INTRODUCTION
Research in the lower Athabasca River drainage of northeastern Alberta, Canada, plays a key role in our understanding of late Quaternary natural and cultural history. Examinations of the routing, timing, and climatic effects of catastrophic drainage from glacial Lake Agassiz are the most widely known Quaternary studies from the region (Smith and Fisher, Reference Smith and Fisher1993; Fisher and Smith, Reference Fisher and Smith1994; Murton et al., Reference Murton, Bateman, Dallimore, Teller and Yang2010; Norris et al., Reference Norris, Tarasov, Monteath, Gosse, Hidy, Margold and Froese2022), and paleoecological work has offered insight into post-glacial ecological change in western Canada (Vance, Reference Vance1986; Bouchet-Bert, Reference Bouchet-Bert2002; Bouchet-Bert and Beaudoin, Reference Bouchet-Bert and Beaudoin2017). A less well-known aspect of the late Quaternary history of this region is its rich archaeological record. Extensive archaeological surveys and excavations have taken place in the area, mostly in advance of oil sands mining development (Ronaghan, Reference Ronaghan and Ronaghan2017). This work has documented one of the highest known concentrations of archaeological sites in the western Canadian boreal forest, with >1,000 sites known within the boundaries of the mineable oil sands region near Fort McMurray, Alberta (Fig. 1; Ronaghan, Reference Ronaghan and Ronaghan2017). Nearly 3.8 million artifacts have been recovered since 1973 (Kristensen et al., Reference Kristensen, Turney, Woywitka, Ives, Tsang, Gingras, Rennie, Robertson, Jones, Speakman and Woywitka2016). The breadth of the archaeological record and the amount of cultural resources management (CRM) work carried out in the region is unprecedented in subarctic Canada (Ronaghan, Reference Ronaghan and Ronaghan2017). Published archaeological research in the area has focused on lithic raw material studies (Fenton and Ives, Reference Fenton, Ives, Lasca and Donahue1990; Tsang, Reference Tsang1998; Kristensen et al., Reference Kristensen, Turney, Woywitka, Ives, Tsang, Gingras, Rennie, Robertson, Jones, Speakman and Woywitka2016), and lithic technology (Le Blanc and Ives, Reference Le Blanc and Ives1986; Ives, Reference Ives, McCormack and Ironside1993, Reference Ives and Ronaghan2017; Younie et al., Reference Younie, Le Blanc and Woywitka2010; Reeves et al., Reference Reeves, Blakey, Lobb and Ronaghan2017; Saxberg and Robertson, Reference Saxberg, Robertson and Ronaghan2017), but accompanying stratigraphic and chronologic studies have been largely lacking (Ronaghan, Reference Ronaghan and Ronaghan2017).

Figure 1. Location of the mineable oil sands region, the Clearwater-Athabasca Spillway (CLAS), and associated archaeological sites. Total extent for glacial Lake Agassiz and glacial Lake McConnell from Teller and Leverington (Reference Teller and Leverington2004) and Smith (Reference Smith1994). Laurentide Ice Sheet margin ca. 11,000 cal years BP (Dyke, Reference Dyke2004).
Cultural chronologies that have been proposed for the region are based on lithic typology and landform associations, mainly due to the low number of available radiocarbon ages from archaeological contexts (Saxberg and Reeves, Reference Saxberg, Reeves, Brink and Dormaar2003; Woywitka, Reference Woywitka and Woywitka2016, Reference Woywitka and Ronaghan2017; Reeves et al., Reference Reeves, Blakey, Lobb and Ronaghan2017; Saxberg and Robertson, Reference Saxberg, Robertson and Ronaghan2017; Woywitka et al., Reference Woywitka, Froese, Wolfe and Ronaghan2017). As such, the regional archaeological record lacks a well-developed temporal framework based on radiometric dates and stratigraphic relations. To fill this knowledge gap, we report stratigraphic sequences and infrared stimulated luminescence (IRSL) ages on potassium feldspar from archaeological and non-archaeological contexts in the region. This work focuses on the immediate post-glacial period, and aims to determine when the landscape became available for human occupation, and to characterize the environmental conditions that existed during initial dispersals into the region. In particular, we address whether the area was initially occupied shortly following late Pleistocene catastrophic drainage, or if it was delayed until the landscape was stabilized by vegetation during ameliorating climatic conditions.
ARCHAEOLOGICAL SETTING
Artifact assemblages are dominated by lithic tools and debitage formed from Beaver River Sandstone (Fenton and Ives, Reference Fenton, Ives, Lasca and Donahue1990), a ubiquitous raw material in northeastern Alberta. This material occurs as a silicified facies in the oilsands-bearing McMurray Formation, and its finer-grained components are well suited to the manufacture of stone tools (Kristensen et al., Reference Kristensen, Turney, Woywitka, Ives, Tsang, Gingras, Rennie, Robertson, Jones, Speakman and Woywitka2016). The richness of the archaeological record has been explained by the presence of two principal source areas for this stone: Beaver River Quarry and Quarry of the Ancestors (Fig. 1). Both provide surface or near-surface access to Beaver River Sandstone in primary context within bedrock, or as reworked gravel deposits (Fenton and Ives, Reference Fenton, Ives, Lasca and Donahue1990; Saxberg, Reference Saxberg2007). Although masked by dense boreal forest today, Beaver River Sandstone sources may have been more apparent to past inhabitants due to the scouring of overlying glacial deposits by catastrophic flooding (Ronaghan and Clarke, Reference Ronaghan and Clarke2000; Saxberg and Reeves, Reference Saxberg, Reeves, Brink and Dormaar2003; Woywitka et al., Reference Woywitka, Froese, Wolfe and Ronaghan2017) and more open vegetation (Bouchet-Bert, Reference Bouchet-Bert2002).
Little is known about the timing of human occupation in the lower Athabasca region. Most sites contain shallow (<1.0 m) cultural deposits that have been mixed by natural processes (e.g., tree root growth, tree stump throws, rodent burrowing), resulting in sediment profiles that contain palimpsests of several occupations (Ives, Reference Ives, McCormack and Ironside1993). The weathering of most organic materials by boreal forest soils has also limited radiocarbon dating to more degradation-resistant calcined bone and charcoal samples from 39 sites collected from various cultural resource management studies. (Woywitka, Reference Woywitka and Woywitka2016; Poletto, Reference Poletto2019). These dates potentially indicate that humans occupied the area from at least 8000 cal yr BP to present. However, confidence in these ages is low given the limited number of dates and inconsistency in analytical methods between studies. Saxberg and Robertson (Reference Saxberg, Robertson and Ronaghan2017) proposed a late Pleistocene-aged occupation at Quarry of the Ancestors based on stratigraphic relations (discussed further below). A projectile point typology-based cultural history also suggests an early deglacial age (ca. 13.0–11.0 ka) for the initial occupation of the region (Saxberg and Reeves, Reference Saxberg, Reeves, Brink and Dormaar2003; Reeves et al., Reference Reeves, Blakey, Lobb and Ronaghan2017) and continued occupation throughout the Holocene (Saxberg and Reeves, Reference Saxberg, Reeves, Brink and Dormaar2003; Reeves et al., Reference Reeves, Blakey, Lobb and Ronaghan2017). A possible fluted point made of Beaver River Sandstone recovered near Cold Lake, Alberta, ~300 km south of our study area suggests that the quarries in the Lower Athabasca were accessible during Clovis times (Kristensen et al., Reference Kristensen, Turney, Woywitka, Ives, Tsang, Gingras, Rennie, Robertson, Jones, Speakman and Woywitka2016). Typological studies such as these provide information about lithic technology, mobility patterns, and subsistence practices. However, the chronological information in these frameworks is based on correlation with radiocarbon-dated sites from distal localities. This type of temporal analysis has proven to yield uncertain results in other parts of the northwestern Canadian boreal forest (Hare et al., Reference Hare, Hammer, Gotthardt, Carlson and Magne2008; Ives, Reference Ives and Ronaghan2017), emphasizing the need for radiometric and stratigraphic ages to complement these frameworks.
