The high level of α-linolenic acid in flaxseed (Linum usitatissimum) makes it a good source of n-3 PUFA from plant origin for humans. Dietary addition of flaxseed increases the n-3:n-6 fatty acid ratio in food, and improvement of the ratio has been recognised as potentially beneficial to human health(Reference Wright, McBride and Holub1). Moreover, flaxseed is the richest source of plant lignans which possess antitumour(Reference Thompson, Seidl and Rickard2), antioxidant(Reference Kitts, Yuan and Wijewickreme3), antithrombic, weakly oestrogenic and anti-oestrogenic(Reference Kitts, Yuan and Wijewickreme3, Reference Martin, Haourigui and Pelissero4) and anti-atherogenic effects(Reference Sano, Oda and Yamashita5). Plant lignans are polyphenolic compounds concentrated in the outer fibre-containing layers and the lignan secoisolariciresinol diglucoside (SDG) represents over 95 % of the total lignans in flaxseed(Reference Thompson, Robb and Serraino6).
The main site for the metabolism of flax lignans in non-ruminant animals is the large intestine(Reference Setchell, Lawson and Mitchell7). On the other hand, recent in vivo results have shown that the rumen is the main site for the metabolism of flax lignans in dairy cows(Reference Gagnon, Côrtes and Da Silva8). Under the action of microflora, the plant lignan SDG is converted mainly into the two mammalian lignans enterodiol and enterolactone (EL), and enterodiol and EL are subsequently absorbed into the blood(Reference Borriello, Setchell and Axelson9). EL is the main lignan metabolite present in ruminal fluid(Reference Gagnon, Côrtes and Da Silva8) and milk(Reference Petit, Gagnon and Priyadarshini10). In non-ruminant animals, mammalian lignans are absorbed in the intestine and under the action of specific enzymes, they are conjugated as sulphate and glucuronide in the intestinal wall and liver(Reference Barnes, Sfakianos and Coward11). Conjugated mammalian lignans are transferred into physiological fluids (e.g. plasma, semen and milk), excreted in the urine or in the intestinal lumen via the enterohepatic circulation(Reference Hoikkala, Schiavoni and Wahala12). The conjugated forms of mammalian lignans are poorly absorbed by the intestine; deconjugation increases the hydrophobicity of lignans and allows their reabsorption. The mammalian lignans are readily cleaved by microbial β-glucuronidases. Microbial β-glucuronidase activity is important for the absorption of mammalian lignans in humans, and this enzyme is positively correlated with the level of plant lignan intake and the urinary excretion of mammalian lignans(Reference Jenab and Thompson13). A recent study(Reference Gagnon, Côrtes and Da Silva8) has shown that concentrations of EL in milk and urine increase similarly with supplementation of flax hulls in the diet of dairy cows, suggesting that microbial β-glucuronidase activity contributes to increasing the amount of lignans in milk as a result of greater gastrointestinal absorption of mammalian lignans.
In dairy cows, microbial β-glucuronidase activity has been shown to be five times higher on average in the faeces than in ruminal fluid, indicating that deconjugation activity may be more important in the large intestine than in the rumen of dairy cows(Reference Gagnon, Côrtes and Da Silva8). Ruminal fluid activity of microbial β-glucuronidase is influenced by the presence of n-3 fatty acids as shown by decreased activity with flax oil supplementation in the rumen(Reference Gagnon, Côrtes and Da Silva8). However, there is no information on the influence of flax oil in the intestine on the activity of microbial β-glucuronidase involved in lignan absorption. Therefore, the objectives of the present study were to investigate the effects of different amounts of flax oil infused in the abomasum on microbial β-glucuronidase activity, the interaction with the presence or absence of flax hulls (source of lignans) in the faeces of dairy cows and the consequent concentrations of EL in the blood, ruminal fluid, urine and milk.
