n-3 Fatty acids: structure, metabolic interrelationships, dietary sources and intakes
n-3 Fatty acids are a family of PUFA( Reference Burdge and Calder 1 ). They are defined by the position of the double bond closest to the methyl terminus of the hydrocarbon (acyl) chain. This is on carbon number three when counting the methyl carbon as number one. EPA (20:5n-3) and DHA (22:6n-3) appear to be the most important n-3 fatty acids( Reference Calder and Yaqoob 2 , Reference Calder 3 ), although roles for docosapentaenoic acid (DPA; 22:5n-3) are now also emerging( Reference Kaur, Cameron-Smith and Garg 4 , Reference Weylandt 5 ). Because of their long hydrocarbon chain, EPA, DPA and DHA are sometimes termed very long-chain n-3 fatty acids, in order to differentiate them from the 18-carbon plant-derived n-3 fatty acids like α-linolenic acid (ALA; 18:3n-3) and stearidonic acid (SDA; 18:4n-3). In the present paper, the term ‘very long-chain n-3 fatty acids’ will be used to individually and collectively describe EPA, DPA and DHA.
EPA, DPA and DHA are metabolically related to one another, and there is a pathway by which EPA can be synthesised from the simpler plant-derived n-3 fatty acids (Fig. 1). The conversion of ALA to EPA involves three steps catalysed, in turn, by delta-6 desaturase, elongase 5 and delta-5 desaturase (Fig. 1). Further conversion of EPA to DHA, via DPA, occurs by a complex pathway (Fig. 1) involving chain elongation catalysed by elongase 5, a second chain elongation catalysed by elongase 2 or 5, desaturation by delta 6-desaturase, and then removal of two carbon atoms by limited β-oxidation in peroxisomes. The enzymes of n-3 fatty acid interconversion are shared with the analogous n-6 fatty acid biosynthetic pathway of conversion of linoleic acid (18:2n-6) to arachidonic acid (ARA; 20:4n-6) and beyond. The high intake of linoleic acid relative to ALA in many Western diets( Reference Blasbalg, Hibbeln and Ramsden 6 ) favours linoleic acid conversion over that of ALA. This may be one explanation for the frequently reported low rate of conversion of ALA along this pathway( Reference Arterburn, Hall and Oken 7 , Reference Baker, Miles and Burdge 8 ), although this rate can be influenced by several other factors including age( Reference Bolton-Smith, Woodward and Tavendale 9 ), sex( Reference Childs, Romeu-Nadal and Burdge 10 – Reference Burdge and Wootton 12 ), hormones( Reference Vessby, Gustafsson and Tengblad 13 ) and genetics( Reference Koletzko, Lattka and Zeilinger 14 ).
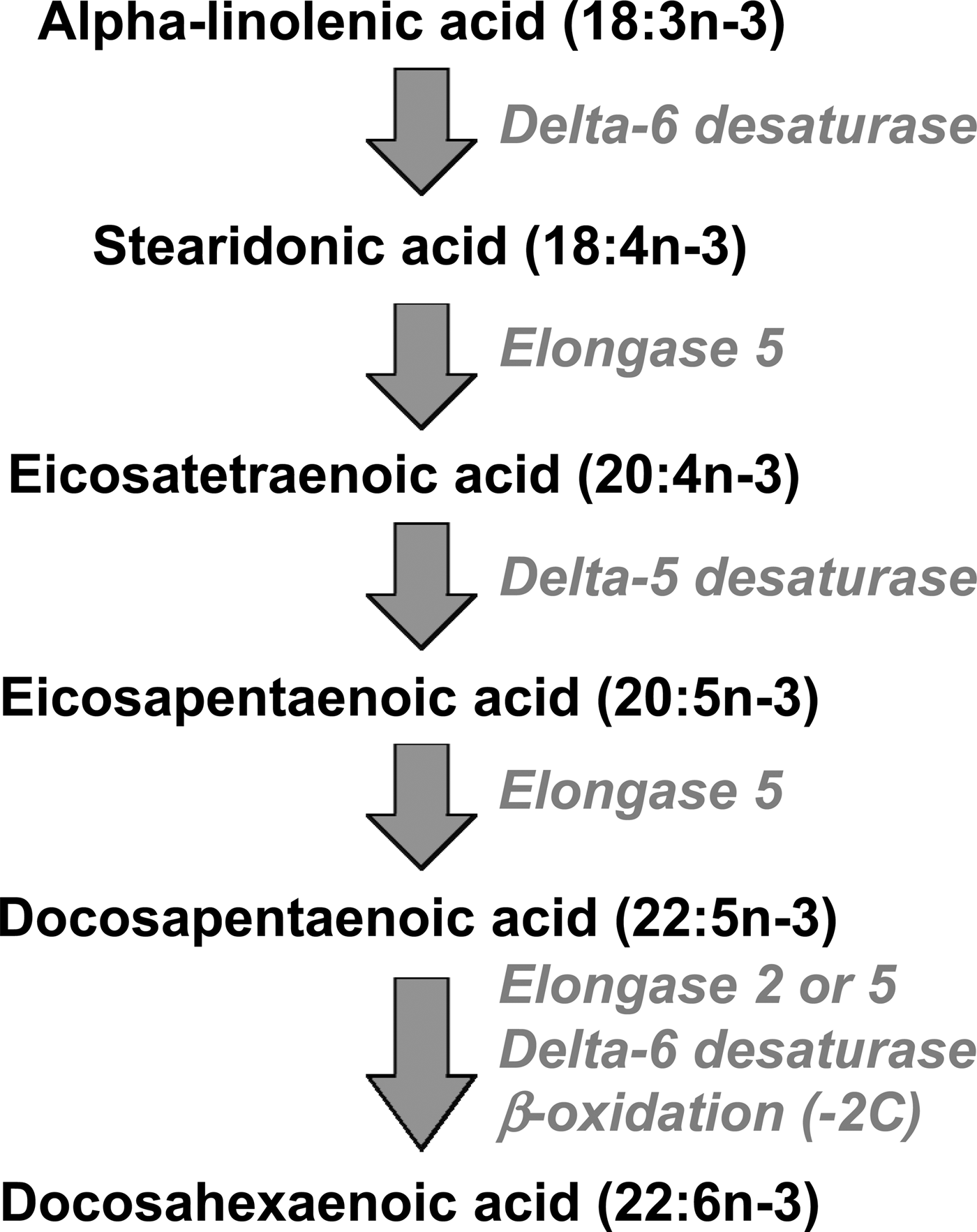
Fig. 1. The metabolic pathway of biosynthesis of EPA, docosapentaenoic acid and DHA.
EPA and DHA are found in fairly high amounts in most seafood, especially in fatty fish (sometimes called oily fish), in the blubber and tissues of sea mammals like whales and seals, in supplements like fish oils, cod liver oil and krill oil, in some algal oils, and in a limited number of pharmaceutical grade preparations( 15 , Reference Calder 16 ). There is at least a 10-fold range in content of these fatty acids per serving of seafood, with fatty fish (e.g. mackerel, salmon, trout, herring, tuna and sardines) being the richest source (Table 1). Hence, intake of very long-chain n-3 fatty acids is strongly influenced by fish consumption. In most Western countries only a relatively small proportion of the population regularly consume fatty fish. For example, in the UK it is estimated that only 25% of the adult population are regular fatty fish consumers( 17 ). Consequently intakes of very long-chain n-3 fatty acids are low in much of the population and mean intakes of EPA + DHA among adults in many Western populations are considered to be about 0·1–0·3 g/d( 17 ). However, it is difficult to be precise about this figure for several reasons, as discussed elsewhere( Reference Calder 3 ). A series of Australian studies in adults and children provide probably the most accurate data on intake of very long-chain n-3 fatty acids. Data from over 10 000 Australian adults identified mean daily intakes of EPA, DPA and DHA as 56, 26 and 106 mg, respectively, to give a total very long-chain n-3 fatty acid intake of 189 mg/d( Reference Meyer, Mann and Lewis 18 ), consistent with the oft-quoted average intake of these fatty acids among Western adults. However, median intakes of EPA, DPA and DHA were found to be only 8, 6 and 15 mg/d, respectively( Reference Meyer, Mann and Lewis 18 ). The large differences between mean and median intakes reflect the skewed distribution of the intake data. A more recent study using an updated nutrient composition database produced mean daily intake data for EPA, DPA and DHA of 75, 71 and 100 mg, respectively, in Australian adults, giving a mean total very long-chain n-3 fatty acid intake of 246 mg/d( Reference Howe, Meyer and Record 19 ). Again, median intakes were lower, being about 50% of the mean. The data suggest that 50% of Australian adults consume less than about 120 mg of very long-chain n-3 fatty acids daily. There is no reason to think that average intakes would be higher than this in other Western countries, and in many they may be lower. Australian children and adolescents aged 2–16 years consumed a mean of 79 mg/d EPA + DPA + DHA, with a median intake of 29 mg/d( Reference Rahmawaty, Charlton and Lyons-Wall 20 ). Intakes increased with age and were much higher in fish eaters than non-fish eaters( Reference Rahmawaty, Charlton and Lyons-Wall 20 ).
