams and Jones, grouped by years. There are no more than two missing katun markers at each site within each group. This leads to the conclusion, applying the Signor-Lipps effect, that four successive abrupt events may have occurred about a.d. 760, 810, 860, and 890–910.
Source: Adams and Jones Reference Adams and Jones1981; Gill Reference Gill1994:404; Lowe Reference Lowe1985:213–216; Joyce Marcus, personal communication 2003; Schele and Freidel Reference Schele and Freidel1990:381.
After Naachtun in 761, the second phase of collapse occurred around a.d. 810 and struck primarily the west and southwest. Within this area, there are no dates on buildings or stelae past a.d. 810. The third phase occurred about a.d. 860 and devastated the southeast. There are no dates there past a.d. 860. The fourth is more difficult to estimate. The 861–910 sites are concentrated in the core and in the north. There appears to have been a major cessation of activity in 10.3.0.0.0, a.d. 890. Only three sites survived to produce monuments later: until a.d. 906 (10.3.17.12.1) at Uxmal; a.d. 909 (10.4.0.0.0) at Tonina; and 910 at Calakmul. These dates may be indicative of two episodes of collapse, one in 890 and the other in 909–910. However, it might indicate one last, devastating catastrophe in 909–910 or, of course, shortly thereafter. A third possibility would be a serious, deepening drought that was under way by 890 and peaked after 910.
No dates are recorded anywhere on Maya monuments after 10.4.0.0.0, January 18, 909 (Goodman-Martinez-Thompson correlation), including Chichen Itza, until Mayapan emerges around 1200. (For a more complete discussion of dates at Chichen Itza, see Gill Reference Gill2000:339–342.) By applying the Signor-Lipps effect to last dates, it is apparent from the map that there were three phases of large-scale abrupt collapse—plus Naachtun and western Peten.
CHRONOLOGICAL CORRESPONDENCE TO DROUGHT
GISP2 Ice Core
Throughout the Northern Hemisphere, as shown by paleoclimatic records from California, Colorado, Alaska, Canada, and northern Europe, the climate of the ninth century was as cold as during the Little Ice Age (Gill Reference Gill2000:273–289; Williams and Wigley Reference Williams and Wigley1983). As we have seen from the meteorological model, a period of severe cold in the Northern Hemisphere would indicate climatic conditions conducive to a southwestward displacement of the North Atlantic High and a southward shift of the ITCZ, causing the summer rains to fail and bringing drought to the Maya Lowlands.
The GISP2 ice core from Greenland demonstrates that Holocene climate has been extremely variable. Although variability is significantly subdued in the Holocene portion of the core compared with the glacial-age portion, atmospheric circulation patterns are perhaps the most complex of the entire 110,000-year record (Mayewski et al. Reference Mayewski, Meeker, Twickler, Whitlow, Yang, Lyons and Prentice1997; O'Brien et al. Reference O'Brien, Mayewski, Meeker, Meese, Twickler and Whitlow1995). The complexity flows from marked changes in boundary conditions, such as the extent of sea and land ice and vegetation, and from changes in atmospheric circulation, temperature, and precipitation, which have become increasingly apparent from research conducted over the past few years (National Research Council 1999). The Holocene portion of the core is the best dated section of the entire record (Meese et al. Reference Meese, Gow, Alley, Zielinski, Grootes, Ram, Taylor, Mayewski and Bolzan1997). It was sampled at a resolution of 2.5 years or finer. The major ion time series recovered from this highly resolved, well-dated record can be used to document changes in the source strength of chemical species and changes in the atmospheric circulation features over North America, the North Atlantic, and Eurasia (Mayewski et al. Reference Mayewski, Meeker, Twickler, Whitlow, Yang, Lyons and Prentice1997; Mayewski et al. Reference Mayewski, Meeker, Morrison, Twickler, Whitlow, Ferland, Meese, Legrand and Steffenson1993; Meeker and Mayewski Reference Meeker and Mayewski2002; O'Brien et al. Reference O'Brien, Mayewski, Meeker, Meese, Twickler and Whitlow1995).
To set the stage for an investigation of climatic variability at the time of the Maya Collapse, we can first examine changes in solar insolation over the past 4,000 years (Berger and Loutre Reference Berger and Loutre1991) compared with the period of proposed drought in the Maya Lowlands. Solar insolation is the exposure to the sun's radiation that varies over the Earth in time and by location as a consequence of the Earth's orbit around the sun (measured in watts per m2).
About 1,000 years ago, for the general region of Maya occupation (20°N latitude), there was a prominent minimum in solar insolation—in other words, in the amount of energy received from the sun—based on changes in the Earth's orbit around the sun (measured in watts per m2 per 1,000 years). While not absolute proof that temperatures in this region were relatively colder, it is clear that changes in solar insolation due to the Earth's orbit, one of the primary controls on climate, would have resulted in a decrease of incoming solar radiation during the time of the Collapse.
A second line of evidence is the potential association between solar variability and proposed drought. Solar variability refers to changes in the output of the sun's energy. We can use as a proxy for solar variability the parts-per-thousand (‰) 14C residual series developed from tree rings (Stuiver and Braziunas Reference Stuiver and Braziunas1989). High values of 14C are generally associated with low solar output (Beer et al. Reference Beer, Mende and Stellmacher2000). Periods of high 14C residuals, and thus low solar output, appear to coincide with several of the major drought periods in the Maya Lowlands. The most notable coincidences occur during the early phase of the Preclassic Abandonment (a.d. 150–200), the Classic Collapse (a.d. 760–930), the Postclassic Abandonment (a.d. 1450–1454), and into the Little Ice Age. All periods of solar output since at least a.d. 1 would have been superimposed on a general period of low solar insolation. Any additional decreases in solar radiation due to low solar output would have exacerbated cooling. During the Holocene, almost all of the calcium deposited at the GISP2 site was derived from continental sources. Elevated concentrations of calcium in the core result from increased storminess over terrestrial surfaces. Detailed analyses of modern snow pits in central Greenland indicate that present-day calcium concentrations peak during mid-winter to early spring (Mayewski et al. Reference Mayewski, Spencer, Lyons and Twickler1987). In addition to the general intensification of atmospheric circulation during this season, the timing may be a manifestation of late winter cyclonic storms that travel over continental North America, incorporating crustal dusts. Increased levels of calcium characterize all of the major and minor cold periods of the last glacial–interglacial cycle (Mayewski et al. Reference Mayewski, Meeker, Twickler, Whitlow, Yang, Lyons and Prentice1997; Mayewski et al. Reference Mayewski, Meeker, Morrison, Twickler, Whitlow, Ferland, Meese, Legrand and Steffenson1993; O'Brien et al. Reference O'Brien, Mayewski, Meeker, Meese, Twickler and Whitlow1995).