Materials and methods
Animals and experimental treatments
A total of six multiparous lactating Holstein cows fitted with ruminal cannulas (10 cm; Bar Diamond, Inc.) were used in a 6 × 6 Latin square design with six 21 d periods balanced for residual effect and a 2 × 3 factorial arrangement of treatments. The cows averaged 95 (se 20) d in milk at the start of the experiment with an average body weight of 650 (se 36) kg and an average body condition score of 3·00 (se 0·15; five-point scale)(Reference Edmonson, Lean and Weaver14). No antibiotics were given for at least 16 weeks before initiation of the experiment. The cows were kept in individual stalls and had free access to water. The cows were milked twice per d at 08.30 and 20.00 hours. All cows were fed for ad libitum intake (10 % refusals on an as-fed basis) twice per d (08.30 and 14.30 hours). The diets (Table 1) were formulated to meet requirements for cows that were 665 kg body weight and producing 35 kg milk/d with 3·8 % fat(Reference NRC15). Cows were weighed at the beginning and end of each experimental period. The national guidelines for the care and use of animals were followed as recommended by the Canadian Council on Animal Care(Reference Offert, Cross and McWilliam16) and all experimental procedures were approved by the local Animal Care Committee.
Table 1 Ingredients and chemical composition of the total mixed diets
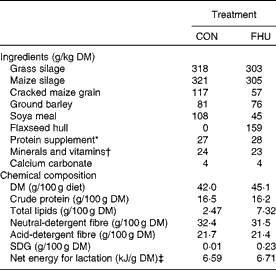
CON, cows fed no flax hulls; FHU, cows supplemented with 15·9 % flax hulls in the DM; SDG, secoisolariciresinol diglucoside.
* Contained 20 % of rapeseed meal, 30 % of maize gluten meal, 20 % of soyabean meal and 30 % of brewer's maize.
† The premix contained (per kg diet DM): 2·3 g Ca; 1·2 g P; 1·2 g Mg; 0·4 g S; 3·5 g Na; 0·4 g K; 51·7 mg Fe; 68 mg Zn; 11·2 mg Cu; 45·4 mg Mn; 1·7 mg I; 0·2 mg Co; 0·5 mg Se; 11 300 IU vitamin A (118·4 μmol/l); 1450 IU vitamin D3 (90 480 nmol/l); 67 300 IU vitamin E (1 048 796 μmol/l).
‡ Calculated using published values of feed ingredients (NRC, 2001).
The six experimental treatments were as follows: (1) no flax hull supplementation in the diet and no flax oil infused in the abomasum (CON-0); (2) no flax hull supplementation and 250 g flax oil/d infused in the abomasum (CON-250); (3) no flax hull supplementation and 500 g flax oil/d infused in the abomasum (CON-500); (4) 15·9 % flax hulls in the DM and no flax oil infused in the abomasum (FHU-0); (5) 15·9 % flax hulls in the DM and 250 g flax oil/d infused in the abomasum (FHU-250); (6) 15·9 % flax hulls in the DM and 500 g flax oil/d infused in the abomasum (FHU-500). Flax oil (Brenntag Canada, Inc.) contained the following (expressed as a percentage of total fatty acids): 16 : 0, 5·3 %; 18 : 0, 3·8 %; cis-9–18 : 1, 18·4 %; cis-11–18 : 1, 0·8 %; cis-9, cis-12–18 : 2, 15·8 %; cis-9, cis-12, cis-15–18 : 3, 52·7 %; others, 3·2 %. Flax hulls (Natunola Health, Inc.) contained the following (expressed as a percentage of DM): crude protein, 23·5 %; neutral-detergent fibre, 19·4 %; acid-detergent fibre, 14·3 %; total lipids, 29·8 %; SDG, 0·99 %; net energy for lactation, 6·86 kJ/g DM.
During the first 7 d of each 21 d period, only 30 % of the experimental dose of oil was infused in the abomasum over a 7 h period. From day 8 to day 21, infusion in the abomasum was conducted with 100 % of the experimental dose of oil over a 23 h period. Samples of the total mixed diets and flax hulls were taken daily from day 16 to day 21 and pooled within period. All samples were frozen at − 20°C for subsequent drying at 55°C and analysed according to the procedures used by Côrtes et al. (Reference Côrtes, da Silva-Kazama and Kazama17). The appropriate amount of oil used for infusion was prepared daily for each cow and was weighed into tared bottles. To perform abomasal infusions, an infusion line was inserted through the rumen cannula and the sulcus omasi into the abomasum as described by Gressley et al. (Reference Gressley, Reynal and Colmenero18). Placement of the infusion lines was monitored daily to ensure post-ruminal delivery. Oil was pumped into the abomasum by using peristaltic pumps (Masterflex L/S; Cole-Parmer Canada, Inc.).