GEOLOGICAL SETTING
Archaeological deposits in the mineable oil sands region typically are present near the surface of a sequence of late Pleistocene glacially derived deposits that overlie the oil sands-bearing Early Cretaceous McMurray and Clearwater formations (Hein and Cotterill, Reference Hein and Cotterill2007). Glacial deposits consist of till, glaciolacustrine sands and silts, and coarse boulder gravels and sands (Dyke, Reference Dyke2004; Fenton et al., Reference Fenton, Waters, Pawley, Atkinson, Utting and Mckay2013). The boulder gravel and associated sand units have been interpreted as glacial lake outburst deposits (Smith and Fisher, Reference Smith and Fisher1993), and are observed in the Clearwater River, Athabasca River, and Muskeg River valleys. This distribution forms the basis for the extent of the Clearwater-Athabasca Spillway (Figs. 1, 2; Smith and Fisher, Reference Smith and Fisher1993). The spillway represents the path of catastrophic flooding following establishment of the northwest outlet of glacial Lake Agassiz. There are currently two general schools of thought on the timing of ice retreat and catastrophic flooding in the region: (1) the Laurentide Ice Sheet (LIS) had retreated from the area ca. 13.0 ka, and that catastrophic flooding originated from the northwestern outlet of glacial Lake Agassiz at the beginning or during the Younger Dryas (Murton et al., Reference Murton, Bateman, Dallimore, Teller and Yang2010; Keigwin et al., Reference Keigwin, Klotsko, Zhao, Reilly, Giosan and Driscoll2018; Norris et al., Reference Norris, Garcia-Castellanos, Jansen, Carling, Margold, Woywitka and Froese2021 2022); or (2) the LIS retreated from the area only after ca. 11.3 ka, and flooding originated from a smaller glacial lake in northwestern Saskatchewan (Fisher et al., Reference Fisher, Waterson, Lowell and Hajdas2009). The main control on timing of the flood is the position of the LIS ice margin—the Clearwater-Athabasca Spillway (CLAS) could only have formed during times when the lower Athabasca valley was ice-free. Deglacial chronologies based on radiocarbon ages indicate that ice-free conditions were established ca. 11,500 cal yr BP (Dyke, Reference Dyke2004; Fisher et al., Reference Fisher, Waterson, Lowell and Hajdas2009; Dalton et al., Reference Dalton, Margold, Stokes, Tarasov, Dyke, Adams and Allard2020), whereas luminescence (Munyikwa et al., Reference Munyikwa, Feathers, Rittenour and Shrimpton2011, Reference Munyikwa, Rittenour and Feathers2017; Froese et al., Reference Froese, Young, Norris and Margold2019) and cosmogenic nuclide ages (Norris et al., Reference Norris, Tarasov, Monteath, Gosse, Hidy, Margold and Froese2022) support ice-free conditions ca. 13.0 ka. This discrepancy is due to differences in what is actually being dated by these methods. Radiocarbon-based deglacial chronologies are more likely to represent the presence or absence of vegetation and/or animals following deglaciation and some period of ecesis, rather than the presence or absence of ice (Wolfe et al., Reference Wolfe, Huntley and Ollerhead2004; Munyikwa et al., Reference Munyikwa, Feathers, Rittenour and Shrimpton2011; Froese et al., Reference Froese, Young, Norris and Margold2019; Norris et al., Reference Norris, Tarasov, Monteath, Gosse, Hidy, Margold and Froese2022). In contrast, luminescence and cosmogenic nuclide methods directly date the emplacement and exposure of sediments by glacial or periglacial processes, and therefore may provide more accurate proxies for ice-free conditions. Based on these principles, Norris et al. (Reference Norris, Tarasov, Monteath, Gosse, Hidy, Margold and Froese2022) performed a Bayesian analysis of “high quality” deglacial radiocarbon ages, luminescence ages of post-glacial aeolian deposits, and cosmogenic nuclide ages of glacial erratics associated with the southwestern LIS. Their results indicate that the lower Athabasca region was ice-free by ca. 13.0 ka (Norris et al., Reference Norris, Tarasov, Monteath, Gosse, Hidy, Margold and Froese2022). Young et al. (Reference Young, Reyes and Froese2021) provided a re-analysis of ages associated with the Moorhead Phase of glacial Lake Agassiz, the establishment of which represents drawdown associated with drainage through the NW outlet. Their results indicate a Moorhead drawdown by at least ca. 12.4–12.0 ka (Young et al., Reference Young, Reyes and Froese2021). Both Young et al. (Reference Young, Reyes and Froese2021) and Norris et al. (Reference Norris, Garcia-Castellanos, Jansen, Carling, Margold, Woywitka and Froese2021) concluded that drainage from the NW outlet of glacial Lake Agassiz occurred within the Younger Dryas, but not necessarily at its beginning (Young et al., Reference Young, Reyes and Froese2021; Norris et al., Reference Norris, Tarasov, Monteath, Gosse, Hidy, Margold and Froese2022).
