Implication
This review article focuses on the exocrine function of the testis with particular emphasis on accumulated knowledge in cattle. The information described will be useful for scientists seeking basic knowledge of bull spermatogenesis.
Introduction
The testis is the site of two major functions: an exocrine function and an endocrine function. The exocrine function involves the production of highly differentiated gametes, the spermatozoa, released into the lumen of the seminiferous tubules and then stored in the epididymis. The endocrine function is performed by the Leydig cells, located in the interstitial compartment, outside the tubules, the main role of which is the production of steroids. In this review, we focus on the exocrine function of the testis, termed spermatogenesis, with particular focus on the accumulated knowledge in the bovine species.
Spermatogenesis is a finely regulated process of germ cell multiplication and differentiation leading to the production of male gametes, the spermatozoa released at the apical pole of Sertoli cells, the somatic component of the seminiferous tubules (Figure 1). Spermatogenesis can be divided into three parts: spermatocytogenesis, meiosis and spermiogenesis.

Figure 1 Seminiferous tubules in the bull testis viewed by brightfield microscopy. Seminiferous tubules are composed of myoid cells (MC), Sertoli cells (SC) and germ cells. In this stage V tubule, the germ cells include A spermatogonia (A), pachytene primary spermatocytes (P), secondary spermatocytes (SS), Sa (Sa) and Sd1 (Sd1) spermatids. Bar length equals 10 µm.
During spermatocytogenesis, stem germ cells engage in the spermatogenetic process by performing a first mitotic division that generates spermatogonia. The latter proliferate in turn by performing several successive mitotic divisions which result in the production of preleptotene spermatocytes. The preleptotene spermatocytes cross the blood-testis barrier and engage in meiotic prophase. During the prophase of the first division of meiosis, the germinal cells differentiate successively into different stages (leptotene, zygotene, pachytene, diplotene) before undergoing the two meiotic divisions. The first meiotic division is the reductional division (reduction of chromosome number, separation of homologous chromosomes), while the second meiotic division is the equational division (separation of the daughter chromatids). Meiosis therefore allows the production of round haploid spermatids. Spermiogenesis consists of the differentiation of round spermatids into spermatids at various degrees of elongation and finally into spermatozoa. Spermatozoa are released into the lumen of seminiferous tubules during a final stage called spermiation.
The cycle of the seminiferous epithelium
The notion of cycle of the seminiferous epithelium was already well established at the beginning of the 20th century, thanks in particular to the works of Brown (Reference Brown1885), Benda (Reference Benda1887), Von Ebner (Reference Von Ebner1888) and Regaud (Reference Regaud1901).
The seminiferous epithelium is composed of several generations of germ cells. This is due to the fact that the engagement of new generations of sperm cells, from spermatogonia to spermatozoa, continues throughout the inner surface of the membrane of the seminiferous tubes without waiting for the preceding generations to complete their evolution and to disappear as spermatozoa into the lumen of the tubules (Regaud, Reference Regaud1901). The fate of a generation of germ cells is closely related to the development of the other generations of neighboring cells in a section of seminiferous tubule. The result is the presence of cellular associations which follow one another in time, at a given point of the seminiferous tubule, in a perfectly regular order, characterizing the so-called cycle of the seminiferous epithelium. The consistency of these cellular associations results from two phenomena:
(i) At one point of the tubule, new spermatogonia begin their divisions and engage in spermatogenesis at time intervals of constant duration.
(ii) Once the germinal cells are involved in spermatogenesis, their rate of differentiation is always the same and each step has a fixed and constant duration.
The cell associations identified within a cycle of the seminiferous epithelium are used to divide the cycle into phases or stages allowing a chronological division of the latter. In the bull, the classification into eight stages, using spermiation as a reference point, serves as a reference (Curtis, Reference Curtis1918; Roosen-Runge and Giesel, Reference Roosen-Runge and Giesel1950; Ortavant, Reference Ortavant1959; Cupps and Laben, Reference Cupps and Laben1960; Amann, Reference Amann1962a; Hochereau, Reference Hochereau1967; Hochereau-de Reviers, Reference Hochereau-de Reviers1970) (Figure 2). However, there is a second classification (Clermont and Leblond, Reference Clermont and Leblond1955; Kramer, Reference Kramer1960; Berndston and Desjardins, Reference Berndston and Desjardins1974), using the meiotic divisions as a reference point, and based on the development of the acrosome during spermiogenesis (Figure 3), which follows the logic of the classifications proposed in rats (Leblond and Clermont, Reference Leblond and Clermont1952) and in mice (Oackberg, Reference Oackberg1956).
