INTRODUCTION
The glacial footprint in the Mediterranean region is restricted to mountain ranges, where later ones overprint earlier glacial cycles. The most prominent glaciation in the Mediterranean region was before 350 ka (Giraudi and Giaccio, Reference Giraudi, Giaccio, Hughes and Woodward2017), conditioning the sediment storage of rivers (Woodward et al., Reference Woodward, Hamlin, Macklin, Hughes and Lewin2008). A glacial cycle is indicated in between the Marine Oxygen Isotope Stage (MIS) 7 interglacial and MIS 12 (Woodward et al., Reference Woodward, Hamlin, Macklin, Hughes and Lewin2008; Hughes et al., Reference Hughes, Woodward, van Calsteren and Thomas2011), matching with the oldest dated outwash deposits on both sides of the Pyrenean mountain belt (Lewis et al., Reference Lewis, Mcdonald, Sancho, Peña Monné and Rhodes2009; Delmas et al., Reference Delmas, Braucher, Gunnell, Guillou, Calvet and Bourlès2015), which are attributed to MIS 8. However, evidence of old glaciations in Iberia is sparse (Turu and Peña-Monné, Reference Turu, Peña-Monné, Pérez-Alberti and López-Bedoya2006; Lewis et al., Reference Lewis, Mcdonald, Sancho, Peña Monné and Rhodes2009; Jalut et al., Reference Jalut, Turu, Dedoubat, Otto, Ezquerra, Fontugne and Belet2010; García-Ruiz et al., Reference García-Ruiz, Martí-Bono, Peña-Monné, Sancho, Rhodes, Valero-Garcés, González-Sampériz and Moreno2013; Villa et al., Reference Villa, Stoll, Farias, Adrados, Edwards and Cheng2013; Delmas et al., Reference Delmas, Gunnell, Calvet, Reixach, Oliva, Palacios, Hughes, García-Ruiz and Andres2021a). Nevertheless, the penultimate glaciation (MIS 6) has been identified in most of the Iberian ranges (Vidal-Romaní et al., Reference Vidal-Romaní, Fernández-Mosquera, Marti and de Brum Ferreira1999, Reference Vidal-Romaní, Fernández-Mosquera and Martin2015; Turu and Peña-Monné, Reference Turu, Peña-Monné, Pérez-Alberti and López-Bedoya2006; García-Ruiz et al., Reference García-Ruiz, Martí-Bono, Peña-Monné, Sancho, Rhodes, Valero-Garcés, González-Sampériz and Moreno2013; Rodríguez-Rodríguez et al., Reference Rodríguez-Rodríguez, Jiménez Sánchez, Domínguez-Cuesta, Rinterknecht, Pallas and Bourlès2016; Vieira et al., Reference Vieira, Palacios, Andrés, Mora, Vázquez-Selem, Woronko, Soncco, Úbeda and Goyanes2021; Fernandes et al., Reference Fernandes, Oliva, Vieira, Palacios, Fernández-Fernández, Delmas, García-Oteyza, Schimmelpfennig and Ventura2021) and coincided with a significant episode of fluvial aggradation (Turu and Peña-Monné, Reference Turu, Peña-Monné, Pérez-Alberti and López-Bedoya2006; Lewis et al., Reference Lewis, Mcdonald, Sancho, Peña Monné and Rhodes2009; Stange et al., Reference Stange, Van Balen, Carcaillet and Vandenberghe2013, Reference Stange, Van Balen, Kasse, Vandenberghe and Carcaillet2014; Delmas et al., Reference Delmas, Braucher, Gunnell, Guillou, Calvet and Bourlès2015).
Regarding the last glacial cycle (LGC), glacial expansion started as early as MIS 5d in the Mediterranean region (Hughes et al., Reference Hughes, Woodward, van Calsteren, Thomas and Adamson2010). In Iberia, the local last glacial maximum would be between 23 and 17.5 ka (Roucoux et al., Reference Roucoux, De Abreu, Shackleton and Tzedakis2005; Oliva et al., Reference Oliva, Palacios, Fernández-Fernández, Rodríguez-Rodríguez, García-Ruíz, Andrés and Carrasco2019). However, in the Pyrenees, the last maximum ice extent (LMIE) predates the last glacial maximum (LGM) of the northern ice sheets (Mardones and Jalut, Reference Mardones and Jalut1983; Vilaplana, Reference Vilaplana1983a; Jalut et al., Reference Jalut, Montserrat-Martí, Fontugne, Delibrias, Vilaplana and Julià1992, Reference Jalut, Turu, Dedoubat, Otto, Ezquerra, Fontugne and Belet2010; Delmas et al., Reference Delmas, Gunnell, Calvet, Reixach, Oliva, Palacios, Hughes, García-Ruiz and Andres2021a and references therein). Based on rapid cooling and precipitation levels similar to those of MIS 2, it has been suggested that the LMIE probably coincided with MIS 4 (Clapperton, Reference Clapperton1997; Doughty et al., Reference Doughty, Kaplan, Peltier and Barker2021). In addition, there is fragmentary evidence of an earlier maximum ice extent (MIE) (Bakalowicz et al., Reference Bakalowicz, Sorriaux and Ford1984; Hetu et al., Reference Hetu, Gangloff and Courchesne1992; Turu and Peña-Monné, Reference Turu, Peña-Monné, Pérez-Alberti and López-Bedoya2006; Turu et al., Reference Turu, Boulton, Ros, Peña Monné, Martí Bono, Bordonau and Serrano2007; Ventura and Turu, Reference Ventura, Turu, Oliva, Palacios and Fernández-Fernández2022). At the end of MIS 4, ice recession matched with the increase of summer daily insolation at 65°N until interstadial GI-17 (Martrat et al., Reference Martrat, Grimalt, López-Martínez, Cacho, Sierro, Flores, Zahn, Canals, Curtis and Hodell2004). During Heinrich event H6 (61–59 ka), steppe pollen increased, suggesting an arid climate (Roucoux et al., Reference Roucoux, De Abreu, Shackleton and Tzedakis2005). However, insolation slightly diminished until interstadial GI-7 (≈35 ka) is more pronounced, declining until stadial GS-3 (≈27 ka), corresponding with a phase of significant glacial readvances in the Pyrenees (Turu, Reference Turu and Aliello2018). To gain a better understanding of the palaeoenvironmental conditions of the LGC, causes of the LMIE/LGM dephasing and the chronological position of the MIE, some authors have investigated relevant glaciolacustrine sequences in the Pyrenees (Jalut et al., Reference Jalut, Montserrat-Martí, Fontugne, Delibrias, Vilaplana and Julià1992, Reference Jalut, Turu, Dedoubat, Otto, Ezquerra, Fontugne and Belet2010; González-Sampériz et al., Reference González-Sampériz, Valero-Garcés, Moreno, Jalut, García-Ruiz, Martí-Bono, Delgado-Huertas, Navas, Otto and Dedoubat2006; Turu and Bordonau, Reference Turu, Bordonau, Baena, Fernández and Guerrero2013; Turu et al., Reference Turu, Calvet, Bordonau, Gunnell, Delmas, Vilaplana, Jalut, Hughes and Woodward2017; Sancho et al., Reference Sancho, Arenas, Pardo, Peña-Monné, Rhodes, Bartolomé, García-Ruiz and Martí-Bono2018), sediments from glacially overdeepened valleys (Bordonau, Reference Bordonau1992; Turu, Reference Turu2000; Turu et al., Reference Turu, Boulton, Ros, Peña Monné, Martí Bono, Bordonau and Serrano2007; Salazar-Rincón et al., Reference Salazar-Rincón, Mata, Rico, Valero-Garcés, Oliva-Urcia, Ibarra and Rubio2013), lacustrine foreland sequences (Pérez-Obiol and Julià, Reference Pérez-Obiol and Julià1994; Höbig et al., Reference Höbig, Weber, Kehl, Weniger, Melles, Fülöp, Vogel and Reicherter2012; González-Sampériz et al., Reference González-Sampériz, Aranbarri, Pérez-Sanz, Gil-Romera, Moreno, Leunda and Sevilla-Callejo2017), or fluvial terraces (Peña-Monné et al., Reference Peña-Monné, Calvet, Turu, Turu and Constante2011; Stange et al., Reference Stange, Van Balen, Kasse, Vandenberghe and Carcaillet2014; Delmas et al., Reference Delmas, Braucher, Gunnell, Guillou, Calvet and Bourlès2015) or have focused on lateral glacial (kame-type deposits) and terminal complexes (end moraines and glaciofluvial deposits) (Turu and Peña-Monné, Reference Turu, Peña-Monné, Pérez-Alberti and López-Bedoya2006; Lewis et al., Reference Lewis, Mcdonald, Sancho, Peña Monné and Rhodes2009, Reference Lewis, Sancho, Mcdonald, Peña-Monné, Pueyo, Rhodes, Calle and Soto2017; Pallàs et al., Reference Pallàs, Rodés, Braucher, Bourlès, Delmas, Calvet and Gunnell2010; Delmas et al., Reference Delmas, Calvet, Gunnell, Braucher and Bourlès2011; Bartolomé et al., Reference Bartolomé, Sancho, Benito, Medialdea, Calle, Moreno and Leunda2021; Fernandes et al., Reference Fernandes, Oliva, Vieira, Palacios, Fernández-Fernández, Delmas, García-Oteyza, Schimmelpfennig and Ventura2021; among others).
Asymmetries arise when comparing both sides of the mountain range. On the one hand, we have the example of the SW Pyrenees, in which the MIE is from the penultimate glaciation (Lewis et al., Reference Lewis, Mcdonald, Sancho, Peña Monné and Rhodes2009; García-Ruiz et al., Reference García-Ruiz, Martí-Bono, Peña-Monné, Sancho, Rhodes, Valero-Garcés, González-Sampériz and Moreno2013), but this is not the case in the SE Pyrenees, where glacial extent during the penultimate glacial cycle (PGC) was less than during the LMIE (Turu et al., Reference Turu, Boulton, Ros, Peña Monné, Martí Bono, Bordonau and Serrano2007; Ventura and Turu, Reference Ventura, Turu, Oliva, Palacios and Fernández-Fernández2022). On the other hand, evidence from the LGM is somewhat elusive in the SW Pyrenees (García-Ruíz et al., Reference García-Ruíz, Valero-Garcés, Martí-Bono and González-Sampériz2003, Reference García-Ruiz, Martí-Bono, Peña-Monné, Sancho, Rhodes, Valero-Garcés, González-Sampériz and Moreno2013; Lewis et al., Reference Lewis, Mcdonald, Sancho, Peña Monné and Rhodes2009; Bartolomé et al., Reference Bartolomé, Sancho, Benito, Medialdea, Calle, Moreno and Leunda2021). Aridity (Höbig et al., Reference Höbig, Weber, Kehl, Weniger, Melles, Fülöp, Vogel and Reicherter2012; Allard et al., Reference Allard, Hughes and Woodward2021) and moisture variations (Jalut et al., Reference Jalut, Turu, Dedoubat, Otto, Ezquerra, Fontugne and Belet2010; Delmas et al., Reference Delmas, Braucher, Gunnell, Guillou, Calvet and Bourlès2015; Turu, Reference Turu and Aliello2018) seem to have provided strong gradients between the Atlantic and the Mediterranean and exerted a significant influence on glacial extent across the Pyrenees. A W–E moisture gradient during the Holocene has been demonstrated in Iberia (Liu et al., Reference Liu, Shen, González-Sampériz, Gil-Romera, ter Braak, Prentice and Harrison2021) and during the last termination period in the Iberian Central System mountain range (Turu et al., Reference Turu, Carrasco, Pedraza, Ros, Ruiz-Zapata, Soriano-López and Mur-Cacuho2018, Reference Turu, Carrasco, López-Sáez, Pontevedra-Pombal, Pedraza, Luelmo-Lautenschlaeger and Pérez-Díaz2021). A similar NW–SE gradient is expected to have existed across the Pyrenean range during glaciations.
Before attempting any discussion comparing the existing data identifying glacial cycles or phases in the Pyrenees, some additional chronological data from relevant sites is needed. To do this task, we chose end moraines and terminal complexes within glacial valleys from the south-central and southeastern Pyrenees, especially far-flung moraines (Anderson et al., Reference Anderson, Dühnforth, Colgan and Anderson2012) and nested moraines (Kirkbride and Winkler, Reference Kirkbride and Winkler2012).
GEOMORPHOLOGICAL SETTINGS AND GLACIAL ANTECEDENTS
The Segre River tributary catchments are located on the southern slope of the French–Spanish–Andorran Pyrenees, draining to the Ebro basin and thus to the Mediterranean Sea (Fig. 1). From east to west, the Segre tributaries are the Valira, Noguera Pallaresa, Noguera Ribagorçana, and Cinca Rivers. Most of the southern Pyrenean glacial valleys are U-shaped, flanked by lateral moraines, kame-type deposits, and former ice-dammed valleys. From west to east, the highest peaks in the studied area are the Pic de Margalida (3251 m) in the Noguera Ribagorçana, followed by Pica d'Estats (3143 m) in the Noguera Pallaresa on the France–Spain border, the Comapedrosa (2942 m) in the northwest of Andorra, and the Carlit (2921 m) in the French Cerdagne (Fig. 1). Most of the end-moraine complexes from the southeastern and south-central Pyrenees did not reach the foreland foothills, with only the Valira and Querol palaeoglaciers having reached the intramountain grabens of the Urgellet and Cerdagne, respectively (Calvet, Reference Calvet, Gómez-Ortíz and Pérez-Alberti1998; Turu et al., Reference Turu, Boulton, Ros, Peña Monné, Martí Bono, Bordonau and Serrano2007).
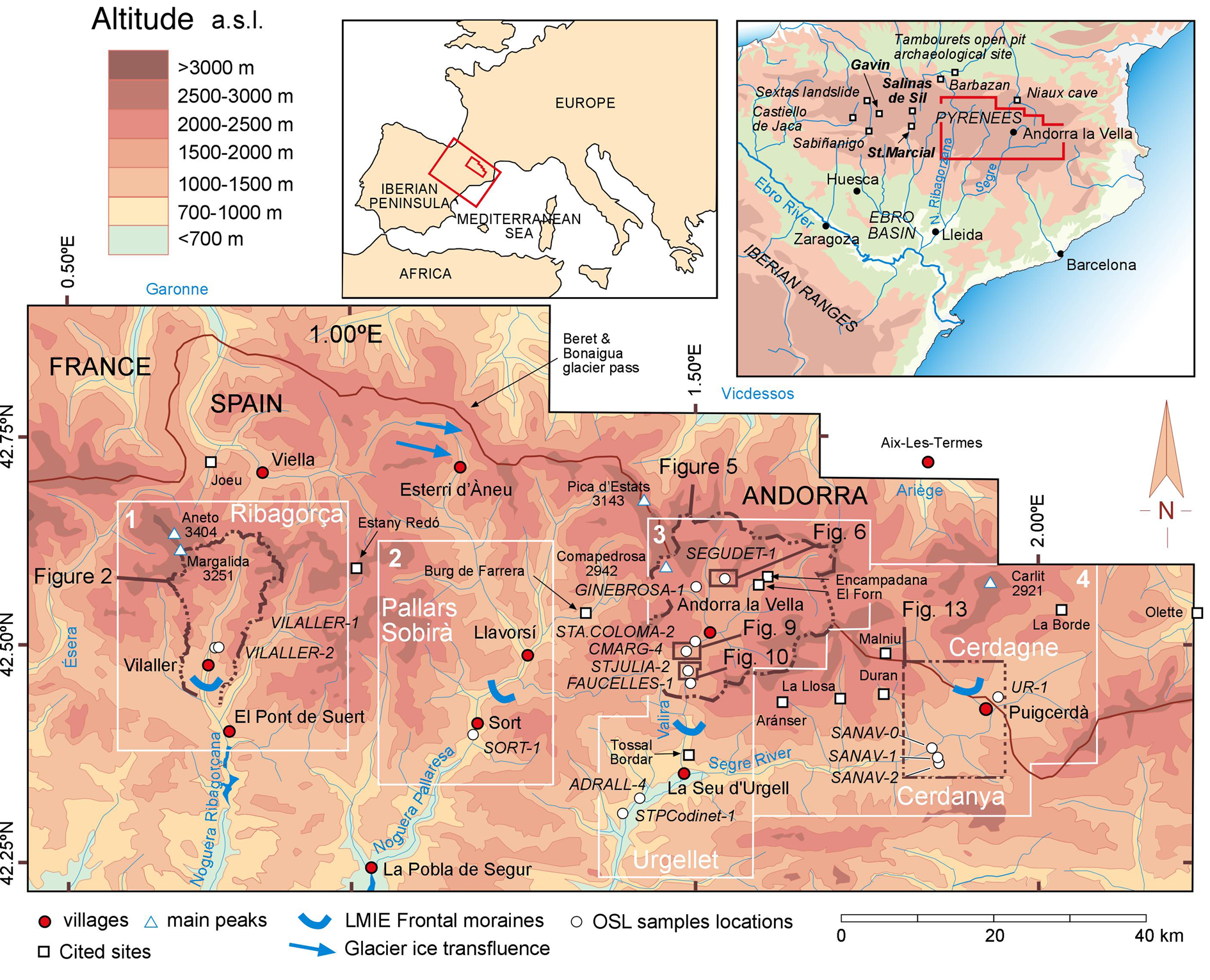
Figure 1. Study area location. Sector 1: Upper Noguera Ribagorzana (Ribagorça region); sector 2: Upper Noguera Pallaresa (Pallars Sobirà region); sector 3: Valira and Segre valleys (Andorra and Urgellet regions); sector 4: Cerdanya (Cerdagne) and Upper Segre valleys. Detailed figures and optically stimulated luminescence (OSL) samples are included in the map. It also contains the glacial ice transfluences and last maximum ice extent (LMIE) frontal moraines.
