Introduction
Toxoplasma gondii is an obligate intracellular apicomplexan protozoan parasite causing toxoplasmosis, a priority zoonotic disease of One Health importance. Countless species of warm-blooded vertebrates including humans are susceptible to Toxoplasma infection (Kistiah et al., Reference Kistiah, Frean, Winiecka-Krusnell and Barragan2012; Galal et al., Reference Galal, Ajzenberg, Hamidović, Durieux, Dardé and Mercier2018; Despommier et al., Reference Despommier, Griffin, Gwadz, Hotez and Knirsh2019; Shah and Khan, Reference Shah, Khan, Yasobant and Saxena2019; Al-Malki, Reference Al-Malki2021; Simpson et al., Reference Simpson, Quesada, Chatterjee, Kakkar, Chersich and Thys2021). There have also been rare occurrences of T. gondii being detected in reptiles and molluscs (Nasiri et al., Reference Nasiri, Teymurzadeh, Karimi and Nasiri2016; Ghozzi et al., Reference Ghozzi, Marangi, Papini, Lahmar, Challouf, Houas, Ben Dhiab, Normanno, Babba and Giangaspero2017; Feitosa et al., Reference Feitosa, Brasil, Parentoni, Vilela, Nety and Pena2018). The lifecycle involves asexual cycles in the intermediate hosts (warm-blooded vertebrates) and both asexual and sexual cycles in the definitive host (felines) (Dubey, Reference Dubey and Baron1996). Infectious stages of the organism include tachyzoites (invasive form), bradyzoites (present in tissue cysts) and oocysts containing sporozoites (shed in feline feces) (Al-Malki, Reference Al-Malki2021). Infection can occur by consuming tissue cysts in raw or undercooked meat, ingesting oocysts present in the environment, contaminated food or water, ingestion of circulating tachyzoites in raw goats' milk, tachyzoite infection via organ or bone marrow transplants or blood transfusions and congenital transmission of tachyzoites crossing the placenta (Dubey, Reference Dubey and Baron1996; Khan et al., Reference Khan, Su, German, Storch, Clifford and Sibley2005; Robert-Gangneux and Dardé, Reference Robert-Gangneux and Dardé2012; Hammond-Aryee et al., Reference Hammond-Aryee, Esser and van Helden2014; Despommier et al., Reference Despommier, Griffin, Gwadz, Hotez and Knirsh2019). According to Galal et al. (Reference Galal, Ajzenberg, Hamidović, Durieux, Dardé and Mercier2018), several factors can influence the prevalence and severity of disease such as the method of infection, the inoculum dose, recurrent infections, age, ethnicity, immune status, the screening system used, the presence of coinfections and the genotypes of the strains involved.
Distribution is worldwide with a seroprevalence rate of 25.7%, which is estimated to be higher in Africa compared to other continents (Molan et al., Reference Molan, Nosaka, Wang and Hunter2019). This zoonotic parasite has classically been grouped into 3 archetypal clonal lineages, types I, II and III, which emerged from a common ancestor ±10 000 years ago (Su et al., Reference Su, Evans, Cole, Kissinger, Ajioka and Sibley2003; Dardé, Reference Dardé2008). In most geographical regions around the world, clonal lineages predominate, with each region having a higher prevalence of a particular clonal or regional genotype with relatively low levels of diversity (Dardé, Reference Dardé2008; Su et al., Reference Su, Khan, Zhou, Majumdar, Ajzenberg, Dardé, Zhu, Ajioka, Rosenthal, Dubey and Sibley2012; Shwab et al., Reference Shwab, Saraf, Zhu, Zhou, McFerrin, Ajzenberg, Schares, Hammond-Aryee, van Helden, Higgins, Gerhold, Rosenthal, Zhao, Dubey and Su2018). In contrast, Central/South America has an abundance of genetic diversity with less common genotypes and genetically distinct isolates in different regions (Dardé, Reference Dardé2008; Su et al., Reference Su, Khan, Zhou, Majumdar, Ajzenberg, Dardé, Zhu, Ajioka, Rosenthal, Dubey and Sibley2012; Shwab et al., Reference Shwab, Saraf, Zhu, Zhou, McFerrin, Ajzenberg, Schares, Hammond-Aryee, van Helden, Higgins, Gerhold, Rosenthal, Zhao, Dubey and Su2018; Galal et al., Reference Galal, Hamidović, Dardé and Mercier2019a).
The expansion of the 3 classic clonal lineages and their abundance across the world has been linked to the simultaneous adaptation of direct oral infectivity traits in combination with the adoption of cats as companion animals and the advent of agricultural animal domestication (Su et al., Reference Su, Evans, Cole, Kissinger, Ajioka and Sibley2003; Sibley et al., Reference Sibley, Khan, Ajioka and Rosenthal2009; Shwab et al., Reference Shwab, Saraf, Zhu, Zhou, McFerrin, Ajzenberg, Schares, Hammond-Aryee, van Helden, Higgins, Gerhold, Rosenthal, Zhao, Dubey and Su2018). The isolates could thus replicate asexually through successive hosts within population groups and bypass sexual recombination creating an expansion on a clonal level.
Identification of T. gondii genotypes is useful in epidemiological studies of toxoplasmosis for the detection of specific strains that can be linked to higher virulence (Khan et al., Reference Khan, Su, German, Storch, Clifford and Sibley2005; Dardé, Reference Dardé2008; Liu et al., Reference Liu, Wang, Huang and Zhu2015). Developed genotyping methods include microsatellite (MS) analysis, multilocus sequence typing (MLST), restriction fragment-length polymorphism PCR (PCR-RFLP), random amplified polymorphic DNA-PCR and high-resolution melting analysis (Liu et al., Reference Liu, Wang, Huang and Zhu2015). Multilocus nested-PCR-RFLP genotyping using 10 molecular markers has aided in the discovery of 189 different genotypes currently listed on the Toxoplasma genome database, http://ToxoDB.org (Liu et al., Reference Liu, Wang, Huang and Zhu2015; Harb and Roos, Reference Harb, Roos and Tonkin2020). Clonal lineages have also been defined by using 15 polymorphic MS markers that are distributed across 11 different chromosomes (Ajzenberg et al., Reference Ajzenberg, Collinet, Mercier, Vignoles and Dardé2010). Classifications of clonal lineages include archetypal lineage (types I, II and III), variants of archetypal lineages, local or regional clonal lineages (Amazonian, Africa 1–4, Caribbean 1–3, Chinese 1 and Guiana) and unique genotypes or atypical genotypes (Galal et al., Reference Galal, Hamidović, Dardé and Mercier2019a). Multilocus sequence typing assays have revealed clusters of 16 haplogroups belonging to 6 ancestral clades with most strains grouped into this clonal structure (Su et al., Reference Su, Khan, Zhou, Majumdar, Ajzenberg, Dardé, Zhu, Ajioka, Rosenthal, Dubey and Sibley2012). Genetic analyses of T. gondii isolates with multiple methods have shown that there are correspondences between whole-genome sequencing, MLST, PCR-RFLP and 15 MS markers (Su et al., Reference Su, Khan, Zhou, Majumdar, Ajzenberg, Dardé, Zhu, Ajioka, Rosenthal, Dubey and Sibley2012; Shwab et al., Reference Shwab, Zhu, Majumdar, Pena, Gennari, Dubey and Su2014; Galal et al., Reference Galal, Hamidović, Dardé and Mercier2019a). These studies, which included a total of 1457 isolates originating from all continents except Antarctica, have made correlations between conventional genotype designations and ToxoDB PCR-RFLP defined genotypes (Shwab et al., Reference Shwab, Zhu, Majumdar, Pena, Gennari, Dubey and Su2014). Designations for some of the lineages derived from the tested isolates which are also of importance in this review include ToxoDB#1 (type II clonal), ToxoDB#3 (type II variant), ToxoDB#2 (type III), ToxoDB#10 (type I), ToxoDB#6 (Africa 1), ToxoDB#203 (Africa 3), ToxoDB#137 and #20 (Africa 4) (Shwab et al., Reference Shwab, Zhu, Majumdar, Pena, Gennari, Dubey and Su2014; Galal et al., Reference Galal, Hamidović, Dardé and Mercier2019a).
Different genotypes of T. gondii have been linked to disease severity with a variance in virulence among the genotypes (Dardé, Reference Dardé2008). Infection with a particular strain is reliant on the geographical origin where the infection was acquired and virulent strains containing type I or atypical alleles have been found to be more pathogenic and more likely to cause severe disease due to the type of immune response elicited inside the host (Xiao and Yolken, Reference Xiao and Yolken2015). Despite evidence that T. gondii genotype might be associated with disease severity, there is a deficiency in investigations focusing on the topic, especially in Africa (Galal et al., Reference Galal, Ajzenberg, Hamidović, Durieux, Dardé and Mercier2018). Knowledge of the population structure of T. gondii can aid in discovering the impact of genotypes on disease manifestations, what geographical determinants influence the genotype and contribute to the development of new diagnosis, treatment and vaccine strategies (Sharif et al., Reference Sharif, Amouei, Sarvi, Mizani, Aarabi, Hosseini and Daryani2017; Lachkhem et al., Reference Lachkhem, Galal, Lahmar, Passebosc, Riahi, Plault, Dardé, Mercier and Babba2021). This study, therefore, provides a summary of the genotypes of T. gondii that have been identified thus far in African countries and an evaluation on the prevalence of genotypes in the different countries and among host species.