Comparisons of both raw (2.5 year and finer) and robust spline-smoothing versions of calcium concentrations reveal timing that coincides with drought events in the Maya Lowlands. Most notable is the increase in calcium that coincides with the Postclassic Abandonment and the onset and duration of the LIA (Mayewski et al. Reference Mayewski, Meeker, Morrison, Twickler, Whitlow, Ferland, Meese, Legrand and Steffenson1993; O'Brien et al. Reference O'Brien, Mayewski, Meeker, Meese, Twickler and Whitlow1995). The next-highest levels of calcium coincide with the Classic Collapse. Levels of calcium are lower during the Classic Collapse than during the Postclassic Abandonment because the latter coincides with the LIA, a period of intensified storminess throughout much of the world, and the former with a stormy portion of the more benign Medieval Warm Period. There is also generally good coincidence between the other drought events discussed in this paper and increased levels of calcium indicating periods of cold in the North Atlantic.
Finally, we explore the association between terrestrial biomass health and periods of drought. Ammonium from the GISP2 ice core offers a proxy for terrestrial biomass health. Periods of low temperatures and moisture, cold and dry, are associated with low ammonium values and decreased terrestrial biomass (Meeker et al. Reference Meeker, Mayewski, Twickler, Whitlow and Meese1997). We can also use the methane gas series developed from the GRIP ice core, although the rise since about 2,500 years ago or longer may be anthropogenically altered in response to the cultivation of rice. In general, increased levels of methane relate to greater availability of standing water and higher temperatures (Blunier et al. Reference Blunier, Chappellaz, Schwander, Stauffer and Raynaud1995). Ammonium levels have generally declined over the past few thousand years, signaling decreased terrestrial-source biomass and thus a poorer environment for biomass growth (colder, drier) coincident with the decrease in insolation. Decreased levels of methane, superimposed on the anthropogenically forced rise of methane, occur during the Classic Collapse and the Postclassic Abandonment periods.
In summary, the beginning of the ninth century was marked by a 14C high close to a.d. 800, indicative of decreased solar activity. In addition, summer insolation due to Milankovitch orbital cycles, which placed the Earth farther from the sun during the northern summer, was near the low in its cycle at this time. Potential proof of the decrease in solar variability and the low in the insolation cycle comes from a significant drop in methane readings in the ice cores, indicating decreased standing water over biologically productive areas, and a drop in ammonium ions, suggesting decreased terrestrial biological productivity. In other words, it was cold and dry.
From the evidence, then, we can conclude that periods of intensified atmospheric circulation, more similar in character to modern winter atmospheric circulation patterns and the modern dry season in Central America, coincide with the major periods of drought examined in this study. Further, these periods of proposed drought appear to coincide with proxies for colder and drier climate in the North Atlantic.
CORROBORATING HISTORICAL EVIDENCE
Historical Records of Severe European Cold
Trevor Palmer and James Palmer have reviewed a number of annals maintained during the ninth century, such as the Annals of St. Bertin, the Royal Frankish Annals, the Annals of Xanten, the Annals of Fulda, and others. They found that following a period of relative climatic well-being in the first half of the eighth century, the following years' accounts were filled with reports of severe cold, beginning in about a.d. 760.
The first mention of cold weather from around this time was in the Annals of Ulster, the entry for 760 saying, “A great snowfall on the fourth of the nones of February.” In January 761, we have, “A great snowfall and a dark moon.” The Royal Frankish Annals in 763 says, “The winter was hard.” In the Annals of Ulster, however, 764 seems to have been the worst year (although this could have been the same winter as the cold one mentioned in the Royal Frankish Annals, as the dates from different regions could appear slightly different because of a different placing of the New Year). The entry for 764 in the Annals of Ulster says, “A great snowfall which lasted almost three months. An abnormally great drought. A great scarcity and famine.” Then, in 765, “A horrible and wonderful sign was seen in the stars at night. A shortage of bread” (Trevor Palmer, personal communication 2004). According to Trevor Palmer, “[T]he ninth century was a time of great hardship throughout Northern Europe.” He points out that, in contrast, the weather reports found in the annals from the eighth century are relatively benign. (The annals are quoted in Palmer and Palmer Reference Palmer and Palmer2002; Palmer Reference Palmer2003:353–357;). The Royal Frankish Annals report that the winter of 810–811 and 813 were very severe. “There were great losses among the cattle and other beasts that year, and the winter was very hard.” A further exceptionally harsh winter was reported in 820.
The Annals of Fulda made their appearance in 838 and reported that the winter of 838–839 was also very hard, while the summer of 841 was cold, delaying the harvest. By 843, famine was reported from Gaul, with people eating earth mixed with flour. Once again, the winter of 844–845 was severe, and famine consumed the western region of Gaul.
Three of the annals reported that the winter of 859–860 was especially severe and that it lasted longer than usual. In the Adriatic region, the winter was so cold that merchants were able to cross the frozen sea and visit Venice by horse and cart. By 868, famine had spread throughout Germany and Gaul.
In 873, snowfall covered the ground for the entire long, hard winter. The Rhine and Mainz rivers froze over, and, according to Palmer, “[H]unger and pestilence raged throughout Germany and Gaul, killing nearly a third of the population.” During the closing years of the ninth century, the Annals of Fulda reported more episodes of harsh winters, floods, plagues, and famine. The final entry was in 901 (Palmer Reference Palmer2003). In the Annals of Ulster, the entry for 911 states, “Two suns ran together the same day, i.e. the day before the nones of May.” The year 912 is even more interesting: “Snow and extreme cold and unnatural ice this year, so that the chief lakes and rivers of Ireland were passable [i.e. frozen] and causing death to cattle, birds and salmon. Horrible portents also; the heavens seemed to glow with comets; and a mass of fire appeared with them in the west beyond Ireland and it went eastwards over the sea.” The following year, 913, was “[a] dark and rainy year” (Trevor Palmer, personal communication 2004). Evidence of severe cold is not restricted to Europe, as the Nile froze over in 829 (Gunn Reference Gunn1998).
The annals also make repeated mention of celestial events that lead Palmer and Palmer to wonder whether the severe cold might have been tied to a period of cometary bombardment. Records from northern Europe throughout the ninth century a.d., especially during its central decades, describe political and environmental turmoil accompanied by frequent sightings of comets and other celestial phenomena. Although the evidence is insufficient for definite conclusions to be drawn, it could, with good reason, be taken to indicate that an encounter between the Earth and debris from a disintegrating giant comet occurred at this time. If ninth-century archaeological, geological and climatic evidence from around the world is also taken into account, it is hard to deny that some major catastrophic mechanism must have been at work, whatever its precise nature (Palmer and Palmer Reference Palmer and Palmer2002:4).