The Upper Noguera Ribagorçana valley
This valley is located on the westernmost side of the studied area (Fig. 1, sector 1). Geologically speaking, three rock types are present in this valley, distributed following a W–E trend (Mey, Reference Mey1965): late Variscan crystalline rocks (granites) on the northern part of the valley (north from the Baserca reservoir; Fig. 2), Mesozoic sedimentary rocks southward from the confluence of the Baliera River and the Noguera Ribagorçana River (south Vilaller; Fig. 2), and finally, Palaeozoic low-grade metamorphic rocks in between the two previous lithological areas. Geomorphologically speaking, the valley harbours thick lateral moraines and kame deposits well suited to study glacial evolution.
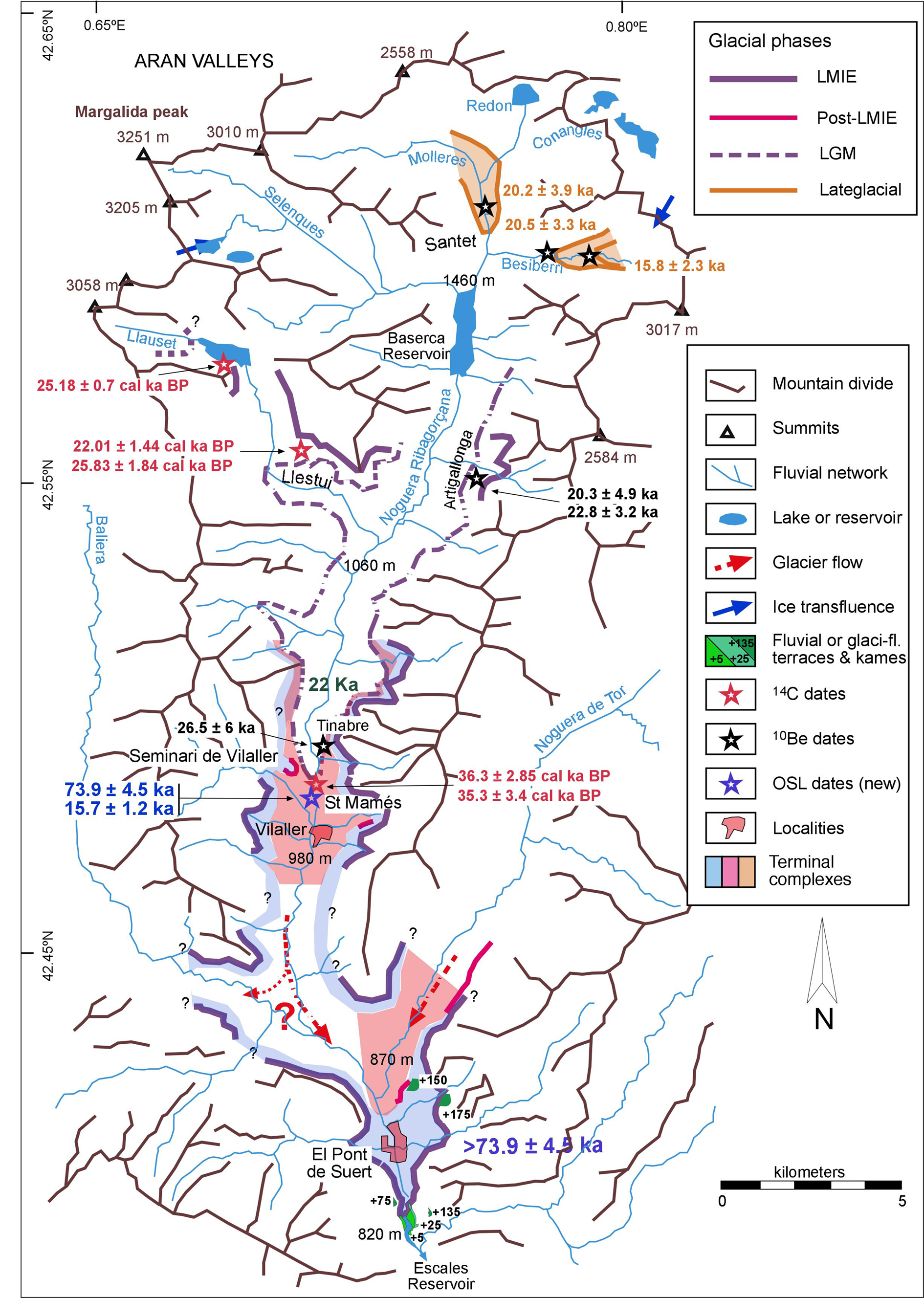
Figure 2. Geomorphological sketch of the upper Ribagorçana valley (reinterpreted from Mey, Reference Mey1965; Vilaplana, Reference Vilaplana1983a). Relevant 10Be samples from Pallàs et al. (Reference Pallàs, Rodés, Braucher, Carcaillet, Ortuno, Bordonau, Bourlès, Vilaplana, Masana and Santanach2006) were recalculated. Bulk carbon samples from Vilaplana (Reference Vilaplana1983a) at Llauset, Vilaplana and Bordonau (Reference Vilaplana and Bordonau1989) at Llestui, and Bordonau (1992) at the Seminari de Vilaller. LMIE, last maximum ice extent; LGM, last glacial maximum; OSL, optically stimulated luminescence.
The upper Noguera Ribagorçana was the first glacial valley from the southern slope of the Pyrenees to be dated, specifically in the Llauset (Fig. 2) glaciolacustrine deposits (Vilaplana, Reference Vilaplana1983a, Reference Vilaplana1983b; Montserrat-Martí, Reference Montserrat-Martí1985) when the lake water level was artificially raised to become the reservoir for a pumped-storage hydroelectric power plant. In the same area, the former Llestui ice-dammed glaciolacustrine complex was the next to be dated (Vilaplana et al., Reference Vilaplana, Montserrat-Martí, Schlüchter, Rose and Schlüchter1989; Vilaplana and Bordonau Reference Vilaplana and Bordonau1989; Bordonau et al., Reference Bordonau, Vilaplana and Fontugne1993) along with the Seminari de Vilaller (Fig. 2) overdeepened trough (Bordonau, Reference Bordonau1992). Half a kilometre northward from Sant Mamés (Fig. 2), a terminal-moraine complex was identified at Seminari de Vilaller (Vilaplana, Reference Vilaplana1983a, Reference Vilaplana1983b; Bordonau et al., Reference Bordonau, Pous, Queralt and Vilaplana1989; Bordonau, Reference Bordonau1992; Pallàs et al., Reference Pallàs, Rodés, Braucher, Carcaillet, Ortuno, Bordonau, Bourlès, Vilaplana, Masana and Santanach2006). Nearby, erratic boulders at Tinabre (Fig. 2) have produced the oldest 10Be cosmic ray exposure (CRE) ages in the valley (Pallas et al., Reference Pallàs, Rodés, Braucher, Carcaillet, Ortuno, Bordonau, Bourlès, Vilaplana, Masana and Santanach2006). No end-moraine exposures south of Vilaller are currently known. However, the remains of glaciofluvial and kame deposits help us to reconstruct the LMIE glacial front in Pont de Suert (Fig. 2).
The upper Noguera Pallaresa valley
Surrounded mainly by low-grade metamorphic rocks (Zandvliet, Reference Zandvliet1960), the former Noguera Pallaresa glacier benefitted from the contribution of the former Garonne glacier coming from the NW side of the valley (the Beret and Bonaigua pass; Fig. 1) and produced the most extensive glacial tongue (63 km) in the southern Pyrenees (Ventura and Turu, Reference Ventura, Turu, Oliva, Palacios and Fernández-Fernández2022). The terminal-moraine complex from the LGC was identified ~2 km upstream from Sort (Fig. 1, sector 2) at 748 m above sea level (m asl; Bastida de Sort; Furdada, Reference Furdada1988; Ventura and Turu, Reference Ventura, Turu, Oliva, Palacios and Fernández-Fernández2022). Unfortunately, no other end moraine has been identified from the Noguera Pallaresa glacier. However, a thick glaciofluvial terrace is located in Sort (Fig. 1).
The Valira valleys
The main Valira glacier (Fig. 1, sector 3) was formed by the confluence of two tributaries, the former Valira del Nord glacier and the Valira d'Orient glacier (Fig. 3). Both tributaries flowed over metamorphic rocks grading from slates to gneiss. However, the floors of these valleys are in granite bedrock (Zwart, Reference Zwart1979). The resulting ice tongue (Fig. 3) extended from Escaldes-Engordany (1050 m) to beyond Sant Julià de Lòria (900 m) (Turu et al., Reference Turu, Boulton, Ros, Peña Monné, Martí Bono, Bordonau and Serrano2007) and formed several terminal-moraine complexes (Cal Tolse, Sant Julià de Lòria, La Margineda, and Santa Coloma; Fig. 3), mainly related to readvances within general deglaciation (Turu et al., Reference Turu, Boulton, Ros, Peña Monné, Martí Bono, Bordonau and Serrano2007, Reference Turu, Calvet, Bordonau, Gunnell, Delmas, Vilaplana, Jalut, Hughes and Woodward2017).
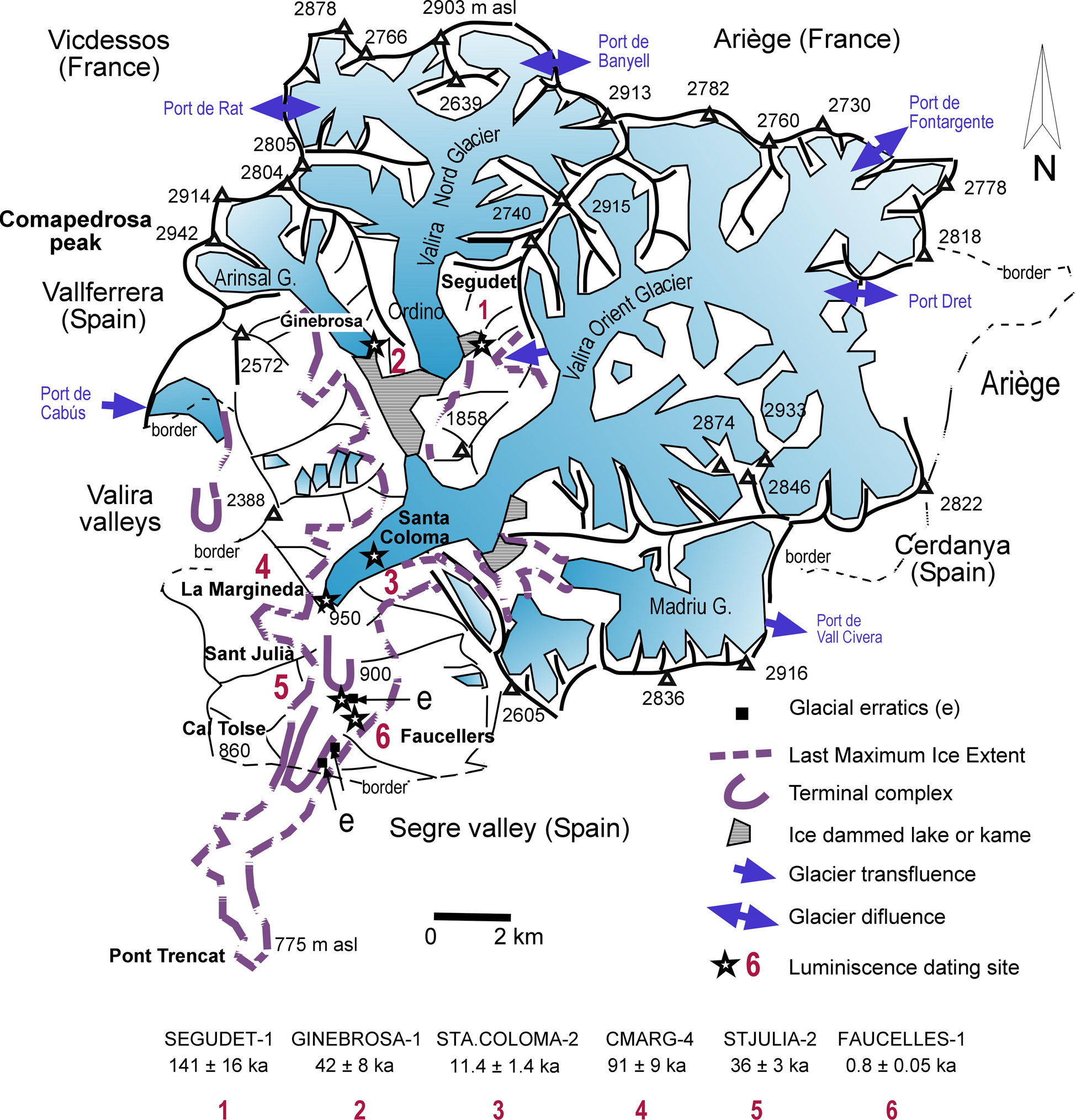
Figure 3. Andorra glacier extension (adapted from Turu et al., Reference Turu, Calvet, Bordonau, Gunnell, Delmas, Vilaplana, Jalut, Hughes and Woodward2017) and sampled site position (Fig. 1, sector 3). The blue colour represents the ice extension. (1) SEGUDET-1; (2) GINEBROSA-1; (3) STA.COLOMA-2; (4) CMARG-4; (5) STJULIA-2; and (6) FAUCELLES-1.
The southernmost deposits are the Cal Tolse terminal-moraine complex (Turu, Reference Turu, Arnáez-Vadillo, García-Ruiz and Gómez-Villar1994), exposed in an ancient quarry located +85 m above the riverbed (m arb), which exploited aggregates from glaciofluvial deposits southward from Sant Julià de Lòria (Fig. 3). This area was exposed by the triggering of a landslide in 2018 (Luzi et al., Reference Luzi, Espín-López, Crosetto, Monserrat, Barra and Gao2021), allowing identification of a supraglacial till that is partially eroded and overlain by glaciofluvial deposits.
The subsequent deposits are in Sant Julià de Lòria (Fig. 3), where Prat (Reference Prat1980) identified erratic boulders on the eastern Valira riverbank. Later, building works allowed a better view of the terminal complex (Jalut and Turu, Reference Jalut, Turu, Canérot, Colin, Platel and Bilotte2008).
Upstream from Sant Julià de Lòria is the La Margineda terminal complex, long established as an end moraine from the Valira glacier (Panzer, Reference Panzer1926; Nussbaum, Reference Nussbaum1934; Llobet, Reference Llobet1947). Here colluvium overlies glaciofluvial deposits located at 905 m (45 m arb; Turu, Reference Turu, Arnáez-Vadillo, García-Ruiz and Gómez-Villar1994); however, only occasional exposures of a supraglacial till have been seen during building excavations. Weathered granite boulders were identified at 980 m and attributed to an old moraine (the Llumaneres moraine from Turu and Peña-Monné, Reference Turu, Peña-Monné, Pérez-Alberti and López-Bedoya2006). Nevertheless, a large outcrop to the south of La Margineda allows the identification of a fresher end moraine.
In Santa Coloma (Fig. 3), an open pit cut into a fluvial terrace +15 m arb by an ancient quarry provided an opportunity for Nussbaum (Reference Nussbaum1956) to recognize a moraine from the Valira glacier when it had a length of 29 km (Chevalier, Reference Chevalier1906, Reference Chevalier1907). Upstream from Santa Coloma many other retreat moraines have been recognized (Bladé, Reference Bladé1875; Penck, Reference Penck1884; Turu et al., Reference Turu, Calvet, Bordonau, Gunnell, Delmas, Vilaplana, Jalut, Hughes and Woodward2017 and references therein), but these were not revisited in this study.
The best-preserved glacial deposits studied in Andorra are from ice-dammed and kame-terrace complexes, as in association with the La Massana palaeolake (Serrat and Vilaplana, Reference Serrat and Vilaplana1979), where glaciolacustrine deposits are common (Serrat et al., Reference Serrat, Vilaplana, Martí-Bono, Everson, Schlüchter and Rabassa1983; Vilaplana, Reference Vilaplana1984, Reference Vilaplana1985; Turu, Reference Turu and Aliello2018 and references therein). We revisited key exposures, such as the Ginebrosa and Segudet kames, to obtain dates. According to Turu and Bordonau (Reference Turu and Bordonau1997), the Segudet and Ginebrosa kames were formed when the Arinsal and Ordino glaciers converged to form a single ice tongue on palaeo-Lake La Massana (1250 m asl) (Turu et al., Reference Turu, Calvet, Bordonau, Gunnell, Delmas, Vilaplana, Jalut, Hughes and Woodward2017).