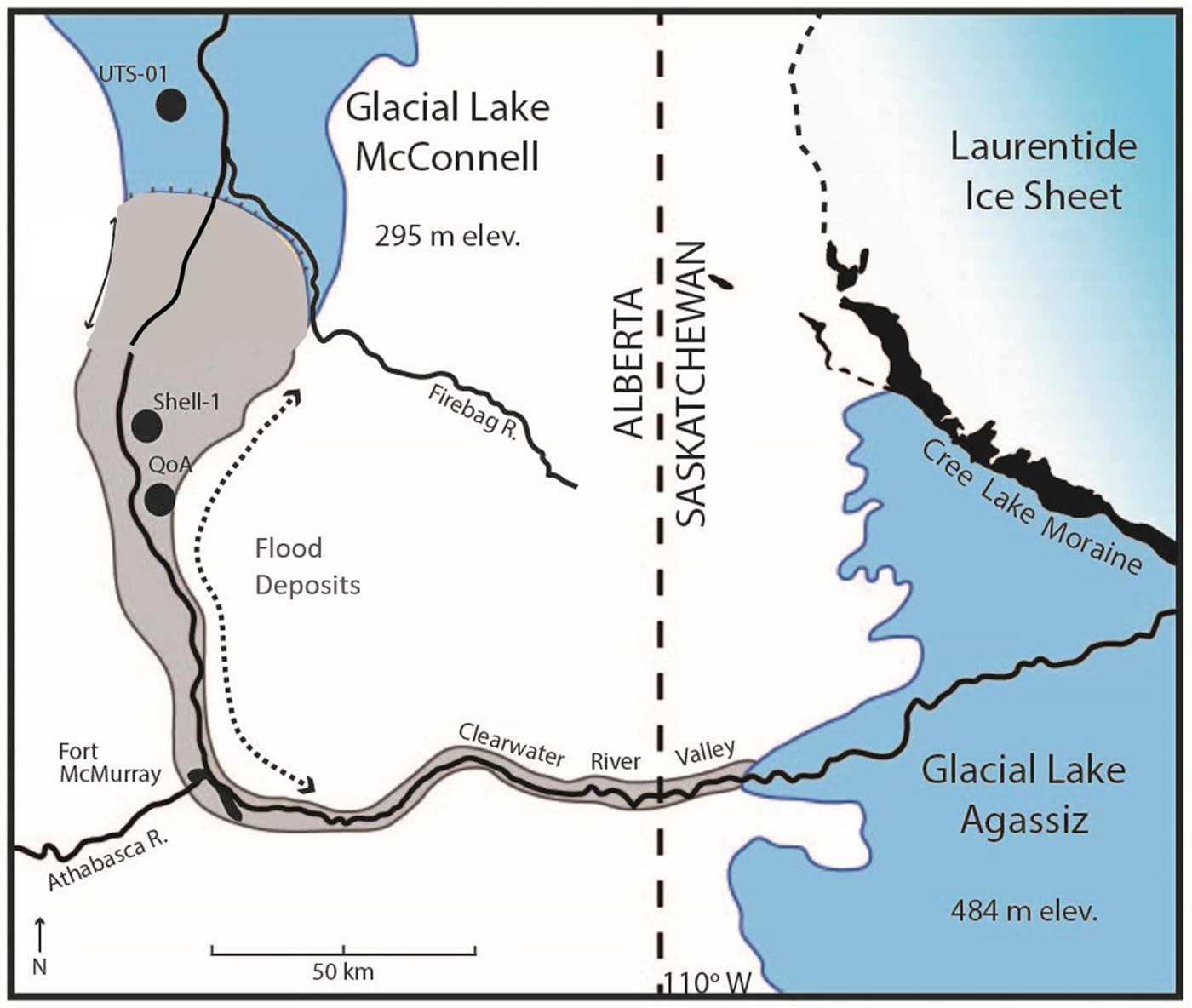
Figure 2. The study area ca. 13,000 years ago with IRSL sample locations (QoA = Quarry of the Ancestors). The northwest outlet of glacial Lake Agassiz and the Clearwater-Athabasca Spillway depositional model is from Smith and Fisher (Reference Smith and Fisher1993). Ice margin north of the Cree Lake Moraine is inferred.
Buried archaeological sites within the spillway commonly occur in association with boulder gravel-cored linear landforms, identified as outburst flood bedforms (Woywitka et al., Reference Woywitka, Froese, Wolfe and Ronaghan2017). Site matrices on these landforms are medium- to fine-grained massive sands that overlie the flood facies described by Smith and Fisher (Reference Smith and Fisher1993). North of the Fort Hills, sites are commonly situated in massive to weakly stratified sands atop stabilized sand dunes derived from delta deposits that prograded into glacial Lake McConnell (Rhine and Smith, Reference Rhine, Smith, Nemec and Steel1988; Woywitka et al., Reference Woywitka, Froese, Wolfe and Ronaghan2017). Artifacts have been recovered at depths from the near surface to ~1 m below the surface in both settings.
Based on these landform associations, the age of flood-bedform exposures and the onset of sand dune deposition should provide a maximum age for human occupation in the flood spillway (or reoccupation of the spillway, if people were present prior to flood deposition). In contrast, a determination of the age of the cessation of aeolian deposition on uplands, representing initial landscape stabilization, should correspond to the beginning of the establishment of forest vegetation communities (c.f., Wolfe et al., Reference Wolfe, Huntley and Ollerhead2004). This landscape transformation would have been characterized by different flora and fauna than the earlier open, windblown conditions, and likely corresponds to the period of initial adoption of subsistence strategies that persisted throughout the Holocene.
METHODS
Sediment exposures at raised landforms and archaeological excavations were logged and photographed in 2006 (DF), 2010 (RW, DF, and SW), and 2012–2014 (RW). Archaeological excavations within Quarry of the Ancestors was carried out at HhOv-319, Locus 1, on the eastern flank of a raised gravel-cored landform (Fig. 4B). This expanded on the work of Saxberg (Reference Saxberg2007). Lithic analysis of the material recovered is presented in Woywitka et al. (in review).
In order to obtain minimum ages for aeolian deposition, we collected eight sediment samples for infrared stimulated luminescence (IRSL) dating at five different landforms, including two boulder-cored bedform features (Fig. 4B, C) and three stabilized dunes north of the Fort Hills (Fig. 4D, E). IRSL dating is an assessment of time elapsed since minerals were last exposed to sunlight, hence the age of deposition for sediments. IRSL samples were taken at depths between 25–155 cm, below visible indicators of bioturbation. At sites Shell-01 and Quarry of the Ancestors, the IRSL samples were taken from sands that overlie boulder gravels. One of the samples from Quarry of the Ancestors (Quarry 1-25) was in direct association with archaeological material. This sample was recovered from sands above a wedge-shaped feature that crosscut lower strata at the site (described in detail below). At the remaining sites, samples were taken from massive and weakly stratified sands on the crests of one transverse dune (FHNW-1), one stabilized parabolic sand dune (UTS-01), and the stabilized blowout of a parabolic dune (NWFH-3). Samples for optical dating were collected using light-tight tubes, whereas bulk samples from surrounding sediments were collected for dose rate and moisture content measurements.
The IRSL dating procedure focused on single grain feldspar analysis. Although we are aware that quartz has yielded luminescence results in previous research in the region (Munyikwa et al., Reference Munyikwa, Feathers, Rittenour and Shrimpton2011, 2017), we use single grain feldspar analysis based on the assumption that feldspar provides a stronger luminescence signal than quartz in this region, and can reduce the potential for contamination from partially bleached grains. Details on dose rate calculations, grain selection, anomalous fading, and age modeling are provided in Supplemental Information-1.
RESULTS
Lithostratigraphy description
Archaeological excavations at Quarry of the Ancestors exposed five sedimentary units. Test pits at the other four sites contained only one or two units (Figs. 5, 6). The following describes the units as encountered at the individual study sites.