Table 1. Typical content of EPA, docosapentaenoic acid (DPA) and DHA (g/100 g food) in a selection of seafood and meat

Data are taken from ref. 15. Note that both very long-chain n-3 fatty acid content and portion size may vary. Modified from ref. Reference Calder3 with permission from John Wiley and Sons.
The oil used in many n-3 fatty acid supplements is sourced from fish; as such this may be referred to as fish oil. Other, non-fish, sources of oil such as krill and algae are also used in supplements. These different oils differ according to their very long-chain n-3 fatty acid content and the relative contributions of EPA and DHA (Table 2). Fig. 2 illustrates the estimated influence of daily consumption of a 1 g standard fish oil capsule, a 1 g ‘concentrated’ supplement, one teaspoon of cod liver oil, one meal of salmon and one or four capsules of a pharmaceutical grade preparation on daily intake of EPA + DHA. This shows that a person who does not consume fatty fish or n-3 fatty acid supplements can markedly increase their EPA + DHA intake through dietary change or through use of supplements.

Fig. 2. Typical intake of EPA + DHA from the background diet in an adult not regularly consuming fatty fish and what would be achieved by also consuming a 1 g standard fish oil (FO) capsule, a 1 g ‘concentrated’ supplement, one teaspoon of cod liver oil, one meal of salmon, or one or four capsules of the pharmaceutical grade preparation Omacor®. Reproduced from ref. Reference Calder3 with permission from John Wiley and Sons.
Table 2. Typical EPA and DHA contents of n-3 supplements

Reproduced from ref. Reference Calder16 with permission from John Wiley and Sons.
* Also known as Lovaza®.
Increased intake of EPA and DHA leads to increased content of EPA and DHA in blood, cells and tissues
As for all long-chain and very long-chain fatty acids, EPA and DHA are transported in the bloodstream esterified into TAG, phospholipids and cholesteryl esters as components of lipoproteins. They also occur in the bloodstream in the non-esterified form non-covalently bound to albumin. EPA and DHA are stored in adipose tissue esterified into TAG and they are found in all cell membranes esterified into phospholipids and related complex lipids. Cell membrane phospholipids and their fatty acid composition are important in determining the physical characteristics of cell membranes( Reference Stubbs and Smith 21 ), the manner in which membranes change in response to external stimuli( Reference Brenner 22 ) and the functional activities of membrane-bound proteins( Reference Murphy 23 ). EPA and DHA circulating in the bloodstream, stored in adipose tissue, and present in cell membranes may be regarded as transport, storage and functional pools, respectively. The contribution of EPA or DHA to the total fatty acids present within any of the transport, storage or functional pools differs according to the pool (Table 3)( Reference Browning, Walker and Mander 24 – Reference Safarinejad 36 ). DHA is most often present in a greater concentration than EPA (Table 3). This is especially true in specific regions of the eye and brain where DHA makes a significant contribution to the fatty acid complement and EPA is virtually absent( Reference Skinner, Watt and Besson 27 , Reference Makrides, Neumann and Byard 28 , Reference Crawford, Casperd and Sinclair 37 ). Within cell membranes, EPA and DHA are distributed differently among the different phospholipid components and in the brain and eye specific phospholipids are especially rich in DHA( Reference Crawford, Casperd and Sinclair 37 , Reference Anderson 38 ).
Table 3. Typical EPA and DHA concentrations reported in different lipid pools in human subjects

Data are taken from the selected references and are expressed as % of total fatty acids. Reproduced from ref. Reference Calder16 with permission from John Wiley and Sons.
* Blood collected after an overnight fast.
Increased intakes of EPA and DHA from fish or from supplements are reflected in increased concentrations (and proportions) of both fatty acids in blood lipids, blood cells and many tissues. This has been reported many times for total plasma and serum lipids and for the complex lipid components of plasma and serum (i.e. TAG, phospholipids and cholesteryl esters)( Reference Browning, Walker and Mander 24 , Reference von Schacky, Fischer and Weber 39 – Reference Popp-Snijders, Schouten and van Blitterswijk 47 ). It is also well described for erythrocytes( Reference Browning, Walker and Mander 24 , Reference Blonk, Bilo and Popp-Snijders 42 , Reference Katan, Deslypere and van Birgelen 43 , Reference Popp-Snijders, Schouten and van Blitterswijk 47 ), platelets ( Reference Browning, Walker and Mander 24 , Reference von Schacky, Fischer and Weber 39 , Reference Sanders and Roshanai 48 ) and leucocytes( Reference Browning, Walker and Mander 24 , Reference Healy, Wallace and Miles 26 , Reference Yaqoob, Pala and Cortina-Borja 44 – Reference Faber, Berkhout and Vos 46 , Reference Sperling, Benincaso and Knoell 49 ). When their intake is increased, the content of EPA and DHA increases in several human tissues, including skeletal muscle( Reference McGlory, Galloway and Hamilton 31 ), heart( Reference Harris, Sands and Windsor 29 ), gut mucosa( Reference Hillier, Jewell and Dorrell 35 , Reference Sorensen, Rasmussen and Aardestrup 50 ) and adipose tissue( Reference Browning, Walker and Mander 24 , Reference Katan, Deslypere and van Birgelen 43 ). There is a dose- and time-dependent pattern of incorporation of both EPA and DHA( Reference Browning, Walker and Mander 24 , Reference Healy, Wallace and Miles 26 , Reference von Schacky, Fischer and Weber 39 , Reference Katan, Deslypere and van Birgelen 43 – Reference Faber, Berkhout and Vos 46 ). Although EPA is incorporated more quickly than DHA( Reference Browning, Walker and Mander 24 , Reference Katan, Deslypere and van Birgelen 43 ), the precise pattern of EPA and DHA incorporation that occurs depends upon the specific nature of the lipid pool( Reference Browning, Walker and Mander 24 , Reference Katan, Deslypere and van Birgelen 43 ). Pools that are turning over rapidly show faster incorporation of EPA and DHA than slower turning over pools. For example, plasma lipids incorporate EPA and DHA more quickly than blood cells( Reference Browning, Walker and Mander 24 , Reference Katan, Deslypere and van Birgelen 43 ), and amongst blood cells, leucocytes have been usually shown to incorporate EPA and DHA more quickly than erythrocytes. However, dose- and time-dependence of EPA and DHA incorporation into tissues is not well investigated in human subjects, apart from for adipose tissue( Reference Browning, Walker and Mander 24 , Reference Katan, Deslypere and van Birgelen 43 ). Modification of human brain fatty acid composition is more difficult than for other tissues, especially beyond childhood.