It is clear that the climatic anomalies of the ninth century were not limited to the Maya Lowlands but were part of a much larger hemispheric, if not worldwide, pattern of climatic change.
Three Phases of Cold
In Sweden, Wibjörn Karlén identified multiyear periods of severe cold from tree rings around a.d. 800, 860, and 910, although the entire century was cold. Karlén also identified multiyear periods of cool summers, conducive to glacier advances in Arctic Scandinavia, also around 800, 860, and 910, although once again, the glacial evidence indicates the century as a whole was generally cold (Karlén Reference Karlén, Mörner and Karlén1984:268).
The three surges of cold in Arctic Sweden occur about the time of the three most severe phases of collapse in the Maya Lowlands. Thus, this same three-pulse pattern can be seen from tree rings, glacier advances, and last dates on buildings and monuments in the lowlands.
Lake Sediment Cores
Lake sediment cores provide evidence of climate change in the Maya area during the last several millennia. As evidence for drought associated with two of the three phases, Hodell, Brenner, and Curtis have previously reported lake sediment studies indicating a severe drought, the most severe of the past 3,500 years, at Punta Laguna, with its peak in 863, and a severe drought, the most severe in the past 7,000 years, at Lake Chichancanab, with its peak around 922. Other studies from Laguna de Cocos on Albion Island and the Belizean wetlands in Belize, Cenote San José Chulchaca in northwestern Yucatan, and probably Lake Salpeten in Guatemala have also indicated increased dryness in the Terminal Classic period (Table 1) (Bradbury et al. Reference Bradbury, Forester, Anthony Bryant, Covich and Mary1990; Curtis et al. Reference Curtis, Hodell and Brenner1996; Hodell et al. Reference Hodell, Brenner and Curtis1995; Leyden et al. Reference Leyden, Brenner, Whitmore, Curtis, Piperno, Dahlin and Fedick1996; Pope and Jacob Reference Pope and Jacob2002; Rosenmeier, Hodell, Brenner, and Curtis Reference Rosenmeier, Hodell, Brenner and Curtis2002).
In 1993 and 2000, sediment cores were retrieved from the bottom of Lake Chichancanab on the north-central Yucatan Peninsula (Figure 7). Lake Chichancanab's water is saturated with gypsum (CaSO) today, but the mineral precipitates only in shallow water near the lake shore. During periods of drier climate, the volume of water in the lake decreases from evaporation, and the concentration of dissolved ions in the remaining water increases. Gypsum becomes supersaturated, precipitates from the water column, and accumulates on the lake bottom, where it is preserved in sediments. Gypsum can be measured two ways in sediment cores. First, the sulfur content (weight % S) of discrete samples can be analyzed. Alternatively, gypsum concentrations can be estimated by sediment density, which is measured by beaming gamma radiation through the sediments while the cores are still in their plastic liners and measuring the attenuation: the denser the sediments, the greater the attenuation. In the Chichancanab cores, stratigraphic levels that are rich in gypsum display high density because gypsum is much denser than the shell-bearing organic matter that dominates most of the sediment core.

Figure 7. Locations of paleoclimatic studies showing evidence of drought (underlying map adapted from Lowe Reference Lowe1985:2).
The 7,000-year-long record of sulfur content from the 1993 core shows low values from about 5000 b.c. to 500 b.c., indicating relatively moist conditions with a low evaporation-to-precipitation ratio during the early Holocene (Figure 8). From about 500 b.c. to a.d. 1000, sulfur content was distinctly higher, suggesting a greater frequency of drought during this interval. The density profile for the 2000 core illustrates the same pattern as the weight % S record (measured every 1 cm on discrete samples) but is considerably more detailed because density measurements were made every .5 cm for the period between about 500 b.c. and a.d. 1200 (Hodell et al. Reference Hodell, Brenner, Curtis and Guilderson2001; Figure 9). In the Middle Preclassic period, a density maximum, or dry period, is centered at about 480 b.c. The onset of the Late Preclassic is marked by three peaks between 370 and 265 b.c. (±50–100). In the Late Preclassic, about the time of the Preclassic Abandonment, two peaks occur between a.d. 120 and 190 (±50–100) that coincide with a relatively larger increase in weight % S (Figure 8). The greatest density occurs in the Terminal Classic and is marked by three distinct subpeaks between a.d. 750 and 870 (±50–100). In the Early Postclassic, two density peaks are apparent between a.d. 1000 and 1060 (±50–100) (Figure 9).

Figure 8. Weight percent sulfur (% S) records from the 1993 (left) and 2000 (right) Chichancanab cores, reflecting gypsum (CaSO) content of the sediments. Shaded intervals are interpreted to represent periods of increased evaporation and precipitation, which indicates drought. Note the general agreement of the two records (David Hodell, personal communication Reference Rosenmeier, Hodell, Brenner and Curtis2002).

Figure 9. Sediment bulk density of the 2000 Chichancanab core measured by gamma radiation attenuation. Density peaks correlate with the high sulfur content seen in Figure 8 but reflect higher sampling intensity, as measurements were made every .5 cm along the length of the core. Intervals of high density (shaded areas) are composed of multiple sub-peaks that are spaced 40–50 years apart. Note in particular the three phases of acutely severe drought in the Terminal Classic, which correspond to three phases of collapse seen in the last hieroglyphic dates from large cities (Table 2; Figure 1). Note especially the intensity and duration of the droughts of the Classic Collapse compared with the droughts of the preceding 1,200 years during the time Maya civilization developed to its peak. The calendar-year dates are based on calibrated radiocarbon dates that have errors of ± 50–100 years. The estimated sedimentation rates are assumed to be constant throughout the length of the core. Sedimentation rates may in fact vary. Both factors may mean that the actual dates of specific events were either slightly earlier or slightly later than the dates indicated in the figure. Based on archaeological evidence, the Terminal Classic droughts probably occurred some 50 years later than shown, still within the dating error. Similarly, other droughts may have occurred 50–100 years later than shown, corresponding more closely to the archaeological record (Hodell, personal communication Reference Rosenmeier, Hodell, Brenner and Curtis2002).