Materials and methods
Search strategy
This review followed the preferred reporting items for systematic reviews guidelines (Page et al., Reference Page, McKenzie, Bossuyt, Boutron, Hoffmann, Mulrow, Shamseer, Tetzlaff, Akl, Brennan, Chou, Glanville, Grimshaw, Hróbjartsson, Lalu, Li, Loder, Mayo-Wilson, McDonald, McGuinness, Stewart, Thomas, Tricco, Welch, Whiting and Moher2021a, Reference Page, Moher, Bossuyt, Boutron, Hoffmann, Mulrow, Shamseer, Tetzlaff, Akl, Brennan, Chou, Glanville, Grimshaw, Hróbjartsson, Lalu, Li, Loder, Mayo-Wilson, McDonald, McGuinness, Stewart, Thomas, Tricco, Welch, Whiting and McKenzie2021b). A systematic review of literature published until April 2022 was conducted to explore the genetic diversity of T. gondii in African countries. Searches were conducted on 6 online databases which included Europe PMC, PubMed, ScienceDirect, Scopus, Google Scholar and Dimensions AI. Boolean operators ‘AND’ and ‘OR’ were used for the searches in each database. Where possible, searches were limited to the title and abstract together with keywords or text words together with Medical Subject Headings (MeSH) terms. Searches were also limited to include only English documents and if possible exclude review articles. The search strings contained the following search terms: ‘Africa’ AND ‘toxoplasma’ OR ‘toxoplasmosis’ AND ‘genotype’ OR ‘genetic’ OR ‘diversity’ OR ‘molecular’ OR ‘PCR’ OR ‘strain*’ OR ‘markers’ (Table S1).
Eligibility criteria
English records reporting on T. gondii genotypes in African countries or genotyped isolates that originated from an African country were included. Records encompassed journal articles, preprint articles, conference papers, clinical case reports and dissertations or theses.
Exclusion criteria consisted of the following:
• publications that did not describe T. gondii genotypes in or from Africa;
• records where the data were from previous studies already included in this review;
• the full text was not retrievable;
• details of genotypes were not indicated;
• isolates that did not originate from African countries;
• origin of isolates not indicated;
• insufficient detail on the methods used and genotypes found, reports on research methods only;
• review articles and books or book chapters.
Selection process
The records retrieved from the databases were exported into RefWorks reference manager. The ‘find duplicates’ functions were used to remove duplicate documents. The remaining records were then exported to an Excel spreadsheet and tabulated showing the reference ID, authors, title, periodical, publication year, abstract, notes, publisher, links and DOI of each record. Duplicates that were missed during RefWorks screening were removed by using the ‘find and highlight duplicates’ function in Excel. Screening was done for relevancy on the remaining records. Titles and abstracts were screened by 1 reviewer based on the eligibility criteria; if the information was unclear in the abstract, the full text was screened to retrieve more information. Excel spreadsheets were used throughout the process, highlighting studies either for further assessment or exclusion. Searching and screening processes were performed by 1 reviewer and were verified by 3 other reviewers.
Data extraction and analysis
After the screening process, records highlighted for comprehensive analysis were analysed in detail by 1 reviewer and the results were verified by 3 other reviewers. Records that did not contain enough data to fulfil the eligible outcomes were not included in the review. Data outcomes encompassed year, first author, country, host species, population details, sample types, sample size, diagnostic techniques, genotyping method, molecular markers, number of isolates, genotyping results and isolate names (if stated). RFLP genotype (ToxoDB) designations were not indicated in all the records. However, some of the published isolates were used in the development of the ToxoDB database (Su et al., Reference Su, Khan, Zhou, Majumdar, Ajzenberg, Dardé, Zhu, Ajioka, Rosenthal, Dubey and Sibley2012; Shwab et al., Reference Shwab, Zhu, Majumdar, Pena, Gennari, Dubey and Su2014). RFLP genotype information for some of the isolates was retrieved by searching the ToxoDB database for isolates from African countries and the specific isolate names indicated in the reviewed records. Data from the results in the included studies were tabulated into Excel spreadsheets and descriptively analysed. Various functions in Excel were used to sort, combine and visualize the data into tables and charts. Distribution maps were created using the free and Open Source QGIS software version 3.26.0 (QGIS Association, 2022). Cohen's D effect size was calculated to determine the difference between the means of the results when including and excluding studies with less than 5 molecular markers; the formula and interpretation from Glen (Reference Glen2022) were used in combination with Excel tools.
Results
Study selection
The selection process is shown (Fig. 1). A total of 2357 records were retrieved from the 6 databases. The coverage dates for records retrieved from the respective databases ranged from 1915 to 2022 (Table S1). During the title/abstract screening process, 1484 records were excluded (17 book chapters, 168 review articles and 1299 other records) that did not meet the inclusion criteria. The remaining 79 records were assessed in their entirety and another 37 records were excluded. Reasons for exclusion of these articles include the full text of 1 article was not available, genotyping data were already used in other records included in the review (n = 10), genotypes were not indicated in the results (n = 14), isolates were not from African countries (n = 6), origins of isolates were not clearly indicated (n = 3), research was done on methodology only (n = 2) and 1 record with not enough information for assessment. Forty-two records were found appropriate for inclusion in this systematic review, these included 37 research papers, 3 case reports, 1 case series and 1 published thesis.

Fig. 1. Flow diagram of the search and selection process for the publications.
Study characteristics
The 42 reviewed records consisted of 70 distinct datasets, some studies conducted genotyping on more than 1 type of host or in more than 1 country. An overview of the characteristics of the studies can be seen in Table 1. Sample data were obtained from 21 different host species, 1383 samples from humans and 9074 animal samples, making up the total sample size of 10 457. A total of 885 T. gondii isolates from 20 countries were characterized into archetypal clonal lineages (types I, II and III), clonal variants, regional or local clonal lineages (Africa 1–4), unique strains, mixed types, recombinant strains and unknown types (Table 2, Fig. 2). The PCR-RFLP ToxoDB genotypes of 203 of these isolates, originating from 10 of the countries, were identified as ToxoDB#1, #2, #3, #6, #15, #20, #41/#145, #132, #137, #168, #169, #176, #203, atypical strains, mixed types and unique types (Table 3, Fig. 3). In the 70 datasets, the genotyping methods were based on multiplex PCR of MS markers, PCR-RFLP and sequencing-only methods (Table 4). The number of molecular markers varied across the studies, ranging from 1 to 15 markers, with multilocus typing (genotyping with 5 or more markers) being used in 70% of the datasets.

Fig. 2. Geographical distribution of T. gondii genotypes in Africa, according to clonal genotype designations. The sizes of the pie charts correlate with the total number of isolates and colours are representative of the different genotypes.

Fig. 3. Geographical distribution of T. gondii genotypes in Africa, according to ToxoDB PCR-RFLP genotype designations. The sizes of the pie charts correlate with the total number of isolates and colours are representative of the different genotypes.
Table 1. Summary of study characteristics for records used in this review
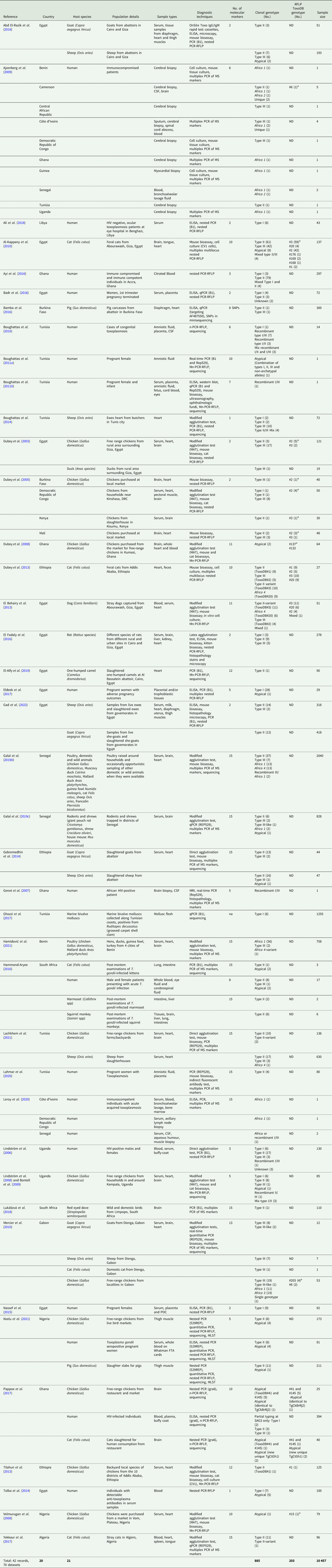
MS, microsatellite marker; ND, not determined; Mn-PCR-RFLP, multilocus nested-PCR-RFLP.
a Genotype on ToxoDB database.
Table 2. Clonal genotypes of T. gondii from reviewed studies, grouped according to country

var, variant; Atyp, atypical; Unk, unknown; Mix, mixed; Recom, recombinant; Unq, unique.
Table 3. ToxoDB PCR-RFLP genotypes of T. gondii from reviewed studies, grouped according to country

Atyp, atypical; Mix, mixed; Unq, unique.