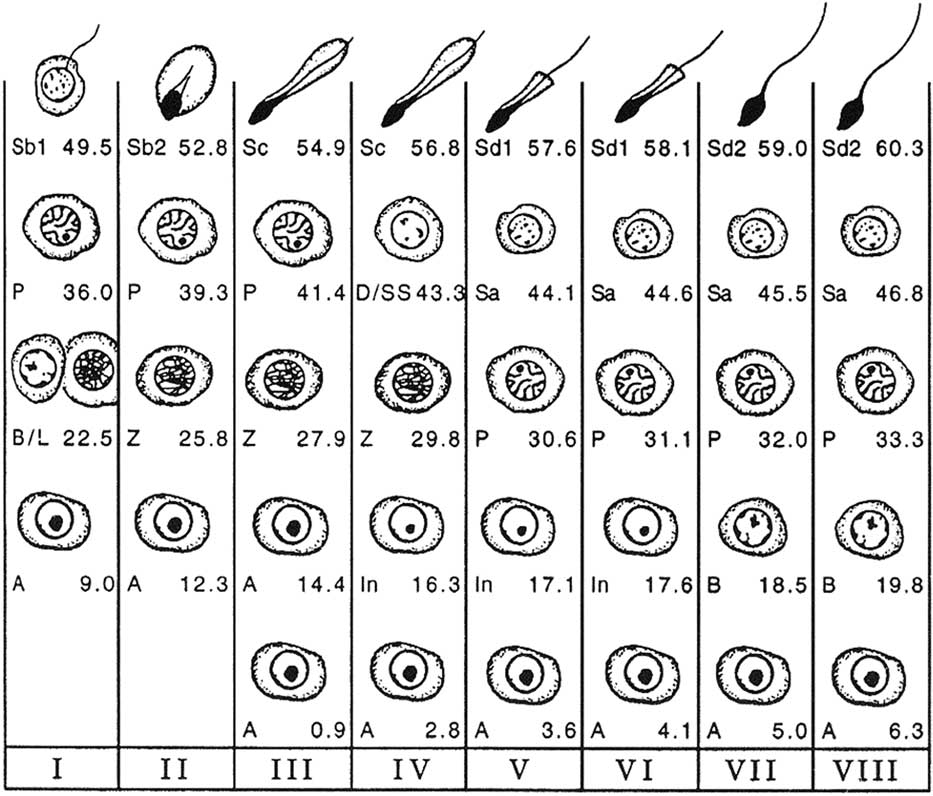
Figure 2 Cellular associations at each of the eight stages of the bovine seminiferous epithelium cycle (Roman numerals I to VIII) using spermiation as a reference point (end of stage VIII). The types of germ cells observed are A=type A spermatogonia; In=intermediate spermatogonia; B=type B spermatogonia; L=leptotene primary spermatocytes; Z=zygotene primary spermatocytes; P=pachytene primary spermatocytes; D=diplotene primary spermatocytes; SS=secondary spermatocytes; Sa=round spermatids; Sb1, Sb2, Sc, Sd1, Sd2=elongating and elongated spermatids at various steps of differentiation (from Amann, Reference Amann1962a).

Figure 3 Cellular associations at each of the 12 stages of the bovine seminiferous epithelium cycle (Roman numerals I to XII) based on the development of the acrosome during spermiogenesis. The types of germ cells observed are A=type A spermatogonia; In=intermediate spermatogonia; B1=type B1 spermatogonia; B2=type B2 spermatogonia; PL=preleptotene primary spermatocytes; L=leptotene primary spermatocytes; Z=zygotene primary spermatocytes; P=pachytene primary spermatocytes; II=secondary spermatocytes; 1 to 14=spermatids (from Berndston and Desjardins, Reference Berndston and Desjardins1974).