The maximum sulfur peak in the 1993 Chichancanab core is dated by accelerator mass spectrometry (AMS) 14C analysis of a single seed extracted from the gypsum (Hodell et al. Reference Hodell, Brenner and Curtis1995). The age of this seed is 1,140 ± 35 radiocarbon years before present (14C years b.p.), which yields a calibrated age range of a.d. 780 to 990 at 95.4 percent probability using OxCal 3.5 (Bronk Ramsey Reference Bronk1998). This age range coincides well with the Terminal Classic period and the collapse of Classic Maya civilization. To evaluate whether evaporation–precipitation changes inferred from the Chichancanab sediment cores were geographically restricted to north-central Yucatan or more regional in extent, Hodell and his colleagues compared the Chichancanab density profile with the oxygen-isotope (δ18O)Footnote 1 record from Punta Laguna, located to the northeast in Quintana Roo Province (Figure 10) (Curtis et al. Reference Curtis, Hodell and Brenner1996).

Figure 10. Comparison of the 2000 Chichancanab density record (left) with the δ18O record from Lake Punta Laguna (right). Note the similarly of the two peaks in both the density and δ18O signals during the Terminal Classic and Early Postclassic periods (Hodell, personal communication Reference Rosenmeier, Hodell, Brenner and Curtis2002).
The ratio of 18O to 16O in tropical closed-basin lakes reflects mainly local evaporation–precipitation because lake waters preferentially lose 16O, the lighter isotope, during evaporation. A higher ratio of 18O therefore indicates increased evaporation. The Terminal Classic and Early Postclassic density peaks recorded in Chichancanab sediments correlate with the two intervals of greatest δ18O values in the Punta Laguna record centered at about a.d. 860 and 1000 (±50–100). Similarly, density peaks between a.d. 120 and 190 (±50–100) in the Chichancanab record (Figure 9) correspond with two Late Preclassic δ18O increases in Punta Laguna. In addition, the Punta Laguna δ18O signal (Figure 10) contains two other maxima centered at a.d. 590 and 1390 (±50–100). Several droughts inferred from Lakes Chichancanab and Punta Laguna sediment records correlate with Maya cultural discontinuities. Increased evaporation–precipitation between a.d. 120 and 190 coincides with a severe dry period detected in the archaeological record at El Mirador that may have contributed to the site's abandonment in the Late Preclassic around a.d. 150 (Dahlin Reference Dahlin1983). The increase in Punta Laguna δ18O values centered at a.d. 590 (±50) correlates with the Hiatus (a.d. 535–590). The greatest droughts occurred during the Terminal Classic and Early Postclassic periods between about a.d. 750 and 870 (±50–100) and from about a.d. 1000 to 1060 (±50–100), respectively, and coincided with the Classic Collapse (Hodell et al. Reference Hodell, Brenner and Curtis1995). According to the Chichancanab sulfur record, the Terminal Classic drought was the most severe of the past 7,000 years (Figure 9).
Hodell, Brenner, and Curtis believe that the Terminal Classic Drought lasted from about a.d. 770 to 1100, with an intervening wetter period that lasted for about 50 years from 870 to 920 (±90–130) (Hodell et al. Reference Hodell, Brenner and Curtis2005). The Chichancanab density profile, which reflects the past 2.5 millennia of sedimentation, contains evidence of recurrent drought with statistically significant periods of about 208, 50, and 39 years (Hodell et al. Reference Hodell, Brenner, Curtis and Guilderson2001). Thus, drought frequency was not entirely random during the past 2,500 years. The 208-year cycle is similar to the documented 206-year period in records of cosmogenic nuclide (14C, 10Be) production that is thought to reflect solar variability or a combination of solar forcing and oceanic response (Stuiver and Braziunas Reference Stuiver and Braziunas1993; Raisbeck et al. Reference Raisbeck, Yiou, Jouzel and Petit1990). This suggests that some of the century-scale variability in Yucatan rainfall was related to changes in solar output (Hodell et al. Reference Hodell, Brenner, Curtis and Guilderson2001). A similar 200-year cycle has been found in other paleoclimate records in the circum-Caribbean, suggesting that solar forcing of climate may have been widespread throughout the region (Nyberg et al. Reference Nyberg, Kuijpers, Malmgren and Kunzendorf2001; Peterson et al. Reference Peterson, Overpeck, Kipp and Imbrie1991).
Three Phases of Drought
The droughts that make up the 208-year cycle often display internal, multi-decadal oscillations with a period near 50 years (Figure 9; Hodell et al. Reference Hodell, Brenner, Curtis and Guilderson2001). For example, the Terminal Classic increase in density between a.d. 750 and 870 (±50–100) contains three sub-peaks with a spacing of about 40–50 years, which is consistent with and provides the first physical evidence from the lowlands for the proposed tripartite pattern of the Collapse.
However, a shortcoming is associated with precisely dating lake sediment cores. The dating process relies on finding terrestrial organic material in the core that can be radiocarbon dated, such as a seed or a stem or a leaf fragment that has blown into the water. Aquatic organic matter is subject to a hard-water error and is therefore not usable. The usual process is to find a date high in the core and one low in the core and to interpolate in between, which introduces the second shortcoming. To interpolate between the two dates, one must assume that the sedimentation rate between them has remained constant, such that 10 cm of the core, say, always represents the same span of time. In reality, however, the sedimentation rate speeds up and slows down depending on rainfall, temperature, anthropogenic effects, or other causes. It is impossible to know what the actual rate of sedimentation was in the past or how it changed, so the interpolation is itself an approximate process. For these reasons, the dates indicated in the core have an error of ±50–100 years. The best one can say, based on the Yucatecan lake cores, is that a severe drought occurred during the century of the Classic Collapse and it showed a three-phase pattern of brutal, devastating drought.
Evidence from a paleoclimatic study carried out on marine sediments in the southwestern Caribbean, however, can help to pinpoint the timing of the three phases of drought. Gerald Haug and his colleagues drilled marine sediment cores in the Cariaco Basin off the Venezuelan coast (Figure 3) that contain sediments made up of silt from the runoff of the Orinoco and other Venezuelan rivers. As the basin is anoxic, or lacks oxygen, no life is present, so there has been no bioturbation of the sediments. In addition, there are no strong currents through the area. This has resulted in perfectly preserved layers of river sediments that can be counted annually, like varves in a lake, based on the varying levels of titanium during the wet and dry seasons and even seasonal color differences in the deposited silt (Haug et al. Reference Haug, Günther, Peterson, Sigman, Hughen and Aeschlimann2003; Larry Peterson, personal communication Reference Haug, Günther, Peterson, Sigman, Hughen and Aeschlimann2003).