Table 4. Molecular markers used in the reviewed studies

NA, not applicable; SNPs, single-nucleotide polymorphisms.
Genetic diversity in Africa
Northern Africa
Studies from Northern Africa (Egypt, Tunisia, Algeria, Libya) made up 35.7% (25/70) of the total datasets in this review. A total of 377 T. gondii isolates were recovered from 10 different host species with most isolates recovered from cats (127 isolates) and the least isolated recovered from ducks (1 isolate) and a one-humped camel (1 isolate) (Table 1). Records from Egypt were the most out of all the countries, with 31.75% of clonal type isolates (281/885) as well as 68.97% (140/203) of ToxoDB categorized isolates (Tables 2 and 3). The most prevalent clonal genotypes in Egyptian studies were type II (113/281) followed by type III (77/281) and type I (51/281) (Table 2). Other clonal types consisted of atypical (16/281), type II variants (11/281), 6 Africa 4 types, 5 mixed types and 2 unknown types. ToxoDB#3 (72/140) was the most dominant of the PCR-RFLP defined types, followed by ToxoDB#2 (51/140) and ToxoDB#20 (10/140). Isolated cases of ToxoDB#1, #168, #169, #176 and a mixed type were also found. ToxoDB isolate IDs have been assigned to 118/140 ToxoDB RFLP genotyped isolates from cats, dogs and chickens in Egypt (Table 5). Atypical genotypes, unique to Egypt included ToxoDB#168, #169 and #176 (Table 6). Tunisia was like Egypt, in that clonal types were dominated by type II (34/78) genotypes but instead followed by recombinant genotypes (types I/III and I/II, 14/78), type III (13/78) and type I (9/78) (Tables 1 and 2). Clonal type II variant, Africa 4 and mixed genotypes were present in low amounts. Only 6 type I clonal isolates were identified in Libya, samples were from ocular toxoplasmosis patients at an eye hospital in Benghazi (Ali et al., Reference Ali, Elkadi, Mokhtar, Kassem and Mansur2018). In Algeria, only clonal type II (11) genotypes and 1 type II variant was found in samples of stray cats in Algiers. No ToxoDB genotypes were identified from Tunisia, Algeria or Libya.
Table 5. Details of T. gondii isolates characterized according to PCR-RFLP ToxoDB genotypes

Table 6. ToxoDB genotypes in this review shared with other geographical regions

Eastern Africa
Studies from East African countries (Ethiopia, Kenya and Uganda) comprised 11.4% (8/70) of the total number of datasets. Toxoplasma gondii genotypes of 119 isolates were determined in samples sourced from chickens (22 isolates), cats (33 isolates), goats (15 isolates), sheep (18 isolates) and 31 isolates from human samples. Genotypes from Ethiopia made up 7.5% of the overall dataset. Genotypes consisted mainly of clonal type II (39/67), other genotypes found were type II variant (10/67), type III (8/67), Africa 4 (9/67) and 1 atypical isolate. Thirty-four ToxoDB genotypes were described; they consisted of 10 isolates each of ToxoDB#1 and #3, 9 ToxoDB#20 isolates and 5 ToxoDB#2 isolates. None of these isolates has been allocated a ToxoDB isolate ID yet. Only 1 isolate was characterized from Kenya, by Dubey et al. (Reference Dubey, Karhemere, Dahl, Sreekumar, Diabaté, Dabiré, Vianna, Kwok and Lehmann2005), the strain was isolated from a chicken and genotyped as type II with nested-PCR RFLP using 2 molecular markers. The isolate has also been characterized as ToxoDB#3, as the strain identified from the publication with the corresponding ToxoDB ID number was found on ToxoDB.org (Table 5). Sampling from Uganda revealed the presence of 51 T. gondii isolates which consisted of the following clonal genotypes: type II (25/51), type I (12/51), type III (4/51), 1 Africa 1 isolate, 1 atypical isolate, 2 recombinant types (I/III and II/III), 3 mixed genotype (I/II) isolates and 3 unknown genotypes. No ToxoDB number designation could be found for these isolates.
Western Africa
Studies from West African countries (Benin, Burkina Faso, Côte d'Ivoire, Ghana, Guinea, Mali, Nigeria and Senegal) made up 32.9% (23/70) of the total datasets. Genotypes were determined in a total of 279 isolates from 12 different host species: chickens (25 isolates), Muscovy ducks (10 isolates), pigs (14 isolates), humans (114 isolates), Giant pouch rats (5 isolates), house mouse (6 isolates), cats (3 isolates), 2 isolates from Mallard ducks, 1 isolate each from sheep, Guinea fowl, Francolin and shrew. Eighty-seven isolates from Senegal were genotyped which constituted 9.83% of the overall dataset. Clonal type II (43/87) was dominant, other clonal types identified were Africa 1 (16/87), Africa 4 (13/87), type III (9/87), 2 recombinant types (III/Africa 1), 1 isolate each of type III-like, atypical, Africa 2 and mixed (Africa/recombinant I/III). No ToxoDB genotypes were identified. Clonal genotypes from Ghana were the second most prevalent (11.86%) out of all the countries in this review (Table 2). Clonal types were predominantly type II (82/105), 9 atypical genotypes were described, 5 type I isolates, 4 type I/II mixed types, 2 unique genotypes, 1 recombinant type, 1 Africa 1 isolate and 1 type III isolate. Eleven ToxoDB type isolates were isolated from chickens and cats: 1 ToxoDB#132, 1 ToxoDB#137, a mix of ToxoDB#41 and #145 (6 isolates), 1 atypical isolate identical to a Brazilian strain (TgCkBrRj2, EUSMPL0041-1-1) and 2 isolates of new unique genotype that had not been reported previously (TgCtGh1) (Tables 3 and 5). ToxoDB IDs have been assigned to 3/11 ToxoDB RFLP genotyped isolates from only chickens in Ghana (Table 5). Atypical genotypes unique to Ghana included ToxoDB#132 and #137 (Table 6). Studies from Burkina Faso yielded 3 clonal-type isolates (1 type II and 2 type III) and 1 ToxoDB#3 strain. ToxoDB isolate ID (EUSMPL0040-1-112) was assigned to this strain (Table 5). Five clonal type (2 type II, 3 type III) and 4 ToxoDB (3 #2, 1 #3) isolates were identified from chickens in Mali. ToxoDB isolate IDs were assigned to these 4 isolates (Table 5). In Nigeria, studies were done on humans, chickens and pigs. Clonal genotypes consisted of type II (23) and atypical (10) isolates, one of the atypical isolates (isolated from a chicken) was identified as ToxoDB#15 (ToxoDB ID, EUSMPL0040-1-131). The overall results of the 2 studies from Benin (40 isolates) revealed a clear dominance of the Africa 1 genotype (37 isolates), in addition, 2 type III isolates and 1 Africa 4 variant were identified. Africa 1 isolates were also predominant in Côte d'Ivoire; however, only 3 isolates were identified as such. Other strains included 1 type III and 1 unique genotype. All the T. gondii isolates from Côte d'Ivoire were from human hosts. Only 1 T. gondii isolate originated from Guinea, genotyped as Africa 1 by Ajzenberg et al. (Reference Ajzenberg, Yera, Marty, Paris, Dalle, Menotti, Aubert, Franck, Bessières, Quinio, Pelloux, Delhaes, Desbois, Thulliez, Robert-Gangneux, Kauffmann-Lacroix, Pujol, Rabodonirina, Bougnoux, Cuisenier, Duhamel, Duong, Filisetti, Flori, Gay-Andrieu, Pratlong, Nevez, Totet, Carme, Bonnabau, Dardé and Villena2009) and isolated from a myocardial biopsy of an immunocompromised patient. No ToxoDB genotypes were identified from Benin, Côte d'Ivoire or Guinea.
Central Africa
Studies from Central Africa (Cameroon, Democratic Republic of Congo, Central African Republic, Gabon) encompassed 12.9% (9/70) of the total datasets. From Gabon, 1 study was found (Mercier et al., Reference Mercier, Devillard, Ngoubangoye, Bonnabau, Bañuls, Durand, Salle, Ajzenberg and Dardé2010) that genotyped a total of 69 T. gondii isolates from goats, sheep, chickens and cats. There was a predominance of type III (35) followed by Africa 3 (19), Africa 1, type III-like and 1 unique isolate. Four of the Africa 3 isolates were also characterized as ToxoDB#203 and 2 Africa 1 isolates were also identified as ToxoDB#6 during the development of the ToxoDB database. Gabon is the only country where Africa 3 and ToxoDB#203 genotypes were identified. ToxoDB IDs for these isolates are listed in Table 5. Five T. gondii isolates originating from samples of immunocompromised patients from Cameroon were genotyped in the study by Ajzenberg et al. (Reference Ajzenberg, Yera, Marty, Paris, Dalle, Menotti, Aubert, Franck, Bessières, Quinio, Pelloux, Delhaes, Desbois, Thulliez, Robert-Gangneux, Kauffmann-Lacroix, Pujol, Rabodonirina, Bougnoux, Cuisenier, Duhamel, Duong, Filisetti, Flori, Gay-Andrieu, Pratlong, Nevez, Totet, Carme, Bonnabau, Dardé and Villena2009). Four different genotypes were detected: 1 each of type II, Africa 1 and Africa 2 and 2 unique strains. The Africa 1 isolate was identified as ToxoDB#6 (ToxoDB ID EUSMPL0040-1-140). A total of 12 isolates from the Democratic Republic of Congo were genotyped which consisted of 9 type III isolates, and 1 each of type I, type II and Africa 1. Four of the type III isolates originating from chickens were also designated as ToxoDB#2 (Table 5). One T. gondii isolate was genotyped as type III, the isolate originated from the cerebral biopsy of an immunocompromised patient from the Central African Republic; this was also part of the previously mentioned study by Ajzenberg et al. (Reference Ajzenberg, Yera, Marty, Paris, Dalle, Menotti, Aubert, Franck, Bessières, Quinio, Pelloux, Delhaes, Desbois, Thulliez, Robert-Gangneux, Kauffmann-Lacroix, Pujol, Rabodonirina, Bougnoux, Cuisenier, Duhamel, Duong, Filisetti, Flori, Gay-Andrieu, Pratlong, Nevez, Totet, Carme, Bonnabau, Dardé and Villena2009). No ToxoDB genotypes were identified from the Central African Republic.