In bulls, the duration of the seminiferous epithelium cycle is 13.5 days. The total duration of spermatogenesis is 61 days, that is 4.5 times the duration of the cycle of the seminiferous epithelium. It can be divided into three parts, mitotic proliferation (also called spermatocytogenesis), meiosis and cytodifferentiation (also called spermiogenesis), whose respective durations are 21, 23 and 17 days.
The spermatogenetic wave
In contrast to the cycle of the seminiferous epithelium, which is a histological phenomenon occurring at a given place in the seminiferous tubule in time, the spermatogenetic wave is used to describe the spatial arrangement of cell associations along the tubules. As early as Reference Von Ebner1871, Von Ebner reported in the rat that the various cell associations are distributed according to a regular order which corresponds to the numerical order of the stages of the cycle. Spermatogenesis progresses along the seminiferous tubule in the manner of a wave, and therefore, it is referred to as the spermatogenetic wave.
Benda (Reference Benda1887) demonstrated the existence of the spermatogenetic wave in a large number of mammalian species (mice, guinea pigs, rabbits, rams, bulls, pigs, dogs and cats). Regaud (Reference Regaud1901) gave a description of the spermatogenetic wave that remained accepted by scientists for more than half a century: ‘The wave is to space what the cycle is to time.’ Curtis (Reference Curtis1918) confirmed the existence of the spermatogenetic wave in mice and rabbits and noted in these species that, from the junction between the tubules and the rete testis, the numerical order of the cell associations decreases to the point of inversion located at the bottom of the tube loop (but this organization is specific to rodents). He noted furthermore the presence of irregularities in this numerical succession but did not question the discovery of Von Ebner, unlike Cleland (Reference Cleland1951), who denied the existence of the spermatogenetic wave, thinking that the seminiferous tubule is divided in regions that operate autonomously. In 1961, Perey, Clermont and Leblond undertook a careful study of the spermatogenetic wave in the rat by examining complete seminiferous tubules reconstructed from serological histological sections. The authors confirmed that the cell associations are distributed along the tubules in the order corresponding to the numerical order of the stages of the cycle, but they noted numerous irregularities which they call ‘modulations’ (Figure 4). A modulation consists of a series of tubule segments having a numerical order of increasing first, then decreasing, until the increasing general progression is seen again. Despite these irregularities, a portion of a tubule occupied by a given cell association (corresponding to a given stage of the cycle) is always bordered by segments that are in the immediately preceding or subsequent stage. This is called ‘the continuity rule.’

Figure 4 Low power photograph of a longitudinally cut rat seminiferous tubule (magnification ×20). The portion of tubule between the two arrows constitutes a spermatogenic wave (from the proximal to the distal end of the tubule) with a modulation (shadowed segment) (from Perey et al., Reference Perey, Clermont and Leblond1961).
Perey et al. (Reference Perey, Clermont and Leblond1961) thus defined the spermatogenetic wave as ‘a sequence of segments showing the complete series of cell associations corresponding to the stages of the cycle of the seminiferous epithelium. One or more modulations must, however, be excluded from the sequence of the segments considered.’ The comparison of the characteristics of the cycle of the seminiferous epithelium and the spermatogenetic wave shows that the wave is not in space what the cycle is in time, contrary to what Regaud proposed in 1901. The wave is not a dynamic process but is a static way to describe in the spatial distribution of the associations along the tubule.
Kinetics of bull spermatogenesis
Listed is the description of the stages of the seminiferous epithelium in the bull from the classification established by Amann (Reference Amann1962a). There are eight spermatogenic stages in the bull:
Stage I is characterized by A spermatogonia, B spermatogonia or leptotene primary spermatocytes, pachytene primary spermatocytes and Sb1 spermatids.
Stage II is characterized by A spermatogonia, zygotene primary spermatocytes, pachytene primary spermatocytes and Sb2 spermatids.
Stage III is characterized by two generations of A spermatogonia, zygotene primary spermatocytes, diplotene primary spermatocytes (some diplotene primary spermatocytes can still be seen in stage IV) and Sc spermatids.
Stage IV is characterized by A spermatogonia, intermediate spermatogonia, zygotene primary spermatocytes (some zygotene primary spermatocytes can still be seen in stage V), secondary spermatocytes and Sc spermatids.