Quarry of the Ancestors
The Quarry of the Ancestors site is located on the toeslope of a large gravel bar landform that is consistent in morphology with gravel-cored megaflood bedforms previously identified in the region (Fig. 4; Smith and Fisher, Reference Smith and Fisher1993; Clarke et al., Reference Clarke, Ronaghan, Bouchet-Bert and Ronaghan2017; Woywitka et al., Reference Woywitka, Froese, Wolfe and Ronaghan2017; Norris et al., Reference Norris, Tarasov, Monteath, Gosse, Hidy, Margold and Froese2022). The basal unit observed at Quarry of the Ancestors is a clast-supported, imbricate boulder gravel (Unit 1; Figs. 5, 6). The largest boulders measure ~100 × 100 cm, and are of quartzite, ironstone, bituminous sandstone, or granite lithology. The upper portion of this gravel is draped by a thin (<5 mm thick), discontinuous, dense gray clay layer. Unit 1 is conformably overlain by sand/clay rhythmites (Unit 2; Figs. 5, 6). The clay layers are gray, massive, and ~2 cm thick. Saxberg (Reference Saxberg2007) recorded desiccation cracks on the surface of the uppermost clay layer in the rhythmite sequence. The sand layers are orange in color, medium to coarse in texture, have massive to weak horizontal bedding, and crude normal grading. The upper 1–2 cm of the sand layers are lighter in color than the sand below. Vertical white sand veins that are present in the two upper rhythmites appear to originate from these light-colored sands (Fig. 6). The veins do not crosscut the enclosing clay layers. The lower portions of the sand layers are cemented and reddened at their contacts with the underlying clays.
Unit 3 is a massive, medium- to coarse-textured orange sand that conformably overlies the sand/clay rhythmites of Unit 2 (Figs. 5, 6). There are two thin (<5 mm), discontinuous fine-grained, white sand interbeds within the unit at ~50–55 cm depth below the surface. Portions of the coarser sand enclosing these interbeds are darkened to red-brown and cemented. A continuous band of red-brown coloration also occurs at 40–41 cm depth below the surface in this unit. White sand veins occur throughout in multiple orientations. A unit of blocky textured, pink clay overlies Unit 3 (Unit 4; Figs. 5, 6). It varies in thickness from 0–10 cm, and is laterally discontinuous. The contact between Unit 3 and Unit 4 is undulating and sharp when present.
Unit 5 is the uppermost mineral sediment unit, and consists of massive to crudely stratified, medium-grained sand varying in thickness from 20–60 cm (Unit 5; Figs. 5, 6). A discontinuous cemented layer occurs at its contact with the pink clay of Unit 4. This cemented layer is well defined where Unit 5 is in direct contact with the underlying pink clay. Where the clay is absent, Unit 5 sands grade into Unit 3 sands. The cemented horizon is absent or not well defined where the Unit 4 clays are thin or absent.
A wedge-shaped feature crosscuts the archaeological excavation at Quarry of the Ancestors. The feature was observed on the eastern and northern excavation walls and crosscut depositional units below the massive pink-red clay of Unit 4 (Fig. 6). The average width of the mouth of the trough is 25 cm, and the structure was filled with the overlying and surrounding sediments, including lithic debitage entrained in the upper massive sands. Some of the artifacts were vertically emplaced in the fill. Beds adjacent to the feature were deformed along the wedge margin, mostly in upturned inflections, although downturned inflections were also present (Fig. 6).
The upper massive sands (Unit 5) contain the majority of artifacts recovered during archaeological excavations at Quarry of the Ancestors (Saxberg and Robertson, Reference Saxberg, Robertson and Ronaghan2017; Woywitka, Reference Woywitka2019). The artifacts recovered were nearly entirely Beaver River Sandstone, and represent a biface workshop (Saxberg and Robertson, Reference Saxberg, Robertson and Ronaghan2017; Woywitka, Reference Woywitka2019). Some unmodified pebbles were observed in this unit along with the archaeological material, and polished clasts were also observed. Lithic debitage was reported from the lower massive sands (Unit 3; Figs. 5, 6) during initial work at the Quarry of the Ancestors (Saxberg and Reeves, Reference Saxberg and Reeves2005), but the vertical provenience of these items is uncertain. This is because the excavation was conducted in arbitrary 10-cm levels, and did not map the discontinuous sedimentary contacts between the clay and enclosing sediments or the lateral extent of the wedge feature in detail (Saxberg, Reference Saxberg2007). It is therefore not possible to determine whether an artifact found at a given depth was above or below the pink-red clay in the Saxberg (Reference Saxberg2007) excavations. In our excavation, no artifacts were recovered from within or below the Unit 4 clay (Woywitka, Reference Woywitka2019). As noted above, IRSL sample Quarry 1-25 was taken from Unit 5 sands above the wedge feature, and several artifacts were recovered from the wedge fill, some of which were vertically emplaced.
Shell-1
Shell-1 is situated on the crest of a gravel-cored bedform 10 km north of Quarry of the Ancestors (Fig. 4). At this location, a clast-supported boulder gravel was encountered at 90–100 cm depth below the surface (Fig. 5). This deposit is consistent with Unit 1 observed at Quarry of the Ancestors. The gravel is conformably overlain by massive and weakly horizontally bedded sands (Fig. 5). Thin clay lamellae occur in the horizontally bedded sands at depths between 10–90 cm. No artifacts were identified during our work at this location, but several known archaeological sites occur in the upper sand unit on adjacent landforms (Fig. 4; Clarke et al., Reference Clarke, Ronaghan, Bouchet-Bert and Ronaghan2017).
FHNW-01, NWFH-03, and UTS-01
FHNW-01, NWFH-03, and UTS-01 are all located on stabilized sand dunes north of the Fort Hills. The test pit at FHNW-01 was located on the crest of a transverse dune adjacent to the Athabasca River (Fig. 4). This pit exposed massive sand to 25 cm depth below surface. These grade into weakly horizontally bedded sands that extend to a depth of at least 135 cm (Fig. 5). The test pit at NWFH-03, which was excavated in the stabilized blowout of a parabolic dune, exposed massive sands to a depth of 60 cm that grade into weakly horizontally bedded sands to a depth of at least 160 cm. Two desiccated clay layers, both ~2 cm thick, occur at depths of 110 and 140 cm (Fig. 3). The test pit at UTS-01 was located on a stabilized dune adjacent to a wetland complex and exposed massive sand to a depth of at least 100 cm (Fig. 5). Previous work on dunes adjacent to these locales have identified several archaeological sites, usually with artifacts confined to the upper 30 cm of the sediment profile (Ronaghan, Reference Ronaghan and Ronaghan2017; Woywitka, Reference Woywitka and Ronaghan2017).