Southern Africa
Only data from South Africa were retrieved (2 records, 5 datasets), which made up 7.1% (5/70) of the total number of datasets (Hammond-Aryee, Reference Hammond-Aryee2016; Lukášová et al., Reference Lukášová, Kobédová, Halajian, Bártová, Murat, Rampedi and Luus-Powell2018). Most isolates from South Africa were genotyped as type II (18), followed by 4 atypical strains and 1 type III strain (Table 2). No ToxoDB genotypes were identified from South African studies.
Genetic diversity of T. gondii in host species
Clonal genotypes of T. gondii were identified from samples of 21 different host species. Thirteen per cent of samples and 28.2% of genotyped isolates were from humans. Eighty-nine per cent of samples and 71.8% of the genotyped isolates were from animals. In the samples from humans, a total of 250 isolates were characterized into clonal type lineages which consisted of types I, II, III, atypical, Africa 1, Africa 2, unique, mixed, recombinant and unknown genotypes (Fig. 4). The dominant clonal genotype isolated from human samples was type II. Most type I, II and recombinant genotypes were identified from human samples. Africa 2 genotype and unknown genotypes were characterized from human samples only. Only 1 ToxoDB genotype was identified among the human samples (Fig. 5), a ToxoDB#6 isolate (EUSMPL0040-1-140) was also genotyped as Africa 1 (Table 5). The sample originated from a Cameroonian acquired immunodeficiency syndrome patient with toxoplasmic encephalitis (Ajzenberg et al., Reference Ajzenberg, Yera, Marty, Paris, Dalle, Menotti, Aubert, Franck, Bessières, Quinio, Pelloux, Delhaes, Desbois, Thulliez, Robert-Gangneux, Kauffmann-Lacroix, Pujol, Rabodonirina, Bougnoux, Cuisenier, Duhamel, Duong, Filisetti, Flori, Gay-Andrieu, Pratlong, Nevez, Totet, Carme, Bonnabau, Dardé and Villena2009).

Fig. 4. Genetic diversity of T. gondii clonal genotypes among host species displayed in percentages. Different colours are representative of each genotype.

Fig. 5. Genetic diversity of T. gondii ToxoDB PCR-RFLP genotypes among host species displayed in percentages. Different colours are representative of each genotype.
The clonal genotypes obtained from clinical samples were grouped according to the population details into 5 patient groups (Fig. 6). Isolates from the immunocompromised group were the most out of all the isolates identified from human samples (136/250). The most diversity of genotypes was found among immunocompromised individuals, with type II being the dominant genotype in this group. In contrast, type I was predominant in the congenital-related group. This group included isolates from studies where the study population consisted of pregnant women, women with terminated pregnancies and cases of congenital toxoplasmosis or infants (Boughattas et al., Reference Boughattas, Ben-Abdallah, Siala, Souissi, Aoun and Bouratbine2010, Reference Boughattas, Abdallah, Siala, Aoun and Bouratbine2011a, Reference Boughattas, Ben-Abdallah, Siala, Souissi, Maatoug, Aoun and Bouratbine2011b; Tolba et al., Reference Tolba, El-Taweel, Khalil, Hazzah and Heshmat2014; Nassef et al., Reference Nassef, Abd El-Ghaffar, El-Nahas, Hassanain, Shams El-Din and Ammar2015; Badr et al., Reference Badr, Attia, El-Sherbiny, Abd-Allah, Hefny and Salem2016; Eldeek et al., Reference Eldeek, Ahmad, El-Mokhtar, Abdel Kader, Mandour and Mounib2017; Lahmar et al., Reference Lahmar, Lachkhem, Babba, Slama, Trabelsi, Passebosc-Faure, Dardé and Babba2020; Nzelu et al., Reference Nzelu, Kwaga, Kabir, Lawal, Beazley, Evans and Blake2021). Type I was also the dominant genotype in cases involving ocular toxoplasmosis (Tolba et al., Reference Tolba, El-Taweel, Khalil, Hazzah and Heshmat2014; Ali et al., Reference Ali, Elkadi, Mokhtar, Kassem and Mansur2018). The acute toxoplasmosis group included populations with acute T. gondii infection, immunocompetent individuals with acute acquired toxoplasmosis and patients presenting with lymphadenopathy (Tolba et al., Reference Tolba, El-Taweel, Khalil, Hazzah and Heshmat2014; Hammond-Aryee, Reference Hammond-Aryee2016; Leroy et al., Reference Leroy, Houzé, Dardé, Yéra, Rossi, Delhaes, Gabriel, Loubet, Deleplancque, Senneville, Ajana, Sendid and Malvy2020). Type II was mostly found in this group. In a study conducted by Ayi et al. (Reference Ayi, Kwofie, Blay, Osei, Frempong, Koku, Ghansah, Lartey, Suzuki, Boakye, Koram and Ohta2016), in the immunocompetent group, 2 type I isolates and 3 type II isolates were identified. Atypical genotypes were exclusively identified from samples in the congenital-related, ocular and acute toxoplasmosis groups. Africa 2 and unique genotypes were only identified in samples from immunocompromised individuals.

Fig. 6. Toxoplasma gondii clonal genotypes isolated from human samples, grouped into patient groups according to population details. Different colours are representative of the patient groups and are displayed proportionately.
Among the samples tested from animal hosts, the most genotyped T. gondii isolates were from chickens. Africa 4 variant and Africa 3 were only isolated from chickens. Type II genotypes dominated among chicken samples as well as in samples from 14 other animal species (Fig. 4). Three or more different genotypes were seen in samples from chickens, cats, sheep, goats, dogs, rats, pigs, Muscovy ducks and giant pouch rats. Less diversity of T. gondii genotypes and fewer isolates were found in samples from house mouse, squirrel monkey, Mallard ducks, marmoset, duck (species unspecified), francolin, guinea fowl, one-humped camel, red-eyed dove and a shrew (Fig. 4).
RFLP ToxoDB genotypes were identified in samples from cats, chickens and dogs (Fig. 5). The most ToxoDB genotyped isolates were from cats (147/203) followed by chickens (33/203) and dogs (22/203). The majority of genotypes were ToxoDB#3 in dogs and cats and ToxoDB#2 in chickens. These 2 genotypes were isolated from all 3 animal hosts. ToxoDB#1 and #41/#145 were only isolated from cats and chickens. ToxoDB genotypes #168, #169, #176 and 2 unique genotypes were isolated only from cats. Genotypes only isolated from chicken samples included ToxoDB#132, #137, #15, #203, #6 and an atypical isolate. ToxoDB#20 was only present in cat and dog samples. One mixed genotype was only identified from a dog sample.
Molecular markers
Reviews on the genetic diversity of T. gondii generally exclude records where less than 5 molecular markers are used for genotype identification. The reason for this is that an insufficient number of molecular markers will not detect diversity across multiple loci, thus polymorphisms present on loci other than the one targeted will not be detected and the true extent of diversity will be underestimated (Chaichan et al., Reference Chaichan, Mercier, Galal, Mahittikorn, Ariey, Morand, Boumédiène, Udonsom, Hamidovic, Murat, Sukthana and Dardé2017; Fernández-Escobar et al., Reference Fernández-Escobar, Schares, Maksimov, Joeres, Ortega-Mora and Calero-Bernal2022). In this review, less than 5 molecular markers were used in 31% (13/42) of studies which also constitute 27% (19/70) of datasets (Table 4). The most popular markers used in these studies were 3′SAG2 and 5′SAG2 targeting the surface antigen 2 gene. A review of T. gondii diversity in Europe found that 40% of the PCR-RFLP studies screened for inclusion used a single-locus method only (Fernández-Escobar et al., Reference Fernández-Escobar, Schares, Maksimov, Joeres, Ortega-Mora and Calero-Bernal2022). In this review, 19% (8/42) of studies used the 3′SAG2/5′SAG2 combination for genotyping of the SAG2 locus, 4.7% (2/42) used only 1 marker (GRA6 or SAG3), 4.7% (2/42) used 3 markers and 1 study, by El-Alfy et al. (Reference El-Alfy, Abu-Elwafa, Abbas, Al-Araby, Al-Kappany, Umeda and Nishikawa2019), used 12 markers but genotyping was only successful with 2 of the 12 markers (Table 4).