The upper Segre and Cerdagne
In the upper Segre and Cerdagne basin (Fig. 1, sectors 3 and 4), exposures are found at the confluence of the Segre River with its tributaries (Adrall and Ur sites) or within the Segre River terrace system (Sanavastre).
At Adrall, a fluvial terrace is located near the confluence between the Segre and its tributary, Castellbó, which exclusively drains low-grade metamorphic rocks (Hartevelt, Reference Hartevelt1970). The Adrall terrace is far from any glacial front; however, it belongs to the glaciofluvial Segre–Valira terrace system (+38 m arb) and has been correlated with the Cal Tolse terminal complex (Turu and Peña-Monné, Reference Turu, Peña-Monné, Pérez-Alberti and López-Bedoya2006; Turu et al., Reference Turu, Boulton, Ros, Peña Monné, Martí Bono, Bordonau and Serrano2007; Peña-Monné et al., Reference Peña-Monné, Calvet, Turu, Turu and Constante2011).
The Cerdanya is located in the uppermost part of the Segre basin and drains low-grade metamorphic rocks and granites on its northern border (ICGC, 2006). This area was divided by the treaty of the Pyrenees in 1659, so we use “Cerdagne” for the French part (in the north) and “Cerdanya” for the Spanish zone. Within the latter area, we found the abandoned quarry of Albellorols (1060 m) close to the village of Sanavastre, in which a weathered coarse deposit, some 7-8 m thick, is located at ~20–35 m arb and belongs to the Segre T4 (the Mala Mort level; Calvet, Reference Calvet, Gómez-Ortíz and Pérez-Alberti1998). Terrace T4 overlapped by the Escadarchs fan (Fig. 4) and was fed by limestone-sourced sediments. In Cerdagne, T4 is decalcified by intense weathering (Calvet, Reference Calvet, Gómez-Ortíz and Pérez-Alberti1998), but this is not the case for the same terrace on the southern flank of the Segre River (Poch et al., Reference Poch, Simó and Boixareda2013). The fluvial terrace system continues downstream in the form of two weathered outliers (terrace T3; Fig. 4). Pedogenic geopetal structures, including carbonate nodules and minor clay accumulation, are common in terrace T3 (Poch et al., Reference Poch, Simó and Boixareda2013), with gruss from weathered cobbles of granite; however, rich-iron minerals remain unweathered.

Figure 4. (a) Quaternary facies from the Cerdanya basin after ICGC (2006) geological map, modified for this work. Moraines (M) and associated terraces (T). Location of the samples for optically stimulated luminescence (OSL) dating: (b) sampling for OSL dating at the Abellorols lignite quarry (near Sanavastre) from deposits of a Segre fluvial terrace, sample SANAV-1 from the upper division; (c) sample SANAV-2 from the lower division.
The well-known nested end-moraine complex of the Carol glacier surrounding Puigcerda is found in Cerdagne (Calvet, Reference Calvet1994, Reference Calvet, Gómez-Ortíz and Pérez-Alberti1998; Calvet et al., Reference Calvet, Delmas, Gunnell, Braucher, Bourlès, Ehlers and Gibbard2011b; Pallàs et al., Reference Pallàs, Rodés, Braucher, Bourlès, Delmas, Calvet and Gunnell2010 and references therein; Fig. 4). Upstream of the Carol–Segre confluence is the Ur-Llaurar rest area (Fig. 4) close to the N20 highway, in which we sampled a sandy layer from a thick deposit of glaciofluvial gravels.
MATERIALS AND METHODS
Fieldwork
Fieldwork included detailed geological characterisation of each exposure, consisting of stratigraphic logging and interpretation of sedimentological features. Sample collection (Table 1) from previously cleaned profiles was undertaken at the sites mentioned above (Fig. 1) from coarse glaciofluvial, colluvial, or fine-grained glaciolacustrine sediments. For luminescence dating, we hammered steel tubes into the sections and then extracted them, avoiding any exposure of the contents to daylight. Adjacent to each tube, a subsample of sediment was collected to determine the dose rate at the laboratory.
Table 1. Optically stimulated luminescence (OSL) dating results obtained by the Nordic Laboratory for Luminescence Dating (NLL).a

aAbbreviations: arb, above riverbed; asl, above sea level; BS, bioturbate silts; CMS, coarse-medium sand; CS, coarse sand; FS, fine sand; LFS, laminated fine sand; LS, laminated silts MLS, medium laminated sand; MS, medium sand; VCS, very coarse sand.
Grain-size analysis
The texture of the sediment samples was determined at the Sedimentology Laboratory of the Department of Earth Sciences, University of Coimbra, Portugal (Supplementary Material 1). The grain-size distribution and statistical parameters of each sample were obtained through the integration of two methods: by sieving the >63 μm fraction and by laser diffraction analysis of the <63 μm fraction with a Coulter laser granulometer (2 mm–0.04 μm). The grain-size distribution provides information about the sediments' maturity, transportation history, and deposition. Because particles transported under subaerial conditions are exposed to daylight, near-complete bleaching (zeroing of the optically stimulated luminescence [OSL] signal during transport) is expected, avoiding luminescence-dose inheritance (see Hoey, Reference Hoey, Evans and Benn2004). Sediments suitable for OSL are those of fluvial or aeolian origin, showing skewed, unimodal, well-sorted grain-size distributions and associated with transport histories in which subaerial conditions are met. Because such ideal conditions would not always be represented in a glacial-type or related deposit (Walker, Reference Walker2005), two modern samples from the Segre riverbed were measured to estimate the offset of possible inheritances (Table 1, SANAV-0 and STPCodinet-1).
Water-content estimations
For each sample, we calculated the field water content (w). First, we estimated the void ratio (e), a parameter related to packing the particles within the sediment. This parameter is readily estimated concerning published tables (Jiménez-Salas and de Justo-Alpañes, Reference Jiménez-Salas and de Justo-Alpañes1975). For example, e = 0.35 equates with the densest packing of spherical particles of equal size bearing on top of each other, whereas e = 0.91 corresponds to the weakest packing of spheres. The void ratio and the porosity (n) are related by n = e (1 + e)−1. Because the density of igneous and metamorphic particles forming the framework of unconsolidated sands are known (ρ ≈ 26.5 kN/m3), the dry density (δd) is associated to the porosity (n) related by δd= ρ ⋅ (1 − n), while the density of a fully saturated soil (δs) is: δs = δd + n ⋅ δw, where δw is the density of liquid water. Estimating the water content (w%) at full saturation may also be an estimate of the maximum water content during the burial period, probably below the water table; thus w% = 100 ⋅ (δs − δd) ⋅ δd−1. However, once the deposits have been uplifted or exposed by erosion, the water content will be lower than in fully saturated conditions. The minimum water content corresponds with the highest void ratio because these are inversely related. When the deposits are unsaturated, capillarity action retains moisture at a rate inversely proportional to their void ratio. Void ratio values for the most common deposits are shown in Supplementary Material 2. Knowledge of water content is important, as water absorbs radiation differently from mineral sediment and thus must be accounted for in dose-rate calculations (Walker, Reference Walker2005).
Luminescence dating
In this section, we include both OSL and thermally stimulated luminescence methods. We dated several deposits twice to identify any biases between these methods. Luminescence dating covers those analytical methods that measure the time elapsed since grains of particular minerals (e.g., quartz or feldspars) were last exposed to significant heating or daylight (Duller, Reference Duller2004). The method was developed mainly by using quartz as the dose meter (Qtz-OSL), but K-feldspar can be used when the Qtz-OSL signal is saturated.
OSL dating
Exposure to daylight during sediment transport removes the latent luminescence signal from the quartz or feldspar crystals. After the burial, the luminescence signal (trapped charge) starts to accumulate in the mineral grains due to ionising radiation arising from the decay of 238U, 232Th, and 40K present in the sediment and from cosmic-ray bombardment (Murray et al., Reference Murray, Marten, Johnston and Martin1987). In the laboratory, the equivalent dose (De, assumed to be the dose absorbed since the last light exposure; i.e., the burial dose, expressed in Gy) is determined by comparing the natural luminescence signal resulting from the charge trapped during a burial with that trapped following laboratory irradiation (Olley et al., Reference Olley, Murray and Roberts1996). For the calculation of the dose rate of sand-sized K-feldspar grains, an internal K content of 12.5 ± 0.5% was assumed (Huntley and Baril, Reference Huntley and Baril1997).
Sample preparation for luminescence analyses was undertaken in darkroom conditions. Samples were wet-sieved to separate the 180–250 μm grain-size fraction, followed by HCl (10%) and H2O2 (10%) treatments to remove carbonates and organic matter, respectively. The K-feldspar–rich fraction was floated off using a heavy-liquid solution of sodium polytungstate (density = 2.58 g/cm3). The quartz fraction was obtained by etching another portion with concentrated hydrofluoric acid (HF) (40%). The K-feldspar fraction was treated with 10% HF for 40 minutes to remove the outer alpha-irradiated layer and clean the grains. After etching, the quartz and K-feldspar fractions were treated with hydrochloric acid (HCl) (10%) to dissolve any remaining fluorides. Quartz purity was confirmed by the absence of a significant infrared-stimulated luminescence (IRSL) signal.
Equivalent doses were measured on automated Risø TL/OSL DA-20 readers, each containing a beta source calibrated for irradiation on stainless steel discs and cups. Quartz measurements were made on large (8 mm) aliquots containing several thousands of grains mounted on stainless steel discs. Small (2 mm) aliquots of K-feldspar were mounted on stainless steel cups. Quartz dose estimates were made using a standard single-aliquot regenerative-dose (SAR) protocol using blue-light stimulation at 125°C for 40 s with a 240°C preheat for 10 s, a 200°C cut the heat, and an elevated temperature (280°C) blue light–stimulated clean-out step (Murray and Wintle, Reference Murray and Wintle2000, Reference Murray and Wintle2003). The OSL signal was detected through a U-340 filter. All samples had a strong, fast component. The net OSL signal was calculated from the stimulation's initial 0.0–0.8 s and an early background between 0.8 and 1.6 s.
The K-feldspar De estimates were measured with a post-IR IRSL SAR protocol using a blue-filter combination (Thomsen et al., Reference Thomsen, Murray, Jain and Bøtter-Jensen2008; Buylaert et al., Reference Buylaert, Jai, Murray, Thomsen, Thiel and Sohbati2012). The preheat was 320°C for 60 s, and the cut heat was 310°C for 60 s. After preheating, the aliquots were IR bleached at 50°C for 200 s (IR50 signal) and subsequently stimulated with IR at 290°C for 200 s (pIRIR290 signal). It has been shown by Buylaert et al. (Reference Buylaert, Jai, Murray, Thomsen, Thiel and Sohbati2012) that the post-IR IRSL signal measured at 290°C can give accurate results without the need for correction for signal instability. For all IR50 and pIRIR290 calculations, the initial 2σ of the luminescence decay curve, less a background derived from the last 50 s, was used. Dividing the De by the environmental dose rate (in Gy/ka) gives the luminescence age of the sediment.
Thermoluminescence dating
Samples were treated in the laboratory following conventional procedures for fine-silt (4–20 μm) sample preparation (Mauz et al., Reference Mauz, Bode, Mainz, Blanchard, Hilger, Dikau and Zöller2002) and etched using 20% and 10% HF for various rounds to remove the feldspar-derived luminescence component (Mauz and Lang, Reference Mauz and Lang2004a). After the grains were etched, the silt of 4–15 μm grain size was separated by settling in acetone. The aliquots of each sample consisted of 2 mg of material pipetted onto 1 cm aluminium discs. All samples were tested using infrared stimulation (Duller, Reference Duller2003), which examines the effect of the feldspar on normalised thermoluminescence (TL) measurements and gives an estimate of the amount of feldspar remaining in the sample after chemical treatment (Mauz and Lang, Reference Mauz and Lang2004a). According to these tests, all samples used for further experiments were pure quartz. The self-attenuation of the samples was calculated in a simplified approach using the following equation:

Where D is the attenuated dose of gamma radiation, DO is the dose delivered by the source, μ is the linear attenuation coefficient of the sample, and r is the inner radius of the container. Equation 1 was derived for homogenous and isotropic radiation around a small container, compared with the mean free path of gamma photons (μ ⋅ r << 1). Any attenuation due to the thickness of the “sample” is assumed to result from a homogenous and isotropic radiation field. The 90–160 μm fraction was obtained by dry-sieving, and the 4–11 μm fraction was extracted by settling in acetone. TL measurements were performed using a blue detection window. Some measures were made with and without silicon oil as a fixing agent. An insignificant tendency toward slightly lower results with silicon was observed. All analyses were performed at the ITN (Instituto Tecnologico Nuclear, C2TN, Campus Tecnológico Nuclear, in Portugal, following laboratory procedures from Mauz and Lang (Reference Mauz and Lang2004b) and Richter et al. (Reference Richter, Zink, Przegietka, Cardoso, Gouveia and Prudêncio2003).
Radiocarbon accelerator mass spectrometry (AMS) dating
Radiocarbon AMS dating was restricted to bulk sediment samples from the Valira valleys. Measurement of 14C activity and the isotope ratio 13C/12C was carried out at the Beta Analytic Inc. (Florida, USA) mass spectrometer facility and used the bulk organic fraction from sedimentary samples. Calib software based on IntCal20 curves was used for calibration (Reimer et al., Reference Reimer, Austin, Bard, Bayliss, Blackwell, Bronk Ramsey and Butzin2020). Because radiocarbon dating is routinely applied to the final part of the LGC and the Holocene (Walker, Reference Walker2005), the method is explained in Supplementary Material 3.
RESULTS
Luminescence results are presented from west to east across the study area (Fig. 1), including the sedimentary records and their ages (Tables 1 and 2), while the radiocarbon (14C) ages are exclusively from the Valira valleys (Table 3). Some deposits were sampled twice to assess the coherence between dating methods. These TL samples are LUM-26, LUM-273, and LUM-274 (Table 2); compared with OSL results of samples 122242, 122244, and 122247 (Table 1), respectively, which show good agreement.
Table 2. Thermoluminescence (TL) finite ages from the studied deposits: Lum-24 to Lum-27 (from Turu and Peña-Monné, Reference Turu, Peña-Monné, Pérez-Alberti and López-Bedoya2006), Lum-272 (from Gascón and Turu, Reference Gascón, Turu, Turu and Constante2011), Lum-273 (from Jalut and Turu, Reference Jalut, Turu, Canérot, Colin, Platel and Bilotte2008), and Lum-274 (not published).a

aAbbreviations: arb, above riverbed; asl, above sea level.
Table 3. Radiocarbon dating results from Ginebrosa (β-133971, colluvium) and La Margineda sites (β-489299 organic fraction from colluvium; β-489301 organic fraction from carbonated silts).a

aCalibration using IntCal20 (Reimer et al., Reference Reimer, Austin, Bard, Bayliss, Blackwell, Bronk Ramsey and Butzin2020), δ13C carbon isotopic fractionation. asl, above sea level; BK, bulk organic matter; C, colluvium; Of, organic fraction.
Qtz-OSL dating provided finite ages only in a few ancient samples (122238, 122239, 122242, and 122246; Table 1) and two modern samples (122235 and 122248; Table 1) from the Segre River. In all the other samples, the Qtz-OSL signal was saturated or nearly saturated and only provided minimum ages (122236, 122237, 122240, 122241, 122243, 122244, 122245, 122247, 122256; Table 1). Therefore, signals from K-rich feldspars were used instead because of the higher saturation dose of the dose-response curve for feldspar (Wintle and Murray, Reference Wintle and Murray2006). The pIRIR290 signal was chosen because of its stability (Buylaert et al., Reference Buylaert, Jai, Murray, Thomsen, Thiel and Sohbati2012). We also made several direct tests of the stability of the pIRIR290 signal and checked the suitability of the applied measurement protocol and the completeness of signal bleaching at deposition by using modern samples (122235 and 122248; Table 1). The pIRIR290 signal of several samples is saturated or nearly so; only minimum ages were obtained (122236, 122237, and 122241; Table 1). More complete information about the laboratory measurements of the OSL samples is available in Supplementary Material 4.
Qtz-OSL dating provided finite ages in samples VILALLER-1, STA.COLOMA-2 and FAUCELLES-1 (Table 1), and the two current Segre River bed-load samples, SANAV-0 and STPCodinet-1. The Qtz-OSL signals were too close to saturation for suitable dating for all other samples. For older samples, signals from K-rich feldspars were used instead because of the higher saturation dose of the feldspar dose–response curve (Wintle and Murray, Reference Wintle and Murray2006). These samples are UR-1, CMARG-4, STJULIA-2, SEGUDET-1, ADRALL-4, and GINEBROSA-1 (Table 1). However, several samples were too close to saturation, and only minimum ages were obtained (SANAV-1, SANAV-2, and SORT-1; Table 1). Further empirical information about the offset and saturation of the luminescence signal is provided in Supplementary Material 5.