Stage V is characterized by A spermatogonia, intermediate spermatogonia, pachytene primary spermatocytes and two generations of spermatids (Sa and Sd1) (Figure 1).
Stage VI is characterized by A spermatogonia, intermediate spermatogonia (some intermediate spermatogonia can still be seen in stage VII), pachytene primary spermatocytes, and two generations of spermatids (Sa and Sd1).
Stage VII is characterized by A spermatogonia, B spermatogonia, pachytene primary spermatocytes, and two generations of spermatids (Sa and Sd2).
Stage VIII is characterized by A spermatogonia, two generations of B spermatogonia (B1 and B2), pachytene primary spermatocytes, and two generations of spermatids (Sa and Sb2 just before spermiation).
The duration of each of the eight stages of the seminiferous epithelium is summarized in Table 1.
Table 1 Time duration (in days) of the eight stages (Roman numerals I to VIII) of bull seminiferous epithelium, and stages at which the mitotic and meiotic divisions of the germ cells take place during the bull spermatogenesis.

M1=first meiotic division; M2=second meiotic division.
a Asymetric divisions where A1 spermatogonia produce A1 and A2 spermatogonia.
b Asynchronous divisions, some A spermatogonia (A) divide into intermediate spermatogonia, others (Ab) divide into A spermatogonia. The latter undergo a division into intermediate spermatogonia in late stage IV.
c Asynchronous divisions, some intermediate spermatogonia divide in stage VII, others in stage VIII.
d Asynchronous divisions, some B spermatogonia divide in stage VIII, others in stage I.
e Asymetric divisions where A2 spermatogonia produce A1 and A3 spermatogonia.
f Asymetric divisions where A0 spermatogonia produce A0 and A1 spermatogonia.
Spermatogonia renewal
Now that the basic notions of the kinetics of spermatogenesis have been reviewed, it is possible to address the complex problem of the renewal of the spermatogonial population. It is still difficult today to state with certainty the exact mode of renewal of spermatogonia in the bull testis. Despite numerous studies on the subject (Clermont and Leblond, Reference Clermont and Leblond1955; Roosen-Runge, Reference Roosen-Runge1955; Ortavant, Reference Ortavant1959; Kramer, Reference Kramer1960; Amann, Reference Amann1962a; Hochereau, Reference Hochereau1967; Hochereau-de Reviers, Reference Hochereau-de Reviers1970 and Reference Hochereau-de Reviers1971), several hypotheses remain. Yet, a number of recurring elements coincide and can be considered likely.
At least three criteria must be taken into account for the study of the renewal of spermatogonia: their rate of division, their rate of degeneration, and the presence of quiescent (resting) cells. These three criteria affect the number of cells in each category. The difference between the studies can be explained in part by the fact that the authors favored some criteria compared with others according to the methodology used. These methodologies include the use of radioelements incorporating into the pre-mitotic cells during the DNA synthesis phase (the duration of which is not equivalent for all types of spermatogonia; Hochereau, Reference Hochereau1967), morphological evolution of the different categories of spermatogonia (shape, size, nucleoli aspect), and stereological studies based on histological sections of testis at different stages of the seminiferous epithelium).
It is now established that there are six divisions allowing the proliferation of spermatogonia involved in spermatogenesis in the bull (Hochereau, Reference Hochereau1967), three for A spermatogonia, one for intermediate spermatogonia, two for B spermatogonia, even though Ortavant (Reference Ortavant1959) and Amann (Reference Amann1962b) only counted five. These divisions occur at precise stages of the seminiferous epithelium, but there are still some slight divergences on that point according to the authors (Table 1).
The second point of debate is the way in which spermatogonia are committed to renew themselves and/or engage in spermatogenesis. There, two theories depend on the presence or the absence of asymmetric divisions in the spermatogonia population (Figure 5). An asymmetric division is a cell division that generates two different daughter cells. In the schemes proposed by the French groups, asymmetric spermatogonial divisions are present. Indeed, in the model proposed by Ortavant (Reference Ortavant1959), the first spermatogonial mitotic division (from A1 spermatogonia), results in A1 spermatogonia and A2 spermatogonia. There is therefore a renewal of the population of A1 spermatogonia while the sister A2 spermatogonia continues its progression in spermatogenesis (Figure 5a).