Haug's team reviewed the data from their cores to see whether they could confirm the three previously proposed periods of drought during the ninth and tenth centuries. By counting annual varves back from a sharp titanium rise in the core at a.d. 930, which coincides with the beginning of the Medieval Warm Period in the Caribbean, they found three periods of severe drought centered on 810, 860, and 910, precisely the dates indicated by the last hieroglyphic dates at large Maya cities (Figure 11). In addition, they found a fourth serious drought, just short of the intensity of the other three, at a.d. 760, which explains the fall of Naachtun and the apparent depopulation of the Petexbatun and western Peten—previously an apparent anomaly. The core also shows a period of severe drought that coincided with the Preclassic Abandonment in a.d. 150–200 (Haug et al. Reference Haug, Günther, Peterson, Sigman, Hughen and Aeschlimann2003:1734).

Figure 11. Titanium content, in three point running means, of a marine sediment core from the Cariaco Basin in the Caribbean off the Venezuelan coast. The sediments are composed of silt deposited in annual layers from runoff from the Orinoco and other Venezuelan rivers. Lower titanium indicates lower precipitation. The time span covers the Classic Collapse. In the top graph, note the four episodes of especially severe drought at about a.d. 760, 810, 860, and 910.
It is interesting to note that the four Classic droughts indicated by the Cariaco cores are spaced about 50 years apart and fall right in line with the 50-year cycle of solar forcing proposed by Hodell, Brenner, and Curtis (Reference Hodell, Brenner, Curtis and Guilderson2001).
By counting annual layers, Haug's team was able to determine the length of the periods of severe drought, at least in the Cariaco Basin. The 760 drought lasted for about three years; the 810 drought lasted for about nine years; the 860 drought lasted for about three years; and the 910 drought lasted for about six years. It is important to remember that these were periods of particularly intense drought embedded within an extended long-term regional drought that lasted from about a.d. 760 to 930 in the Cariaco Basin (Haug et al. Reference Haug, Günther, Peterson, Sigman, Hughen and Aeschlimann2003:1735).
Of course, a paleoclimatic record from the southeastern Caribbean is not the same as a paleoclimatic record from the Yucatan Peninsula, so we cannot tell exactly what the duration of the four phases was in the Maya Lowlands. But it does provide suggestive evidence that the three phases of devastating drought were likely multiyear in duration, and it is not out of the question to believe that they may have lasted up to a decade or more. Multiyear droughts accompanied by severe famine are also known from Colonial historical records in Yucatan (Gill Reference Gill2000).
According to Haug and his colleagues, the four periods of intense drought were the result of the failure of the ITCZ to move north during the northern summer; the summer rains failed in Central America. If the summer rains failed for multiyear periods in different regions of the Maya Lowlands, the effect on the people would have been devastating.
How can we explain the apparent discrepancy between the 2000 Chichancanab core, which shows three phases of severe drought, and the Cariaco Basin core, which shows four? What the Chichancanab core indicates is that once the long-term drought started, there was no let-up until after the 810 episode. The drought may not have abated between 760 and 810 in the Maya Lowlands. In the Cariaco Basin, however, the intensity diminished somewhat after 760 before returning in 810. At this point, however, is not possible to be more definitive.
We have presented paleoclimatic evidence for recurrent drought in the northern Yucatan Peninsula and the eastern Caribbean between about 500 b.c. and a.d. 1000, but did this pattern of moisture variability extend farther south in the lowlands, where Late Classic population densities were greatest and the Collapse was most pronounced? The δ18O oxygen-isotope record from a core taken in Lake Peten Itza, Guatemala, indicates little 18O variability for the past 5,000 years (Curtis et al. Reference Curtis, Hodell, Brenner, Balser, Islebe and Hooghiemstra1998). This may simply reflect the lake's insensitivity to climate change because of its large volume, long residence time, and possible underground sources of inflow. That is to say, even large evaporative losses from the basin may not alter the isotopic signature of the water appreciably.
In cores from nearby Lake Salpeten, oxygen isotopes increase in three steps that coincide with the Preclassic Abandonment (a.d. 150), Hiatus (a.d. 550), and Collapse (Rosenmeier, Hodell, Brenner, and Curtis Reference Rosenmeier, Hodell, Brenner and Curtis2002). It is uncertain whether these steps reflect increases in the evaporation–precipitation ratio or human-induced changes in vegetation cover that altered surface runoff and groundwater inflow to the lake (Rosenmeier, Hodell, Brenner, Curtis, Martin, Anselmetti, Ariztegui, and Guilderson Reference Rosenmeier, Hodell, Brenner, Curtis, Martin, Anselmetti, Ariztegui and Guilderson2002). During periods of reduced human impact on local vegetation, forests re-grew and effectively reduced runoff to the lake through the increased evapotranspiration of the trees and the promotion of soil moisture storage. This reduction of water delivery to the lake could mimic dry climate episodes.
Distinguishing between the effects of human hydrologic disturbance and climate change is less of a problem in northern Yucatan because the watersheds studied were not as densely settled. The removal of the relatively low-stature vegetation in the north would have had less impact on runoff, the northern lakes are located in a relatively flat area. Watersheds in the Guatemalan Peten, however, are characterized by steep slopes that promote rapid runoff when local forests are removed. Even if the droughts recorded in the northern Yucatan Peninsula extended into the central Maya Lowlands, human-mediated changes in catchment hydrology in the Peten could possibly obscure the signal (Rosenmeier, Hodell, Brenner, and Curtis Reference Rosenmeier, Hodell, Brenner and Curtis2002).
Given the widespread evidence of coincident drought throughout the Yucatan Peninsula, however, it is reasonable to conclude that drought is the more likely of the two possible causes of the steps seen at Salpeten, especially when the three steps are coincident with three periods of serious drought over some 600 years. Recently, K. Pope and J. Jacob (Reference Pope and Jacob2002) reevaluated an episode of massive gypsum precipitation in the wetlands of northern Belize and concluded that it was related to the Terminal Classic drought recorded in northern Yucatan between a.d. 800 and 1000. This new interpretation constitutes further evidence that the Terminal Classic drought extended southward into the central Maya Lowlands.
GLOBAL EVIDENCE OF DROUGHT
Mesoamerican Droughts
Elsewhere in Mexico, detailed studies of 21 lake cores from Lake Patzcuaro in the central Mexican state of Michoacan indicate that a period of heavy soil erosion from the surrounding watershed, presumably due to agriculture during the Classic period, came to an end about a.d. 750. Soil-erosion rates then dropped sharply, indicating a sharp drop in agricultural activity that lasted until 1100. Sr/Ca ratios in the core indicate a severe, prolonged drought that occurred between roughly a.d. 750 and 850. Populations, in fact, may have disappeared from the basin during this drought (Metcalfe et al. Reference Metcalfe, Alayne Street-Perrott, O'Hara, Hales, Perrott, Millington and Pye1994:141; O'Hara et al. Reference O'Hara, Alayne Street-Perrott and Burt1993:49; Street-Perrott Reference Street-Perrott1994:42).