Results from the upper Noguera Ribagorçana valley
The results from the westernmost side of the studied area (Fig. 1, sector 1) are presented in this section. We briefly summarize the existing knowledge of the area and set our results in context. Radiocarbon dates performed on bulk sediment samples retrieved from glaciolacustrine sediments recovered by coring at a site 500 m north of Sant Mamés (Fig. 2) returned inverted ages (Bordonau, Reference Bordonau1992). Contamination probably affected one or all of these samples, as was presumed to be the case with glaciolacustrine deposits from the Llestui lateral ice-dammed lacustrine complex (Bordonau et al., Reference Bordonau, Vilaplana and Fontugne1993), located upstream in the same valley (Fig. 2). The problem was apparently solved at Llestui, where Bordonau et al. (Reference Bordonau, Vilaplana and Fontugne1993) reported two new ages (23,497–20,612 b2k and 27,671–23,993 b2k) from the LGM glaciolacustrine deposits (Pallàs et al., Reference Pallàs, Rodés, Braucher, Carcaillet, Ortuno, Bordonau, Bourlès, Vilaplana, Masana and Santanach2006).
The exposure of the abandoned Sant Mamés de Vilaller quarry has two divisions: a non-deformed glaciofluvial unit and a syn-sedimentary deformed unit. Within the deformed unit, lenses of laminated sand are embedded in a matrix-supported diamicton, which is interpreted as a melt-out till. An overlapping contact divides the Sant Mamés deposit into two units between glaciofluvial sediments that overlap a diamicton (Fig. 5a). The glaciofluvial unit is predominantly sandy but also includes layers of imbricated gravels, indicating that the sedimentation was influenced by episodes of high meltwater discharge (Fig. 5b). Within the diamicton, we identified a massive lodgement till at the base of the outcrop that grades to crudely bedded sands and gravels including boulders (Fig. 5c) (type C1b facies based on Krzyszkowski and Zieliński [2002]), whereas deformed sandy layers are interbedded at the top of the outcrop (Fig. 5d) (melt-out till facies; Bordonau, Reference Bordonau1992).
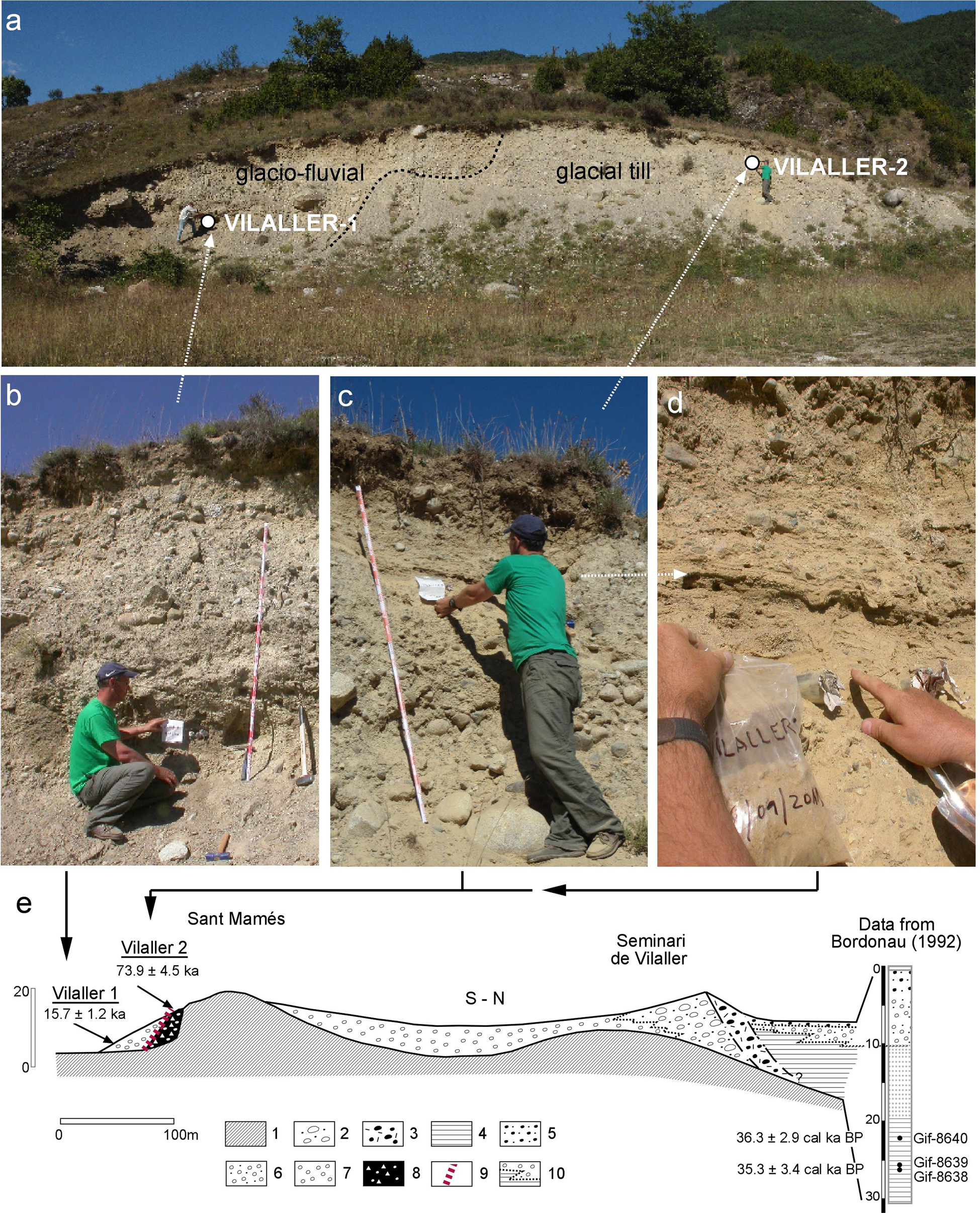
Figure 5. (a) Sketch of glaciofluvial deposits overlapping (dashed line) the glacial till (Fig. 1, sector 1). Collected samples are VILALLER-1 and VILALLER-2; (b) the western side of the Sant Mamés outcrop; (c) the eastern side of the Sant Mamés outcrop; (d) a closer look at the sampled layer embedded in the diamicton; (e) Sant Mamés outcrop. The interpretative section from Bordonau (Reference Bordonau1992) was modified and enlarged, with the location of the new optically stimulated luminescence (OSL) samples added. Accelerator mass spectrometry (AMS) 14C ages are available from a borehole made in the early 1990s and plotted conformably at its vertical scale (Gif-8638: 30.8 ± 1.4 ka BP; Gif-8639: 20.18 ± 0.35 ka BP; and Gif-8640: 31.41 ± 1.2 ka BP; only valid calibrated ages are shown). 1, Rock basement; 2, supraglacial sediments; 3, lodgement; 4, bottom set (lacustrine facies); 5, fluvial; 6, topset (prograding fluvial facies); 7, outwash facies (glaciofluvial); 8, melt-out till; 9, unconformity; 10, facies changes. The black arrows indicate the location of the photographs in part e.
A sample from the glaciofluvial unit provided an age of 15.7 ± 1.2 ka (VILALLER-1; Table 1), whereas the melt-out till gave an age of 73.9 ± 4.5 ka (VILALLER-2; Table 1). The time interval between these ages sheds light on an unconformity between the melt-out till and the later glaciofluvial unit. Further field descriptions are available in Supplementary Material 6.
Results from the upper Noguera Pallaresa Valley
The neighboring glaciated valley eastward from the upper Noguera Ribagorçana is the upper Noguera Pallaresa valley (Fig. 1, sector 2). In this valley, we analysed a glaciofluvial terrace exposure in Sort. Southward from the village of Sort (692 m), a 120-m-long outcrop was visible in 1992, on the right side of the widened N260 highway, revealing a glaciofluvial terrace (Peña-Monné et al., Reference Peña-Monné, Calvet, Turu, Turu and Constante2011; Ventura and Turu, Reference Ventura, Turu, Oliva, Palacios and Fernández-Fernández2022) at the toe of a large and currently inactive landslide (Turu et al., Reference Turu, Hirsch, Peña-Monné, Raab, Ros, Martí-Bono, Constante-Orrios, Turu and Constante2011). A sandy layer from this terrace was sampled for OSL dating (SORT-1; Fig. 6, Table 1) and provided a minimum age >162 ka. The sampled layer overlies coarser deposits and is partly eroded in its upper part (Fig. 6). From bottom to top, we distinguished massive and crudely bedded gravels arranged in sets of low-angle sheets at the bottom of the exposed deposit (Fig. 6); these are partly cut by erosion with a broad channel bar sheet formed by imbricate massive gravels overlying its erosive surface. Crudely bedded sands deposited in low-angle sheets sit concordantly above the coarse deposits. Unfortunately, an unexposed section between this sandy layer and the top of the sequence prevented a complete determination of the stratigraphic record (Fig. 6). However, no significant changes in the sedimentary environment are apparent, there being a profusion of existing imbricated gravels in all visible parts of the sequence. At the top, a poorly consolidated coarse-grained diamicton marks the end of the sedimentary sequence at Sort.

Figure 6. Sample site for optically stimulated luminescence (OSL) dating (SORT-1) at the Noguera Pallaresa glaciofluvial terrace +40 m at Sort (Fig 1, sector 2). Yellow lines = oriented layers; facies C1a = bedded gravels; C1b = channel bar, C2a = low-angle sands sheet; B1b = coarse-grained proximal end-moraine fan.
We attribute the Sort deposit to a type C end-moraine fan, as described by Krzyszkowski and Zieliński (Reference Krzyszkowski and Zieliński2002). The crudely bedded gravels at the base of the deposit corresponding to facies C1a, the channel bars to C1b, and the low-angle sand sheet to C2a. The broad range of facies represents a proximal end-moraine fan (Krzyszkowski and Zieliński, Reference Krzyszkowski and Zieliński2002), above which are the remains of the frontal moraine (uppermost facies B1b; Fig. 6).
Results from the Valira valleys
The Valira River joins the Segre at La Seu d'Urgell (Fig. 1, sector 3). Its basin (592 km2) covers most of the Principality of Andorra (Fig. 3).
The Segudet kame
The Segudet kame complex is located at the confluence of the Cassamanya and Les Aubes streams (Fig. 7a). A subglacial till deposited at Segudet (1350 m; Fig. 7a) may indicate the limits of the ice-dammed valley impounded by the Ordino glacier (Turu et al., Reference Turu, Calvet, Bordonau, Gunnell, Delmas, Vilaplana, Jalut, Hughes and Woodward2017). A supraglacial till exists on the hillslope (1520 m; Fig. 7b), deposited by the 200-m-thick trunk glacier. The kame comprises thick glaciofluvial gravels, mainly located in its central part. It is possible to trace this gravely unit to the edge of the sedimentary complex, where it grades to sands and silts. The OSL sample was taken from a laminated fine sand layer (Fig. 7c and d) and provided an age of 141 ± 16 ka (SEGUDET-1; Table 1). Complementary field descriptions are provided in Supplementary Material 6.

Figure 7. (a) Facies from the Segudet sedimentary sequence (Fig. 1, section 3). 1a, undifferentiated bedrock; 1b, not exposed; 2, diamicton; 3, glaciofluvial kame terrace laying over a polished surface; 4, glaciolacustrine deposits; 5, stratified colluvium; 6, identified unconformity (erosive surface) or supposed; 7, streams; 8, buildings; 9, road; 10, OSL sampling site. (b) Cross section (A-B) and location of sample SEGUDET-1 collected from rhythmites of the kame terrace complex. (c and d) Pictures of the stratigraphic position of sample SEGUDET-1.
The Ginebrosa kame
The Ginebrosa deposits are found 200 m above the Arinsal riverbed (1449 m; Fig. 3) and are related to a large kame terrace formed in a lee-side position between the Arinsal glacier (Fig. 3) and adjacent bedrock. Silts and sand lenses are located away from the former slope–glacier ice contact (Fig. 8a). These lenses are interbedded with colluvium from nearby bedrock erosion. On the opposite side of the kame deposits, close to the former ice contact, deformed sediments include intrusions of cohesive clay lenses.
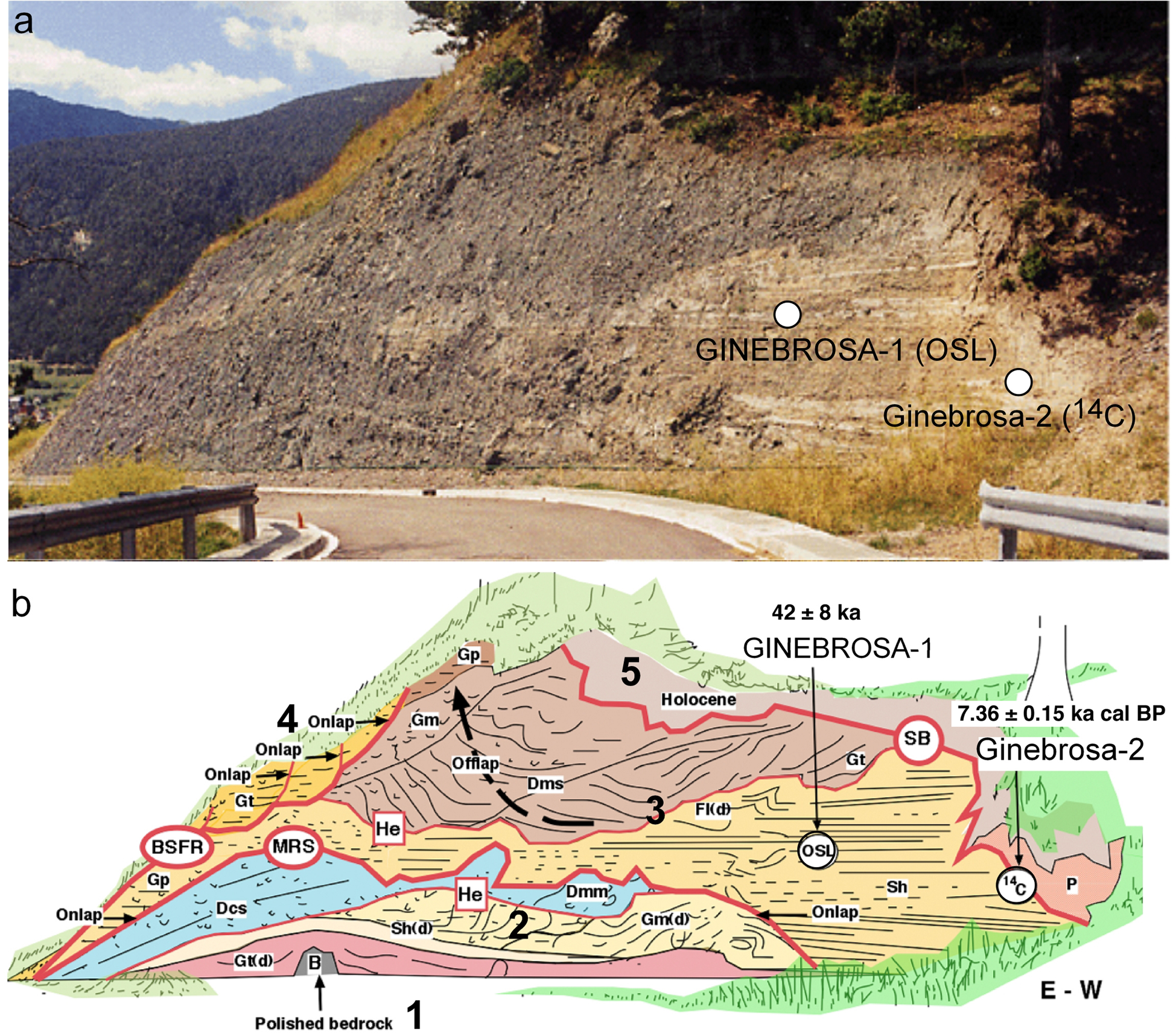
Figure 8. (a) Picture from the Ginebrosa outcrop (60 m long, 15 m high), showing the location of the samples. (b) Sedimentary units: 1, polished bedrock; 2, glacial unit; 3, main infill sequence; 4, final sedimentary stage; 5, recent colluvium and soil. Sedimentary facies identification: Dms: diamicton, matrix-supported, stratified; Dmm: diamicton, matrix-supported, massive; Dcs: diamicton, clast supported, stratified; Gm: massive or crudely bedded gravels, imbrication; Gm(d): deformed Gm; Gt: stratified gravels, trough crossbedding, minor channel fills; Gt(d): deformed Gt; Gp: stratified gravels, planar crossbedding; Sh: sand and pebbly sand, horizontal lamination; Sh(d): deformed Sh; Fl(d): deformed laminated fines (sands–silts and clays); P: pedogenic features, soils. Sequence stratigraphy surfaces and geometric relationships: arrows: offlap and onlap contacts; He: erosive surface; MRS: maximum regressive surface; BSFR: the basal surface of forced regression; SB: sequence boundary. Locations of the 14C dating (GINEBROSA-2) and optically stimulated luminescence (OSL; GINEBROSA-1) samples as labelled.