Sampling
Feed intake and milk yield were measured daily. Milk samples were taken twice daily from day 16 to day 21, pooled on a 6 d basis proportionally to the corresponding milk yield, and frozen at − 20°C for EL analysis. On day 20, blood was withdrawn into K3EDTA-vacutainer tubes (Becton Dickinson and Cie) from the jugular vein 6 h after the morning meal. Plasma samples were kept frozen at − 20°C until EL analysis. On day 21, a sample of urine was taken 2 h after the morning meal by hand stimulation of the perineal region and kept frozen at − 20°C for EL analysis. Also, ruminal contents were collected 0, 2, 4 and 6 h after the morning meal from different locations within the rumen (the anterior dorsal, anterior ventral, medium ventral, posterior dorsal and posterior ventral locations) to obtain a representative sample. Ruminal pH was monitored immediately after sample collection with a portable pH meter (Oakton; Eutech Instruments Pte Limited). The ruminal contents were then strained through four layers of cheesecloth. A 350 ml sample of strained ruminal fluid was mixed with rumen particles (26 g) and frozen at − 20°C until assay for β-glucuronidase activity. Then, one portion of filtered ruminal fluid was acidified to pH 2 with 50 % H2SO4 and frozen at − 20°C for the later determination of volatile fatty acids (VFA) and NH3-N concentrations. Concentrations of NH3 and VFA in ruminal fluid were analysed, respectively, with the indophenol blue method and a HPLC Waters Alliance 2695 system (Waters) fitted with a flame ionisation detector. Another portion of ruminal fluid was kept at − 20°C and freeze-dried for the analysis of EL. Ruminal samples for the three post-feeding times (2, 4 and 6 h) were pooled within cow and period, as previously carried out by Gagnon et al. (Reference Gagnon, Côrtes and Da Silva8), to obtain only one composite sample for EL analysis. Moreover, faecal grab samples (250 g) were collected directly from the rectum 2, 4, 6 and 8 h post-feeding on the same day (day 21). Faecal pH was monitored immediately after sample collection. Faecal samples were pooled and kept frozen at − 20°C for further β-glucuronidase analysis.
Lignan analysis and β-glucuronidase activity
The analysis of SDG in the diets was performed according to the procedures described by Muir & Westcott(Reference Muir and Westcott19). Lignans in ruminal fluid, plasma, urine and milk samples were hydrolysed, extracted and analysed as described by Gagnon et al. (Reference Gagnon, Côrtes and Da Silva8). The determination of β-glucuronidase activity was based on a modified method of Jenab & Thompson(Reference Jenab and Thompson13). Briefly, faecal samples (5 g) were homogenised with a Polytron (Kinematica AG) in a final volume of 20 ml of cold KH2PO4 (pH 6·8) for 15 s, while ruminal samples were homogenised using a stomacher set at normal for 30 s. (A. J. Seward & Company Limited). Samples were then filtered through two layers of cheesecloth, sonicated on ice (two bursts, 1 min; Sonics and Materials, Inc.) and centrifuged at 10 000 g for 15 min at 4°C. The supernatant fraction was stored at − 80°C until the enzyme assay as described by Gagnon et al. (Reference Gagnon, Côrtes and Da Silva8).
Statistical analysis
All data were analysed using the MIXED procedure of SAS (2000; SAS Institute) according to a 6 × 6 Latin square with the following model:

where Y ijk is the response variable; μ is the overall mean; a i is the random effect of cow i; βj is the effect of period j; τk is the global effect of treatment combination k; and e ijk is the residual error. Treatments were compared by contrasts in order to test the main effect of flax hulls and to test the polynomial effects (linear and quadratic) of oil in each level of flax hulls. Data on EL concentrations were log-transformed as previously done by Nesbitt et al. (Reference Nesbitt, Lam and Thompson20), but the results in Table 3 present the adjusted mean values (with CI) back-transformed on the original scale of measurements. The model for specific β-glucuronidase activity and pH was augmented with time and time × treatment interaction for repeated measurements and values reported are adjusted means with their standard errors. Data on ruminal fermentation characteristics (VFA, NH3-N and pH) were also analysed as repeated measurements and the compound symmetry was used as the covariance structure. The two-sided level of significance was set at P< 0·05, although probability values up to P< 0·10, suggesting a trend, are shown in the text. However, one cow was removed from the experiment due to udder damage that resulted in acute mastitis. As a result, data from treatments CON-0 and CON-500, which corresponded to the two last periods, were missing for the statistical analysis.