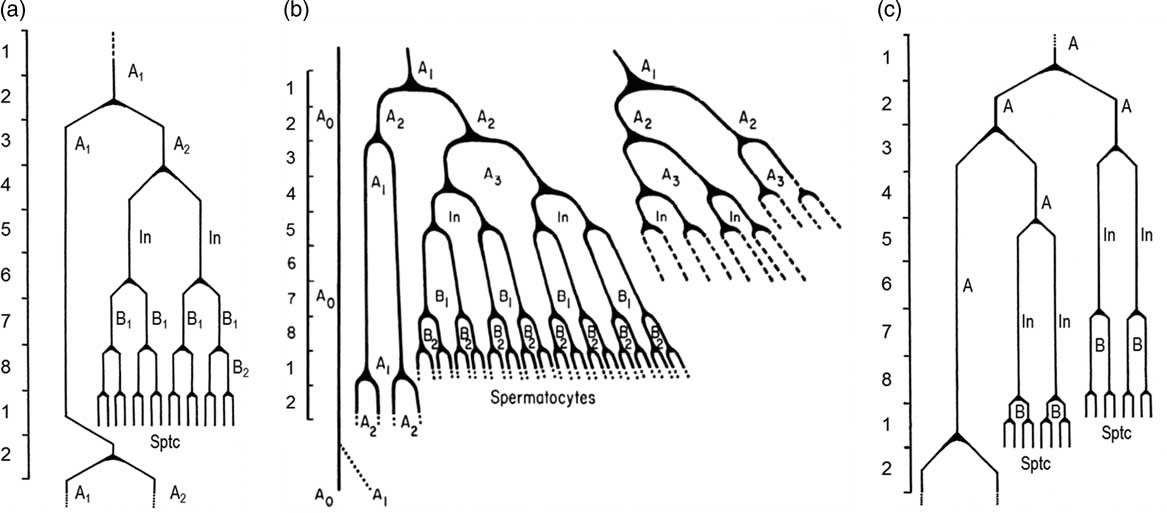
Figure 5 Model of spermatogonial renewal in the bull proposed by Ortavant (Reference Ortavant1959), Amann (Reference Amann1962b), and Hochereau-de Reviers (Reference Hochereau-de Reviers1971). The major difference comes from the presence of asymmetric mitotic divisions of A1 spermatogonia in the model proposed by Ortavant (a), of A0 and A2 spermatogonia in the model proposed by Hochereau-de Reviers (b), whereas these asymmetric divisions do not exist in the model proposed by Amann (c). On the other hand, Amann proposes an asynchrony of the mitotic divisions which is not found in the models proposed by Ortavant and Hochereau-de Reviers. The types of germ cells are A0=type A0 spermatogonia ; A1=type A1 spermatogonia; A2=type A2 spermatogonia; In=intermediate spermatogonia; B1=type B1 spermatogonia; B2=type B2 spermatogonia; Sptc=spermatocytes.
In the model proposed by Hochereau-de Reviers (Reference Hochereau-de Reviers1970 and Reference Hochereau-de Reviers1971), the second spermatogonial mitotic division (from A2 spermatogonia), results in A1 spermatogonia and A3 spermatogonia. Again, there is a renewal of the A1 spermatogonia population while A3 spermatogonia continues its progression in spermatogenesis. In addition, A0 spermatogonia are capable of generating A1 spermatogonia while maintaining a reserve of A0 spermatogonia during an asymmetric mitotic division (Figure 5b). In fact, it is very likely that both types of mitotic divisions (symmetric and asymmetric) coexist as it has been shown that an increase in the germ stem cell population is present long after puberty in the bull (Attal and Courot, Reference Attal and Courot1963).