This small valley has been accessible since 1997, and several outcrops created by building work have been available for analysis. The sedimentary sequence was emplaced directly over polished bedrock from a previous MIE of unknown age (Fig. 8b, 1). Four sedimentary units were identified (Figure 8b, 2–5). Sandy lenses (Shr) and horizontally bedded imbricated gravels (Gt) form the lowermost unit (Fig. 8b, 2), draped over the bedrock and covered by massive gravels (Gm) beneath a diamicton layer (Dmm, Dcs) deposited by a glacial advance. A final sedimentary unit (Fig. 8b, 3), overlapped by sands and silts (Sh) grading to coarse gravels (Gp facies) (Fig. 8b) filled the irregular surface (Catuneanu, Reference Catuneanu2006; Fig. 8b, MRS). Instability caused by lateral glacial thrusting produced lenticular injections of cohesive clay. The age of the interbedded laminated sand lenses is 42 ± 8 ka (GINEBROSA-1; Table 1). A Holocene postglacial unit (Fig. 8b, 5) formed by fine-grained colluvium gave dates of 9.17 ± 0.15 ka cal BP using a 14C AMS determination on bulk organic matter (GINEBROSA-2; Table 3).
The Santa Coloma quarry
In the Santa Coloma terminal complex (Fig. 9a), several fining-upward fluvial sequences deposited from high-energy floods (Fig. 9b) were described by Turu and Peña-Monné (Reference Turu, Peña-Monné, Pérez-Alberti and López-Bedoya2006). Two field campaigns in December 2005 (Fig. 9a and c) and September 2011 (Fig. 9b) were conducted to collect luminescence dating samples. Respective results are quite similar: 9.5 ± 0.3 ka using TL (STA.COLOMA-1; Table 2) and 11.4 ± 1.4 ka using OSL (STA.COLOMA-2; Table 1). Further field descriptions are available in Supplementary Material 6.

Figure 9. (a) Interpretative section from Nussbaum (Reference Nussbaum1956) at Santa Coloma (Andorra); m, moraine, gr, gravels, eb, boulders. (b) Arrows indicate sedimentary units from Nussbaum (Reference Nussbaum1956) and in the 1990s outcrop. Two samples were collected from the same layer and position of the Santa Coloma fluvial terrace.
La Margineda
The main Andorran valley becomes narrower southward of Santa Coloma (Fig. 10a), and an urbanized hill, known as La Margineda (990 m), stands on the valley's eastern side eastern. We examined an existing outcrop rich in sedimentary facies located at +65–75 m arb (1008 m asl) in a road cutting (Fig. 10b). Here, colluvial facies overlie a glaciofluvial deposit, both densely compacted (Fig. 10c), from which samples were collected (Fig. 10c) for AMS and luminescence determinations. Two sedimentary discontinuities are identified in the road cut (Fig. 10d), differentiating three units (Fig. 10e). The lowermost unit, sampled for OSL dating, comprises imbricated gravels with a significant presence of allochthonous lithologies (e.g., granites), probably transported as bedload by meltwater mixed with colluvium from a melting glacial front and the end-moraine (Llobet, Reference Llobet1947; Turu and Peña-Monné, Reference Turu, Peña-Monné, Pérez-Alberti and López-Bedoya2006) reworking. This unit is from MIS 5c (91 ± 9 ka; CMARG-4; Table 1); however, the mentioned end-moraine buildup occurred earlier, probably during MIS 5d. In the middle unit (Fig. 10e), clasts are arranged with their long axes parallel to the slope (colluvium). However, some clasts are imbricated, and others set as though belonging to palaeochannels (lower unit). The occasional presence of allochtonous lithologies (e.g., granites) may come from the erosion of the terminal-moraine complex. The age of the colluvium (Fig. 10e, LM-1) is 26.931–26.201 ka cal BP (22.290 ± 0.090 ka BP; ß-489299; Table 3). The upper unit (Fig. 10e) is formed mainly by angular locally sourced gravels supported by a sandy-silt matrix belonging to the slope's current scree. The general glacial recession from La Margineda predated the deposition of the upper unit (unit 3; Fig. 10e). We were able to date this last unit in an existing road cutting 100 m south of the one described above. Here, unit 3 is very coarse and may come from a rock avalanche. We could date the matrix of this rock avalanche deposit using 14C AMS. The age of unit 3 is 16.018 ± 0.183 ka cal BP (sample LM2b; Table 3). These stratigraphic units belonging to MIS 2 are also identified 650 m southward from La Margineda, in Aixovall (Fig. 3, sector 3) from an existing open pit excavation (Fig. 11). Complementary field descriptions are provided in Supplementary Material 6.

Figure 10. (a) La Margineda and Santa Coloma geomorphology, overprinted on an image from Google Earth. Cited sites are indicated with the location of the dated samples: 1, LM; 2, LM-2a; 3, LM-2b; 4, CMARG-4. The lines mark the limits of the Valira glacier at different dates. (b) A general overview of deposits on top of the hill. The rectangular area shows the consolidated colluviums in parts c to e. (c) A closer look at the consolidated colluvium. (d) Sedimentary facies: Gm: massive or crudely bedded gravels, imbrication; Gt: stratified gravels, trough crossbedding, minor channel fills; Gp: stratified gravels, with planar crossbedding; Sh: sand and pebbly sand, with horizontal lamination. The sequence boundaries (SB) between units are erosive (He). (e) La Margineda sedimentary units: unit 1: gravels and sands having coarse crossbedding; units 2 and 3: fining-upward sequences of colluvium; g: granite. The locations of the samples for optically stimulated luminescence (OSL) and 14C dating are represented in parts c and e.
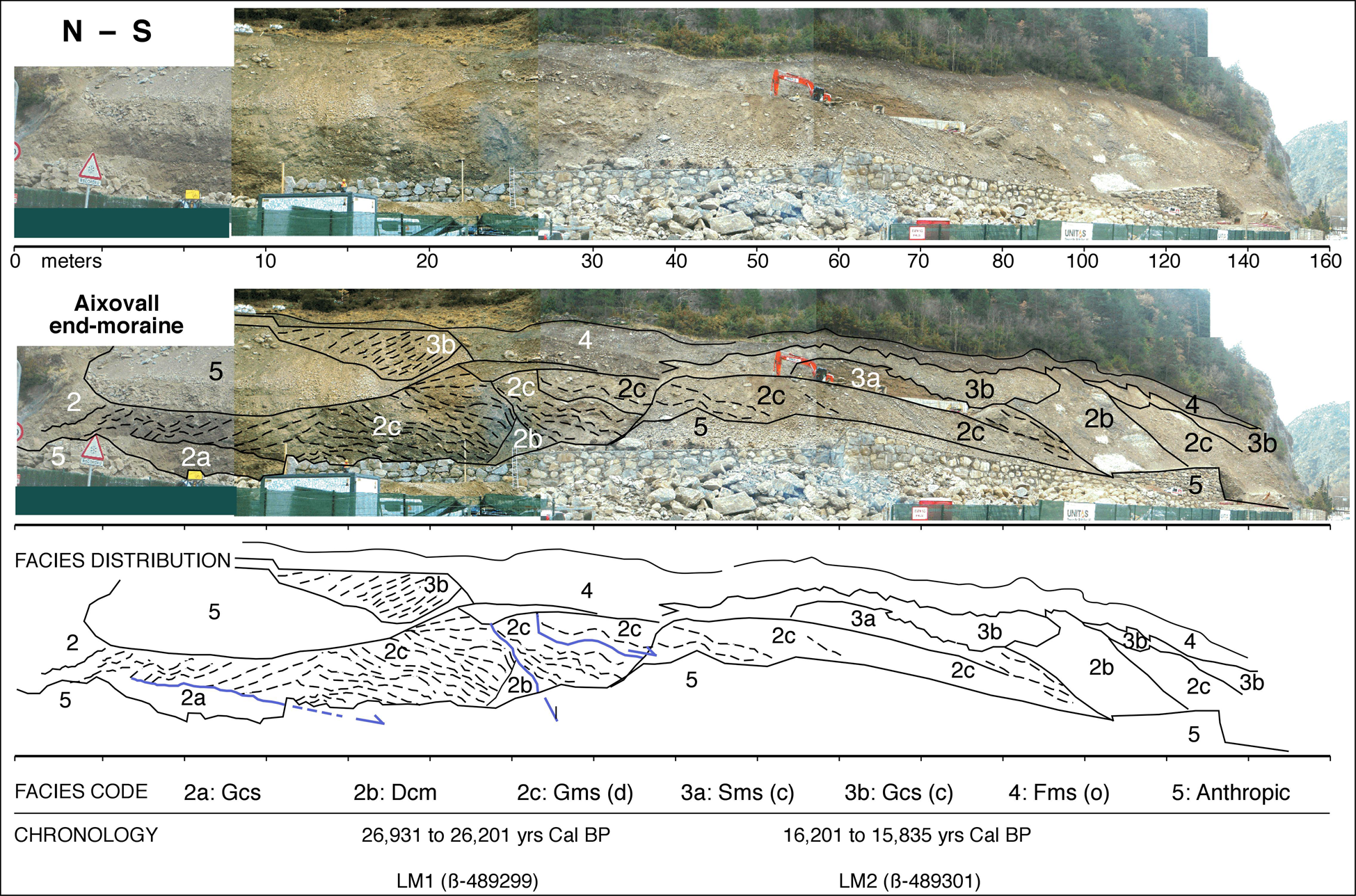
Figure 11. The Aixovall LGM end moraine (937 m asl) and stratigraphy from La Margineda at Aixovall. Facies 2a: glaciofluvial imbricate gravels; facies 2b: supraglacial till, including boulders of local (calcschists) and allochthonous (black slates) lithology; facies 2c: tilted bedding of glaciofluvial gravels mixed with coarse colluvium within thrusted blocks induced by glaciotectonics; facies 3a: stratified slope deposits, matrix-supported; facies 3b: cemented grèzes litées; facies 4: organic colluvium; facies 5: masonry, artificial slopes, and sections disturbed by excavation.
The Sant Julià de Lòria outcrop
The village of Sant Julià de Lòria (900 m) is located in a V-shaped valley (Fig. 12). Here, the narrow Valira valley is a fluvial gorge carved in calcshists running southward from La Margineda. Cross-stratified sands were dated using TL and OSL (Fig. 13a). When error bars are considered, the dates from different methods converge: the TL age is 32.8 ± 1.2 ka (STJULIA-1; Fig. 13b, Table 2), and the OSL age is 36 ± 3 ka (STJULIA-2; Fig. 13c, Table 1). Geotechnical boreholes for a new bridge connecting a local road to the highway (Fig. 13d, profile) allowed us to identify a glacial diamicton 6 m beneath the Valira riverbed and beneath the studied exposure. The dated melt-out till (Fig. 13d, Till 2) should be younger than the diamicton located beneath the riverbed (Fig. 13d, Till 1). A previous southward terminal glacial-front position is deduced by erratics accumulated on the eastern margin of the valley at Cal Tolse (Fig. 12) and farther down the valley (Fig. 3), close to the Spanish–Andorran border (Bastida de Ponts glacial front; Fig. 3), but in an inner position regarding the LMIE far-flung moraine (Barr and Lovell, Reference Barr and Lovell2014) at Pont Trencat (Turu et al., Reference Turu, Boulton, Ros, Peña Monné, Martí Bono, Bordonau and Serrano2007, Reference Turu, Calvet, Bordonau, Gunnell, Delmas, Vilaplana, Jalut, Hughes and Woodward2017; Fig. 3). Further field descriptions can be found in Supplementary Material 6.
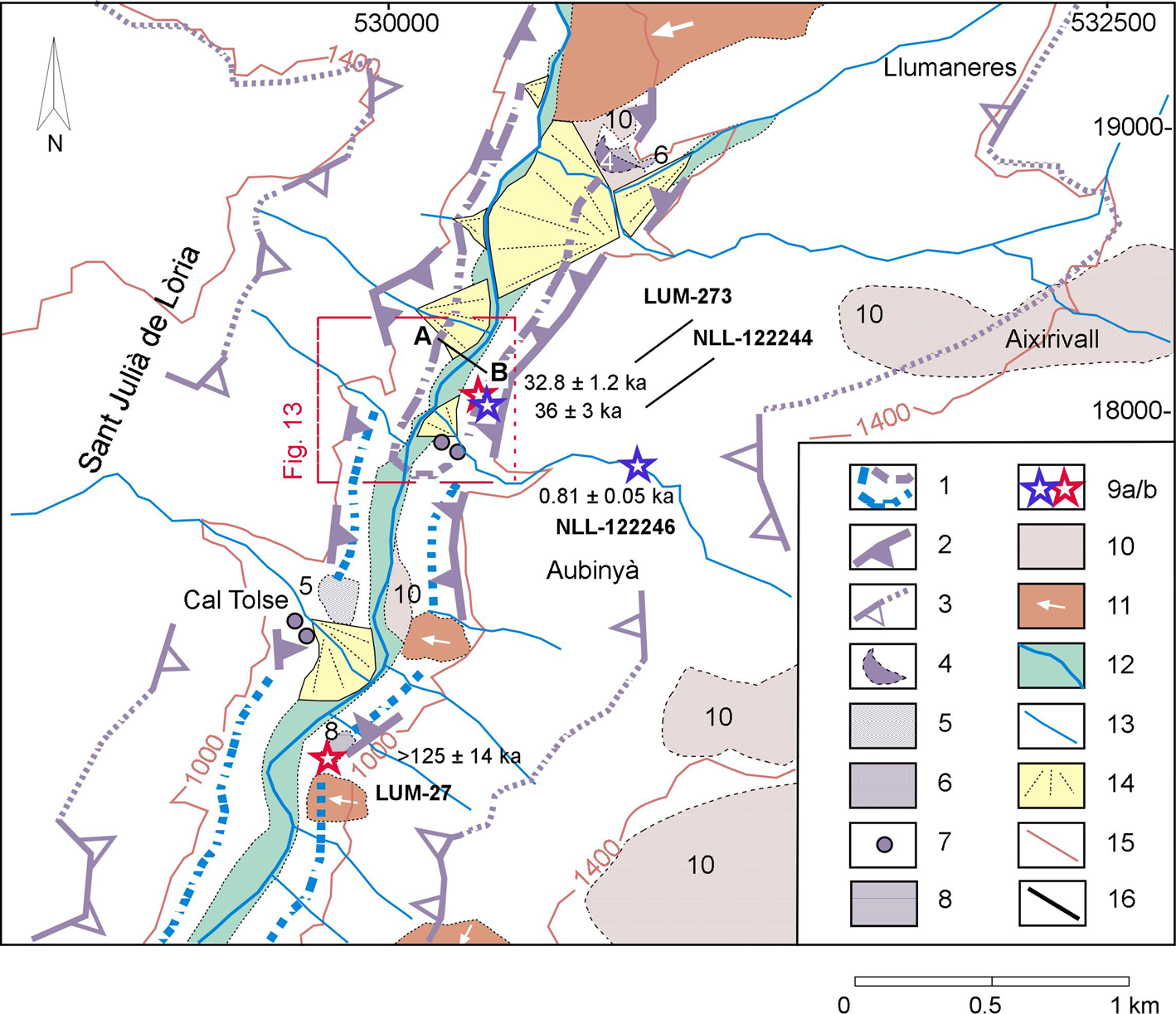
Figure 12. Geomorphological sketch map from the Sant Julià de Lòria area: 1, the younger than MIS 4 glacial fronts at Sant Julià de Lòria and Bastida de Ponts glacial front; 2, glacier ridge previous to 180 ka after the Cal Tolse till; 3, the MIS 4 LMIE glacial ridges of Pont Trencat glacial front; 4, front-lateral till deposits from the Cal Tolse–Adrall 4 outwash (~180 ka); 5, hump (glacial); 6, undifferentiated till; 7, erratic boulders; 8, glaciofluvial deposits recorded to (4); 9, available dates (red: thermoluminescence [TL] sample; blue: optically stimulated luminescence [OSL] sample); 10, colluvium; 11, landslide; 12, modern riverbed deposits; 13, stream; 14, alluvial fan; 15, topographic contour lines (meters above sea level); 16. Profile A-B interpreted in Fig. 13d.