Results
Oil supplementation and DM intake
The actual amounts of oil infused in the abomasum averaged, respectively, 257 and 509 g/d for cows that were planned to receive 250 and 500 g/d. There was a trend (P= 0·06) for an interaction between flax hulls and flax oil for DM intake expressed in kg/d, and the interaction was significant (P= 0·04) when DM intake was expressed as a percentage of body weight. There were linear (P< 0·0001) and quadratic (P= 0·01) effects of abomasal infusion of oil on DM intake as a result of a decrease from 0 to 250 g flax oil while a plateau was reached when infusing 250 or 500 g flax oil (Table 2). However, the decrease in DM intake when no hulls were supplemented in the diet was greater than when the diets were supplemented with flax hulls.
Table 2 Total input of DM (intake of DM+flax oil infused in the abomasum), intake of DM and ruminal fermentation characteristics of Holstein cows fed no flax hulls (CON) or supplemented with 15·9 % flax hulls in the DM (FHU) and infused with three different amounts of flax oil in the abomasum (0, 250 and 500 g/d) (Adjusted mean values with their standard errors)
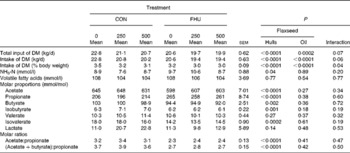
Ruminal fermentation characteristics
There were interactions between sampling time and flax hull supplementation for NH3-N concentration (P= 0·001), molar proportions of acetate (P= 0·001), propionate (P< 0·0001), valerate (P= 0·009) and isovalerate (P= 0·005), the acetate: propionate ratio (P< 0·0001) and the (acetate+butyrate): propionate ratio (P< 0·0001) in the rumen. Moreover, the interaction tended (P= 0·08) to be significant for molar proportions of butyrate and isobutyrate (data not shown). However, there was no interaction (P>0·10) between flax hull supplementation and flax oil infusion. NH3-N concentration in the rumen was higher (P= 0·04) for cows receiving flax hulls (Table 2). The concentration of total VFA in the rumen was not different among the treatments. Cows supplemented with flax hulls had a higher proportion of propionate and lower proportions of acetate, butyrate, isobutyrate and isovalerate in the rumen than those receiving the control diet (Table 2). Molar proportions of valerate and lactate in the rumen were similar for the CON and FHU treatments. The acetate:propionate ratio and the (acetate+butyrate): propionate ratio in the rumen were lower for cows fed the FHU diet compared with those fed the CON diet.
Concentration of enterolactone in ruminal fluid, plasma, milk and urine
There was no significant interaction (P>0·10) between hulls and oil for concentrations of EL in ruminal fluid, plasma, milk and urine. Concentrations of EL in ruminal fluid before feeding and post-feeding, plasma, urine and milk were higher (P< 0·0001) for cows fed flax hulls than for those fed the control diets (Table 3). Flax oil infusion had no effect on the EL concentration of ruminal fluid, plasma, urine and milk.
Table 3 Concentration of enterolactone (μmol/l) in biological fluids of Holstein cows fed no flax hulls (CON) or supplemented with 15·9 % flax hulls in the DM (FHU) and infused with three different amounts of flax oil in the abomasum (0, 250 and 500 g/d)* (Adjusted mean values and 95 % confidence intervals on the original scale of measurement)
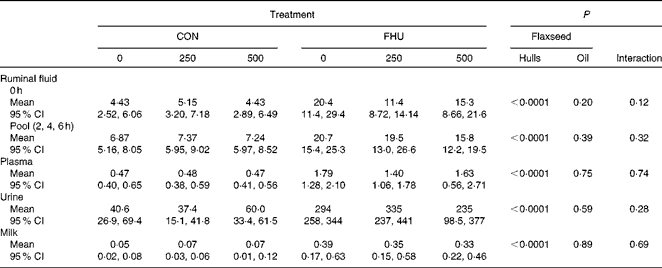
* Data on enterolactone concentrations were log-transformed for statistical analysis.