Lithostratigraphy interpretation
The gravel at the base of Quarry of the Ancestors and Shell-1 is a local occurrence of the regional boulder gravel described by Smith and Fisher (Reference Smith and Fisher1993) that represents peak flow of catastrophic flooding in the region (Norris et al., Reference Norris, Tarasov, Monteath, Gosse, Hidy, Margold and Froese2022). We interpret the overlying weakly laminated sands and clays (Units 2–4 at Quarry of the Ancestors; Fig. 5) as low-energy fluvial deposits based on these sedimentary characteristics and residual luminescence properties described in the following section and SI-1. Local flow conditions promoted deposition of these units in topographic lows, and pulses in discharge produced the sand/clay rhythmites. In at least one instance, discharge was low enough that water receded sufficiently to subaerially expose a clay surface, as evinced by desiccation cracks in one of the clay layers (Saxberg and Robertson, Reference Saxberg, Robertson and Ronaghan2017). The cemented red sand layers on top of the clay layers in the rhythmites are likely the result of redox processes associated with the blocking of soil water drainage by the underlying clay units. Our interpretation of Units 2–4 differ from previous work that attributed deposition to backflow of flood waters, aeolian processes, and interdune ponding (Saxberg and Robertson Reference Saxberg, Robertson and Ronaghan2017).
The upper massive sand at Quarry of the Ancestors (Unit 5; Figs. 5, 6) is interpreted as aeolian sand based on its looser compaction, massive to crudely stratified structure, and the presence of well-bleached feldspars (discussed below). This unit is also evident at Shell-1 and the three dune sites (Fig. 5). The crudely stratified sands and desiccated clay surfaces that underlie massive sand at Shell-1 and in the parabolic dune blowout at NWFH-3 are interpreted as interdune sandsheet deposits and sediments deposited in ephemeral interdune ponds, based on their geomorphic setting and IRSL characteristics (see below).
The wedge-shaped feature at Quarry of the Ancestors is interpreted as an ice wedge cast. The u-shaped involution at the top and tapering near the bottom of the feature are characteristic of infilled ice wedge casts, as are the inflections of adjacent sediment (Murton and French, Reference Murton and French1993; French, Reference French1996). The cast is filled with overlying and surrounding sediment, with the upper u-shaped area containing a fill of mostly sand (Units 3 and 5; Figs. 5, 6), with deformed red-pink clay lining the margins. The lower v-shaped portion is filled primarily with sand derived from the unit directly overlying the gravel (Unit 2; Fig. 6). No visible vertical foliation is apparent in this fill, suggesting secondary infilling of an ice-wedge cast, rather than primary infilling of a sand wedge (Murton and French, Reference Murton and French1993; French, Reference French1996). The wedge crosscuts units below the red-pink clay, indicating it was formed following deposition of the clay. Several pieces of lithic debitage were excavated from the massive sands that infilled the upper portion of the cast, including a vertically emplaced flake (Figs. 5, 6). The orientation of this flake indicates that artifacts and the upper aeolian sands were deposited when the ice was still present, and slumped into the fill following thaw. The presence of this feature and a similar one identified 3 m south of our excavation in 2004 (Saxberg Reference Saxberg2007; Saxberg and Robertson, Reference Saxberg, Robertson and Ronaghan2017), along with sand veins underlying the red-pink clay, indicate that the site was subject to climatic conditions cold enough to experience thermal contraction cracking following deposition of catastrophic flood deposits, and during deposition of the aeolian sands. Our interpretation of the feature as an ice-wedge cast is consistent with previous descriptions (Saxberg, Reference Saxberg2007; Saxberg and Robertson, Reference Saxberg, Robertson and Ronaghan2017).
Chronology
Eight IRSL ages were obtained from the five sampled landforms (Table 1; Figs. 3, 5, 7). Six of the ages were recovered from aeolian deposits, and two were from fluvial deposits (Table1; Fig. 7). Three stratigraphically coherent ages were obtained from the profile of the archaeological excavation at the Quarry of the Ancestors site. Two samples were recovered from the two lower fluvial sand units (Units 2 and 3; Fig. 5) and one was taken from the upper aeolian sand unit (Unit 5; Figs. 5, 6b, 6e). The lower two samples yielded ages of 14.3 ± 1.1 ka and 12.0 ± 0.8 ka (Table 1). There were abundant grains with residual luminescence identified in these samples during the single-grain selection process, indicating that some grains were not well bleached prior to deposition. As noted above, these results lend support to the interpretation that the lower deposits at Quarry of the Ancestors were deposited in a fluvial environment (see Supplemental Information). The upper eolian sand sample contained well-bleached grains with minimal residual luminescence that yielded an age of 11.3 ± 0.8 ka (Table 1; Figs. 3, 5, 7).
Table 1. IRSL dating results. Datum year 2010 CE. Uncertainty is quoted using 1 σ (68% confidence interval). This reflects common use in the luminescence dating community and allows direct comparison with other luminescence ages. This error includes both random and systematic uncertainty.

An age of 12.3 ± 0.7 ka was recovered from well-bleached, horizontally bedded Unit 5 aeolian sands that overlie boulder gravels at Shell-1, ~10 km north of the Quarry of the Ancestors site (Figs. 3–5, 7). Ages from NWFH-3, a stabilized dune blowout, bracket interdune pond-clay deposition between ca. 14.5 and 11.7 ka, with error (Table 1; Fig. 7). Stabilized dunes at FHNW-1 and UTS-1 yielded ages of 10.6 ± 0.7 and 11.8 ± 0.8 ka, respectively (Table 1; Fig. 7). All samples recovered from stabilized dune areas were from Unit 5 aeolian sands, and were well bleached prior to burial (Figs. 5, 7). The ages from aeolian deposits at the Quarry of the Ancestors, Shell-1, and the stabilized dunes range between 14.5–9.9 ka, including associated uncertainty (Table 1; Figs. 5, 7). Figure 7 illustrates our ages along with other deglacial luminescence ages from the northwest plains (Wolfe et al., Reference Wolfe, Huntley and Ollerhead2004; Munyikwa et al., Reference Munyikwa, Feathers, Rittenour and Shrimpton2011, Reference Munyikwa, Rittenour and Feathers2017) as individual dates and as spatially clustered averages (following Froese et al., Reference Froese, Young, Norris and Margold2019). When considering associated error, these ages provide minimum limiting dates for the emplacement of aeolian deposits, which may be time transgressive between ca. 15–10 ka (Table1; Fig. 7).

Figure 3. Compilation of optical ages obtained from previous works and this study. Ages and their associated uncertainty (1 σ) that occur within 30 km of each other have been averaged, following Froese et al. (Reference Froese, Young, Norris and Margold2019). Optical ages are shown in ka BP. Three outlier ages identified by the original authors have been excluded (see Supplemental Information-2). Ice margins are from Dyke (Reference Dyke2004). Wind directions are from Wolfe et al. (Reference Wolfe, Huntley and Ollerhead2004). Significant archaeological sites are labeled as follows: Wally's Beach (WB), Vermilion Lakes and Minnewanka Lake (VM-LM), and Charlie Lake Cave (CLC). Bracketed abbreviations refer to luminescence age sites from Wolfe et al. (Reference Wolfe, Huntley and Ollerhead2004, Reference Wolfe, Ollerhead, Huntley and Lian2006, Reference Wolfe, Paulen, Smith and Lamothe2007), Munyikwa et al. (Reference Munyikwa, Feathers, Rittenour and Shrimpton2011, Reference Munyikwa, Rittenour and Feathers2017), and this study. Fontas River (FR), Watino (WT), Grande Prairie (GP), Windfall (WF), Fort Assiniboine (FA), Holmes Crossing (HC), Chisholm (CH), Hondo (HO), Decrene (DE), Nelson Lake (NL), Lac La Biche (LLB), Fort McMurray (FM), Quarry of the Ancestors-Fort Hills (QFH), the area around UTS-01 (UTS), High Level (HC), La Crete (LC).