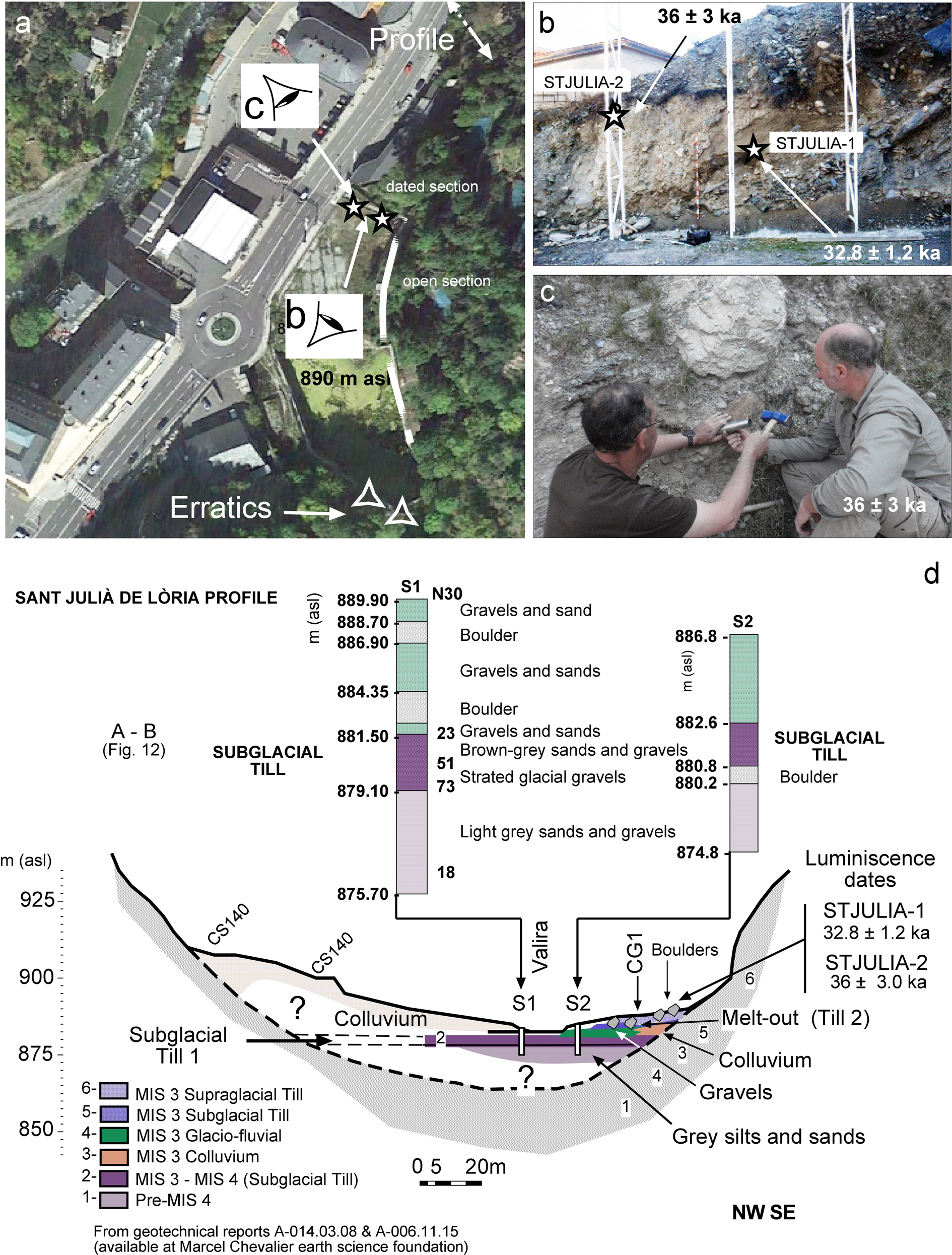
Figure 13. (a) Google Earth image from the south Sant Julià de Lòria area. Point of view (eye symbol) of extensive open-pit excavation and sedimentary descriptions. The position of the dated section is labelled. (b and c) Pictures of the outcrop with the location of the sample ST JULIA-1 from cross-stratified sands (March 2006) and ST JULIA-2 from laminated sands on a melt-out (taken September 2011). (d) Stratigraphy of the Sant Julià de Lòria valley floor. The dated supraglacial system (samples STJULIA-1 and STJULIA-2) includes colluvium facies (3), glaciofluvial gravels (4), and melt-out till (5).
Results from the upper Segre
This section refers to three exposures, one close to the River Segre and two in the Cerdanya depression.
The upper Segre at Adrall
The exposure here is located 4.5 km below the Valira–Segre confluence (Fig. 1, sector 3). In Adrall (635 m asl), a 75-m-long outcrop was opened in 1986 during the widening of the N260 highway; however, it was not until 30 years later that the fluvial deposits were studied (Turu and Peña-Monné et al., Reference Turu, Peña-Monné, Pérez-Alberti and López-Bedoya2006) and were shown to belong to the glaciofluvial Segre–Valira terrace system (Turu et al., Reference Turu, Boulton, Ros, Peña Monné, Martí Bono, Bordonau and Serrano2007; Peña-Monné et al., Reference Peña-Monné, Calvet, Turu, Turu and Constante2011). The lowermost terrace level at Adrall (+38 m arb) incorporates three fining-upward sequences, each starting above an erosive surface (Fig. 14). These sequences grade upward from granite-rich gravels of Segre origin to schist-rich deposits of local derivation (Fig. 14). The whole terrace has been reddened by advanced weathering of iron minerals (oxidation), coatings, and meniscuses. TL ages (ADRALL-3; Table 2) are 120 ± 5.5 ka, but older ages were obtained using the OSL pIRIR290 procedure (179 ± 15 ka; ADRALL-4; Fig. 14, Table 1).

Figure 14. The upper division of the Segre-Valira glaciofluvial T5 terrace at the Adrall site. Location of the ADRALL-3 (thermoluminescence [TL]) and ADRALL-4 (optically stimulated luminescence [OSL]). The lower part of the exposure contains fine to coarse sands, while the upper portion comprises rounded boulders and imbricated cobbles and pebbles (g: granite boulder).
The upper Segre at Sanavastre
In the southern part of the Cerdagne basin (Fig. 1, sector 4), the Abellorols quarry in Sanavastre provided appropriate outcrops for OSL sampling in cross-stratified sandy layers surrounded by lenses of large cobbles. Samples from two levels (SANAV-1 and SANAV-2; Fig. 4b and c, Table 1) proved to be older than 200 ka. Available field descriptions are provided in Supplementary Material 6.
The Ur-Llaurar rest area
The Ur exposure is located 3 km above the Rahur–Segre confluence, on the eastern side of the N20 highway at the French village of Ur (Fig. 1, sector 4). An ancient quarry from the French N20 highway widening work in the mid-1980s was restored in a rest area. Exposures reveal several units (Fig. 15) of glaciofluvial channel deposits (units 1, 2, 4, and 5) and diamictons (units 3 and 6) belonging to an old glaciofluvial terrace from the Segre–Rahur river system (Fig. 4). The lowermost layer, unit 1, is a gravelly layer with trough cross-bedding. In contrast, unit 2 is a pebbly sand body with some imbricated rolled cobbles in an ochrous sandy matrix and with a ribbon geometry. It was partly eroded before or during the emplacement of the overlying units 3 and 4. An intercalated sand layer was dated to 103 ± 13 ka (UR-1; Table 1). Unit 3 is a massive diamicton with angular boulders, and unit 4 is composed of cross-stratified gravels with intercalated sheet sands. Unit 5 is mainly formed by gravels following a trough cross-bedding pattern, with a maximum thickness of several tens of meters. Unit 6 corresponds to a diamicton that includes striated clasts (Calvet et al., Reference Calvet, Delmas and Gunnell2013).

Figure 15. Drawing showing an interdigitation of glaciofluvial channel deposits (units 1, 2, 4, and 5) with tills (units 3 and 6). Original photography from Calvet et al. (Reference Calvet, Delmas, Gunnell, Braucher, Bourlès, Turu and Constante2011a). The glaciofluvial unit was sampled (UR-1), indicating its correlative location. (Colours are only for guidance.)
Correlation between valleys
Based on AMS 14C dates from kame deposits and in situ 10Be CRE ages from both erratic boulders and moraines, the LGM phase is now well-defined in the eastern Pyrenees (Delmas, Reference Delmas2005; Delmas et al., Reference Delmas, Gunnell, Braucher, Calvet and Bourlès2008; Pallàs et al., Reference Pallàs, Rodés, Braucher, Bourlès, Delmas, Calvet and Gunnell2010; Palacios et al., Reference Palacios, Gómez-Ortiz, Andres, Vazquez-Selem, Salvador-Franch and Oliva2015; Turu et al., Reference Turu, Calvet, Bordonau, Gunnell, Delmas, Vilaplana, Jalut, Hughes and Woodward2017). However, the number and the magnitude of glacial recession events during the LGC, their chronology, and those of the PGC still remain obscure.
In the studied area, the new OSL ages confirm glacial sedimentary records from MIS 6 to late MIS 2; nonetheless, glaciations older than MIS 6 are also indicated in the region. In the upper Noguera Pallaresa basin, the glaciofluvial terrace (+30–40 m arb) at Sort (Fig. 6) is older than 162 ka (Table 1). At Sanavastre (Fig. 4), +35–40 m and +25–30 m terrace levels are older than 200–180 ka (Table 1); both levels may correlate with Calvet's T4 terrace (Calvet, Reference Calvet, Gómez-Ortíz and Pérez-Alberti1998). Calvet's T3 terrace is 3–4 m thick and consists of sand lenses within gravels and cobbles. Cryoturbed colluvium deposits are connected to this terrace in the upstream area and suggest a cold context affecting this T3 glacis (Calvet, Reference Calvet, Gómez-Ortíz and Pérez-Alberti1998). The age obtained for UR-1 (Fig. 4, 103 ± 13 ka) constrains the T3 terrace.
Near the Segre–Valira confluence (Fig. 1, sector 3), Turu and Peña-Monné (Reference Turu, Peña-Monné, Pérez-Alberti and López-Bedoya2006) correlated the Adrall deposits (ADRALL-3 and ADRALL-4; Table 1) and the Cal Tolse glaciofluvial sequence (LUM-27; Fig. 12) based on their similar states of weathering. Moreover, the ADRALL-3 and ADRALL-4 samples may come from a distal terrace (Fig. 14) of the Cal Tolse outwash when the Valira glacier front remained upstream at Sant Julià de Lòria (Llumeneres end-moraine till; Turu and Peña-Monné, Reference Turu, Peña-Monné, Pérez-Alberti and López-Bedoya2006; Fig. 12). The recurrence of the main ice front in Sant Julià de Lòria was able to build several terminal complexes (Fig. 12); similarly, the tributary glacier was able to produce kame terraces in Segudet (Fig. 7), at both PGC (alpine Riss glaciation) and the LGC (alpine Würm glaciation). The Segudet kame complex, comprising the Cal Tolse terminal complex and the Adrall terrace (ADRALL-3 and ADRALL-4 samples; Tables 1 and 2), belongs to the Riss alpine glaciation (PGC). However, the glaciofluvial terraces are from the ADRALL-1 and ADRALL-2 samples (Table 2) and, therefore older than 180 ka (ADRALL-4; Table 1). Close to the Valira–Segre confluence, the glaciofluvial terrace represented by sample ADRALL-2 correlates with the Tossal Bordar far-flung moraine (Turu and Peña-Monné, Reference Turu, Peña-Monné, Pérez-Alberti and López-Bedoya2006; Fig. 1, sector 3) located +100 m arb. The intense weathering of the ADRALL-2 glaciofluvial terrace (Poch et al., Reference Poch, Simó and Boixareda2013) advocates for an age older than the MIS 6 glaciation, but it is unfortunately still undated.
The western area
The Sant Mamés melt-out till (73.9 ± 4.5 ka; VILALLER-2; Table 1) provides information about the upper age limit for the LMIE phase, belonging to the end of the MIS 5a–MIS 4 interval. The distribution of erratic boulders over the slopes in Vilaller (Mey, Reference Mey1965; Vilaplana, Reference Vilaplana1983a, Reference Vilaplana1983b; Rodés, Reference Rodés2008), as well as a few remains of kame terraces close to the Noguera de Tor confluence, indicates that the tributary glacier joined the main glacier (Noguera Ribagorçana) during the LMIE and the resulting glacial front finished at the village of Pont the Suert. Unfortunately, no end moraine could be identified there. However, the former glacial front should be close to its outwash, which is the glaciofluvial terrace located south of Pont de Suert (Fig. 2).
Once the Noguera Ribagorçana glacier retreated from the LMIE position, it emplaced the southernmost till identified from outcrops in the study area that was 2 km south of Vilaller (Fig. 2); this is the Sant Antoni till (~940 m) (Vilaplana, Reference Vilaplana1983a, Reference Vilaplana1983b; Bordonau, Reference Bordonau1992; Pallàs et al., Reference Pallàs, Rodés, Braucher, Carcaillet, Ortuno, Bordonau, Bourlès, Vilaplana, Masana and Santanach2006). Located ~15 m above the Noguera Ribagorçana riverbed, it includes abundant rounded to subangular granodiorite boulders ≈1 m in diameter (Pallàs et al., Reference Pallàs, Rodés, Braucher, Carcaillet, Ortuno, Bordonau, Bourlès, Vilaplana, Masana and Santanach2006 and references therein). However, the absolute age of the Sant Antoni till is still unknown.
The glaciofluvial deposits of Sant Mamés (Vilaplana, Reference Vilaplana1983a; Bordonau et al., Reference Bordonau, Pous, Queralt and Vilaplana1989; Bordonau, Reference Bordonau1992; Pallàs et al., Reference Pallàs, Rodés, Braucher, Carcaillet, Ortuno, Bordonau, Bourlès, Vilaplana, Masana and Santanach2006; Fig. 2) correspond to the end of the infilling of the Seminari de Vilaller overdeepened trough (Fig. 5). Three AMS 14C ages were obtained in the glaciolacustrine infill (Bordonau, Reference Bordonau1992; Fig. 5) just behind the Seminari de Vilaller frontal moraine (Gif-8638 at ~26.15 m depth; Gif-8639 at ~26.00 m depth; and Gif-8640 at ~21.95 m depth). Their ages were as follows: Gif-8638 is 38.684–31.891 ka cal BP (δ13C = −23.55‰); Gif-8639 is 25.201–23.372 ka cal BP (δ13C = −24.02‰); and Gif-8640 is 39.149–33.449 ka cal BP (δ13C = −23.45‰). The age reversal between samples (Fig. 5e) left the chronology of the moraine buildup as an unsolved question (Bordonau, Reference Bordonau1992). Nevertheless, two moraine ridges flank the valleys at this point, the Llestui within the Llauset tributary and the Artigallonga (1715 m), which is a lateral moraine complex (Fig. 2) at +600 m (Bordonau, Reference Bordonau1992), where Pallàs et al. (Reference Pallàs, Rodés, Braucher, Carcaillet, Ortuno, Bordonau, Bourlès, Vilaplana, Masana and Santanach2006) obtained 10Be CRE ages from glacial boulders. The updated CRE ages from Tinabre and Artigallonga can be found in Supplementary Material 7: 26.9 ± 6.2 ka (TIN01), 23.4 ± 3.4 ka (ART02), and 20.8 ± 5.1 ka (ART03) (Fig. 2); they are similar to those reported by Delmas et al. (Reference Delmas, Gunnell, Calvet, Reixach, Oliva, Palacios, Hughes, García-Ruiz and Andres2021b). However, these ages are compatible with those from Lake Llauset (25.886–24.467 ka b2k; Vilaplana, Reference Vilaplana1983a), which was then ice-free (Montserrat-Martí, Reference Montserrat-Martí1985), a circumstance not reported by Delmas et al. (Reference Delmas, Gunnell, Calvet, Reixach, Oliva, Palacios, Hughes, García-Ruiz and Andres2021b). Therefore, the main Noguera Ribagorçana glacial system penetrated the Llauset valley and formed a paleolake at Llestui (Fig. 2) at 26.9 ± 6.2 ka (TIN01; 1301 m) and extended beyond Vilaller, not in the Seminari de Vilaller (993 m) as Pallàs et al. (Reference Pallàs, Rodés, Braucher, Carcaillet, Ortuno, Bordonau, Bourlès, Vilaplana, Masana and Santanach2006) suggested. Thus, the age of the Seminari de Vilaller frontal moraine complex (Fig. 5e) is probably equivalent to the youngest age of the Artigallonga lateral moraine complex (Fig. 2), and that is 20.8 ± 5.1 ka (ART03). Moreover, the infill of the valley bottom behind the Seminari de Vilaller (Fig. 5e) should be older than the age of Tinabre (TIN01), returning a minimum age of 39.149–31.891 ka cal BP (Gif-8638 and Gif-8640); thus, the age of Gif-8639 (Fig. 5e) must be rejected. It should be noted that δ13C from Gif-8636 is slightly depleted compared to the other two, which could imply the inclusion of younger bulk carbon within the sample, not inactive carbon (cf. Pallàs et al., Reference Pallàs, Rodés, Braucher, Carcaillet, Ortuno, Bordonau, Bourlès, Vilaplana, Masana and Santanach2006), giving an older age. If not amended, it is usual that contamination occurs during the borehole extractive procedure (Turu et al., Reference Turu, Carrasco, Pedraza, Ros, Ruiz-Zapata, Soriano-López and Mur-Cacuho2018).