Activity of β-glucuronidase in ruminal fluid and faeces
There was no interaction (P= 0·91) between time and treatment for β-glucuronidase activity in ruminal fluid, although there was an interaction (P= 0·03) for β-glucuronidase activity in the faeces (data not shown). The mean values for the 6 h faecal and ruminal sampling periods are presented in Table 4. On average, cows fed the FHU diet tended (P= 0·06) to have higher faecal β-glucuronidase activity than those fed the CON diet, and flax oil infusion had no effect. There was a significant interaction between hulls and oil for β-glucuronidase activity in ruminal fluid before and post-feeding (P= 0·01 and 0·04, respectively). There was a linear decrease (P= 0·01) in ruminal β-glucuronidase activity before feeding with a higher amount of oil infused in the abomasum when cows were fed the CON diets, while there was a linear increase (P= 0·02) when cows were fed the FHU diets. Similarly, post-feeding, β-glucuronidase activity in ruminal fluid decreased linearly (P= 0·04) with increased abomasal infusion of oil for cows fed the CON diets, while the activity only tended (P= 0·09) to increase for cows fed the FHU diets.
Table 4 Activity of β-glucuronidase (nmol phenolphthalein/min per mg protein) in the faeces and ruminal fluid of Holstein cows fed no flax hulls (CON) or supplemented with 15·9 % flax hulls in the DM (FHU) and infused with three different amounts of flax oil in the abomasum (0, 250 and 500 g/d) (Adjusted mean values with their standard errors)
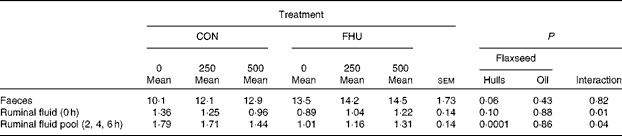
Ruminal fluid pH
There was no significant interaction (P= 0·43) between time and treatment for ruminal fluid pH. Ruminal pH of cows fed flax hulls was lower (P< 0·01) than that of cows fed the control diets, and abomasal infusion of flax oil had no effect.
Faecal pH
There was an interaction between sampling time and supplementation with flax hulls (P= 0·04) and between sampling time and oil (P= 0·09) for faecal pH. Faecal pH was higher 2 h post-feeding for cows fed flax hulls than for those fed the control diets (Fig. 1(a)), and faecal pH decreased linearly from 2 to 4 h post-feeding with a greater amount of oil infused in the abomasum of cows (Fig. 1(b)). However, there was no linear or quadratic effect of oil on the average value of faecal pH when cows were fed the CON or FHU diets.
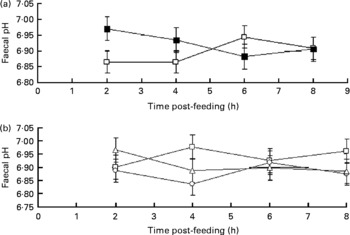
Fig. 1 Post-feeding pH in the faeces (a) of dairy cows supplemented with flax hulls and flax oil. For (a), the treatments were as follows: (1) no flax hull supplementation in the diet (, CON); (2) 15·9 % flax hulls in the DM (
, FHU). For (b), the treatments were as follows: (1) no flax oil infused in the abomasum (
, 0); (2) 250 g flax oil/d infused in the abomasum (
, 250); (3) 500 g flax oil/d infused in the abomasum (
, 500). Values are adjusted mean values with their standard errors represented by vertical bars. The effect of flax hull supplementation on ruminal pH was significant (P< 0·01) and there was an interaction between time and oil (P= 0·04) and time and hull (P= 0·08) for faecal pH.
Discussion
In human subjects, several studies have reported a positive relationship between the intake of flax lignans and the excretion of mammalian lignans in the urine and blood. In ruminants, our recent in vivo results(Reference Gagnon, Côrtes and Da Silva8) have shown that the ruminal microbiome plays an important role in the metabolism of flax lignans as shown by higher concentrations of EL in the urine, plasma and milk of cows supplemented with flax hulls in the rumen when compared with cows administered with flax hulls in the abomasum. Other feed ingredients such as forages and cereals also contain lignans(Reference Steinshamn, Purup and Thuen21, Reference Peñalvo, Haajanen and Botting22), which may lead to the production of EL and explain the presence of EL in the ruminal fluid of dairy cows fed no flax hulls. To our knowledge, this is the first in vivo study investigating the effect of rumen bypass of PUFA on the metabolism and absorption of the mammalian lignan EL and its concentration in physiological fluids of dairy cows.