In the schemes proposed by the Dutch (Kramer, Reference Kramer1960) and the American teams (Clermont and Leblond, Reference Clermont and Leblond1955; Roosen-Runge, Reference Roosen-Runge1955; Amann, Reference Amann1962b), asymmetric spermatogonial divisions do not exist. Therefore, a proportion of the A1 spermatogonia divide to renew the population of A1 spermatogonia and the remainder divides into A2 spermatogonia which engage in the spermatogenetic process. Moreover, Kramer (Reference Kramer1960) and Amann (Reference Amann1962b) propose a model of asynchronous spermatogonial generations (Figure 5c) which is not shared by Ortavant (Reference Ortavant1959) and Hochereau-de Reviers (Reference Hochereau-de Reviers1970, Reference Hochereau-de Reviers1971) and Hochereau-de Reviers et al. (Reference Hochereau-de Reviers, Monet-Kuntz and Courot1987) (Table 1).
Comparative efficiency of spermatogenesis across species
Spermatogenesis has been evaluated qualitatively by the general appearance of seminiferous tubules in histologic sections and quantitatively by differential cell counts. Given the life span and theoretical yield of a specific germ cell, a potential daily expression of spermatozoan production can be obtained from the number of germ cells of each type in the testis (Kennelly and Foote, Reference Kennelly and Foote1964; Amann, Reference Amann1970). Daily sperm production per gram of decapsulated testis is a measure of efficiency of spermatogenesis, and it is useful for species comparison (Amann et al., Reference Amann, Johnson, Thompson and Pickett1976). Humans have a much lower efficiency (4 to 6×106/g) of spermatogenesis (Johnson et al., Reference Johnson, Petty and Neaves1981) than other species, including rabbits (25×106/g), hamsters (24×106/g), rats (20 to 24×106/g), rhesus monkeys (23×106/g), boars (23×106/g), rams (21×106/g), stallions (16 to 19×106/g) and bulls (12×106/g)(Amann et al., Reference Amann, Johnson, Thompson and Pickett1976; Amann, Reference Amann1981; Johnson, Reference Johnson1986). The lower efficiency of spermatogenesis in human testes compared with other species results from a longer duration of spermatogenesis, longer cycle length and lower density of germ cells (Johnson, Reference Johnson1986). For instance, the percentage of the human testis occupied by seminiferous tubules and seminiferous epithelium is lower than that for rats, horses and bulls (Figure 6; Johnson, Reference Johnson1986).

Figure 6 Percentage of testicular parenchyma of the rat, horse, bull, and human occupied by seminiferous tubules or seminiferous epithelium (from Johnson, Reference Johnson1986).
Germ cell degeneration at specific developmental steps of spermatogenesis has been qualified by comparing daily sperm production/gram based on germ cell types in different steps of development on various species such as the human (Bar et al., Reference Bar, Moore and Paulsen1971; Johnson, Reference Johnson1986), rats (Johnson et al., Reference Johnson, Lebovitz and Samson1984), horse (Johnson, Reference Johnson1985), and bull (Amann, Reference Amann1962b; Amann et al., Reference Amann, Johnson, Thompson and Pickett1976) (Figure 7). While humans experience a 30% to 40% reduction in potential sperm production during the end of meiosis, there is no comparable loss or degeneration during meiosis of the stallion or bull. In the breeding season of the stallion, there is a larger number of A spermatogonia whose progeny are sustained, resulting in significant degeneration of B spermatogonia during spermatocytogenesis and the end of meiosis (Johnson, Reference Johnson1985). In the bull, a significant number of degenerated germ cells have been noted during spermatocytogenesis. A significant loss of about 30% occur between the steps of A and intermediate spermatogonia, a second phase of degeneration of about 30% occur at the steps of B1 and between B1 and B2 spermatogonia (Hochereau-de Reviers, Reference Hochereau-de Reviers1970; Berndston and Desjardins, Reference Berndston and Desjardins1974). Without these early phases of germ cells degeneration, bulls would have a daily sperm production around 30×106/g. Bulls have no significant germ cell loss during spermiogenesis (Johnson, Reference Johnson1986).

Figure 7 Daily sperm production per gram of testicular parenchyma based on different germ cell types in the rat, horse, bull and human (from Amann et al., Reference Amann, Johnson, Thompson and Pickett1976; modified from Johnson, Reference Johnson1986).