The higher concentration of EPA and DHA achieved in various lipid pools through increased intake of EPA and DHA is maintained so long as the higher intake of EPA and DHA is maintained. If, after a period of increased intake of EPA and DHA, intake returns to the earlier lower level, then EPA and DHA concentrations decline, eventually returning to earlier levels. This is well described for blood lipids( Reference von Schacky, Fischer and Weber 39 , Reference Katan, Deslypere and van Birgelen 43 , Reference Yaqoob, Pala and Cortina-Borja 44 ), platelets( Reference von Schacky, Fischer and Weber 39 ), leucocytes( Reference Yaqoob, Pala and Cortina-Borja 44 ) and erythrocytes( Reference Katan, Deslypere and van Birgelen 43 , Reference Popp-Snijders, Schouten and van Blitterswijk 47 ). Just as the incorporation of EPA into different pools is faster than the incorporation of DHA, the loss of EPA is faster than the loss of DHA( Reference von Schacky, Fischer and Weber 39 , Reference Katan, Deslypere and van Birgelen 43 , Reference Yaqoob, Pala and Cortina-Borja 44 , Reference Popp-Snijders, Schouten and van Blitterswijk 47 ). The better retention of DHA than EPA may be because DHA is structurally and/or functionally preferred over EPA and that metabolic mechanisms have evolved to preserve it.
Molecular and cellular effects of increased EPA and DHA content
Many, though not all, of the functional effects of EPA and DHA are considered to require their incorporation into cell membrane phospholipids( Reference Calder 51 – Reference Calder 53 ) (Fig. 3). EPA and DHA are highly unsaturated and as a consequence they have been shown in some studies to decrease membrane order (i.e. increase membrane fluidity)( Reference Stubbs and Smith 21 , Reference Calder, Yaqoob and Harvey 54 ), although cells have mechanisms such as modifying membrane cholesterol content, to limit this effect. Through modulation of the physical properties of membranes, EPA and DHA provide a specific environment for membrane proteins like receptors, transporters, ion channels and signalling enzymes to function( Reference Brenner 22 , Reference Murphy 23 , Reference Miles and Calder 55 ). As a result, EPA and DHA can modulate cell responses that are dependent upon membrane protein function. This has been shown to be especially important in the eye where the presence of DHA enables optimal activity of the photoreceptor protein rhodopsin( Reference Niu, Mitchell and Lim 56 ). Cell membranes contain microdomains called rafts( Reference Pike 57 ). These have specific lipid and fatty acid compositions and act as platforms for receptor action and for the initiation of intracellular signalling pathways( Reference Pike 57 – Reference Yaqoob 59 ). EPA and DHA can modify raft formation in a variety of cell types including neurons, immune cells and cancer cells( Reference Yaqoob 59 – Reference Hou, McMurray and Chapkin 61 ), so affecting intracellular signalling pathways( Reference Yaqoob 59 – Reference Calder 62 ). As a result of their effects on membrane-generated intracellular signals, EPA and DHA can modulate transcription factor activation and, subsequently, gene expression patterns in a variety of cell types( Reference Calder 51 , Reference Miles and Calder 55 , Reference Calder 63 ). NF-κB ( Reference Novak, Babcock and Jho 64 ), the peroxisome proliferator activated receptors( Reference Krey, Braissant and L'Horset 65 , Reference Marion-Letellier, Savoye and Ghosh 66 ), and the sterol regulatory element binding proteins( Reference Clarke 67 – Reference Deckelbaum, Worgall and Seo 71 ) are amongst the transcription factors shown to be affected by EPA and DHA. The effects of EPA and DHA on transcription factor activation and modulation of gene expression are central to their role in controlling fatty acid and TAG metabolism, inflammation and adipocyte differentiation( Reference Calder 51 – Reference Calder 53 , Reference Miles and Calder 55 , Reference Calder 63 ).
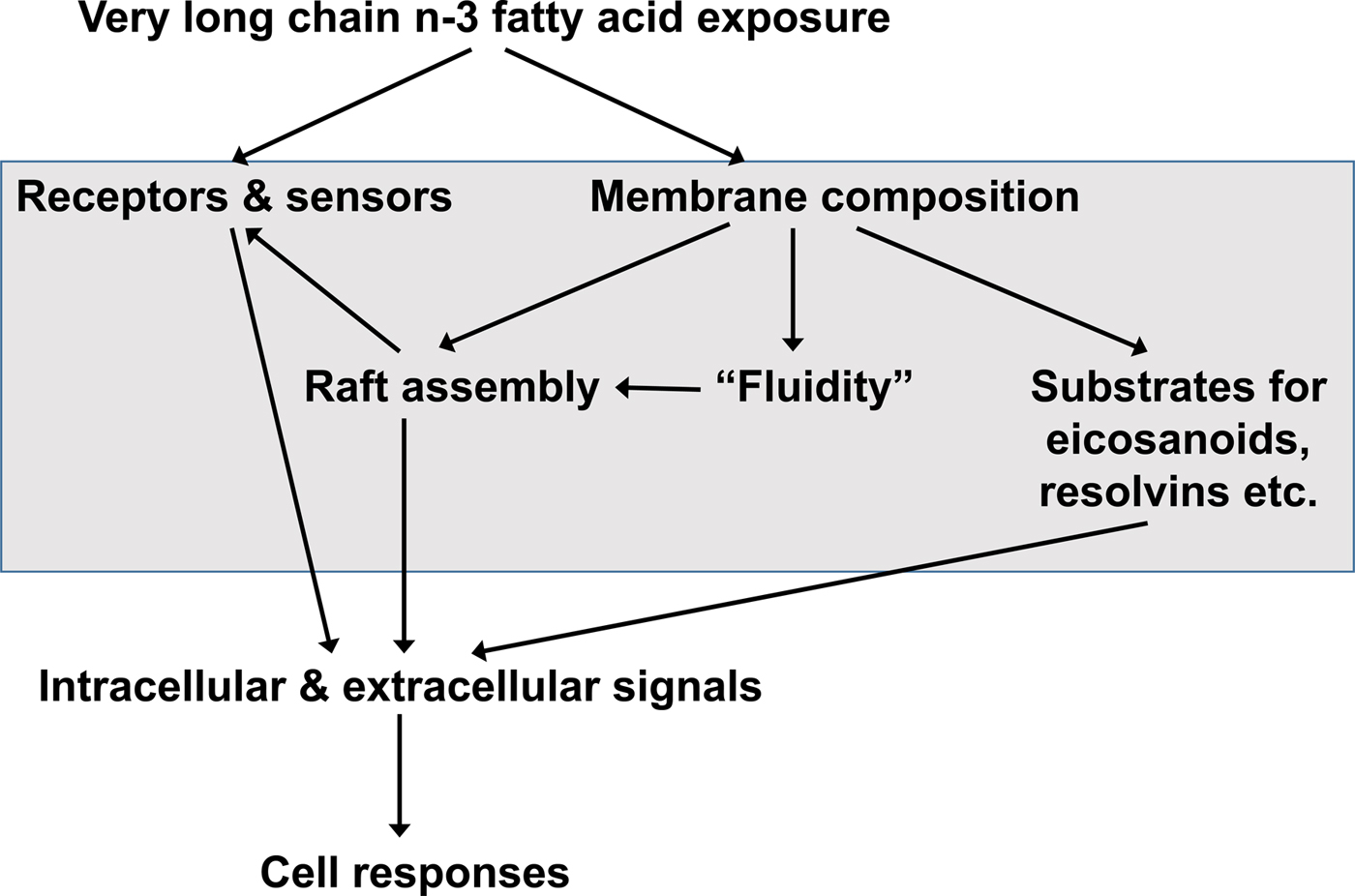
Fig. 3. General overview of the mechanisms by which very long-chain n-3 fatty acids can influence the function of cells. Modified from Calder( Reference Calder 72 ), Copyright (2011), with permission from Elsevier.