Analysis was performed by comparing the overall diversity data of studies that used any number of molecular markers to the diversity results of studies using 5 or more molecular markers (Fig. 7). Differences were minimal, lower percentages of type I (−2.48%), type II (−5.37%), type III (−0.81%), mixed (−0.60%) and unknown (−0.41%) strains would be seen but it would not change the overall diversity pattern. Type II and III would still be the most prevalent genotypes, and type I prevalence would decrease to be slightly less than Africa 1. Cohen's D effect size (standardized mean difference) of 0.21 was calculated (Table 7). The difference between the means of the overall results, after including or excluding studies with less than 5 molecular markers, is 0.2 standard deviations, which is small and of little practical significance (Glen, Reference Glen2022). All studies irrespective of the number of molecular markers were therefore included in this review.

Fig. 7. Toxoplasma gondii clonal genotypes identified from all studies compared to only studies that used 5 or more molecular markers.
Table 7. Effect size (Cohen's D) between all studies vs studies with only ≥5 markers

a Formula and interpretation according to Glen (Reference Glen2022).
The ToxoDB database was explored for isolates from African countries and isolate names from the original publications were cross-checked. All the isolates on the ToxoDB database from African countries are also present in the studies used in this review. This was a good way to screen for missing results and to confirm the sensitivity of the initial screening process. ToxoDB RFLP genotypes can also be determined by entering genotyping results for each molecular marker of the isolates into the ToxoDB ‘RFLP type’ search function. However, this only works if RFLP results are available and if 10 or more RFLP markers were used.
Discussion
Risk of bias in studies and reporting
There is a scarcity of information on T. gondii genetic diversity in African countries, especially in Southern Africa. In this review, apart from 2 studies from South Africa, no other records were found for Southern African countries. Most African studies on T. gondii primarily focus on prevalence and associated risk factors; molecular tests in general are an expensive commodity. Hence, in this review, studies using less than 5 molecular markers were included to avoid missing any information pertaining to the genotypes of T. gondii in Africa. Several isolates identified in this manner were later characterized using Mn-RFLP-PCR during the construction of the ToxoDB database (Dubey et al., Reference Dubey, Graham, Dahl, Hilali, El-Ghaysh, Sreekumar, Kwok, Shen and Lehmann2003, Reference Dubey, Karhemere, Dahl, Sreekumar, Diabaté, Dabiré, Vianna, Kwok and Lehmann2005).
It should be noted that results from studies that only target the SAG2 locus with PCR-RFLP should be treated with caution. In these instances, atypical, recombinant or exotic strains could be misclassified as type I strains (Ajzenberg et al., Reference Ajzenberg, Cogné, Paris, Bessières, Thulliez, Filisetti, Pelloux, Marty and Dardé2002; Boughattas et al., Reference Boughattas, Ben-Abdallah, Siala, Souissi, Aoun and Bouratbine2010). It is thus possible that some of the type I cases in this review (Nassef et al., Reference Nassef, Abd El-Ghaffar, El-Nahas, Hassanain, Shams El-Din and Ammar2015; Badr et al., Reference Badr, Attia, El-Sherbiny, Abd-Allah, Hefny and Salem2016) are perhaps infected with recombinant or atypical strains or even Africa 1 strains which also contain type I alleles. Repeat testing of these strains using either multiplex PCR of MS markers or Mn-PCR-RFLP genotyping would be advantageous. The Africa 1 genotype like clonal type I is also highly virulent in laboratory mice (Mercier et al., Reference Mercier, Devillard, Ngoubangoye, Bonnabau, Bañuls, Durand, Salle, Ajzenberg and Dardé2010).
The high number of ToxoDB RFLP isolates from Egypt (68.97%) should be taken into consideration before concluding on the overall prevalence of T. gondii genotypes in Africa. An overrepresentation of strains from one geographical area will undoubtedly skew the results, which will not be representative of Africa but rather the areas where more sampling was done.
In compiling this review, 1 reviewer screened records and collected data which could introduce some risk of error. However, all search results and data were verified by 3 other reviewers. The number of databases used in this review was limited to 6, but by using well-known databases with a wide coverage, the possibility of missing major records and datasets is reduced.
Genetic diversity and distribution
The genetic diversity of T. gondii in Africa has so far been described to have a mostly clonal structure, like that of North America and Europe, but with occurrences of African-specific regional or local genotypes, atypical isolates and unique ToxoDB-defined isolates (Dardé, Reference Dardé2008; Sibley et al., Reference Sibley, Khan, Ajioka and Rosenthal2009; Galal et al., Reference Galal, Ajzenberg, Hamidović, Durieux, Dardé and Mercier2018, Reference Galal, Hamidović, Dardé and Mercier2019a). In this review, clonal type II strains were widely distributed among the regions except in Central Africa where type III was more dominant. In West Africa, clonal type II isolates were not found in Benin, Guinea and Côte d'Ivoire; however, the Africa 1 genotype was dominant. ToxoDB#2 and #3 amounted to similarly elevated prevalences as clonal types III and II, although ToxoDB#3 strains were mostly from North (Egypt) and East Africa (Ethiopia, Kenya). ToxoDB#1, which like ToxoDB#3 is of the type II lineage, was only present in Egypt and Ethiopia.
ToxoDB#2 (type III lineage) was more widely distributed but not found in the countries around the Gulf of Guinea (Ghana, Nigeria, Cameroon and Gabon). Similarly, a low incidence of type III clonal genotypes was found in this geographical area, apart from Gabon where a relatively high number of clonal type III isolates were identified using multiplex PCR of 13 MS markers by Mercier et al. (Reference Mercier, Devillard, Ngoubangoye, Bonnabau, Bañuls, Durand, Salle, Ajzenberg and Dardé2010). If the type III isolates identified by Mercier et al. (Reference Mercier, Devillard, Ngoubangoye, Bonnabau, Bañuls, Durand, Salle, Ajzenberg and Dardé2010) were to be analysed by Mn-PCR-RFLP, they would probably be classified as ToxoDB#2; however, only the Africa 1 and Africa 3 isolates from Gabon (identified by Mercier et al., Reference Mercier, Devillard, Ngoubangoye, Bonnabau, Bañuls, Durand, Salle, Ajzenberg and Dardé2010) were further defined as ToxoDB#6 and #203 by Shwab et al. (Reference Shwab, Zhu, Majumdar, Pena, Gennari, Dubey and Su2014).
Type I clonal genotypes were found in studies from Egypt, DRC, Ghana, Libya, Tunisia and Uganda. The bulk of the type I isolates were from studies in Egypt; studies on humans were mostly related to congenital toxoplasmosis and 1 study was done on rats. The type I isolates from rats genotyped by El Fadaly et al. (Reference El Fadaly, Ahmad, Barakat, Soror and Zaki2016) were all from Rattus norvegicus and were shown to be highly virulent in mouse bioassays. Wild rodent species are significant intermediate reservoirs for T. gondii as they are commonly preyed upon by cats and form an integral part of the life cycle (Webster, Reference Webster1994; Galal et al., Reference Galal, Schares, Stragier, Vignoles, Brouat, Cuny, Dubois, Rohart, Glodas, Dardé, Kane, Niang, Diallo, Sow, Aubert, Hamidović, Ajzenberg and Mercier2019c). The virulence effect of T. gondii in wild rodent species is not the same as in laboratory mice. Native rodent species in a geographical area can develop resistance to virulent T. gondii strains circulating within that environment (Galal et al., Reference Galal, Schares, Stragier, Vignoles, Brouat, Cuny, Dubois, Rohart, Glodas, Dardé, Kane, Niang, Diallo, Sow, Aubert, Hamidović, Ajzenberg and Mercier2019c).
Type I isolates from Tunisia were sourced from cases of human congenital toxoplasmosis, sheep and marine bivalve molluscs. The sheep and human samples were from the same geographical area and the type I strains grouped phylogenetically with the virulent RH type I reference strain as well as type I strains from Ugandan chickens (Boughattas et al., Reference Boughattas, Ayari, Sa, Aoun and Bouratbine2014). Among ruminants, type I strains have been identified in samples from sheep in Spain, the UK and Iran, in samples from goats from Japan, China and Italy, in cattle from China and camels in Abu Dhabi (Sharif et al., Reference Sharif, Amouei, Sarvi, Mizani, Aarabi, Hosseini and Daryani2017). Ghozzi et al. (Reference Ghozzi, Marangi, Papini, Lahmar, Challouf, Houas, Ben Dhiab, Normanno, Babba and Giangaspero2017) tested wild shellfish as bioindicators to investigate the contamination of Tunisian coastal areas with protozoan parasites. The authors attributed the high level of oocyst-contaminated clams (Ruditapes decussatus) to the large numbers of stray cats in Tunisia.