The highest DM intake observed for cows fed the CON-0 diet may be the result of a lower intake of fat compared with those fed the other five diets. Abomasal infusion of unsaturated long-chain fatty acids has been previously shown to decrease the DM intake of dairy cows(Reference Benson, Reynolds and Humphries23, Reference Litherland, Thire and Beaulieu24). According to Benson et al. (Reference Benson, Reynolds and Humphries23), the suppressive effects of unsaturated long-chain fatty acids may be mediated by the release of gut peptides, in particular glucagon-like peptide 1, in response to increased quantities of unsaturated fatty acids reaching the proximal small intestine. The absence of flax hull supplementation resulted in a lower consumption of plant lignans and intakes of SDG averaged, respectively, 2·28, 2·08, 2·02, 47·38, 44·62 and 44·62 g/d for cows fed the CON-0, CON-250, CON-500, FH-0, FHU-250 and FHU-500 diets. As previous results have shown that the rumen is the main site for the conversion of plant lignans into mammalian lignans(Reference Gagnon, Côrtes and Da Silva8), greater intake of plant SDG for cows fed the flax hull-based diets will probably increase the concentration of EL in the rumen, as observed in the present experiment. Similarly, Zhou et al. (Reference Zhou, Wang and Han25) reported increased ruminal EL concentrations and decreased pH when the pure lignan SDG was infused in the rumen of goats. In the present experiment, ruminal fluid pH also decreased with flax hull supplementation, as previously reported by Gagnon et al. (Reference Gagnon, Côrtes and Da Silva8). Flax hulls are a rich source of fermentable carbohydrates, which may contribute to lower pH and increase the proportion of propionate in the rumen of cows supplemented with flax hulls due to greater availability of carbohydrates in the rumen(Reference Slavin26). Moreover, diets based on flax hulls may contribute to increasing the concentration of NH3-N in the rumen, as previously reported for beef cattle fed increasing levels of whole flaxseed(Reference Mustafa, Gonthier and Ouellet27) as a result of high crude protein degradability (67·3 %) of flaxseed in the rumen(Reference Mustafa, Gonthier and Ouellet28).
Cows fed flax hulls (diets FHU-0, FHU-250 and FHU-500) had concentrations of EL that were on average (mean of the three flax hull treatments) 3·4, 6·7 and 5·8 times higher in the plasma, urine, and milk, respectively. This agrees with the results of Petit & Gagnon(Reference Petit and Gagnon29) who reported a linear increase in milk EL concentration when dairy cows were fed from 0 to 15 % flaxseed meal in the diet. Moreover, other results(Reference Gagnon, Côrtes and Da Silva8) have shown that administration of 1800 g flax hulls/d in the rumen compared with the abomasum significantly increases the concentrations of EL in the plasma, urine and milk.
In the present study, SDG metabolism was not affected by the presence of PUFA in the small intestine, as indicated by similar concentrations of EL in the plasma, urine and milk of cows infused or not with flax oil in the abomasum. A study has shown that a high intake of fat was significantly associated with lower concentrations of EL in the plasma of women(Reference Johnsen, Hausner and Olsen30). In rats, fat has been shown to reduce the absorption and recirculation of lignans(Reference Bylund, Zhang and Bergh31), which may suggest that fat reduces the capacity of the colonic microflora to convert plant lignans into mammalian lignans in single-stomached animals. Therefore, it seems likely that the main site of conversion of the plant lignan SDG into the mammalian lignan EL is the rumen, as previously reported by Gagnon et al. (Reference Gagnon, Côrtes and Da Silva8). Moreover, the results of the present study may indicate that the presence of PUFA in the small intestine does not interfere with the absorption of the mammalian lignan EL in ruminant animals. However, further investigations on the metabolism of mammalian lignans along the gastrointestinal tract of cattle are required to confirm the site of absorption of EL to improve the understanding of its metabolic pathway in dairy cows, in order to enable targeted manipulation of its quantity in milk.
Although Jenab & Thompson(Reference Jenab and Thompson13) observed a positive correlation between SDG dietary concentration and microbial β-glucuronidase activity with colonic microbiota from rats, the opposite was observed in the present experiment for β-glucuronidase activity in the ruminal fluid. Indeed, supplementation with flax hulls resulted in lower post-feeding microbial β-glucuronidase activity in cows fed the 0 and 250 oil treatments compared with cows fed the same treatments but unsupplemented with flax oil. In goats, Zhou et al. (Reference Zhou, Wang and Han25) suggested that SDG supplementation stimulates the growth of Ruminococcus gnavus, which plays a role in glucuronidase activity of the rumen. However, as flax hulls are rich in fat and n-3 fatty acids, this may indicate a negative effect of fatty acids on β-glucuronidase activity in the rumen.