Increased abundance of EPA and DHA in cell membrane phospholipids is associated with decreased abundance of the n-6 PUFA ARA( Reference Healy, Wallace and Miles 26 , Reference von Schacky, Fischer and Weber 39 , Reference Yaqoob, Pala and Cortina-Borja 44 , Reference Rees, Miles and Banerjee 45 , Reference Calder 72 ). This alters the availability of substrates for synthesis of bioactive lipid mediators (Fig. 4). ARA is quantitatively the major substrate for the biosynthesis of various prostaglandins, thromboxanes and leukotrienes, together termed eicosanoids, which have well-established roles in regulation of inflammation, immunity, platelet aggregation, smooth muscle contraction and renal function. Eicosanoids are oxidised derivatives of 20-carbon PUFA and are produced via the cyclooxygenase (prostaglandins, thromboxanes), lipoxygenase (leukotrienes and other products) and cytochrome P450 pathways. Although they have a number of obvious important physiological roles, excess or inappropriate production of eicosanoids from ARA is associated with many disease processes( Reference Lewis, Austen and Soberman 73 , Reference Tilley, Coffman and Koller 74 ). Increasing the EPA and DHA content of cell membranes results in decreased production of eicosanoids from ARA( Reference von Schacky, Fischer and Weber 39 , Reference Rees, Miles and Banerjee 45 ), resulting in an impact of EPA and DHA on inflammation, immune function, blood clotting, vasoconstriction, and bone turnover amongst other processes. In addition to decreasing production of eicosanoids from ARA, EPA and DHA are themselves substrates for the synthesis of lipid mediators (Fig. 4). Some of these are simply analogues of those produced from ARA. For example, PG E3 produced from EPA is an analogue of PG E2 produced from ARA. Frequently, though not always, the EPA-derived mediator has weaker biological activity than the mediator derived from ARA( Reference Wada, DeLong and Hong 75 ). For example, thromboxane A3 produced from EPA is a much weaker platelet aggregator than thromboxane A2 produced from ARA( Reference Moncada and Vane 76 ). EPA and DHA are also substrates for more complex biosynthetic pathways that result in generation of mediators known as resolvins (E-series formed from EPA and D-series formed from DHA), protectins/neuroprotectins (formed from DHA) and maresins (formed from DHA)( Reference Bannenberg and Serhan 77 – Reference Serhan and Chiang 79 ) (Fig. 4). It has recently been discovered that DPA gives rise to a similar family of mediators( Reference Weylandt 5 , Reference Dalli, Colas and Serhan 80 ). The major role of resolvins, protectins and maresins appears to be in the resolution of inflammation and modulation of immune function( Reference Bannenberg and Serhan 77 – Reference Serhan and Chiang 79 ). It seems likely that many of the anti-inflammatory and immune modulating actions of EPA and DHA that are described in the literature( Reference Calder 52 , Reference Calder 53 , Reference Calder 72 , Reference Calder 81 ) are mediated through resolvins, protectins and maresins.
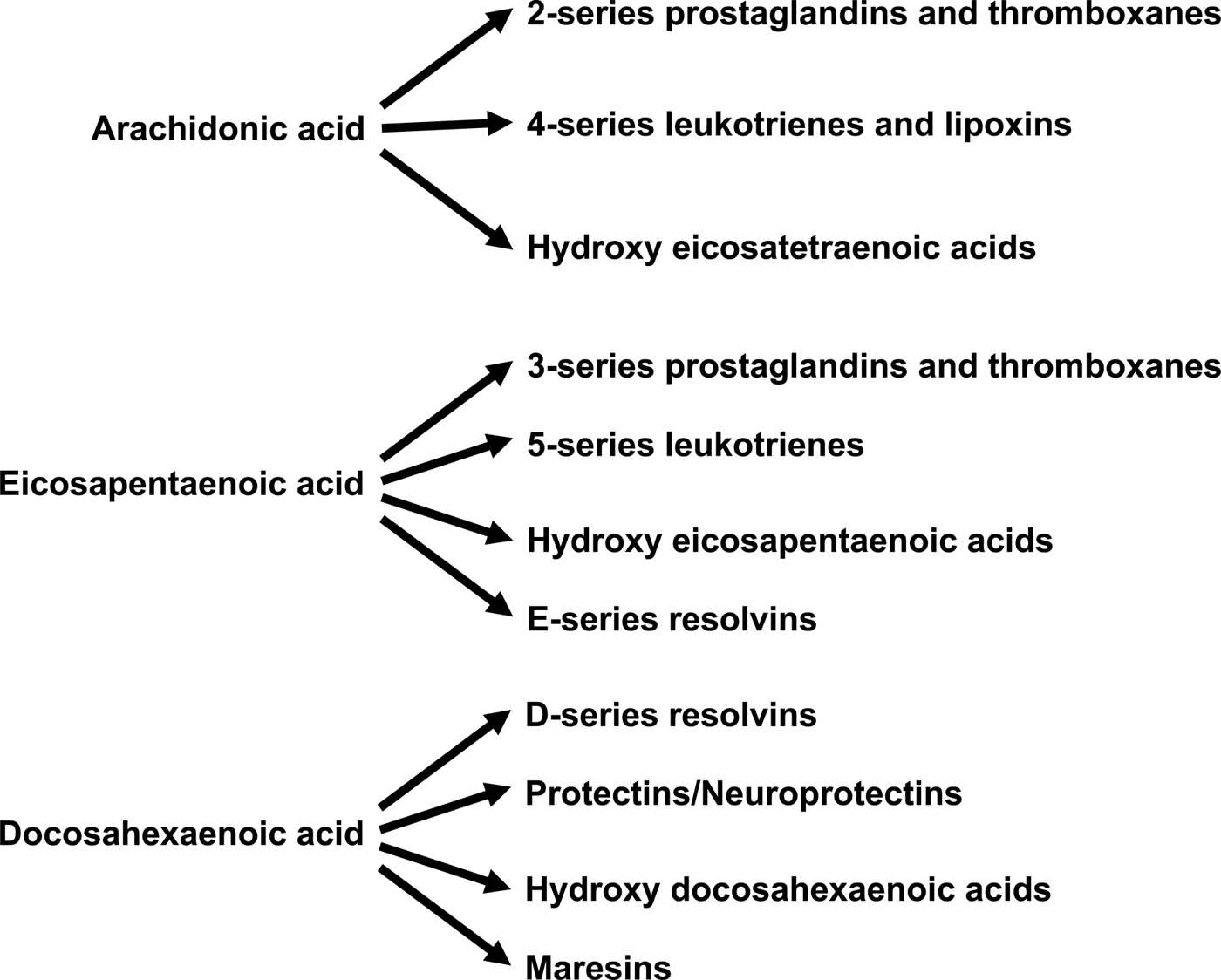
Fig. 4. Overview of the bioactive lipid mediators produced from arachidonic acid, EPA and DHA.
The above-mentioned mechanisms of action of EPA and DHA rely upon incorporation of those fatty acids into cell membrane phospholipids (Fig. 3). It is now recognised that EPA and DHA (in their non-esterified form) can also act directly via membrane G-protein coupled receptors that exhibit some specificity for very long-chain n-3 fatty acids over other fatty acids as ligands( Reference Oh, Talukdar and Bae 82 ). In particular, free fatty acid receptor 4 (also known as GPR120), which is highly expressed on inflammatory macrophages and on adipocytes, was shown in cell culture experiments to play a central role in mediating the anti-inflammatory effects of DHA on macrophages and the insulin-sensitising effects of DHA on adipocytes( Reference Oh, Talukdar and Bae 82 ).