In the Zacapu Basin, also in Michoacan, diatoms record a very rapid drop in lake level about a.d. 850. Sarah Metcalfe concluded that the drying record at Zacapu a millennium ago “seems to reflect a drying of the climate across the region as a whole, the most pronounced of the whole of the Holocene” (Metcalfe Reference Metcalfe1995:205–206).
A lake sediment core from La Piscina de Yuriría, Guanajuato, in central Mexico, shows farming activity in the region from approximately a.d. 300 to about 950 from the presence of Zea mays pollen in the sediments. The pollen drops sharply after about 950 (±60), indicating a decrease in agricultural activity. During this period, pine pollen also decreases and juniper increases, indicating dryness. In fact, the lake itself probably dried up sometime during the most intense period of desiccation (Metcalfe et al. Reference Metcalfe, Alayne Street-Perrott, O'Hara, Hales, Perrott, Millington and Pye1994:138).
Still, in the central Mexican highlands, reconstructions of lake levels for three basins indicate that the most severe climatic episode was a period of marked aridity occurring between a.d. 550 and 1100 in Lakes Patzcuaro, Hoya San Nicolás de Parangueo, La Piscina de Yuriría, and Zacapu. La Piscina de Yuriría probably went dry, and Lake Patzcuaro was significantly reduced in both depth and area. Many of the highland lake basins were abandoned. According to S. L. O'Hara and colleagues, new centers that appeared during this period were built in defensive positions, indicating the need for protection against attack. They believe that repeated periods of severe drought in Mexico have been associated with civil unrest and military conflict (O'Hara et al. Reference O'Hara, Metcalfe and Street-Perrott1994:967–968, 976–977).
Central and South American Droughts
Farther south in Central America, fires raged through the tropical rainforests of the La Selva Biological Station in the northern Caribbean lowlands of Costa Rica and in the páramo surrounding Cerro Chirrip—in the Cordillera de Talamanca at the same time. Carbonized plant fragments from those fires have been carbon dated to cal a.d. 880–900 (±70). Soil analyses done in the mountains of Costa Rica, at La Chonta in the Cordillera de Talamanca, suggest increased aridity in the century leading up to the fires (Horn and Sanford Reference Horn and Sanford1992:354; Islebe and Hooghiemstra Reference Islebe and Hooghiemstra1997:600).
In the Andes, an increase in the microparticle concentration in the Quelcayya ice cap was seen between a.d. 535 and 665, coincident with the Hiatus. During the sixth century, the Moche, or Mochica, culture abandoned its largest cities, lost its dominion over other cultures, and relocated to other areas. There was an interval of peak microparticle concentration between a.d. 855 and 985, indicative of drought conditions in the Southern Hemisphere, at least around Quelcayya. More recent work has provided evidence of a drought centered on a.d. 900 in the form of increased dust particles and decreased precipitation (Shimada et al. Reference Shimada, Schaaf, Thompson and Mosley-Thompson1991:261; Thompson et al. Reference Thompson, Mosley-Thompson, Bolzan and Koci1985:973; Lonnie Thompson, personal correspondence Reference Thompson, Davis, Mosley-Thompson, Sowers, Henderson, Zagorodnov, Lin, Mikhalenko, Campen, Bolzan, Cole-Dai and Francou1998).
At the nearby Sajama ice cap in Bolivia, an ice core shows an increase in dust particles around a.d. 900, as well. Thus, ice cores from two Andean glaciers demonstrate that the central Andes region was also in the throes of drought at the time of the Maya Collapse (Thompson et al. Reference Thompson, Davis, Mosley-Thompson, Sowers, Henderson, Zagorodnov, Lin, Mikhalenko, Campen, Bolzan, Cole-Dai and Francou1998:1862).
African Drought
Palaeolimnological studies from Kajemarum Oasis in the Manga Grasslands of northeastern Nigeria have determined that the most severe drought of the past 5,500 years occurred between a.d. 770 and 960. The δ18O and Sr/Ca studies seem to show the same three-phase pattern that we see in the lake cores from the Yucatan Peninsula. The ninth- and tenth-century African drought is also seen in studies of the desiccation of several neighboring lakes, by a large increase in salinity at Bougdouma Oasis, and by high-resolution paleo-ecological data from Ethiopia (Street-Perrott et al. Reference Street-Perrott, Holmes, Waller, Allen, Barber, Fothergill, Harkness, Ivanovich, Kroon and Perrott2000:297–299).
It is clear from the broad extent of reported drought from the highlands of Mexico south to the Andes and east to Nigeria and Ethiopia that the climatic aberrations of the Terminal Classic were not localized events but, rather, were part of a global pattern.
HISTORICAL EVIDENCE OF DROUGHT
Mayapan and the Famine of 1 Rabbit
By about a.d. 1200, population levels in the north had recovered to the point that political integration and centralization could occur. The political hegemony of Mayapan lasted until about 1450–1454, when disaster again befell the Maya. Their major northern cities were abandoned during a severe drought. When the Spaniards arrived in a.d. 1528, they found petty, warring states with little political cohesion.