In the present experiment, supplementation with flax hulls tended to increase faecal β-glucuronidase activity, which agrees with the positive correlation between SDG dietary concentration and microbial β-glucuronidase activity with colonic microbiota from rats(Reference Jenab and Thompson13). This result suggests that microbial β-glucuronidase activity in the colon may contribute to the absorption of mammalian lignans in ruminants as previously reported in human subjects(Reference Jenab and Thompson13). Gagnon et al. (Reference Gagnon, Côrtes and Da Silva8) have reported that flax hulls introduced directly in the rumen had no effect on faecal β-glucuronidase activity. Discrepancies between experiments may be explained by the site of supplementation as hulls were administered in the rumen in the experiment of Gagnon et al. (Reference Gagnon, Côrtes and Da Silva8) while hulls were added to the diets in the present experiment. Intake of hulls by cows may result in greater breakdown of seed coat than administration in the rumen due to chewing, which in turn may result in a greater release of plant lignans from seeds.
Lower faecal pH for cows not supplemented with flax hulls may be the result of greater amounts of starch reaching the colon and being fermented by intestinal microflora, which probably results from the production of short-chain VFA that lower colonic pH and serve as an energy source for the colonocytes(Reference Slavin26). Indeed, the control diets contained greater amounts of cereals than the FHU treatments (198 v. 133 g/kg DM) and previous results have shown that fermentation of starch in the hindgut decreases faecal pH(Reference Gressley, Hall and Armentano32).
A study has shown a decrease in the incidence of CVD with greater blood concentration of EL in human subjects(Reference Vanharanta, Voutilainen and Lakka33). Moreover, our recent results have demonstrated that antioxidants such as flax hulls tended (P= 0·09) to increase the activity of superoxide dismutase in the mammary gland(Reference Côrtes, Palin and Gagnon34). Greater activity of superoxide dismutase, which is the first enzyme involved in the conversion of oxygen radicals to peroxides(Reference Yu35), may then contribute to improve the health of the mammary gland and milk quality. Other results have shown(Reference Rajesha, Murthy and Kumar36) in rat models that flax lignans up-regulate the expression of hepatic genes encoding for enzymes such as superoxide dismutase, catalase and glutathione peroxidase that are involved in defence mechanisms against oxidative stress. Altogether, these results have suggested beneficial effects of flax hulls on the health of human subjects and animals. However, further investigations are required to better understand the impact of increasing mammalian lignans in biological fluids on the productivity and health of dairy cows.
In conclusion, the presence of n-3 fatty acids such as flax oil in the small intestine does not interfere with the absorption of the mammalian lignan EL in ruminant animals. Moreover, the results suggest a potential role of colonic microbiota in the absorption of mammalian lignans, although further investigations on the metabolism of mammalian lignans along the gastrointestinal tract of cattle are required to better improve our knowledge on the metabolism of plant lignans in order to increase the concentration of the mammalian lignan EL in the milk of dairy cows.
Acknowledgements
The present study was funded by the Agriculture and Agri-Food Canada. The authors would like to express their gratitude to the staff of the Dairy and Swine Research and Development Centre for their contribution to the present study. We especially want to thank Véronique Roy, Liette Veilleux and Sylvie Dallaire for their technical assistance and Steve Méthot for his help in the statistical analyses. R. K. and D. d. S.-K. were recipients of a studentship and G. d. S. and L. M. Z. were recipients of a fellowship from Conselho Nacional de Desenvolvimento Científico e Tecnológico and Fundação Araucária do Paraná. C. C. was a recipient of a fellowship from the National Science and Engineering Research Council of Canada. C. C. and H. V. P. drafted the manuscript. H. V. P. conceived and directed the study and C. C. coordinated the study. C. C. and R. K. were in charge of the infusions and of collecting the data from the animals. D. d. S.-K. and N. G. were in charge of performing the laboratory work. G. d. S. and L. M. Z. participated in the design of the study. C. B. contributed to the conception and design of the experiment and to the interpretation and discussion of the results. All authors were involved in revising the paper critically and approved the final version of the manuscript. H. V. P. supervised the work of R. K. and D. d. S.-K. in Canada. None of the authors had a personal or professional conflict of interest.