Roles of EPA and DHA in supporting optimal cell and tissue function and promoting health
CVD
Native populations in Greenland, Northern Canada and Alaska consuming their traditional diet were found to have much lower rates of death from CVD than predicted, despite their high dietary fat intake( Reference Dyerberg, Bang and Stoffersen 83 – Reference Newman, Middaugh and Propst 86 ). The protective component of the diet was suggested to be the very long-chain n-3 fatty acids consumed in very high amounts as a result of the regular intake of seal and whale meat, whale blubber and fatty fish( Reference Bang, Dyerberg and Hjorne 87 ). Low cardiovascular mortality is also seen in Japanese consuming a traditional diet( Reference Yano, MacLean and Reed 88 ) and this diet is rich in seafood including fatty fish and sometimes marine mammals, which contain significant amounts of EPA and DHA. Much evidence has now accumulated from prospective and case–control studies indicating that higher intake of EPA and DHA is associated with reduced risk of CVD outcomes in Western populations, although not all studies agree. These studies have been summarised and discussed in detail elsewhere( Reference Kris-Etherton, Harris and Appel 89 – Reference Calder 93 ) and they have been subject to systematic review and meta-analysis. For example, Chowdhury et al. ( Reference Chowdhury, Warnakula and Kunutsor 94 ) brought together prospective studies examining the association of dietary or circulating fatty acids, including very long-chain n-3 fatty acids, with risk of coronary outcomes. Data from sixteen studies involving over 422 000 individuals showed a relative risk of 0·87 for those in the top third of dietary intake of very long-chain n-3 fatty acids compared with those in the lower third of intake. Data from thirteen studies involving over 20 000 individuals showed relative risks of 0·78, 0·79 and 0·75 for those in the top third of circulating EPA, DHA and EPA + DHA, respectively, compared with those in the lower third( Reference Chowdhury, Warnakula and Kunutsor 94 ). A smaller number of studies in fewer individuals gave a relative risk of 0·64 for circulating DPA( Reference Chowdhury, Warnakula and Kunutsor 94 ). A more recent analysis pooled data from nineteen studies that investigated the association between EPA or DHA concentration in a body compartment like plasma, serum, erythrocytes or adipose tissue and risk of future CHD in adults who were healthy at study entry( Reference Del Gobbo, Imamura and Aslibekyan 95 ). EPA and DHA were each associated with a lower risk of fatal CHD, with relative risks of 0·91 and 0·90, respectively( Reference Del Gobbo, Imamura and Aslibekyan 95 ). These analyses( Reference Chowdhury, Warnakula and Kunutsor 94 , Reference Del Gobbo, Imamura and Aslibekyan 95 ) support a clear role for EPA and DHA in primary prevention of CHD, and perhaps, more widely, of CVD, as discussed elsewhere( Reference Calder 96 ).
Beneficial modification of a broad range of risk factors probably explains the protective effect of very long-chain n-3 fatty acids towards CHD. These risk factors include plasma/serum TAG concentrations, blood pressure, thrombosis, cardiac function, vascular function and inflammation, which are all improved by very long-chain n-3 fatty acids( Reference Kris-Etherton, Harris and Appel 89 , Reference Calder 93 , Reference Harris 97 – Reference Leslie, Cohen and Liddle 101 ). A recent meta-analysis of randomised controlled trials (RCT) evaluated the effects of EPA + DHA on a range of risk factors for CVD( Reference AbuMweis, Jew and Tayyem 102 ); the findings are summarised in Table 4. Significant effects of EPA + DHA were identified for plasma/serum TAG and HDL cholesterol concentrations, systolic and diastolic blood pressure, heart rate and C-reactive protein concentration, a marker of inflammation (Table 4). There was also a significant elevation of LDL concentration (Table 4), but this apparently deleterious effect may be offset by an increased size of LDL particles( Reference Mori, Burke and Puddey 103 ), rendering them less atherogenic. The improvement in the risk factor profile with very long-chain n-3 fatty acids would account for the lowered risk of coronary disease reported in many previous epidemiological studies and identified in the recent meta-analysis( Reference Chowdhury, Warnakula and Kunutsor 94 ) and pooled analysis( Reference Del Gobbo, Imamura and Aslibekyan 95 ) of cohort studies.
Table 4. Summary of the effects of very long-chain n-3 fatty acids on risk factors for CVD identified through the meta-analysis of AbuMweis et al. ( Reference AbuMweis, Jew and Tayyem 102 )

A role in prevention of CVD is an obvious important health benefit of EPA + DHA. There has also been great interest in the ability of very long-chain n-3 fatty acids to treat people with existing CVD. The outcome in studies in this area has most often been the occurrence of a major cardiovascular event (e.g. myocardial infarction (MI)), fatal MI, or death. Several large studies published between 1989 and 2008 reported lower rates of death in patients receiving very long-chain n-3 fatty acids( Reference Burr, Fehily and Gilbert 104 – Reference Tavazzi, Maggioni and Marchioli 108 ). Doses of very long-chain n-3 fatty acids used in these studies were 500 mg to 1·6 g/d and durations of intervention were 1–5 years. As a result of these positive findings, meta-analyses published in the period 2002–2009 supported that very long-chain n-3 fatty acids lower mortality in patients with existing CVD( Reference Bucher, Hengstler and Schindler 109 – Reference Leon, Shibata and Sivakumaran 111 ). For example, a meta-analysis including eleven studies involving almost 16 000 patients reported that, compared with control, very long-chain n-3 fatty acids lower the risk of fatal MI, sudden death and all-cause mortality (relative risks 0·7, 0·7 and 0·8, respectively)( Reference Bucher, Hengstler and Schindler 109 ). A second meta-analysis including fourteen studies involving over 20 000 patients reported that, compared with control, very long-chain n-3 fatty acids lower the risk of cardiac mortality and all-cause mortality (relative risks 0·68 and 0·77, respectively)( Reference Studer, Briel and Leimenstoll 110 ). It is likely that the mechanisms that reduce the likelihood of cardiovascular events and mortality in patients with established disease are different from the mechanisms that act to slow the development of atherosclerosis. Three key mechanisms have been suggested to contribute to this therapeutic effect of very long-chain n-3 fatty acids. The first is altered cardiac electrophysiology seen as lower heart rate( Reference Harris, Miller and Tighe 112 ), increased heart rate variability( Reference Xin, Wei and Li 113 ) and fewer arrhythmias( Reference Leaf and Xiao 114 ). These effects make the heart more able to respond robustly to stress. The second is an anti-thrombotic action resulting from the altered pattern of production of eicosanoid mediators from ARA and from EPA that control platelet aggregation( Reference von Schacky, Fischer and Weber 39 ). This effect would lower the likelihood of clot formation or would result in weaker clots less able to stop blood flow to affected organs. The third mechanism is the well-documented anti-inflammatory effect of very long-chain n-3 fatty acids, which would serve to stabilise atherosclerotic plaques preventing their rupture( Reference Thies, Garry and Yaqoob 115 , Reference Cawood, Ding and Napper 116 ). This effect would reduce the likelihood of a cardiovascular event (MI, stroke) from happening( Reference Calder and Yaqoob 117 ). Thus, there is a biological plausibility to EPA + DHA having a benefit in secondary prevention of cardiovascular events and mortality.
Despite the positive findings with very long-chain n-3 fatty acids, supported by meta-analyses and biologically plausible candidate mechanisms, more recent studies in patients with existing CVD have failed to replicate the earlier findings( Reference Galan, Kesse-Guyot and Czernichow 118 – Reference Bosch, Gerstein and Dagenais 122 ). This has influenced some of the most recent meta-analyses, which have concluded that there is little protective effect of very long-chain n-3 fatty acids on cardiovascular mortality( Reference Kwak, Myung and Lee 123 – Reference Rizos, Ntzani and Bika 125 ). Nevertheless, the meta-analysis by Rizos et al. ( Reference Rizos, Ntzani and Bika 125 ) which included the recent studies, but excluded the landmark GISSI Prevenzione trial( 105 ), did identify a reduction in cardiac death with very long-chain n-3 fatty acids (relative risk 0·91) and trends towards reductions in sudden death and MI (relative risks 0·91 and 0·89, respectively). It is important to recognise that the most recent studies of very long-chain n-3 fatty acids and cardiovascular mortality have been criticised for various reasons related to small sample size, the low dose of EPA + DHA used, the too short duration of follow up, and, in some studies, the low rate of events( Reference Calder and Yaqoob 126 ). Despite this, meta-analyses that include the GISSI-Prevenzione study and some of the more recent neutral studies, still report benefits from very long-chain n-3 fatty acids( Reference Casula, Soranna and Catapano 127 , Reference Wen, Dai and Gao 128 ). For example, Casula et al.( Reference Casula, Soranna and Catapano 127 ) identified reductions in cardiac death, sudden death and MI (relative risks 0·68, 0·67 and 0·75, respectively) with very long-chain n-3 fatty acids and a trend towards lower all-cause mortality (relative risk 0·89). Likewise, Wen et al.( Reference Wen, Dai and Gao 128 ) identified reductions in cardiac death, sudden death, MI and all-cause mortality (relative risks 0·88, 0·86, 0·86 and 0·92, respectively) with very long-chain n-3 fatty acids.