Figure 4. (A) Map showing the location of all IRSL sampling locations. (B) Gravel-cored bedform, IRSL sample location in Quarry of the Ancestors. (C) LiDAR image of gravel-cored bedforms near Ronaghan's Ridge and IRSL sampling location (Shell-1). (D) Transverse (FHNW-1) and parabolic (NWFH-1 and NWFH-3) dune forms north of the Fort Hills and IRSL sampling locations. (E) Parabolic dunes west of the Athabasca River and IRSL sampling location (UTS-01).

Figure 5. Stratigraphic profiles and ages from IRSL sampling locations. All units observed are depicted in the Quarry of the Ancestors profile. Unit 1 boulder gravels appear at the base of Shell-1, overlain by Unit 5 aeolian sands. FHNW, NWFH, and UTS profiles consist entirely of Unit 5 aeolian sands.

Figure 6. (A) Excavation layout at HhOv-319, Locus 1, Quarry of the Ancestors (corresponds to Quarry-1 on Fig. 3). (B) Vertically emplaced flake in ice wedge cast, Unit 104N 101E. (C) Stratigraphy in north wall of Unit 104N 101E. (D) Ice wedge cast, south wall Unit 104N 101E; wedge is ~75 cm in height.

Figure 7. IRSL ages from this study compared to other deglacial aeolian ages in the ice-free corridor/western Canadian great plains (Wolfe et al., Reference Wolfe, Huntley and Ollerhead2004, Reference Wolfe, Ollerhead, Huntley and Lian2006, Reference Wolfe, Paulen, Smith and Lamothe2007; Munyikwa et al., Reference Munyikwa, Feathers, Rittenour and Shrimpton2011, Reference Munyikwa, Rittenour and Feathers2017). Abbreviations as listed in Figure 3. Error bars = 1 σ. Individual ages on left, clustered average ages on right, following Froese (Reference Froese, Young, Norris and Margold2019).
DISCUSSION
Ages from flood deposits
The single-grain ages obtained from waning stage flood deposits at Quarry of the Ancestors range from 15.4 ka (Unit 2) to 11.2 ka (Unit 3) with associated error (Table 1). When considered as individual dates, the Unit 2 age is ca. 1.0 ka older than the earliest estimations of catastrophic drainage in the region (Murton et al., Reference Murton, Bateman, Dallimore, Teller and Yang2010), and the Unit 3 age is consistent with the younger flood chronology forwarded by Fisher et al. (Reference Fisher, Waterson, Lowell and Hajdas2009). When considered together, these dates have a weighted mean age of 13.2 ± 1.0 ka, which overlaps with glacial Lake Agassiz outburst flood ages of 13.2 ± 0.2 ka reported by Murton et al. (Reference Murton, Bateman, Dallimore, Teller and Yang2010). Although our work indicates that these sediments can be dated by single grain IRSL methods, the low numbers of flood deposit samples analyzed here prevent substantive conclusions about the timing of catastrophic flooding.
Post-glacial aeolian deposition
Our results indicate that aeolian deposition was active in the study area shortly following retreat of the LIS and catastrophic outburst flooding. In the Clearwater-Athabasca Spillway, aeolian sands were likely sourced from waning stage flood deposits that capped the boulder-gravel deposits. These waning stage sands would have presented a smaller sediment supply than the deposits to the north of the Fort Hills, where even during peak flow conditions, sand-sized sediment dominated the depositional regime due to expanding flow. This difference in sediment supply is likely the reason why few prominent dunes formed within the spillway, but are abundant north of the Fort Hills. The reworking of these deposits may also account for the overdispersion observed in the luminescence ages (see Table 1 and Supplemental Information-1).
The stratigraphic position of aeolian sands above catastrophic flood deposits indicates that aeolian deposition at Quarry of the Ancestors followed flood sedimentation. The IRSL dates obtained from Quarry of the Ancestors and Shell-1-70 indicate a minimum limiting age range of ca. 13.0–10.5 ka for deposition of the artifact-bearing aeolian sediment in this part of the Clearwater-Athabasca Spillway (Table 1; Figs. 3, 7). The collapse of overlying aeolian sediment and artifacts into the ice wedge cast at Quarry of the Ancestors indicates that windblown sediment and cultural material were deposited following catastrophic flooding, but while ground ice was still present in the form of an ice wedge, and temperatures were cold enough to support permafrost. The minimum age of deposition of the aeolian sands above the ice wedge cast is ca. 12.1–10.5 ka (Quarry 1-25, Table 1; Fig. 5), and indicates that the aeolian deposits and artifacts within the wedge fill were buried by at least this time. Collectively, the sedimentological observations and minimum ages for aeolian deposition are more consistent with late Pleistocene climate conditions than with Early Holocene conditions, and likely relate to cold, dry, and windy conditions that were prevalent during the Younger Dryas. Human occupation elsewhere in the deglacial corridor has been documented during the Younger Dryas (Fedje et al., Reference Fedje, White, Wilson, Nelson, Vogel and Southon1995; Driver et al., Reference Driver, Handly, Fladmark, Nelson, Sullivan and Preston1996; Landals, Reference Landals2008; Waters et al., Reference Waters, Stafford, Kooyman and Hills2015).