The eastern area
In the Valira valley, the CMARG-4 (91 ± 9 ka; Table 1) sample gave an age within MIS 5c for the Valira glacier terminal complex at La Margineda, which is possibly equivalent to the Ur glaciofluvial terrace (103 ± 13 ka, MIS 5c; UR-1; Table 1). The early Würm (MIS 5c) glacial front of La Margineda (Fig. 10) is 4.6 km upstream from the Cal Tolse Rissian terminal complex (Fig. 3); thus, in Cerdagne, the corresponding T3 end moraine would have been located upstream of the Ur locality and would have been surpassed by later glacial advances forming the M1 and M2 close-nested moraine complex (Barr and Lovell, Reference Barr and Lovell2014) of the River Querol (Pallàs et al., Reference Pallàs, Rodés, Braucher, Bourlès, Delmas, Calvet and Gunnell2010; Fig. 4).
From the tributary valley of Arinsal (NW Andorra), four sedimentary episodes are distinguished in the Ginebrosa kame complex (Fig. 8), documenting glacial evolution during mid-MIS 3: (1) deposition of lodgement till at the beginning of MIS 3 or earlier; (2) a mid-MIS 3 glacial thinning and stabilisation phase (42 ± 8 ka; GINEBROSA-1; Table 1) with deposition of rhythmites and a predominance of fine sands; (3) glacial advance and deformation of previous deposits, shearing, and supraglacial till deposition; (4) rapid glacial retreat during MIS 3 affecting also the main glacier (Turu et al., Reference Turu, Calvet, Bordonau, Gunnell, Delmas, Vilaplana, Jalut, Hughes and Woodward2017).
The main glacial front retreated back to Sant Julià de Lòria (Fig. 13), and there was buildup of a new terminal complex between 39 and 31 ka (STJULIA-1 and STJULIA-2; Tables 1 and 2). However, the Santa Coloma fluvial terrace from the Valira River is much younger, dating from MIS 1 (terrace T9 of Peña-Monné et al. [2011]). The MIS 2 end moraine in Santa Coloma (Nussbaum, Reference Nussbaum1956; Turu et al., Reference Turu, Boulton, Ros, Peña Monné, Martí Bono, Bordonau and Serrano2007, Reference Turu, Calvet, Bordonau, Gunnell, Delmas, Vilaplana, Jalut, Hughes and Woodward2017) had been partially eroded or overlapped by later outwash.
DISCUSSION
We found some key deposits from the Segre River tributaries that are beyond the range of the K-feldspar OSL dating method (Fig. 16), always weathered, and mainly located beyond the limits of the maximum Würmian glaciation (LGC) (Turu and Peña-Monné, Reference Turu, Peña-Monné, Pérez-Alberti and López-Bedoya2006; Lewis et al., Reference Lewis, Mcdonald, Sancho, Peña Monné and Rhodes2009; Jalut et al., Reference Jalut, Turu, Dedoubat, Otto, Ezquerra, Fontugne and Belet2010; García-Ruiz et al., Reference García-Ruiz, Martí-Bono, Peña-Monné, Sancho, Rhodes, Valero-Garcés, González-Sampériz and Moreno2013; Oliva et al., Reference Oliva, Palacios, Fernández-Fernández, Rodríguez-Rodríguez, García-Ruíz, Andrés and Carrasco2019). An example of this is in Cerdagne, where moraine M3 was assigned to the middle Pleistocene (Calvet et al., Reference Calvet, Delmas and Gunnell2013), and we now correlate it with the older than 200 ka Sanavastre terrace (Fig. 17a, T4).
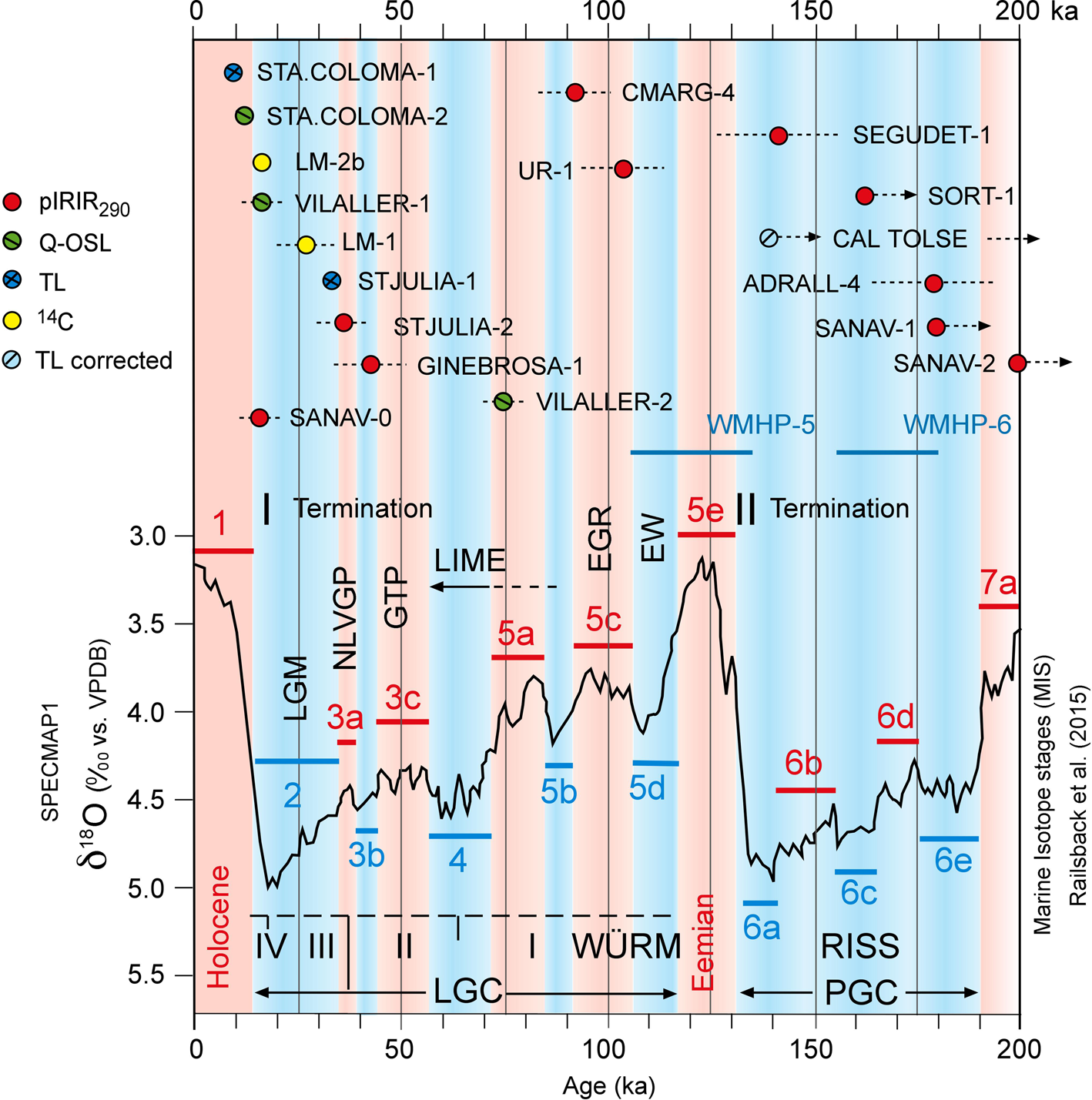
Figure 16. Thermoluminescence (TL), quartz optically stimulated luminescence (Qtz-OSL), pIRIR290, and 14C ages plotted over the Specmap1 curve and the marine isotope stages (MIS) for the last 200 ka (modified from Railsback et al., Reference Railsback, Gibbard, Head, Voarintsoa and Toucanne2015; Martinson et al., Reference Martinson, Pisias, Hays, Imbrie, Moore and Shackleton1987). SANAV-0 may indicate the pIRIR290 offset in the uppermost River Segre. Penultimate glacial cycle (PGC) or Riss, the last interglacial (Eemian), the last glacial cycle (LGC) or Würm and subdivisions (I, II, III, IV; based on Renault-Miskovsky and Girard, Reference Renault-Miskovsky and Girard1998). Terminations I and II indicated; as well, the Western Mediterranean Humid Periods (WMHP) 6 and 5. South-central eastern Pyrenean glacial phases: early Würm (EW), early glacial recession (EGR), last maximum ice extent (LMIE), glaciers thinning period (GTP), no large valley glaciers period (NLVGP), and the global last glacial maximum (LGM) period.

Figure 17. Classification of the glacial terminal complexes from the south-central and southeastern slope of the Pyrenees, including, for comparison, a case from the SW Pyrenees and the north-central slope of the mountain range. (a) Querol terminal complex formed by nested end moraines (Pallàs et al., Reference Pallàs, Rodés, Braucher, Bourlès, Delmas, Calvet and Gunnell2010) and the related glaciofluvial system of terraces (Poch et al., Reference Poch, Simó and Boixareda2013). Terrace T3 onlap, the oldest end-moraine M3 correlated with the oldest glaciofluvial level (T4). (b) The Garonne terminal complex, formed by close-nested and nested end moraines (Stange et al. (Reference Stange, Van Balen, Kasse, Vandenberghe and Carcaillet2014) and Fernandes et al. (Reference Fernandes, Oliva, Palma, Ruiz-Fernández and Lopes2017)). The oldest ages from the outer end moraine are from MIS 6; however, younger ages were also reported by Fernandes et al. (Reference Fernandes, Oliva, Vieira, Palacios, Fernández-Fernández, Delmas, García-Oteyza, Schimmelpfennig and Ventura2021) in this terminal complex that can be correlated with the glaciofluvial terraces (Bricker et al. Reference Bricker2014) and the chronology of lake Barbazan (Andrieu et al., Reference Andrieu, Hubschman, Jalut and Hérail1988) and their surrounding end moraines. (c) The Valira terminal complex is the most diverse case (Turu and Peña-Monné, Reference Turu, Peña-Monné, Pérez-Alberti and López-Bedoya2006). The Valira glacier partially eroded MIS 6 end moraines during the LGC, building multifold-nested and close-nested moraines (Turu et al., Reference Turu, Calvet, Bordonau, Gunnell, Delmas, Vilaplana, Jalut, Hughes and Woodward2017). Beyond, multiple far-flung end moraines correlated with the Segre-Valira staircase system of glaciofluvial terraces (Turu et al., Reference Turu, Boulton, Ros, Peña Monné, Martí Bono, Bordonau and Serrano2007). (d) The Gállego terminal complex centred around Senegüé (Turu et al., Reference Turu, Boulton, Ros, Peña Monné, Martí Bono, Bordonau and Serrano2007), the best-preserved end moraine (Lewis et al., Reference Lewis, Mcdonald, Sancho, Peña Monné and Rhodes2009). Unpublished dates (Supplementary Material) from the Gavin lateral moraine ridges (Serrano et al., Reference Serrano, Martínez de Pisón, González-Trueba, Turu and Constante2011) allow the reconstruction of glacial evolution behind and beyond Senegüé (Turu et al., Reference Turu, Hirsch, Peña-Monné, Raab, Ros, Martí-Bono, Constante-Orrios, Turu and Constante2011). (e) The Ribagorçana terminal complex around its best-preserved end moraine (Mey, Reference Mey1965; Vilaplana Reference Vilaplana1983a; Bordonau Reference Bordonau1992), the Seminari de Vilaller (Bordonau et al. Reference Bordonau, Vilaplana and Fontugne1993; Pallàs et al. Reference Pallàs, Rodés, Braucher, Carcaillet, Ortuno, Bordonau, Bourlès, Vilaplana, Masana and Santanach2006). Calibrated and recalculated dates (this work) from the lateral moraine ridges and kames behind the end moraine (Delmas et al. Reference Delmas, Gunnell, Calvet, Reixach, Oliva, Palacios, Hughes, García-Ruiz and Andres2021b). Glacial front in Pont de Suert (reconstructed). The ages of the glaciofluvial deposits (Peña-Monné et al. Reference Peña-Monné, Calvet, Turu, Turu and Constante2011) are from the archaeological data of Martínez-Moreno et al. (Reference Martínez-Moreno, Mora and Casanova2004) and Peña-Monné et al. (Reference Peña-Monné, Montes, Sampietro-Vattuone, Domingo, Medialdea, Bartolomé and Rubio Fernández2021).
No dates were found from the last interglacial (MIS 5e, the Eemian interglacial) (Fig. 16). Very few dates were also found by Lewis et al. (Reference Lewis, Mcdonald, Sancho, Peña Monné and Rhodes2009) in fluvial and glaciofluvial terraces from the SW Pyrenees that belong to the Eemian interglacial. However, deposits yielding MIS 6 ages are much more common. We can highlight the following examples within the Western Mediterranean Humid Period 6 and 5 (WMHP, Camuera et al., Reference Camuera, Ramos-Román, Jiménez-Moreno, García-Alix, Ilvonen, Ruha, Gil-Romera, González-Sampériz and Seppä2022): (1) the end moraine of Castiello de Jaca (171 ± 22 ka), a far-flung moraine from the Aragon glacier (García-Ruiz et al., Reference García-Ruiz, Martí-Bono, Peña-Monné, Sancho, Rhodes, Valero-Garcés, González-Sampériz, Constante, Turu and Constante2011, Reference García-Ruiz, Martí-Bono, Peña-Monné, Sancho, Rhodes, Valero-Garcés, González-Sampériz and Moreno2013; Fig. 1); (2) the glaciofluvial terrace in the upper Gállego valley at Sabiñanigo-Alto (155 ± 24 ka; 156 ± 10 ka; Lewis et al., Reference Lewis, Mcdonald, Sancho, Peña Monné and Rhodes2009; Fig. 1); (3) a terrace of the Cinca River (the westernmost tributary of the Segre) at Belver (171 ± 21; terrace Qt5, 180 ± 22; Lewis et al., Reference Lewis, Mcdonald, Sancho, Peña Monné and Rhodes2009), all belonging the WMHP-6; (4) the terrace at Arén in the Noguera Ribagorçana valley (Qt13 terrace, 131 ± 7 ka; Peña-Monné et al., Reference Peña-Monné, Montes, Sampietro-Vattuone, Domingo, Medialdea, Bartolomé and Rubio Fernández2021); and (5) in the lower Segre (Ebro depression at Lleida; Fig. 1), or the MIS 6 ages obtained in the fluvial Qt2 terrace (+80 m) by Stange et al. (Reference Stange, Van Balen, Carcaillet and Vandenberghe2013); belongs to the WMHP-5. From the northern slope of the Pyrenees, we highlight the Garonne (Fig. 1) terminal complex of La Serre (Fernandes et al., Reference Fernandes, Oliva, Palma, Ruiz-Fernández and Lopes2017), where an end-MIS 6 age from the WMHP-5 were reported by Fernandes et al. (Reference Fernandes, Oliva, Vieira, Palacios, Fernández-Fernández, Delmas, García-Oteyza, Schimmelpfennig and Ventura2021) for the outermost end moraine (MIE; Fig. 17b), and later glacial advances built a close-nested end moraine (Fig. 17b) ranging from >40 ka cal BP to MIS 2 around Lake Barbazan (Andrieu et al., Reference Andrieu, Hubschman, Jalut and Hérail1988).
Deposits that are beyond the range of the Qtz-OSL dating method (>80 ka) are found beneath the LMIE glacial front limits and show weathering according to their ages (Rixhon, Reference Rixhon2022). The best example in our area corresponds to the multi–glacial cycle nested moraine of La Margineda, where the early Würm end moraine (+65–75 m arb; 91 ± 9 ka, MIS 5b–5c; CMARG-4; Fig. 10e; Table 1) was surpassed by later glacial advances (Fig. 17c). In the NE Pyrenees (Niaux Cave, NE Pyrenees, 678 m; Fig. 1) an early Würm glacial progression has also been dated using U–Th methods (Bakalowicz et al., Reference Bakalowicz, Sorriaux and Ford1984), leading to the conclusion that the Ariège glacier was in the vicinity of the cave at ~95 ka, within the MIS 5d–5c interval (Sorriaux et al., Reference Sorriaux, Delmas, Calvet, Gunnell, Durand and Pons-Branchu2016), 13 km upstream from the MIS 6 terminal complex (Delmas et al., Reference Delmas, Calvet, Gunnell, Braucher and Bourlès2011). The onset of the LGC was probably synchronous on both sides of the Pyrenees because a similar age comes from the glaciofluvial terrace at Ur (Fig. 1, sector 4).