Age influence on spermatogenesis and reproductive performance
In addition to significant germ cell loss at the end of meiosis in younger men, older men experience additional germ cell degeneration during prophase of meiosis (Johnson, Reference Johnson1986). Loss of late leptotene, zygotene, and pachytene spermatocytes in older men was the major source of the aged-related decrease in daily sperm production whereas there appears to be no age-related difference during spermiogenesis in humans. Age-related reduction of bull fertility has been poorly described in the scientific literature. This is because the bulls in AI centers are replaced before experiencing a real decline in their fertility and a real testicular degeneration. Puberty is defined by Wolf et al. (Reference Wolf, Almquist and Hale1965) as the age at which the first ejaculate containing a minimum of 50 million sperm with at least 10% of motility is collected. This definition is still valid today; puberty is reached at 42 weeks on average but this age can vary from 38 to 46 depending on the breed (Lunstra et al., Reference Lunstra, Ford and Echternkamp1978). Because their spermatic efficiency continues to progress until full maturity, a bull is then able to produce enough spermatozoa for two useable ejaculates a day. In production, bulls are typically harvested three times a week for what seems to be a good compromise between the number of doses of semen produced and what is possible to manage for the AI center staff. With age, sperm production tends to decrease but the existence of fertile bulls has been reported until 19 years of age (Bishop, Reference Bishop1970).
Several hypotheses can be advanced regarding the decrease of spermatic potential in older individuals. Germ cell degeneration in meiotic prophase is certainly a way of eliminating cells with chromosomal abnormalities (Johnson, Reference Johnson1986), but this explanation is apparently not the only one. Germ cell degeneration may also be a mechanism limiting the number of cells that can be sustained by available Sertoli cells (Huckins, Reference Huckins1978) because the number of A spermatogonia is directly correlated with the number of Sertoli cells in many species (Hochereau-de Reviers and Courot, Reference Hochereau-de Reviers and Courot1978). The age-related decline in spermatogenic potential could therefore be explained by the decrease in the number of Sertoli cells.
Sertoli cells and puberty
Sertoli cells are named after Enrico Sertoli, who first described them in 1865 (Sertoli, Reference Sertoli1865). Sertoli cells are the main somatic component of the seminiferous tubules. Sertoli cells have a multi-lobed nucleus, a cytoplasm rich in endoplasmic reticulum, glycoproteins and cytoplasmic droplets, the amount of which is inversely proportional to spermatogenic development. Similarly, the cytoskeleton of Sertoli cells varies during the cycle of the seminiferous epithelium, which is indicative of the high plasticity of the Sertoli cell that is synchronized with the cyclic evolution of germ cells (Russell and Griswold, Reference Russell and Griswold1993). Sertoli cells are large pyramidal epithelial cells whose base is in contact with the basement membrane of the seminiferous tubes (which they produce with the peritubular cells) and whose apical part goes up to the level of the light of the seminiferous tubes. The Sertoli cells establish tight junctions between them at puberty, which contribute to the formation of the blood-testis barrier (Dym and Fawcett, Reference Dym and Fawcett1970). Several other types of cell junctions are present between Sertoli cells and germ cells such as lacunar or communicating junctions, adherent junctions (desmosome junctions and specialized junctions such as tubulobulbar complexes around spermatids) (Russell and Griswold, Reference Russell and Griswold1993).
Sertoli cells have many functions within the testicle; they constitute a barrier which prevents the free access of the blood components to the germinal cells located in the adluminal compartment of the testicle. The latter are then protected from possible toxic or mutagenic factors and an autoimmune reaction of the body to its own spermatozoa. It is probably also preventing loss of specific concentrations of androgen-binding protein (ABP), inhibin, activin, and enzyme inhibitors within the luminal compartment of the tubules (Garner and Hafez, Reference Garner and Hafez1980).
Sertoli cells contribute to the elaboration the adluminal fluid and create a specific ionic environment, essential for the differentiation of male germ cells toward the apical part of the seminiferous tubules (Setchell, Reference Setchell1970). They facilitate the passage of certain important compounds such as testosterone and glucose and more generally, they have a nurturing role toward the germinal cells and provide them with energetic substrates such as lactate and pyruvate that are necessary for the survival of the germinal cells (Jutte et al., Reference Jutte, Grootegoed, Rommerts and van der Molen1982). These functions are controlled by FSH (Jutte et al., Reference Jutte, Jansen, Grootegoed, Rommerts, Clausen and van der Molen1983).