Thus, there is a significant literature gathered over more than 45 years from association studies, from RCT investigating the impact on risk factors, and from RCT investigating the effect on hard clinical outcomes like mortality that very long-chain n-3 fatty acids lower the risk of developing CVD, especially CHD and can be used to treat people with CVD. Although the most recent RCT in patients with CVD have produced findings that do not agree with the previously accumulated literature, it is too early to discard the earlier evidence. Instead reasons to explain the different findings need to be identified, in order to better understand the actions of EPA and DHA. The conclusion that very long-chain n-3 fatty acids have a role in reducing risk of CVD, especially CHD, remains well supported, for example by the American Heart Association( Reference Kris-Etherton, Harris and Appel 89 , Reference Siscovick, Barringer and Fretts 129 ).
Cancer
EPA and DHA have a number of biological activities that may influence tumour cell viability and proliferation. For example, DHA can promote tumour cell apoptosis( Reference Gleissman, Johnsen and Kogner 130 , Reference Merendino, Costantini and Manzi 131 ), possibly through inducing oxidative stress. The replacement of ARA in cell membranes by EPA and DHA is also an important anti-cancer mechanism of action of n-3 PUFA, since ARA-derived eicosanoids like PG E2 and leukotriene B4 promote tumour cell proliferation, survival, migration and invasion( Reference Merendino, Costantini and Manzi 131 – Reference Vaughan, Hassing and Lewandowski 133 ). Through these effects, EPA and DHA can directly influence cancer cells and the tumour environment, and they can also influence the host response to tumour bearing. Recent reviews provide a detailed description of the mechanisms by which very long-chain n-3 fatty acids affect tumour cell proliferation, survival, invasion and metastasis( Reference Gleissman, Johnsen and Kogner 130 , Reference Merendino, Costantini and Manzi 131 ); the ability of very long-chain n-3 fatty acids to enhance the effectiveness of anti-cancer treatments( Reference Merendino, Costantini and Manzi 131 , Reference Vaughan, Hassing and Lewandowski 133 ); and the current evidence of the efficacy of very long-chain n-3 fatty acids in human subjects in the context of cancer and its treatment( Reference Merendino, Costantini and Manzi 131 , Reference Vaughan, Hassing and Lewandowski 133 ).
Some prospective and case–control studies show that very long-chain n-3 fatty acids are associated with lower risk of colorectal and breast cancers, but there is inconsistency in the findings from such studies( Reference Murphy, Mourtzakis and Mazurak 134 ). Recent systematic reviews conclude that very long-chain n-3 fatty acids are protective against colorectal( Reference Yang, Wang and Ren 135 ) and breast( Reference Makarem, Chandran and Bandera 136 ) cancers, while a fairly recent prospective cohort study among post-menopausal women found that higher dietary intake of either EPA or DHA was associated with lower risk of developing breast cancer( Reference Sczaniecka, Brasky and Lampe 137 ). Whether very long-chain n-3 fatty acids increase or decrease risk of prostate cancer is currently under debate( Reference Brasky, Darke and Song 138 , Reference Crowe, Appleby and Travis 139 ).
In addition to effects that lower the risk of developing some forms of cancer, there seems to be a role for very long-chain n-3 fatty acids in patients who already have cancer. For example, quality of life and physical functioning can be improved in cancer patients with oral provision of very long-chain n-3 fatty acids. A systematic review( Reference Elia, Van Bokhorst-de van der Schueren and Garvey 140 ) concluded that lung cancer patients receiving supplements containing EPA and DHA had improved appetite, energy intake, body weight and quality of life. Breast cancer patients with a higher concentration of very long-chain n-3 fatty acids in their bloodstream had lower inflammation and less physical fatigue than seen in patients with a lower concentration of very long-chain n-3 fatty acids( Reference Alfano, Imayama and Neuhouser 141 ). Lung cancer patients given 1·8 g EPA + DHA daily had improved appetite and less fatigue than controls( Reference Cerchietti, Navigante and Castro 142 ). Van der Meij et al.( Reference van der Meij, Langius and Spreeuwenberg 143 ) reported improved quality of life, physical function, cognitive function and health status in patients with non-small cell lung cancer receiving 2·9 g EPA + DHA per day. The patients receiving very long-chain n-3 fatty acids also tended to have greater physical activity compared with the control group( Reference van der Meij, Langius and Spreeuwenberg 143 ).
EPA and DHA sensitise cultured tumour cells to chemotherapeutic agents, increasing the efficacy of those agents( Reference Sala-Vila, Folkes and Calder 144 ). The mechanism by which this occurs is not clear, but it might involve increased EPA and DHA content of tumour cell membranes resulting in increased lipid peroxidation in those membranes in the presence of the cancer therapeutic. If this occurred in vivo it would result in improved efficacy of the therapy and perhaps reduced side effects. Murphy et al. ( Reference Murphy, Mourtzakis and Chu 145 ) conducted a trial in patients with non-small cell lung cancer and showed that 2·5 g EPA + DHA daily caused a 2-fold increase in response rate to the chemotherapy being used and prolonged the period over which patients could receive the chemotherapy. They also reported a trend towards improved survival with very long-chain n-3 fatty acids. Bougnoux et al.( Reference Bougnoux, Hajjaji and Ferrasson 146 ) reported improved chemotherapy outcomes in breast cancer patients receiving 1·8 g DHA daily.
Cancer cachexia (loss of lean and fat tissue) is a complication that occurs in patients with advanced solid tumors and greatly increases risk of mortality. Weed et al. ( Reference Weed, Ferguson and Gaff 147 ) reported that patients with squamous cell cancer of the head and neck taking 3·08 g EPA + DHA daily had increased lean body mass. Murphy et al.( Reference Murphy, Mourtzakis and Chu 148 ) showed that 2·2 g EPA + DHA daily was able to maintain body weight and muscle mass during chemotherapy in patients with non-small cell lung cancer. In other studies in patients with cancer, very long-chain n-3 fatty acids increased body weight( Reference Fearon, Barber and Moses 149 , Reference Guarcello, Riso and Buosi 150 ).
Thus, there is increasing evidence from studies in human subjects that very long-chain n-3 fatty acids have a role in reducing risk of developing some cancers, particularly colorectal and breast. Furthermore, a number of intervention trials demonstrate that very long-chain n-3 fatty acids have a range of benefits in patients with various types of cancer. Most intervention studies have used approximately 2 g/d EPA + DHA. From their review of the literature Vaughan et al.( Reference Vaughan, Hassing and Lewandowski 133 ) concluded ‘There is now sufficient literature to suggest that the use of supplements containing EPA and DHA may have potential use as an effective adjuvant to chemotherapy treatment and may help ameliorate some of the secondary complications associated with cancer…… our investigations indicate that supplementation with fish oil or EPA/DHA (>1 g EPA and >0·8 g DHA daily) is associated with positive clinical outcomes.’
Inflammation
Inflammation is an essential component of normal host defence mechanisms, initiating the immune response and later playing a role in tissue repair. The inflammatory response is normally self-limiting (i.e. resolving) in order to protect the host from damage. However, the loss of the normal mechanisms inducing tolerance (e.g. to self, to endogenous commensal micro-organisms, or to environmental components such as foods) or loss of resolving factors can allow inflammation to become chronic and in this state the damage done to host tissues may become pathological( Reference Calder, Albers and Antoine 151 , Reference Calder, Ahluwalia and Albers 152 ). As such, inflammation is the central adverse response seen in a range of conditions including rheumatoid arthritis (RA), inflammatory bowel disease, multiple sclerosis, asthma, psoriasis, and atopic dermatitis. Furthermore, chronic low-grade inflammation is now recognised to be a contributor to CVD( Reference Ross 153 , Reference Hansson and Hermansson 154 ) and to play a role in cardiometabolic diseases like obesity, type-2 diabetes and non-alcoholic fatty liver disease and in cognitive decline( Reference Calder, Ahluwalia and Brouns 155 ). Cancer also has a permissive inflammatory component( Reference Colotta, Allavena and Sica 156 ).