The Postclassic drought is best known from historical records from the central highlands of Mexico written soon after the Conquest. Although the cold weather began as early as 1447, a devastating famine lasted from 1450 to 1454, which culminated in the famine year of 1 Rabbit, the Mexica name of the year 1454. The following historical reports are among those compiled by Anton Kovar (Hassig Reference Hassig1981; Kovar Reference Kovar, Sanders, Kovar, Charlton and Diehl1970:28–31):
- 1450
[T]he snow that fell over the entire land was so high that it reached in most parts a stadium and a half, which caused the destruction and collapse of many houses as well as the destruction of all trees and plants and the country was so cold that many people died and especially older people; and during the three following years all plantings and products of the earth were lost so that most people died. (Alva Ixtlilxochitl)
- 1451
Third year of hunger. All the way to Chalco came the ferocious beasts and buzzards and birds of prey, the same as to all other villages. Everywhere, in the forest and grasslands died even young men and women. Their flesh was so wrinkled and dry as if they were old persons. Hunger was very great. (Relations of Chalco Amaquemecan [seventh relation])
- 1452
Second year of hunger, need, and suffering for lack of food in Chalco. The buzzards were searching everywhere for corpses to eat in the hills, lowlands, and forests. The young men looked like old people because their flesh was so full of folds and wrinkles. The boys and girls became so because of hunger. (Relations of Chalco Amaquemecan [third relation])
[A]nother frost fell during the Tecuilhuitl [the Feast of the Lords, June 12–July 1]. Then the ears froze. In this year there was also an earthquake and fissures appeared in the ground and chinampas collapsed; and people were selling themselves to others because of hunger. (Anales de Tlatelolco and Códice de Tlatelolco)
- 1454
At the beginning there was a very large eclipse of the sun, and then illness grew and so many people were dying that it appeared as if nobody was going to survive, such was the calamity that afflicted this land and hunger was so great that many sold their children in exchange for maize. (Alva Ixtlilxochitl)
It is said that this year, 1 Rabbit, was an extremely unfortunate one; there were many deaths. The people died of thirst. From Chalco came foxes, ferocious beasts, lizards, etc. and they devoured the people. The famine was so great that the old Mexicans sold themselves; they took refuge in the woods where they lived unhappy and feeble. For four years there was nothing to eat in the country, so that the older Mexicans sold themselves and two divisions, it is said, delivered themselves into servitude …. They put themselves into holes and died in any place they found and the lizards devoured them for there was no one to bury them. (Annals of Chimalpahin)
The drought of 1450–1454 also devastated Yucatan. The year of 1 Rabbit occurred, in the Yucatecan dating system, during Katun 8 Ahau. A written record of drought in Yucatan is from the Book of Chilam Balam of Maní and can be dated by its reference to the fall of Mayapan, which occurred in Katun 8 Ahau (Craine and Reindorp Reference Craine and Reindorp1979):
The city of Mayapán was destroyed on a day of a Katún 8 Ahau. Lahun Chablé will establish, to the south, a Katún 8 Ahau. With his eyes on the heavens, the ruler will implore food and drink. The warrior will thank him who will give him food and drink. The fields, having been impoverished, shall be searched for food and water which will not be found anywhere in the Petén or in the entire land, wherever there are Bacabs. (Craine and Reindorp Reference Craine and Reindorp1979:83)
The destruction of Mayapán, which is in the south, took place in the Katún 8 Ahau. Lahun Chan established the Katún 8 Ahau and directed his powerful gaze toward heaven, imploring hard bread and water. No one entertained the soldier with bread or water. The people, on the plain and in the forest, were chilled. Everything was searched, but nothing [neither food nor water] could be found on the plains, or in the forest, or in the whole world, or in all of Petén. (Craine and Reindorp Reference Craine and Reindorp1979:156)
The physical destruction of Mayapán was the result of an internal revolt of its vassals during a time of cold and drought. Once again, we see the pattern of drought, cold, famine, and conflict. The famine which began in Mesoamerica around 1450 was not a localized event, but was part of a global pattern of weather changes, including the onset of the Little Ice Age in Europe in 1400–1500 and one of the two severest periods of cold in the Sierra Nevada in the past 2,000 years (Lamb Reference Lamb1982).
The Book of Chilam Balam of Maní goes on to recount other droughts, as well. In one, it reports that, “[b]eginning with and during Kan, vultures will enter the houses in your villages because of the great numbers of dead Mayas and dead animals” (Craine and Reindorp Reference Craine and Reindorp1979: 67).
Historical Colonial Drought and Famine
In Yucatan, thirteen famines due to drought were reported in historical records between 1440 and 1840. The historian Juan Francisco Molina Solís describes two of them. The year 1651 left very painful memories in Yucatan. As Molina Solís recounts:
The starvation as it developed was terrible and desolating, because there was neither corn nor wheat, nor any other grain for ordinary sustenance: at first the lack of cereals was supplemented with meat, which also became scarce; hens ran out; there were no pigs; cattle were rare. The poor, wasted and gaunt, desperately left for the countryside in search of sustenance, and pulling up grass and roots, ate them to avoid starvation; the streets, roads, parks, plazas, and other public places were sown with corpses; the inhabitants of the towns looked more like specters than living men, and many abandoned their homes, driven by hunger: entire places were abandoned, because their inhabitants went to the forests of the south in search of food, the best populated sites of Yucatan were threatened with being left deserted and barren. (Quoted in Molina Hübbe Reference Molina Hübbe1941:20–21; translation in Gill Reference Gill1994:380)
In 1725, the rains again failed and caused a famine that lasted until 1727, during the administration of the conqueror of Belize, Marshall don Antonio de Figueroa y Silva:
[T]he bones of any animal found in the streets and fields were converted into powder to be eaten as flour, and everywhere squalid specters wandered in search of necessary food; although in vain, because there was nothing with which to provide the most urgent necessities of life; the starving fell of dissipation and hunger, the number of corpses was so high, that they were collected in wagons to be taken to the cemetery. (Quoted in Molina Hübbe Reference Molina Hübbe1941: 24–25; translation in Gill Reference Gill1994:380)
Over a period of 400 years, drought and famine repeatedly ravaged the Maya. The historical records of the time document 13 famines that were attributed to drought. In one colonial drought/famine, as many as 50% of the population may have died.
Historical records document that drought and its accompanying death have been frequent visitors to the Maya Lowlands and have had devastating effects on the Maya.
SUMMARY AND CONCLUSION
Although it has been treated as such in the past, the Collapse was not a singular event. Periods of demographic disaster were recurring phenomena in the preindustrial Maya Lowlands, as can be seen in both the archaeological and historical records.
• Prior to the arrival of the Spaniards, the Maya suffered four major demographic disasters when large numbers of people died: the Preclassic Abandonment (a.d. 150–200), the Hiatus (535–595), the Collapse (760–930), and the Postclassic Abandonment (1450–1454).
• A marine sediment core from the northeastern Caribbean has shown that sea-surface salinity was lowered at each of the four periods of demographic disaster, indicating a failure of the ITCZ to move north in the summer, leading to a failure of the vitally necessary summer rainfall in the Maya Lowlands.
• The stalagmite record from Macal Chasm in western Belize records significant drought in good agreement with each of the four periods of disaster.
• For each of these periods of disaster, other paleoclimatic studies from the Yucatan Peninsula have indicated evidence of severe drought in the lowlands at the time of the demographic disaster, as can be seen in Table 1.
The-sea surface salinity record suggests the recurring droughts were associated with the same pattern of specific atmospheric circulation regimes when the ITCZ stayed far to the south, the North Atlantic High moved to the southwest, and the summer rains failed in the Maya Lowlands. The Collapse, then, was not a singular event but, rather, part of a repetitive process. It was, however, by far the worst of the four periods of disaster.
The repeating nature of drought can also be seen in historical records during the period 1440–1840, which describe multiple episodes of brutal drought, famine, suffering, pain, and death in the Yucatan Peninsula. The physical evidence also supports the causal role of drought in the Collapse.
• The GISP2 ice core shows that the ninth century was particularly cold and dry in Greenland and the North Atlantic, conditions that are propitious for drought and famine in the Maya Lowlands.
• Tree-ring records from Scandinavia indicate severe cold episodes centered in a.d. 800, 860, and 910.