Luminescence dates from the upper meter of stabilized sand dunes provide minimum limiting dates for aeolian deposition north of the Fort Hills. The dates range from ca. 13.6–10.6 ka, and were collected from landforms oriented in a direction consistent with southeasterly winds (Table 1; Fig. 4). David (Reference David1981) and Wolfe et al. (Reference Wolfe, Huntley and Ollerhead2004) attributed southeasterly trending dunes in other parts of western Canada to the prevalence of anticyclonic winds around the waning LIS caused by a persistent low pressure cell (Fig. 3). These winds were prevalent within a ~200 km belt of the ice margin. Based on a series of optically stimulated luminescence (OSL) and IRSL ages on stabilized dunes, Wolfe et al. (Reference Wolfe, Huntley and Ollerhead2004) proposed that anticyclonic circulation began to dominate ice marginal wind regimes at ca. 13.0 ka, when the LIS had retreated far enough to the east to allow anticyclonic flow to overtake the influence of westerly winds from Pacific air masses. This belt of anticyclonic winds retreated along with the LIS through time, with northwesterly winds eventually re-establishing dominance in an area once the ice sheet had retreated farther to the northeast (Wolfe et al., Reference Wolfe, Huntley and Ollerhead2004). In this context, dunes oriented with winds originating from the southeast represent the oldest, immediately post-glacial landforms, while dunes oriented with winds originating from the northwest represent a later phase of aeolian deposition (Fig. 3). Anticyclonic winds in western Canada were replaced by Pacific westerly winds by ca. 9.0 ka (Wolfe et al., Reference Wolfe, Huntley and Ollerhead2004). The minimum ages and orientations in this study are consistent with early post-glacial deposition, both in age and orientation, and indicate that anticyclonic winds were active until ca. 10.0 ka (Fig. 3; Supplemental Information-2). Based on this evidence, the LIS margin was likely within ~200 km of the mineable oil sands region (i.e., the Cree Lake Moraine) from immediately after ice recession until ca. 10.0 ka, consistent with earlier ice sheet reconstructions (Dyke, Reference Dyke2004; Froese et al., Reference Froese, Young, Norris and Margold2019; Norris et al., Reference Norris, Garcia-Castellanos, Jansen, Carling, Margold, Woywitka and Froese2021). These ages also indicate that the timing of landscape stabilization was variable through the immediate post-glacial period, with some areas becoming stable as early as ca. 13.0 ka, and other areas remaining active until ca. 10.0 ka.
The IRSL dates from Shell-1, Quarry of the Ancestors, and the dunes constitute an interval from initial LIS retreat to ca. 10.0 ka, during which aeolian deposition was active in the region (Fig. 3). Paleoenvironmental work completed at Kearl Lake, 5 km east of the study area, shows that regional vegetation during the period from ca. 12.0–9.0 ka was a non-analogous open spruce and birch forest with a considerable herbaceous component (Bouchet-Bert and Beaudoin, Reference Bouchet-Bert and Beaudoin2017). Other lake records in the region indicate that prior to 12.0 ka, tundra-like conditions were prevalent (Vance, Reference Vance1986; Hutton et al., Reference Hutton, MacDonald and Mott1994). The immediate post-glacial landscape was likely a mosaic of stable, vegetated areas of shrub-tundra and early boreal parkland (Dyke, Reference Dyke2004) with pockets of aeolian deposition in active dune fields to the north of the Fort Hills, and in isolated areas within the Clearwater-Athabasca Spillway. As previously discussed, continuous permafrost also would have been present during earliest post-glacial times.
Our dates for landscape stabilization roughly coincide with the arrival of Picea glauca (white spruce) in the region, and are consistent with a white spruce dispersal model presented by Ritchie and MacDonald (Reference Ritchie and MacDonald1986). This model proposes that a rapid rise in white spruce in western Canada (ca. 9.5 ka) was the result of seed transport by strong southeasterly anticyclonic winds; seeds were picked up as the winds passed over newly established spruce forests in the central North American interior, and carried northwest along the margin of the LIS (Ritchie and MacDonald, Reference Ritchie and MacDonald1986). The establishment of spruce forest would have reduced sediment mobility by root anchoring and increased shelter from winds. This drop in available sediment and the gradual replacement of anticyclonic winds with weaker westerlies combined to produce regional landscape stability by 9.0 ka (Wolfe et al., Reference Wolfe, Huntley and Ollerhead2004). This pattern is observable in the averaged luminescence ages presented in Figures 3 and 7, where stabilization ages roughly mimic the SW-NE retreat of the LIS, and tend to be older to the southeast of the ice sheet, nearer to the source area of incoming spruce forest.
Our minimum dates for aeolian deposition extend from the Younger Dryas into the early Holocene (ca. 13.0–10.0 ka), and average 12.0 ka ± 0.8 ka. Anticyclonic winds were active during this time; an indication that the LIS margin was within ~200 km of the lower Athabasca valley. As such, the lower Athabasca valley was likely at the very eastern edge of the deglacial corridor when early inhabitants arrived in western Canada. As noted above, our IRSL dates alone do not provide the precision required to determine the timing of deglaciation or catastrophic drainage. However, the presence of permafrost features at Quarry of the Ancestors prior to the emplacement of aeolian deposits strongly suggests subaerial exposure of the landscape during a cold interval, most likely the Younger Dryas. This interpretation is consistent with the deglacial chronology presented by Norris et al. (Reference Norris, Tarasov, Monteath, Gosse, Hidy, Margold and Froese2022) that places the LIS at the Cree Lake moraine, ~200 km northeast of our study area at ca. 13.0 ka. Our findings also are consistent with other deglacial chronologies based on glacial erratic exposure ages and aeolian deposition in the western corridor that indicate ice-free conditions across most of Alberta by ca. 13.0 ka (Figs. 3, 7; Wolfe et al., Reference Wolfe, Huntley and Ollerhead2004; Munyikwa et al., Reference Munyikwa, Feathers, Rittenour and Shrimpton2011, 2017; Froese et al., Reference Froese, Young, Norris and Margold2019; Margold et al., Reference Margold, Gosse, Hidy, Woywitka, Young and Froese2019; Norris et al., Reference Norris, Tarasov, Monteath, Gosse, Hidy, Margold and Froese2022).
In terms of the glacial Lake Agassiz catastrophic drainage, the ice wedge cast that crosscuts flood deposits at Quarry of the Ancestors, is consistent with a Younger Dryas-aged flood, as proposed by Murton et al. (Reference Murton, Bateman, Dallimore, Teller and Yang2010), Young et al. (Reference Young, Reyes and Froese2021), and Norris et al. (Reference Norris, Tarasov, Monteath, Gosse, Hidy, Margold and Froese2022). Our results are not consistent with radiocarbon-based deglacial chronologies (Fisher et al., Reference Fisher, Waterson, Lowell and Hajdas2009; Pedersen et al., Reference Pedersen, Ruter, Schweger, Friebe, Staff, Kjeldsen and Mendoza2016; Dalton et al., Reference Dalton, Margold, Stokes, Tarasov, Dyke, Adams and Allard2020), which place the LIS margin west of our study area at ca. 13.0 ka, and at the Creek Lake moraine only after ca. 11.5 ka (Dalton et al., Reference Dalton, Margold, Stokes, Tarasov, Dyke, Adams and Allard2020). In this scenario, glacial ice would have been present in the lower Athabasca during the interval of aeolian deposition.