Sertoli cells ensure the phagocytosis of degenerative germ cells and residual bodies and the spermiation of mature spermatids. Sertoli cells synthesize, under the control of FSH and androgens, a large number of proteins that they secrete toward the light of the seminiferous tubes, toward the germinal cells and the interstitial space (Lejeune et al., Reference Lejeune, Jégou, Carreau and Saez1996). To mention only the main ones, inhibin and activin play a role in the hypothalamic–pituitary axis in the release of gonadotropins, but they also act on the function of Leydig cells within the testis; ABP released under the influence of FSH and androgens, plays a role in the bioavailability of androgens, regulating spermatogenesis and sperm production (Ritzèn et al., Reference Ritzèn, Boitani, Parvinen, French and Feldman1982); transferrin, a glycoprotein that carries iron to iron-requiring cells, whose concentration in seminal plasma is directly linked to spermatic production and fertility (Gilmont et al., Reference Gilmont, Senger, Sylvester and Griswold1990; Ing et al., 2018). In addition, many growth factors are produced such as seminiferous growth factor which plays a role in the proliferation of spermatogonia cells, nerve growth factor which plays a role during the DNA synthesis phase of preleptoten spermatocytes, and insulin-like growth factor 1 (IGF-1) which acts on germ cell differentiation (Mauduit and Benahmed, Reference Mauduit and Benahmed1996).
Finally, Sertoli cells in co-culture with germ cells have shown to be able to stimulate RNA and DNA synthesis by the germ cells (Rivarola et al., Reference Rivarola, Sanchez and Saez1985). After a massive proliferation phase during fetal life, Sertoli cells gradually lose their ability to divide. In the bull, there is no further division of Sertoli cells 6 months after birth (Attal and Courot, Reference Attal and Courot1963), their number reaching a maximum at 28 weeks in Holstein bulls (Curtis and Amann, Reference Curtis and Amann1981). Sperm production is therefore directly correlated with the number of Sertoli cells fixed before puberty (Berndston et al., Reference Berndston, Igboeli and Parker1987). Failing to act on the efficiency of spermatogenesis after puberty, it is possible to hasten the onset of puberty by subjecting bulls to a high plane of nutrition during the first 6 months of life, optimizing the availability of saleable semen doses in young Holstein bulls (Dance et al., Reference Dance, Thundathil, Blondin and Kastelic2015) and Holstein-Friesen bulls (Byrne et al., Reference Byrne, Fair, English, Cirot, Staub, Lonergan and Kenny2018) without affecting semen quality (Dance et al., Reference Dance, Thundathil, Wilde, Blondin and Kastelic2016). Finally, some authors have also shown that it is possible to obtain mature Angus-Charolais bulls with greater testicular weight and sperm production by improving the nutrition during calfhood between 10 and 30 weeks of age (Brito et al., Reference Brito, Barth, Rawlings, Wilde, Crews, Mir and Kastelic2007).
Conclusion
Spermatogenesis is a long and orderly process through which spermatozoa are produced within seminiferous tubules and is divided into spermatocytogenesis (mitosis), meiosis, and spermiogenesis (differentiation without division). Spermatocytogenesis involves mitotic cell division to increase the yield of spermatogenesis and to produce stem cells and primary spermatocytes. Spermiogenesis involves an unsurpassed example of cell differentiation in the production of a self-propelled, penetrative enzyme-containing, and male genome delivery system, spermatozoa. Germ cell degeneration occurs throughout spermatogenesis, but is greater during spermatocytogenesis and meiosis and can vary with pubertal development, age, and species. Sertoli cell number is important in determining daily production of spermatozoa in bulls as well as in other species. The reason bulls have a lower efficiency of spermatogenesis than most species, other than humans, is not clear.
Acknowledgments
The authors thank Marie-Thérèse Hochereau-de Reviers for helpful discussion, Marie-Laure Touzé for bibliographic research, and Caitlin Edwards for English proofreading.
Declaration of interest
Both authors declare no conflict of interest and nor competing interest.
Ethics statement
Not applicable.
Software and data repository resources
Not applicable.