Eicosanoids and related lipid mediators provide a direct link between fatty acids and inflammatory processes. As described earlier, the n-6 PUFA ARA is the precursor for the production of prostaglandins and leukotrienes that are directly involved in inflammatory processes( Reference Lewis, Austen and Soberman 73 , Reference Tilley, Coffman and Koller 74 ). In contrast to the effects of ARA, EPA and DHA give rise to mediators which are less pro-inflammatory, anti-inflammatory or inflammation resolving( Reference Calder 52 , Reference Calder 53 , Reference Calder 72 , Reference Bannenberg and Serhan 77 – Reference Serhan and Chiang 79 , Reference Calder 81 ). In addition to their effects on lipid mediators (prostaglandins, leukotrienes, resolvins, protectins, maresins), EPA and DHA modulate many other aspects of inflammatory processes including leucocyte migration and production of inflammatory cytokines( Reference Calder 52 , Reference Calder 53 , Reference Calder 72 , Reference Calder 81 ). These effects on inflammation relate to the modulation of cell signalling, transcription factor activation and gene expression by EPA and DHA. There is good evidence that EPA and DHA given in combination at sufficiently high doses are anti-inflammatory and have a therapeutic role in inflammatory diseases. This has been most widely studied in RA( Reference Miles and Calder 157 ), inflammatory bowel disease( Reference Calder 158 ) and asthma( Reference Calder 159 ). Evidence of efficacy is strongest in RA, although high doses (several g/d EPA + DHA) are typically used( Reference Miles and Calder 157 ). Recent systematic reviews and meta-analyses have highlighted the benefits of EPA + DHA on arthritic pain( Reference Abdulrazaq, Innes and Calder 160 , Reference Senftleber, Nielsen and Andersen 161 ).
Allergic disease begins in infancy and the immune imbalances that predispose to allergy may be influenced by the relative exposure to n-6 and n-3 PUFA( Reference Calder, Kremmyda and Vlachava 162 ). Therefore, there has been significant interest in whether increased intake of very long-chain n-3 fatty acids by pregnant and breast-feeding women will reduce the risk of allergic disease in their babies. There is some evidence that increased intake of EPA and DHA during human pregnancy has an effect on the immune system of the baby( Reference Dunstan, Mori and Barden 163 – Reference Noakes, Vlachava and Kremmyda 165 ) and that this is linked to reduced allergic symptoms later in childhood( Reference Dunstan, Mori and Barden 163 , Reference Furuhjelm, Warstedt and Larsson 166 , Reference Palmer, Sullivan and Gold 167 ). Best et al. ( Reference Best, Gold and Kennedy 168 ) reported a meta-analysis of offspring clinical outcomes from trials of increased maternal intake of very long-chain n-3 fatty acids in pregnancy. They identified lower risk of atopic eczema, and less likelihood of having a positive skin prick test to any allergen tested, to hens’ egg, or to any food extract, all in the first 12 months of life. A recent study reported that fish oil consumption by pregnant women decreased risk of persistent wheeze and asthma in the offspring at ages 3–5 years( Reference Bisgaard, Stokholm and Chawes 169 ). Supplementing the diets of very young infants has also now been shown to have immune effects consistent with reduced likelihood of allergy ( Reference D'Vaz, Meldrum and Dunstan 170 ). This area of research has been reviewed recently( Reference Willemsen 171 – Reference Miles and Calder 173 ).
Thus, the anti-inflammatory actions of EPA and DHA are extensively demonstrated and the underlying mechanisms are increasingly understood. High doses of very long-chain n-3 fatty acids can be used to treat frank inflammatory conditions like RA, while lower doses likely have a role in protecting against development of childhood allergic disease and low-grade inflammatory conditions in adulthood.
Neurocognitive health
More than 50% of the dry weight of the brain is lipid, particularly structural and functional lipid (i.e. phospholipids). The human brain and retina contain an especially high proportion of DHA relative to other tissues but little EPA (Table 3). Grey matter phosphatidylethanolamine contains 24% of its fatty acids as DHA, whereas grey matter phosphatidylserine contains 37% of its fatty acids as DHA( Reference Lauritzen, Hansen and Jorgensen 174 ). DHA contributes 50–70% of the fatty acids present in the rod outer segments of the retina( Reference Anderson 38 ). These rod outer segments contain the eye's photoreceptors and DHA has been clearly shown to be essential for optimal visual functioning( Reference Niu, Mitchell and Lim 56 ). DHA is important for neurotransmission, neuronal membrane stability, neuroplasticity and signal transduction( Reference Lauritzen, Hansen and Jorgensen 174 – Reference Parletta, Milte and Meyer 176 ). An adequate supply of very long-chain n-3 fatty acids, especially DHA, seems essential for optimal visual, neural and behavioural development of the infant/child. The need for DHA so early in life was demonstrated in studies conducted in the 1980s and 1990s with pre-term infants, where formulas that included DHA (and often also ARA) were shown to improve visual development( Reference Uauy, Birch and Birch 177 – Reference Carlson, Werkman and Tolley 182 ). However, a recent meta-analysis of seventeen trials of inclusion of DHA (and ARA) in infant feeds involving 2260 preterm infants found little evidence to support improved visual acuity or neurodevelopment, although three out of seven studies of neurodevelopment did report some benefit of the PUFA( Reference Moon, Rao and Schulzke 183 ). Studies of DHA in preterm infants are discussed in detail elsewhere( Reference Molloy, Doyle and Makrides 184 ). The literature on the effect of DHA on visual and cognitive outcomes in term infants is mixed with some studies reporting benefits( Reference Agostoni, Trojan and Bellu 185 , Reference Willatts, Forsyth and DiModugno 186 ) and others not( Reference Scott, Janowsky and Carroll 187 – Reference Auested, Scott and Janowsky 190 ). One reason for this might be that an early beneficial effect of DHA is lost with time so that early assessments show benefit and later assessments do not; at least one study has shown this( Reference Agostoni, Trojan and Bellu 185 , Reference Agostoni, Trojan and Bellu 191 ). A recent meta-analysis of fifteen trials of inclusion of DHA (and ARA) in infant feeds involving 1889 term infants found little evidence to support improved visual or neurodevelopment, although four out of nine studies of visual acuity and two out of eleven studies of cognitive development reported benefit( Reference Jasani, Simmer and Patole 192 ). Despite the inconsistencies in the literature, it still seems important that pregnant and breastfeeding women and infants consuming formula instead of breast milk have adequate intakes of very long-chain n-3 fatty acids, especially DHA. A systematic review and meta-analysis of eleven RCT of very long-chain n-3 fatty acids in pregnancy involving over 5000 women could not conclusively support or refute that very long-chain n-3 fatty acids in pregnancy improve infant visual or cognitive development( Reference Gould, Smithers and Makrides 193 ).