Free-range chickens have also been classified as good bioindicators of T. gondii prevalence in the environment; as they roam freely, they are more likely to ingest oocysts mixed with foodstuffs in the soil (Dubey et al., Reference Dubey, Karhemere, Dahl, Sreekumar, Diabaté, Dabiré, Vianna, Kwok and Lehmann2005). Samples from chickens of the DRC and Uganda harboured a small number of type I isolates (Dubey et al., Reference Dubey, Karhemere, Dahl, Sreekumar, Diabaté, Dabiré, Vianna, Kwok and Lehmann2005; Lindström et al., Reference Lindström, Sundar, Lindh, Kironde, Kabasa, Kwok, Dubey and Smith2008; Bontell et al., Reference Bontell, Hall, Ashelford, Dubey, Boyle, Lindh and Smith2009). Interestingly, no type I isolates were identified in a study by Lachkhem et al. (Reference Lachkhem, Galal, Lahmar, Passebosc, Riahi, Plault, Dardé, Mercier and Babba2021) on genotypes of T. gondii in chickens from Tunisia. Similarly, no type I isolates were found in chickens from Egypt by Dubey et al. (Reference Dubey, Graham, Dahl, Hilali, El-Ghaysh, Sreekumar, Kwok, Shen and Lehmann2003). In this review, no ToxoDB#10 genotypes (that correlates to clonal type I) were recorded among the isolates genotyped by Mn-PCR-RFLP.
The Africa 1 genotype was identified in West- and Central African countries as well as Uganda in East Africa. The distribution seems to overlap with the countries that have tropical rainforest biomes, near the equator. ToxoDB#6 (RFLP type of Africa 1) was also identified only within this geographical area. This corresponds with findings in a review by Galal et al. (Reference Galal, Ajzenberg, Hamidović, Durieux, Dardé and Mercier2018). The Africa 1 genotype belongs to haplogroup 6 which also comprises several Brazilian strains. In addition, haplogroup 6 is clustered into clade A together with strains from haplogroup 1 (contains clonal type I and atypical Central/South American strains) and haplogroup 14 (consisting of Africa 3, equivalent to ToxoDB#203 and atypical Brazilian strains), an obvious shared ancestry has been revealed among these haplogroups (Su et al., Reference Su, Khan, Zhou, Majumdar, Ajzenberg, Dardé, Zhu, Ajioka, Rosenthal, Dubey and Sibley2012; Shwab et al., Reference Shwab, Zhu, Majumdar, Pena, Gennari, Dubey and Su2014). It has been proposed that type I could be a divergent strain of Africa 1 and that this genotype might have been introduced from Africa to the Americas via the transatlantic slave trade between the Americas, Europe and Africa from the 16th to the 19th century (Mercier et al., Reference Mercier, Devillard, Ngoubangoye, Bonnabau, Bañuls, Durand, Salle, Ajzenberg and Dardé2010; Hamidović et al., Reference Hamidović, Etougbétché, Tonouhewa, Galal, Dobigny, Houémènou, Da Zoclanclounon, Amagbégnon, Laleye, Fievet, Piry, Berthier, Pena, Dardé and Mercier2021). Cases of infection with Africa 1 have also been recorded in Europe and Turkey. Isolates were found in human new-borns and stray cats in Turkey, human amniotic fluid and a central nervous system sample in Denmark as well as ocular fluid samples from humans in France (Fekkar et al., Reference Fekkar, Ajzenberg, Bodaghi, Touafek, Le Hoang, Delmas, Robert, Dardé, Mazier and Paris2011; Can et al., Reference Can, Döşkaya, Ajzenberg, Özdemir, Caner, İz, Döşkaya, Atalay, Çetinkaya, Ürgen, Karaçalı, Ün, Dardé and Gürüz2014; Chaichan et al., Reference Chaichan, Mercier, Galal, Mahittikorn, Ariey, Morand, Boumédiène, Udonsom, Hamidovic, Murat, Sukthana and Dardé2017; Jokelainen et al., Reference Jokelainen, Murat and Nielsen2018).
Mercier et al. (Reference Mercier, Devillard, Ngoubangoye, Bonnabau, Bañuls, Durand, Salle, Ajzenberg and Dardé2010) suggested that Africa 1 and Africa 3 strains may be new major clonal lineages due to the extensive distribution of these types and the predominantly clonal propagation of T. gondii in the domestic environment. The authors themselves however state that more sampling is needed to confirm such a theory. In this review, Africa 3 (ToxoDB#203) strains were isolated from chickens in several towns in Gabon. This regional genotype was found to be virulent for mice but less virulent than Africa 1 (Mercier et al., Reference Mercier, Devillard, Ngoubangoye, Bonnabau, Bañuls, Durand, Salle, Ajzenberg and Dardé2010). Its presence only in Gabon could indicate that this strain has established itself in this country and is circulating readily within the environment.
Africa 4 isolates and the equivalent ToxoDB#20 RFLP type isolates are present in North-, East- and West Africa, Asia and the United Arab Emirates (Dubey et al., Reference Dubey, Choudhary, Tilahun, Tiao, Gebreyes, Zou and Su2013; El Behairy et al., Reference El Behairy, Choudhary, Ferreira, Kwok, Hilali, Su and Dubey2013; Chaichan et al., Reference Chaichan, Mercier, Galal, Mahittikorn, Ariey, Morand, Boumédiène, Udonsom, Hamidovic, Murat, Sukthana and Dardé2017; Galal et al., Reference Galal, Sarr, Cuny, Brouat, Coulibaly, Sembène, Diagne, Diallo, Sow, Hamidović, Plault, Dardé, Ajzenberg and Mercier2019b; Lachkhem et al., Reference Lachkhem, Galal, Lahmar, Passebosc, Riahi, Plault, Dardé, Mercier and Babba2021). These genotypes have been isolated from: dogs in Egypt and Sri Lanka, cats in Ethiopia and China, sand cats in the United Arab Emirates; sheep in Tunisia and a variety of hosts consisting of chickens, Muscovy ducks and a cat in Senegal. The Africa 4 variant was isolated from a chicken in Benin and ToxoDB#137, which is the RFLP equivalent to the Africa 4 variant, was isolated from a chicken in Ghana (Dubey et al., Reference Dubey, Huong, Lawson, Subekti, Tassi, Cabaj, Sundar, Velmurugan, Kwok and Su2008; Hamidović et al., Reference Hamidović, Etougbétché, Tonouhewa, Galal, Dobigny, Houémènou, Da Zoclanclounon, Amagbégnon, Laleye, Fievet, Piry, Berthier, Pena, Dardé and Mercier2021). This genotype has also been described in Serbia from the amniotic fluid of an immunocompromised patient with no history of travel and in a congenitally infected case from Bulgaria (Uzelac et al., Reference Uzelac, Klun, Ćirković, Bauman, Bobić, Štajner, Srbljanović, Lijeskić and Djurković-Djaković2021).
The dispersal of African T. gondii genotypes to other countries could involve several pathways. The land and maritime trade between Northeast Africa, Asia and Europe via the Silk Road may have contributed to the dissemination of T. gondii genotypes (Chaichan et al., Reference Chaichan, Mercier, Galal, Mahittikorn, Ariey, Morand, Boumédiène, Udonsom, Hamidovic, Murat, Sukthana and Dardé2017; Galal et al., Reference Galal, Sarr, Cuny, Brouat, Coulibaly, Sembène, Diagne, Diallo, Sow, Hamidović, Plault, Dardé, Ajzenberg and Mercier2019b). Seasonal migration of wild birds between Africa and Europe may also play a role in the accrual of African genotypes in European countries (Uzelac et al., Reference Uzelac, Klun, Ćirković, Bauman, Bobić, Štajner, Srbljanović, Lijeskić and Djurković-Djaković2021). Every year 5 billion wild birds of 187 species migrate from Europe and Asia to Africa (Parin et al., Reference Parin, Kirkan, Erbaş, Kideghesho and Rija2018). Migratory birds can spread a variety of diseases to resident bird populations and poultry during stopovers along their flyways (Parin et al., Reference Parin, Kirkan, Erbaş, Kideghesho and Rija2018). It is feasible that Toxoplasma-infected migratory birds may en route to their destination introduce new genotypes into an environment, via predation or succumbing to disease and being eaten by scavengers (Uzelac et al., Reference Uzelac, Klun, Ćirković, Bauman, Bobić, Štajner, Srbljanović, Lijeskić and Djurković-Djaković2021).
Illegal animal smuggling and illicit animal shipments contribute massively to the distribution of zoonotic diseases across the world. Between 2009 and 2019, approximately 500 high-zoonotic-risk trafficking instances were identified in the aviation sector (Spevack, Reference Spevack2020). These high-risk instances involve live animals, bushmeat, domesticated animals and animal products that transit through or are destined for virtually every region across the globe (Spevack, Reference Spevack2020). Practices such as these can undoubtedly contribute to the proliferation of non-native T. gondii genotypes into a new region.