Deposits that are within the range of the Qtz-OSL dating method (<80 ka) belong to the LMIE or are found upstream from the LMIE glacial front limits, always weakly weathered. Our best example is the Sant Mamés melt-out till (Fig. 17d) from the MIS 5a–MIS 4 interval. The Sant Mamés melt-out till (73.9 ± 4.5 ka; VILALLER-2; Fig. 5, Table 1) can be correlated with the M2 end moraine from the Aragon glacier at Castiello de Jaca (SW Pyrenees; García-Ruiz et al., Reference García-Ruiz, Martí-Bono, Peña-Monné, Sancho, Rhodes, Valero-Garcés, González-Sampériz, Constante, Turu and Constante2011, Reference García-Ruiz, Martí-Bono, Peña-Monné, Sancho, Rhodes, Valero-Garcés, González-Sampériz and Moreno2013; Fig. 1). The Sant Mamés diamicton also correlates with the Sant Marcial (Fig. 1) glaciofluvial deposits of the Cinca glacier (SW Pyrenees; 76 ± 13 ka; Lewis et al., Reference Lewis, Mcdonald, Sancho, Peña Monné and Rhodes2009). Glaciers must then have retreated from their maximum positions, as a glaciofluvial terrace at Castiello (Fig. 1) gave an OSL age of 68 ± 7 ka, indicating a less extensive glacial front than during the LMIE (moraine m2, 51 ± 4.5 ka; García-Ruiz et al., Reference García-Ruiz, Martí-Bono, Peña-Monné, Sancho, Rhodes, Valero-Garcés, González-Sampériz, Constante, Turu and Constante2011, Reference García-Ruiz, Martí-Bono, Peña-Monné, Sancho, Rhodes, Valero-Garcés, González-Sampériz and Moreno2013). In the upper Cinca valley, OSL dates from glaciofluvial terraces gave ages of 63 ± 6 ka and 49.5 ± 3.9 ka (Salinas de Sin; Supplementary Material 8). To the east, the Sant Mamés melt-out till (Fig. 5) correlates with the oldest available exposure ages from the outermost Malniu moraine: 76.5 ± 7.1 ka, 10Be (GS-20), and 49.2 ± 4.5 ka, 10Be (GS-14), both dates falling within the MIS 5a–MIS 4 interval (Fig. 1, sector 4). However, Tomkins et al. (Reference Tomkins, Dortch, Hughes, Huck, Stimson, Delmas, Calvet and Pallàs2018, Reference Tomkins, Dortch, Hughes, Huck, Pallàs, Rodés and Allard2021) suggested that the LMIE in the Pyrenees was at 40.9 ± 1.1 ka (MIS 3) and coincided with the Hengelo interstadial. It seems unlikely that the LMIE coincided with the French archaeological Würm II–Würm III subdivision (Renault-Miskovsky and Girard, Reference Renault-Miskovsky and Girard1998). Evidence from glaciated mountains and ice sheets worldwide (Hughes et al., Reference Hughes, Gibbard and Ehlers2013) does not indicate more significant glacial advances in MIS 3 than during MIS 2 or MIS 4 (Allard et al., Reference Allard, Hughes and Woodward2021). The constrained LMIE age obtained by Tomkins et al. (Reference Tomkins, Dortch, Hughes, Huck, Stimson, Delmas, Calvet and Pallàs2018, Reference Tomkins, Dortch, Hughes, Huck, Pallàs, Rodés and Allard2021) is probably beyond the limits of their method (Moses et al., Reference Moses, Robinson and Barlow2014).
Unexpectedly, some of the deposits within the MIS 3 range of the AMS 14C-dating method (≈45 ka) are found in an inner position within the glacial valley far from the LMIE and LGM glacial front limits. We refer to these deposits as resulting from an ice or glacial thinning recession within the LGC and corresponding to the relevant palaeoenvironmental evidence from the northern foothills of the Pyrenees, which were occupied by the Neanderthals during the Châtelperronian, spanning from ca. 44.5 ka cal yr BP to 38.5 ka cal yr BP (Bricker, Reference Bricker2014). The archaeological site of Tambourets is only a few kilometres beyond Barbazan (Fig. 1), where a close-nested end moraine from the Garonne glacier is located (Andrieu et al., Reference Andrieu, Hubschman, Jalut and Hérail1988; Fernandes et al., Reference Fernandes, Oliva, Vieira, Palacios, Fernández-Fernández, Delmas, García-Oteyza, Schimmelpfennig and Ventura2021). Bricker (Reference Bricker2014) described archaeological remains within loess-type deposits from the beginning of Heinrich Event 4 (GS-10; Rasmussen et al., Reference Rasmussen, Bigler, Blockley, Blunier, Buchardt, Clausen and Cvijanovic2014). However, a brief episode of milder climate (Bricker, Reference Bricker2014) during GI-9 (Rasmussen et al., Reference Rasmussen, Bigler, Blockley, Blunier, Buchardt, Clausen and Cvijanovic2014) preceded the resumption of severe cold and dry conditions during stadial GS-9 (Rasmussen et al., Reference Rasmussen, Bigler, Blockley, Blunier, Buchardt, Clausen and Cvijanovic2014). We attempt to correlate this to the available sedimentary record from the southern slope of the Pyrenees as follows:
1. The highest flooding level in Ginebrosa (Fig. 8, unit 3), recorded after 42 ± 8 ka, may be related to an increase in ice-damming that we assign to stadial GS-11 (42,240–41,460 b2k; Rasmussen et al., Reference Rasmussen, Bigler, Blockley, Blunier, Buchardt, Clausen and Cvijanovic2014). Unit 4 (Fig. 8) indicates that the ice tongue thinned rapidly, probably during GI-10 (41,460–40,800 b2k; Rasmussen et al., Reference Rasmussen, Bigler, Blockley, Blunier, Buchardt, Clausen and Cvijanovic2014).
2. The Sia (SW Pyrenees; Serrano et al., Reference Serrano, Martínez de Pisón, González-Trueba, Turu and Constante2011) and Gavin lateral moraine complex (Fig. 1) was built (Fig. 17e) by the Gállego glacier at 42 ± 7 ka (49–35 ka; X1596; Supplementary Material 8). Guerrero et al. (Reference Guerrero, Gutiérrez, García-Ruiz, Carbonel, Lucha and Arnold2018) reported a major ice-thinning phase of the Gállego glacier ~41.53 ± 3.95 ka (OSL) from the Sextas earthflow (1630 m asl, SW Pyrenees; Fig. 1). The erstwhile Ara glacier thinned shortly after unblocking the Linas de Broto ice-dammed palaeolake (Sancho et al., Reference Sancho, Arenas, Pardo, Peña-Monné, Rhodes, Bartolomé, García-Ruiz and Martí-Bono2018; Bartolomé et al., Reference Bartolomé, Sancho, Benito, Medialdea, Calle, Moreno and Leunda2021).
Daura et al. (Reference Daura, Sanz, García, Allué, Vaquero, Fierro and Carrión2013) indicated a significantly arid climate during GS-9 in NE Iberia, matching the cold and dry climate reported at Tambourets by Bricker (Reference Bricker2014) and the recession mentioned above of the Gállego, Ara, and Valira glaciers. At the end of MIS 3 (ca. 39.7–34.2 ka cal BP; Turu et al., Reference Turu, Calvet, Bordonau, Gunnell, Delmas, Vilaplana, Jalut, Hughes and Woodward2017), the retreat of the Valira d'Orient glacier at Canillo (NE Andorra; 1530 m asl; Fig. 1) may have been responsible for an early triggering of the El Forn landslide (Corominas et al., Reference Corominas, Iglesias, Aguasca, Mallorquí, Fàbregas, Planas, Gili, Lollino, Giordan, Crosta, Corominas, Azzam, Wasowski and Sciarra2015). Between GI-10 and GI-7 (Rasmussen et al., Reference Rasmussen, Bigler, Blockley, Blunier, Buchardt, Clausen and Cvijanovic2014), glaciers receded synchronously at both extremities of the southern slopes of the Pyrenees.
On the northern slopes of the Pyrenees, the glacial recession has also been documented at Lourdes (Mardones and Jalut, Reference Mardones and Jalut1983) and Barbazan (Andrieu et al., Reference Andrieu, Hubschman, Jalut and Hérail1988). However, during the MIS 3–2 transition, the Garonne glacier again spread across Barbazan (36.2– 31.3 ka b2k, 29.5 −1.182/+1.38 ka BP; Andrieu, Reference Andrieu1991). On the southern slope of the Pyrenees, the late MIS 3 terminal complex of Sant Julià de Lòria (STJULIA-2 and STJULIA-1; Tables 1 and 2) correlates with the Senegüé (Fig. 17e) glacial phase in the upper Gállego (Lewis et al., Reference Lewis, Mcdonald, Sancho, Peña Monné and Rhodes2009).
In La Margineda (Fig. 10c), a set of colluvia (26.65 ± 0.3 ka cal BP; LM1; Table 3) were consolidated by the regrowth of the Andorra glacier when it reached its LGM positions 1 km downstream of La Margineda (Fig. 11). An early LGM (Clark et al., Reference Clark, Dyke, Shakun, Carlson, Clark, Wohlfarth, Mitrovica, Hostetler and McCabe2009) was registered in the southern slope of the Pyrenees by the buildup of the Tinabre lateral moraine (Fig. 2), and it is also evident from the buildup of the innermost lateral moraine ridge from Gavin (Fig. 17e) at 28 ± 3 ka (sample X1595; Supplementary Material 8). However, the LGM period (Clark et al., Reference Clark, Dyke, Shakun, Carlson, Clark, Wohlfarth, Mitrovica, Hostetler and McCabe2009) was quite unstable in the southern slope of the Pyrenees because the glacial recession and regrowth produced reworking and deformation of the sediments in the La Massana palaeolake (Turu et al., Reference Turu, Calvet, Bordonau, Gunnell, Delmas, Vilaplana, Jalut, Hughes and Woodward2017); slope instability in Andorra (VB05-30.2, 26.010–25.828 ka b2k, 21.52 ± 0.070 ka BP; Planas and Torrebadella, Reference Planas, Torrebadella, Hürlimann and Pinyol2022); moraine ridge duplication (Tomkins et al., Reference Tomkins, Dortch, Hughes, Huck, Pallàs, Rodés and Allard2021), as in the case of the Artigallonga moraines (Fig. 2); and the recurrence of ice-damming at Llestui (Fig. 2). Evidence from the late LGM period was also reported upstream of Sextas (Fig. 1) by Gonzalez-Sampériz et al. (Reference González-Sampériz, Valero-Garcés, Moreno, Jalut, García-Ruiz, Martí-Bono, Delgado-Huertas, Navas, Otto and Dedoubat2006), and for the Santa Coloma end moraine (Turu et al., Reference Turu, Boulton, Ros, Peña Monné, Martí Bono, Bordonau and Serrano2007, Reference Turu, Calvet, Bordonau, Gunnell, Delmas, Vilaplana, Jalut, Hughes and Woodward2017; Fig. 9).
Glacial tongue asymmetries within the LGM period (22.25 ± 4.25 ka; Clark et al., Reference Clark, Dyke, Shakun, Carlson, Clark, Wohlfarth, Mitrovica, Hostetler and McCabe2009) and between the LMIE and LGM phases occur between Pyrenean valleys; only in Cerdagne does the close-nested end moraine (Fig. 4, M1 and M2) from the Querol glacier seem to span from MIS 4 (Delmas et al., Reference Delmas, Gunnell, Braucher, Calvet and Bourlès2008; Calvet et al., Reference Calvet, Delmas, Gunnell, Braucher, Bourlès, Turu and Constante2011a) to MIS 2 (Pallàs et al., Reference Pallàs, Rodés, Braucher, Bourlès, Delmas, Calvet and Gunnell2010), highlighting that the LMIE and the LGM had similar glacial extents in the southeasternmost slope of the Pyrenees (Fig. 17a). However, this is not seen in the south-central Pyrenees, where an LGM end moraine (Fig. 17d) in the Seminari de Vilaller is far from the LMIE limits at Pont de Suert (Fig. 2). Subsequently, the final glacial retreat occurred toward the end of the last termination. In the Noguera Ribagorçana (Fig. 17d), it is represented by the end of the infilling of the overdeepened trough at around 15.7 ± 1.2 ka (VILALLER-1; Table 1), when the local glacial fronts were located at Santet and Bissiberri (Pallàs et al., Reference Pallàs, Rodés, Braucher, Carcaillet, Ortuno, Bordonau, Bourlès, Vilaplana, Masana and Santanach2006; Fig. 2; 10Be exposure ages recalculated, Supplementary Material 7). However, in the neighboring eastern valley, the Noguera de Tor (Fig. 2), the disappearance of cirque glaciers took place at 16.4–16.0 cal ka BP (Copons and Bordonau, Reference Copons and Bordonau1996), and only at ≈2200 m did small glaciers survive in this valley until 12.05 ± 0.4 ka (Tomkins et al., Reference Tomkins, Dortch, Hughes, Huck, Pallàs, Rodés and Allard2021). In the Valira d'Orient valley (Fig. 3), the glacial thickness was less than 250 m at 15.3 ± 1.1 ka, the timing of the triggering of the Encampadana sackung (1625 m asl; McCalpin and Corominas, Reference McCalpin and Corominas2019; Fig. 1). The retreat of the Valira d'Orient glacier from Canillo preceded the second triggering of the Forn landslide at 13.144 ± 0.175 ka cal BP (Turu and Planas, Reference Turu, Planas, Corominas, Romana, Hürliman and Alonso2005; Fig. 1, sector 3) and the blockage of the Valira d'Orient valley at Canillo (1530 m asl).
CONCLUSIONS
We distinguish between two types of terminal complexes, those in which there is at least one far-flung end moraine (Fig. 17d and e) and those in which there is not (Fig. 17b); however, both include nested moraines and/or a close-nested end-moraine complex (Fig. 17a and b). Close-nested end-moraine complexes encompass at least two glacial cycles: the LGC and the penultimate glacial cycle (Fig. 17b), and these moraines could be termed “multiple nested moraines” (Fig. 17c). Of note, the end moraines from the penultimate glacial cycle (MIS 6) are common in the whole range of the Pyrenees and were formed during the Western Mediterranean Humid Period 6 and 5.
The starting point for cooling in the LGC was generally at ~97 −15/+19 ka (Niaux, UR-1; Fig. 16), in MIS 5d, synchronous on both sides of the Pyrenees, and we refer to the early Würm (Fig. 16, EW). However, the MIS 5d Valira glacier front (Fig. 17c) was far from the LMIE far-flung moraine, and this could also be the case for other glaciated valleys in the Pyrenees (Fig. 17a).
The LMIE advance occurred at the beginning of MIS 4 (Fig. 16, VILALLER-2), after an early glacial recession (Fig. 16, EGR) after/following MIS 5d. Literature consultation enabled us to compare the glaciated Noguera Ribagorça valley with its neighbouring valleys, allowing the identification of a significant glacial thinning (Fig. 16, GTP) and glacial front retreat during the second half of MIS 3 (Fig. 16, GINEBROSA-1), during which glaciers thinned and receded or almost disappeared (no large valley glacial period [NLVGP]; Fig. 16). Evidence of this glacial ice thinning pertains to the Gállego glacier (SW Pyrenees) and almost disappeared in the Valira glacier (SE Pyrenees). It probably affected both slopes of the Pyrenees. However, the duration of the NLVGP was short on the eastern side of the mountain belt. Most of the nested moraines within the terminal complexes were formed during the latest part of MIS 3 (Fig. 16, STJULIA-2) and progressed during the LGM period of MIS 2 (Fig. 16, LM-1).
Within the terminal complexes incorporating multiple far-flung end moraines (Fig. 17c and d), the oldest end moraine could be beyond the range of luminescence dating (Fig. 17c); this applies to their linked terraces as well (Fig. 17a), like in the Noguera Pallaresa (Fig. 16, SORT-1) and at Cerdanya (Fig. 16, SANAV-1 and SANAV-2).
Acknowledgments
We wish to acknowledge Peter Mey, who was the first to produce modern geological cartography for the study area in which the Quaternary was differentiated by its sedimentological facies and which helped identify the maximum ice extension in the Noguera Ribagorzana glaciated valley. Marc Calvet is thanked for his participation in the fieldwork and fruitful discussions. We also acknowledge J.M. Vilaplana for his helpfulness in clarifying his Noguera Ribagorzana data. J. Bordonau is thanked for the scientific discussions about his Noguera Ribagorzana data, even for his disagreement with Llauset and Llestui diagnostics. We acknowledge P.D. Hughes, T. Lowell, and the first and third anonymous reviewers for their valuable comments, which helped to improve the paper. We recognise D. Booth, the senior editor, for encouraging us to produce a better submission at each step of the review.
Financial Support
This study was partially funded by the Fundação para a Ciência e Tecnologia (FCT) through FEDER (European Regional Development Fund) and COMPETE for 2020 (Operational Programme for Competitiveness and Internationalization) funds; project UID/MAR/04292/2020—MARE (Marine and Environmental Sciences Centre); and a sabbatical grant (ref. SFRH/BSAB/150395/2019) for PPC (Programa Operacional Capital Humano). This work is a contribution of the Primeros pobladores y Patrimonio Arqueológico del Valle del Ebro Aragon Research Group (Government and European Regional Development Fund) and Instituto Universitario de Investigación en Ciencias Ambientales de Aragón (IUCA). The project FINICES-PID2020-117685GB-I00 partially funded this study for RMC (University of Castilla–La Mancha, Spain). VT acknowledges a research grant (AM079-AND-2019) funded by the government of Andorra.
Supplementary Material
The Supplementary Material for this article can be found at https://doi.org/10.1017/qua.2022.68