• Pulses of Scandinavian glacier advances can also be dated to around 800, 860, and 910.
• There are historical accounts of severe winters in Europe in 810 and 860 and throughout the ninth century.
• Sea-surface salinity was lower in the northeastern Caribbean, indicating that the ITCZ had failed to move to the north, and the North Atlantic High had moved to the southwest.
• The 1993 Lake Chichancanab sediment core showed a devastatingly brutal drought at the time of the Collapse, the worst in the past 7,000 years. Drought was also demonstrated in studies at other sites.
• The 2000 Lake Chichancanab core has greater temporal resolution than the 1993 core and indicates that, within the long-term period of serious drought at the time of the Collapse, there were three episodes of particularly brutal drought, spaced about 50 years apart.
• The marine sediment cores from the Cariaco Basin show four periods of severe drought embedded in a long-term drought that lasted from 760 to 930. The four pulses of devastating drought were centered on 760, 810, 860, and 910, although the 760 pulse, while serious, was not quite as severe as the other three.
• The last dates from large Maya cities show four episodes of abandonment during the Collapse: 760, 810, 860, and 910. The Chichancanab cores showed that the drought lasted 150–200 years and was characterized by three peaks that correspond to three of the phases of collapse seen from the last hieroglyphic dates at large cities and three pulses of severe cold seen from tree-ring data in Sweden. The multiple-phase pattern of the intense Classic droughts has been confirmed in a paleoclimatic record from the Cariaco Basin of the Caribbean and, since the core can be resolved annually, the phases have been dated to 810, 860, and 910, which coincide exactly with the archaeological evidence. In addition, the Cariaco Basin evidence establishes the existence of a fourth phase, not quite as intense as the other three, in about 760, coincident with the last date of 761 at Naachtun, which marks the beginning of the Collapse. Note that all four phases are dated about 50 years apart, consistent with a pattern of solar forcing of drought during periods of cold.
The two paleoclimatic records from Caribbean sediments indicate that the ITCZ was displaced far to the south during the ninth and early tenth centuries and did not move north during the summer, as it does today. The North Atlantic High was displaced to the southwest. The result was a failure of the rains in Central America and Mexico, leading to a cessation of agriculture and the failure of the water-supply system—which in most Maya cities depended on significant annual rainfall to replenish surface-water reservoirs.
The second issue to be resolved in discussing the drought theory is whether the Maya could have done anything to deal with the drought to mitigate its effects. Some Maya archaeologists have wanted to view the Classic droughts as a trigger that set off a series of cultural effects that resulted in the collapse of Classic civilization.
There are indications that survivors tried to repopulate some of the larger cities after the end of the Collapse. For example, there is evidence of squatters living in the temples and palaces at Tikal. As one can see from Figure 9, however, the Maya suffered additional droughts in the Postclassic that would have driven out or killed any colonists living away from the stable sources of drinking water. (It is possible that two of the Postclassic droughts occurred around the time of the terrible Toltec droughts in the tenth and eleventh centuries, which destroyed Tula and the Toltec empire. The radiocarbon dates, while consistent with this idea, are not precise enough for definitive dating.)
The evidence shows that the Classic droughts were of an intensity and duration that the Maya had never before experienced (Figure 12). They were devastating, obliterating droughts in the face of which no political system, no agricultural practices, no population density, no religious rituals had any effects or could have had any effects. There was nothing they could do or could have done.
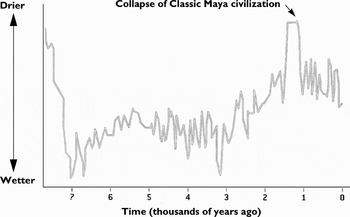
Figure 12. Fossil shells in lake sediments from the Yucatan Peninsula record sudden and severe droughts. By analyzing the ratio of oxygen isotopes in shells deposited in lake sediments, periods of drought can be identified. More 18O is present in the lake water during times of evaporation—and used in making the shells—because oxygen's lighter isotope, 16O, evaporates more readily. The droughts at the time of the Classic Collapse were far greater than anything the Maya had experienced for 7,000 years (adapted from Alley Reference Alley2004:65).
In the end, the food and water ran out—and they died.
RESUMEN
Entre 760 y 930 d.C., millones de mayas desaparecieron de la faz de la Tierra. Examinamos cambios en el medio ambiente físico en que vivieron los mayas. La evidencia de núcleo de hielo tomado de Groenlandia señala que, alrededor de la época del colapso maya, en Groenlandia hubo un mínimo en la insolación solar y una baja en la actividad solar junto con frío intenso y sequedad, es decir, había condiciones climáticas hemisféricas propicias para la sequía en las tierras bajas mayas. En el Caribe nororiental, la salinidad de la superficie del mar disminuyó. La sequía más grave de los últimos 7 000 años devastó la península de Yucatán. Las ciudades grandes de los mayas se colapsaron en cuatro etapas de abandono separadas por periodos de unos cincuenta años, alrededor de 760, 810, 860 y 910 d.C. Un nuevo núcleo tomado del lago Chichancanab en Quintana Roo muestra tres episodios pico de sequía brutal dentro de una sequía de 150 a 200 años. Un núcleo marino tomado de la Fosa de Cariaco frente a las costas de Venezuela fecha con precisión cuatro episodios graves de sequía en 760, 810, 860 y 910, lo que coincide con las cuatro fases de abandono de las ciudades mayas. Al parecer, la sequía de largo plazo de la Fosa de Cariaco duró de 760 a 930. Los cambios climáticos fueron los más drásticos que los mayas habían enfrentado en los 1,500 años anteriores y los más severos de los últimos 7,000 años.
ACKNOWLEDGMENTS
David Hodell and Mark Brenner have been extremely generous with data, discussions, constructive criticism, and suggested wording of the discussion of the Chichancanab and other cores from the Maya Lowlands. That section of this article is immeasurably better for their contributions.
The authors appreciate the efforts of Cynthia Page, producer, and Sophie Harris, associate producer, of Ancient Apocalypse: The Maya Collapse, a television documentary produced by the BBC, for turning up evidence and making connections presented in this paper. We express our gratitude to Ofelia Arruti for her translation of the abstract into Spanish.
This paper brings together the fields of archaeology and paleoclimatology as they pertain to Maya cultural development. P. Mayewski summarized the GISP2 ice core record; J. Nyberg discussed the sea-surface salinity record in the Caribbean and its implications for past atmospheric circulation patterns; G. Haug and L. Peterson presented the Cariaco Basin evidence; and R. B. Gill interpreted the archaeological record, suggested mechanisms for droughts in Yucatan, and discussed the cultural consequences of drought.