Initial human occupation
Although details of the stratigraphy at Quarry of the Ancestors presented here differ from Saxberg and Robertson (Reference Saxberg, Robertson and Ronaghan2017), our results agree with their interpretation that ice wedge formation and early occupation of the site occurred near the Younger Dryas. As discussed above, IRSL ages from Quarry of the Ancestors provide a minimum age of 11.3 ± 0.8 ka (i.e., 12.1–10.5 ka) for deposition of aeolian sands above the wedge, indicating that artifacts were emplaced prior to that time—a span that could reasonably extend into the Younger Dryas. This interpretation of a Younger Dryas-aged occupation at Quarry of the Ancestors places the site in a similar temporal range as the earliest sites in the deglacial corridor to the south and west, including Wally's Beach (ca. 13.3 ka; Waters et al., Reference Waters, Stafford, Kooyman and Hills2015), Vermilion Lakes (ca.12.7 ka; Fedje et al., Reference Fedje, White, Wilson, Nelson, Vogel and Southon1995), Lake Minnewanka (ca. 12.7 ka; Landals, Reference Landals2008), and Charlie Lake Cave (ca. 12.4 ka; Fladmark, et al., Reference Fladmark, Driver and Alexander1989; Driver et al., Reference Driver, Handly, Fladmark, Nelson, Sullivan and Preston1996). This period is well represented in undated sites and collections from northern Alberta (Ives et al., Reference Ives, Froese, Supernant, Yanicki, Graf, Ketron and Waters2013), including potential early blades (Ives, Reference Ives, McCormack and Ironside1993) from the lower Athabasca region, and a Clovis point made of Beaver River Sandstone from Cold Lake, ~300 km south of the Quarry of the Ancestors (Ives et al., Reference Ives, Froese, Supernant, Yanicki, Graf, Ketron and Waters2013). The limiting age presented for cultural material at Quarry of the Ancestors indicates that northeastern Alberta was a viable environment for human occupation at this time. This contrasts with Mandryk's (Reference Mandryk1996) characterization of the central ice-free corridor as inhospitable to humans during the immediate deglacial period. It also contrasts with paleoenvironmental reconstructions of Pedersen et al. (Reference Pedersen, Ruter, Schweger, Friebe, Staff, Kjeldsen and Mendoza2016) that characterize the corridor area around Fort St. John, British Columbia (~600 km west of our study area) as closed or uninhabitable until after 12.8 ka. Our results are consistent with the work of Heintzman et al. (Reference Heintzman, Froese, Ives, Soares, Zazula and Letts2016) that describes the presence of a biome capable of supporting bison and other large mammals, including humans, by ca. 13.0 ka in the central deglacial corridor.
Other typological studies have indicated similarities in lithic types with Pleistocene-Holocene transition assemblages from the northern Plains, particularly Agate Basin and the Cody Complex (Dawe, Reference Dawe2013; Reeves et al., Reference Reeves, Blakey, Lobb and Ronaghan2017). Denali-style microblade assemblages recovered from the oil sands region connect the area to the Alaskan record, although the temporal extent of this technology in Alberta is limited to the Early Holocene (Younie et al., Reference Younie, Le Blanc and Woywitka2010; Magne et al., Reference Magne, Hughes and Kristensen2019). What is clear, however, is that people in northeastern Alberta used stone tool technology similar to groups in central and northwestern North America during the late Pleistocene and Early Holocene.
Whether initial occupation of northeastern Alberta was tied to fluted and stemmed point traditions emanating from the south (Goebel et al., Reference Goebel, Smith, DiPietro, Waters, Hockett, Graf and Gal2013; Heintzman et al., Reference Heintzman, Froese, Ives, Soares, Zazula and Letts2016; Smith and Goebel, Reference Smith and Goebel2018) or microblade traditions from the northwest is not known (Ives et al., Reference Ives, Froese, Supernant, Yanicki, Graf, Ketron and Waters2013). Work at Wally's Beach places of the earliest known occupations of the corridor at or just before the onset of Clovis culture, and provides a potential link between ice-free corridor sites and pre-Clovis occupations south of the LIS and CIS (Waters et al., Reference Waters, Stafford, Kooyman and Hills2015, Reference Waters, Keene, Forman, Prewitt, Carlson and Wiederhold2018; Halligan et al., Reference Halligan, Waters, Perrotti, Owens, Feinberg, Bourne and Fenerty2016). There are currently not enough archaeological sites of early deglacial age in the deglacial corridor to confidently assess its role in the temporal, genomic, and spatial dynamics of the peopling of North America (Raghavan et al., Reference Raghavan, Steinrücken, Harris, Schiffels, Rasmussen, DeGiorgio and Albrechtsen2015; Potter et al., Reference Potter, Reuther, Holliday, Holmes, Miller and Schmuck2017, Reference Potter, Baichtal, Beaudoin, Fehren-Schmitz, Haynes, Holliday and Holmes2018; Froese et al., Reference Froese, Young, Norris and Margold2019). However, it is likely that northeastern Alberta contains additional sites that can provide insight into late Pleistocene and Early Holocene human dispersal relative to the retreating LIS and concomitant ecological changes (Woywitka and Froese, Reference Woywitka and Froese2020). Our results show the potential of stratigraphic analysis and IRSL dating to provide temporal context to these earliest human activities in western North America.
CONCLUSIONS
IRSL ages from windblown sediment in the mineable oil sands region indicate that aeolian deposition occurred shortly after retreat of the LIS from the region. In the Clearwater-Athabasca Spillway, this deposition started following recession of catastrophic flood waters from glacial Lake Agassiz, and ceased by ca. 10.0 ka. In braided delta deposits at the northern terminus of the spillway, fluvial and delta sands were reworked into dunes, with some stabilizing as early as ca. 13.0 ka, and deposition continuing until ca. 10.0 ka. Widespread regional landscape stabilization is likely to have occurred ca. 10.0 ka with the arrival of spruce forests and the replacement of anticyclonic winds with weaker westerly winds originating from Pacific air masses.
Human occupation within the Clearwater-Athabasca Spillway occurred shortly after the recession of catastrophic flood waters from the area, likely during the Younger Dryas. The initial post-flood occupation occurred during a time when climatic conditions were cold enough to support permafrost. This is consistent with a Younger Dryas-aged human occupation of the area. The environment at this time would have included a mosaic of stable, vegetated areas of tundra or thin spruce/birch forest, and open areas of aeolian deposition. Near-surface and surface sources of Beaver River Sandstone were likely readily visible at this time. Beaver River Sandstone continued to be the primary lithic raw material used in the mineable oil sands region throughout the prehistoric record. The Quarry of the Ancestors occupation is potentially among the earliest recorded in northwestern Canada, marking the eastern edge of the deglacial corridor at this time.
Supplementary material
The supplementary material for this article can be found at https://doi.org/10.1017/qua.2022.14
Acknowledgments
Funding for fieldwork in 2012–2013 was provided by the Canadian Circumpolar Institute (CCI) and Northern Scientific Training Program (NSTP) to RW. Primary funding was provided by a Natural Sciences Engineering and Research Council Discovery Grant to DF and funds from the Canada Research Chairs program. DF and ML supplemented field costs and provided analytical funds for IRSL and radiocarbon analysis. The Archaeological Survey of Alberta provided in-kind contributions to the fieldwork and funds for radiocarbon dating. T. Kristensen, R. Graham, B. Romano, J. Pumple, A. Lints, and D. Bereziuk provided field and laboratory assistance. J. Young assisted with drafting figures. The paper was improved considerably by comments from A. Reyes, T. Goebel, J. Ives, and A. Beaudoin and especially by thorough reviews from D. Fedje and S. Forman.