In Central/South America, the assortment of T. gondii genotypes could demonstrate that recombination of the parasite takes place more frequently, possibly due to less intensive agricultural breeding, the presence of a wide variety of wildlife hosts, the more frequent sexual replication within a higher number of wild felids that cover large territories and the already established presence of a large gene pool of ancient parasite lineages with more recombinations and mutations (Ajzenberg et al., Reference Ajzenberg, Bañuls, Su, Dumètre, Demar, Carme and Dardé2004; Wendte et al., Reference Wendte, Gibson and Grigg2011; Su et al., Reference Su, Khan, Zhou, Majumdar, Ajzenberg, Dardé, Zhu, Ajioka, Rosenthal, Dubey and Sibley2012; Shwab et al., Reference Shwab, Saraf, Zhu, Zhou, McFerrin, Ajzenberg, Schares, Hammond-Aryee, van Helden, Higgins, Gerhold, Rosenthal, Zhao, Dubey and Su2018; Galal et al., Reference Galal, Hamidović, Dardé and Mercier2019a). In this review, atypical strains solely identified in Africa are ToxoDB#132, #168, #169, #176 and #203. These strains were found in cats from Egypt, and chickens in Gabon and Ghana (Dubey et al., Reference Dubey, Huong, Lawson, Subekti, Tassi, Cabaj, Sundar, Velmurugan, Kwok and Su2008; Al-Kappany et al., Reference Al-Kappany, Rajendran, Abu-Elwafa, Hilali, Su and Dubey2010; Mercier et al., Reference Mercier, Devillard, Ngoubangoye, Bonnabau, Bañuls, Durand, Salle, Ajzenberg and Dardé2010). Unclassified unique T. gondii genotypes were isolated exclusively in samples from Cameroon, Gabon, Côte d'Ivoire and Ghana (Ajzenberg et al., Reference Ajzenberg, Yera, Marty, Paris, Dalle, Menotti, Aubert, Franck, Bessières, Quinio, Pelloux, Delhaes, Desbois, Thulliez, Robert-Gangneux, Kauffmann-Lacroix, Pujol, Rabodonirina, Bougnoux, Cuisenier, Duhamel, Duong, Filisetti, Flori, Gay-Andrieu, Pratlong, Nevez, Totet, Carme, Bonnabau, Dardé and Villena2009; Mercier et al., Reference Mercier, Devillard, Ngoubangoye, Bonnabau, Bañuls, Durand, Salle, Ajzenberg and Dardé2010; Pappoe et al., Reference Pappoe, Cheng, Wang, Li, Obiri-Yeboah, Nuvor, Ambachew, Hu, Luo, Chu, Xu and Shen2017). This indicates that there is a relative amount of genetic diversity different to the predominantly clonal structures seen in Europe. Most studies across the world have been focused on sampling from only humans and domestic animals, the genetic diversity of T. gondii isolates infecting wildlife is much greater, with more virulent isolates in the wild, which could be a public health concern as humans encroach into previously unpopulated areas (Wendte et al., Reference Wendte, Gibson and Grigg2011). Widespread sampling of all African countries, especially of wildlife, could perhaps reveal a variety of genotypes like Central/South America.
Disease in humans
A high prevalence of type I strains which are more prone to reactivation was found in samples of HIV-positive patients by Khan et al. (Reference Khan, Su, German, Storch, Clifford and Sibley2005). In contrast, Ajzenberg et al. (Reference Ajzenberg, Yera, Marty, Paris, Dalle, Menotti, Aubert, Franck, Bessières, Quinio, Pelloux, Delhaes, Desbois, Thulliez, Robert-Gangneux, Kauffmann-Lacroix, Pujol, Rabodonirina, Bougnoux, Cuisenier, Duhamel, Duong, Filisetti, Flori, Gay-Andrieu, Pratlong, Nevez, Totet, Carme, Bonnabau, Dardé and Villena2009) found a predominance of type II isolates among 88 samples from immunocompromised individuals around the world, with a large proportion of type I/III recombinant isolates identified from African patients with reactivated chronic infections. Although a correlation between geographic origin and strain types was established, the authors concluded that there was no significant difference in the clinical indicators and the outcome when graded against the genotype involved. In this review, different genotypes were found among the immunocompromised clinical group with a dominance of type II isolates, corresponding to overall observations by Ajzenberg et al. (Reference Ajzenberg, Yera, Marty, Paris, Dalle, Menotti, Aubert, Franck, Bessières, Quinio, Pelloux, Delhaes, Desbois, Thulliez, Robert-Gangneux, Kauffmann-Lacroix, Pujol, Rabodonirina, Bougnoux, Cuisenier, Duhamel, Duong, Filisetti, Flori, Gay-Andrieu, Pratlong, Nevez, Totet, Carme, Bonnabau, Dardé and Villena2009). Another observation was that Africa 1 isolates were found more in the immunocompromised group than in the other clinical groups. Seeing as Africa 1 types contain type I alleles, a possible correlation can also be formed with the findings of Khan et al. (Reference Khan, Su, German, Storch, Clifford and Sibley2005). Africa 2 and unique strains were only identified in the immunocompromised group and not in any of the other clinical groups. Africa 2 was also not identified among any of the other species mentioned in this review. Unique genotypes were however also identified in cats and a chicken (Mercier et al., Reference Mercier, Devillard, Ngoubangoye, Bonnabau, Bañuls, Durand, Salle, Ajzenberg and Dardé2010; Pappoe et al., Reference Pappoe, Cheng, Wang, Li, Obiri-Yeboah, Nuvor, Ambachew, Hu, Luo, Chu, Xu and Shen2017).
A possible connection between specific T. gondii genotype and congenital toxoplasmosis as well as ocular toxoplasmosis is seen in this review. There have been reports on severe or complicated cases of congenital toxoplasmosis linked to infections with type I, atypical and recombinant I/III or I/II strains (Boughattas et al., Reference Boughattas, Ben-Abdallah, Siala, Souissi, Aoun and Bouratbine2010, Reference Boughattas, Abdallah, Siala, Aoun and Bouratbine2011a, Reference Boughattas, Ben-Abdallah, Siala, Souissi, Maatoug, Aoun and Bouratbine2011b; Delhaes et al., Reference Delhaes, Ajzenberg, Sicot, Bourgeot, Dardé, Dei-Cas and Houfflin-Debarge2010; Eldeek et al., Reference Eldeek, Ahmad, El-Mokhtar, Abdel Kader, Mandour and Mounib2017). The main factor for the severity of congenital toxoplasmosis is the stage of pregnancy at the time of infection; infection in early pregnancy has more severe consequences than infection late in pregnancy (Ajzenberg et al., Reference Ajzenberg, Cogné, Paris, Bessières, Thulliez, Filisetti, Pelloux, Marty and Dardé2002). A review of the strain hypothesis showed that cases of congenital toxoplasmosis with atypical strains are more likely to have a poor outcome irrespective of the pregnancy trimester when compared to type II strains that cause fewer complications when the infection is acquired at the beginning of the third trimester (Ajzenberg et al., Reference Ajzenberg, Cogné, Paris, Bessières, Thulliez, Filisetti, Pelloux, Marty and Dardé2002; Delhaes et al., Reference Delhaes, Ajzenberg, Sicot, Bourgeot, Dardé, Dei-Cas and Houfflin-Debarge2010; Xiao and Yolken, Reference Xiao and Yolken2015). In addition, the activation of host susceptibility genes for psychoses has been linked specifically to type I strains, with the adult offspring of type I infected mothers having an increased risk for the development of psychotic illnesses and schizophrenia (Xiao et al., Reference Xiao, Buka, Cannon, Suzuki, Viscidi, Torrey and Yolken2009).
On analysis of clinical manifestations in humans, most T. gondii type I strains in this review were from samples of congenital-related toxoplasmosis cases; these type I isolates were exclusively from Tunisia and Egypt. Other genotypes in this clinical group were type II, recombinant types I/III and I/II, atypical genotypes and unknown types. Most studies were on women that had abnormal or severe pregnancy outcomes (Boughattas et al., Reference Boughattas, Ben-Abdallah, Siala, Souissi, Aoun and Bouratbine2010, Reference Boughattas, Abdallah, Siala, Aoun and Bouratbine2011a, Reference Boughattas, Ben-Abdallah, Siala, Souissi, Maatoug, Aoun and Bouratbine2011b; Nassef et al., Reference Nassef, Abd El-Ghaffar, El-Nahas, Hassanain, Shams El-Din and Ammar2015; Badr et al., Reference Badr, Attia, El-Sherbiny, Abd-Allah, Hefny and Salem2016; Eldeek et al., Reference Eldeek, Ahmad, El-Mokhtar, Abdel Kader, Mandour and Mounib2017). In all but one of the studies in this review, the congenital cases related to type I infections had severe outcomes. Patients in the study by Tolba et al. (Reference Tolba, El-Taweel, Khalil, Hazzah and Heshmat2014) were asymptomatic, despite evidence of type I and atypical genotypes of T. gondii in the samples. Another Egyptian study on females with adverse pregnancy outcomes stated in their abstract that T. gondii type II was found more (87%) than type I (13%) (Abdel-Hameed and Hassanein, Reference Abdel-Hameed and Hassanein2008). This record was not recovered during the compiling of articles for this review and only discovered later in the review process; full text for this publication could not be retrieved upon an investigation of this omission. Type I, II and unknown genotypes were detected from samples of women infected in their first trimester that presented with terminated pregnancies (Badr et al., Reference Badr, Attia, El-Sherbiny, Abd-Allah, Hefny and Salem2016). The higher prevalence of type I genotypes in this review and the detection of type II in first-trimester-infected cases, having adverse outcomes in both instances, correlate with the findings of Rico-Torres et al. (Reference Rico-Torres, Vargas-Villavicencio and Correa2016) and Ajzenberg et al. (Reference Ajzenberg, Cogné, Paris, Bessières, Thulliez, Filisetti, Pelloux, Marty and Dardé2002). Type I and atypical isolates were linked to severe congenital toxoplasmosis. Moreover, type I as well as type II are implicated in lethal infections of untreated congenital toxoplasmosis and maternal infections with type II during the first trimester were more likely to lead to fetal damage.
In Lahmar et al. (Reference Lahmar, Lachkhem, Babba, Slama, Trabelsi, Passebosc-Faure, Dardé and Babba2020), Tunisian women acquired infection with type II during pregnancy, 1 infant out of 4 presented with peripheral chorioretinitis scarring at 1-month follow-up. In the 3 asymptomatic infants, the mothers became infected late in the second trimester. Type II strains cause fewer complications when infection is acquired late in the pregnancy (Ajzenberg et al., Reference Ajzenberg, Cogné, Paris, Bessières, Thulliez, Filisetti, Pelloux, Marty and Dardé2002; Xiao and Yolken, Reference Xiao and Yolken2015). Even though exposed infants are asymptomatic after birth, there are risks for complications later in life from non-treatment, such as brain calcifications, hydrocephalus, ocular disease or psychoses (Xiao and Yolken, Reference Xiao and Yolken2015; Lahmar et al., Reference Lahmar, Lachkhem, Babba, Slama, Trabelsi, Passebosc-Faure, Dardé and Babba2020). In a Nigerian study on T. gondii seropositive pregnant women, the authors found type II and atypical strains that shared haplotypes with isolates from samples of free-range chickens and/or pigs collected in the same study areas (Nzelu et al., Reference Nzelu, Kwaga, Kabir, Lawal, Beazley, Evans and Blake2021). This highlights the importance of using genotyping in epidemiological studies and determining infection sources.
Recombinant (I/III and I/II) and atypical genotypes pose a great threat regarding the severity of congenital toxoplasmosis (Ajzenberg et al., Reference Ajzenberg, Cogné, Paris, Bessières, Thulliez, Filisetti, Pelloux, Marty and Dardé2002; Delhaes et al., Reference Delhaes, Ajzenberg, Sicot, Bourgeot, Dardé, Dei-Cas and Houfflin-Debarge2010; Xiao and Yolken, Reference Xiao and Yolken2015). A T. gondii I/III recombinant strain led to the demise of an infected preterm infant diagnosed with chorioretinitis and cardiopathy (Boughattas et al., Reference Boughattas, Ben-Abdallah, Siala, Souissi, Maatoug, Aoun and Bouratbine2011b). In a different study by Boughattas et al. (Reference Boughattas, Ben-Abdallah, Siala, Souissi, Aoun and Bouratbine2010), recombinant strains I/III and I/II as well as mixed infections with both I/II and I/III were detected in congenital toxoplasmosis cases in Tunisia. Unfortunately, the clinical outcomes could only be established in 3 of the cases, 1 neonate developed chorioretinitis and 2 had no toxoplasmic symptoms. A Tunisian case study on a diabetic woman with first-trimester toxoplasmosis discovered an atypical T. gondii strain. Multilocus genotyping of the isolate confirmed a new genotype with an uncommon pattern of type I, II, III and non-archetypal alleles (Boughattas et al., Reference Boughattas, Abdallah, Siala, Aoun and Bouratbine2011a). Due to the gestational age and the increased risk of severe damage to the fetus from such atypical strains, the pregnancy was terminated. Similarly, Delhaes et al. (Reference Delhaes, Ajzenberg, Sicot, Bourgeot, Dardé, Dei-Cas and Houfflin-Debarge2010) reported on a congenital toxoplasmosis case from a French woman infected with an atypical multilocus genotype. Despite proper management with anti-Toxoplasma treatments, bilateral ventricular enlargement and calcifications developed in the fetus; subsequently, this pregnancy was terminated.
Africa 1 strains have been isolated from CSF samples of new borns with congenital toxoplasmosis in Turkey by Döşkaya et al. (Reference Döşkaya, Caner, Ajzenberg, Değirmenci, Dardé, Can, Erdoğan, Korkmaz, Üner, Güngör, Altıntaş and Gürüz2013); none of the Africa 1 strains in this review was from congenital-related samples. Africa 1 was only found in samples from immunocompromised patients and immunocompetent individuals with acute acquired toxoplasmosis (Ajzenberg et al., Reference Ajzenberg, Yera, Marty, Paris, Dalle, Menotti, Aubert, Franck, Bessières, Quinio, Pelloux, Delhaes, Desbois, Thulliez, Robert-Gangneux, Kauffmann-Lacroix, Pujol, Rabodonirina, Bougnoux, Cuisenier, Duhamel, Duong, Filisetti, Flori, Gay-Andrieu, Pratlong, Nevez, Totet, Carme, Bonnabau, Dardé and Villena2009; Leroy et al., Reference Leroy, Houzé, Dardé, Yéra, Rossi, Delhaes, Gabriel, Loubet, Deleplancque, Senneville, Ajana, Sendid and Malvy2020). ToxoDB#41 was identified among the genotypes in congenital toxoplasmosis neonates from southeast Brazil (Carneiro et al., Reference Carneiro, Andrade, Costa, Pinheiro, Vasconcelos-Santos, Ferreira, Su, Januário and Vitor2013). These neonates presented with retinochoroidal lesions. Interestingly, similar strains were isolated from samples of chickens and cats in Ghana. They were characterized as both ToxoDB#41 and #145; these types have also been found in chickens and opossums in Brazil. No ToxoDB genotypes were characterized among the congenital related infections in this review. An opportunity thus arises for the development of surveillance programmes for T. gondii genotypes linked to congenital toxoplasmosis in African countries, to find relationships between genotypes in Africa and those in other continents and to aid in tracking sources of infection such as food animals.
Healthy people can develop ocular toxoplasmosis, and choroiditis may lead to permanent loss of vision (Hammond-Aryee et al., Reference Hammond-Aryee, Esser and van Helden2014; Despommier et al., Reference Despommier, Griffin, Gwadz, Hotez and Knirsh2019). Ocular toxoplasmosis has been frequently associated with infection by type I, atypical and recombinant strains (Khan et al., Reference Khan, Su, German, Storch, Clifford and Sibley2005; Dardé, Reference Dardé2008; Tolba et al., Reference Tolba, El-Taweel, Khalil, Hazzah and Heshmat2014; Xiao and Yolken, Reference Xiao and Yolken2015; Ali et al., Reference Ali, Elkadi, Mokhtar, Kassem and Mansur2018; Pomares et al., Reference Pomares, Devillard, Holmes, Olariu, Press, Ramirez, Talucod, Estran, Su, Dubey, Ajzenberg and Montoya2018). In the ocular toxoplasmosis group in this review, apart from 1 atypical isolate, all other strains were type I (Tolba et al., Reference Tolba, El-Taweel, Khalil, Hazzah and Heshmat2014; Ali et al., Reference Ali, Elkadi, Mokhtar, Kassem and Mansur2018).
Severe and fatal cases of visceral toxoplasmosis, from atypical strains, have been reported in immunocompetent individuals. A highly pathogenic Amazonian strain has been associated with numerous cases of disseminated toxoplasmosis in immunocompetent individuals in French Guiana (Carme et al., Reference Carme, Bissuel, Ajzenberg, Bouyne, Aznar, Demar, Bichat, Louvel, Bourbigot, Peneau, Neron and Dardé2002). An outbreak from a single atypical strain caused mild illness in some individuals and serious illness or death in others (Demar et al., Reference Demar, Ajzenberg, Maubon, Djossou, Panchoe, Punwasi, Valery, Peneau, Daigre, Aznar, Cottrelle, Terzan, Dardé and Carme2007). In the acute toxoplasmosis clinical group of this review, type I, II, III, atypical, Africa 1 and mixed genotypes were identified. The presence of atypical isolates in this clinical group and the absence of such isolates among the immunocompetent group do indicate a degree of involvement. Only type I and type II strains were identified in the immunocompetent group in this review.
Conclusion
Determining which genotypes of T. gondii are prevalent in Africa can aid in its prevention and control. It is clear from this review that connections exist between specific T. gondii genotypes, disease manifestations and severity as well as geographic locale. There is however a scarcity of information on T. gondii in Africa, especially in Southern Africa. Future studies should not only focus in determining the prevalence of T. gondii infection in Africa but also on the genotypes involved. Uniform genotyping methods need to be employed with a continent-wide sampling of an extensive host range involving humans, domestic animals and wildlife. One would only then be able to form a clear concept of the diversity of T. gondii in Africa.
Supplementary material
To view supplementary material for this article, please visit https://doi.org/10.1017/S0031182023000252.
Data availability
A protocol of this systematic review with supplementary data on the search results and data analysis is registered on the Open Science Framework (OSF, https://www.cos.io/). The registration link is: https://doi.org/10.17605/OSF.IO/VM4GS and the project data are available at https://osf.io/gxkst.
Author's contribution
Systematic searching, screening and data analysis processes were performed by I. d. P. and verified by M. M., R. C. and A. M. T.-K. Tables and figures were compiled by I. d. P. The article was written by I. d. P., M. M., R. C. and A. M. T.-K.
Financial support
Funding for the project work of the PhD affiliated with this review is provided by the Women in Research funding programme through the University of South Africa. Bursary funding has been provided by the University of South Africa.
Conflict of interest
None.
Ethical standards
The PhD project affiliated with this review has received ethical clearance from the University of South Africa's College of Agriculture and Environmental Sciences Health Research Ethics Committee. Ethical clearance reference number: 2021/CAES_HREC/190.