Very long-chain n-3 fatty acids are likely to have important roles in the brain beyond infancy and are probably important for brain function throughout the life course. A number of studies have reported lower levels of EPA and DHA in the bloodstream of children with attention deficit hyperactivity disorder (ADHD) or autistic spectrum disorders than in control children( Reference Richardson 194 ). It is possible that these and other developmental disorders might be related to some sort of fatty acid deficiency state. If this is the case, then normalisation of fatty acid levels should lead to clinical benefit in these conditions. This has been examined in a number of trials in children and adolescents with attention, learning, or behavioural disorders, some showing some improvements( Reference Stevens, Zhang and Peck 195 – Reference Sheppard, Boone and Gracious 205 ) and others finding no effect( Reference Voigt, Llorente and Jensen 206 – Reference Mankad, Dupuis and Smile 214 ). These trials have been reviewed many times, with differing conclusions. The different findings may relate to the dose of n-3 fatty acids used, the duration of supplementation, the precise outcome(s) measured and differences in the children studied. Indeed, one review concluded that studies using higher doses of very long-chain n-3 fatty acids or of longer duration or in children/adolescents with low socioeconomic status were more likely to find effects( Reference Frensham, Bryan and Parletta 215 ). A recent meta-analysis of five RCT involving 189 children with autism spectrum disorder identified benefit of very long-chain n-3 fatty acids on some outcomes, but concluded that the limited number of studies and small sample sizes restrict the ability to make firm conclusions( Reference Horvath, Łukasik and Szajewska 216 ). Another recent meta-analysis used data from fifteen case–control studies involving 1193 individuals with autism to show lower blood EPA, DHA and ARA and higher ratio of n-6 to n-3 PUFA in autism( Reference Mazahery, Stonehouse and Delshad 217 ). Data from four RCT involving 107 individuals with autism showed improvements in some outcomes with very long-chain n-3 fatty acids, but the authors suggested the need for larger and longer studies in order to be clear about the effect( Reference Mazahery, Stonehouse and Delshad 217 ). A meta-analysis of ten RCT of very long-chain n-3 fatty acids in children with ADHD showed no improvements in measures of emotional lability, oppositional behaviour, conduct problems or aggression( Reference Cooper, Tye and Kuntsi 218 ). However, subgroup analyses of higher quality studies and those meeting strict inclusion criteria found a significant reduction in emotional lability and oppositional behaviour( Reference Cooper, Tye and Kuntsi 218 ). The authors concluded that a number of treatment effects may have failed to reach statistical significance due to small sample sizes and within and between study heterogeneity in terms of design and study participants( Reference Cooper, Tye and Kuntsi 218 ). In seven RCT involving 534 adolescents with ADHD, very long-chain n-3 fatty acids improved ADHD clinical symptom scores, while in three RCT involving 214 adolescents, very long-chain n-3 fatty acids improved cognitive measures associated with attention( Reference Chang, Su and Mondelli 219 ). A very recent systematic review was supportive of use of n-3 fatty acid supplements in children with ADHD( Reference Derbyshire 220 ).
Over 35 years ago Rudin suggested that mental disorders might result from a deficiency in very long-chain n-3 fatty acids and might respond to provision of these fatty acids( Reference Rudin 221 ). Data from nine countries demonstrated a significant correlation between high annual fish consumption and lower prevalence of major depression( Reference Hibbeln 222 ), an observation that is compatible with a proposed protective effect of very long-chain n-3 fatty acids. A reduction in depressive symptoms was reported in a small study using a very high dose of EPA + DHA (9·6 g/d)( Reference Su, Huang and Chiu 223 ), while this effect was not seen in a study using a lower dose of DHA alone (2 g/d)( Reference Marangell, Martinez and Zboyan 224 ). Intervention with 6·2 g/d EPA + DHA in patients with bipolar manic depression resulted in significant improvements in nearly all outcomes, especially with respect to depressive symptoms, after 4 months( Reference Stoll, Severus and Freeman 225 ). Likewise, 2 g/d EPA improved symptoms in patients with unipolar depressive disorder after 4 weeks( Reference Nemets, Stahl and Belmaker 226 ). A meta-analysis of thirteen RCT involving 1233 participants with major depressive disorder showed an overall beneficial effect of very long-chain n-3 fatty acids on depressive symptoms( Reference Mocking, Harmsen and Assies 227 ); interestingly higher EPA dose was one factor associated with better outcome for n-3 PUFA supplementation. This finding fits with an earlier analysis of fifteen RCT investigating the effects of EPA, which concluded that supplements containing ≥60% EPA in doses ranging from 0·2 to 2·2 g/d EPA were effective against primary depression( Reference Sublette, Ellis and Geant 228 ). There is also evidence from meta-analysis that depressive symptoms seen in bipolar disorder may be improved by the adjunctive use of very long-chain n-3 fatty acids( Reference Sarris, Mischoulon and Schweitzer 229 ).Another meta-analysis that included twenty-five studies involving 1373 participants identified a ‘small-to-modest benefit’ for depressive symptomology with very long-chain n-3 fatty acids( Reference Appleton, Sallis and Perry 230 ) but the authors expressed doubts about the robustness of their finding.
Schizophrenic patients have lower levels of EPA and DHA in their erythrocytes than do controls( Reference Peet, Laugharne and Rangarajan 231 – Reference Hoen, Lijmer and Duran 235 ). The first trial of very long-chain n-3 fatty acids in schizophrenia identified clinical improvement with EPA (2 g/d), but not with DHA( Reference Peet, Brind and Ramchand 236 ), while subsequent trials also showed benefit with EPA( Reference Peet and Horrobin 237 , Reference Fenton, Dickerson and Boronow 238 ), but not all studies have seen this( Reference Emsley, Myburgh and Oosthuizen 239 ). A recent study reported significant benefits of very long-chain n-3 fatty acids given for 26 weeks to patients with schizophrenia( Reference Pawełczyk, Grancow-Grabka and Kotlicka-Antczak 240 ). Although these findings are encouraging, a Cochrane review concluded that very long-chain n-3 fatty acids should be regarded only as an experimental treatment for schizophrenia( Reference Joy, Mumby-Croft and Joy 241 ). A study reported significant benefit from 1 g/d EPA in borderline personality disorder( Reference Zanarini and Frankenburg 242 ), while a small number of studies report anti-aggressive effects of DHA( Reference Hamazaki, Sawazaki and Itomura 243 , Reference Hamazaki, Thienprasert and Kheovichai 244 ).
Post-mortem studies showed that the brains of people with Alzheimer's disease contain less DHA than those without the disease( Reference Prasad, Lovell and Yatin 245 – Reference Soderberg, Edlund and Kristensson 248 ) and some studies have linked low blood levels of very long-chain n-3 fatty acids to dementia( Reference Conquer, Tierney and Zecevic 249 ). Sinn et al. reported that 1·8 g EPA + DHA daily for 6 months reduced depressive symptoms and improved cognition in adults with mild cognitive impairment( Reference Sinn, Milte and Street 250 ). Improved memory performance in subjects with mild Alzheimer's disease was reported with 1·5 g EPA + DHA daily for 6 months( Reference Scheltens, Twisk and Blesa 251 ). However, a number of other studies using various doses and ratios of EPA and DHA reported no effect on cognitive performance in people with Alzheimer's disease( Reference Quinn, Raman and Thomas 252 – Reference Phillips, Childs and Calder 257 ). Meta-analyses provide a mixed view of the findings for n-3 fatty acid treatment in the area of cognitive impairment, reflecting the mixed findings from individual trials. A Cochrane review of RCT studying the role of very long-chain n-3 fatty acids in preventing cognitive decline in healthy older people showed no benefits( Reference Sydenham, Dangour and Lim 258 ), while two more recent meta-analyses differ in their findings. One meta-analysis of six RCT of duration 3–40 months and using 0·l4–1·8 g EPA + DHA daily identified a slower rate of cognitive decline in those receiving very long-chain n-3 fatty acids( Reference Zhang, Hou and Li 259 ). In contrast, a meta-analysis of three RCT involving 632 participants with mild to moderate Alzheimer's disease and followed for 6, 12 or 18 months found no evidence of a benefit from very long-chain n-3 fatty acids on any outcome that was assessed( Reference Burckhardt, Herke and Wustmann 260 ). A recent large trial found no effects of very long-chain n-3 fatty acids (0·225 g EPA plus 0·8 g DHA daily) over 3 years on cognitive decline( Reference Andrieu, Guyonnet and Coley 261 ).
Thus, DHA is a key structural component of the brain and retina, where it plays particular, unique functional roles. A supply of DHA is very important early in life, especially during the fetal and early infant periods when the eye and central nervous system are developing. Since the supply must come from maternal sources (via the placenta and breast milk), maternal DHA status is likely to be important in determining eye and brain development early in life. Newly emerging areas of interest relate to the influence of very long-chain n-3 fatty acids on childhood developmental disorders, adult psychiatric and psychological disorders, and neurodegenerative diseases of ageing. These conditions appear to be associated with a lower status of very long-chain n-3 fatty acids. Additionally, there is some epidemiological evidence for a lowered risk of psychiatric, psychological disorders and neurodegenerative disorders with increased dietary intake of very long-chain n-3 fatty acids. Intervention studies indicate that there may be some benefit from very long-chain n-3 fatty acids in childhood developmental and adult psychiatric and psychological disorders, particularly in depression. Interestingly many of these studies are indicative that EPA is more important than DHA, which contrasts with the relative roles of these two fatty acids in very early eye and brain development. Although there may be a role for very long-chain n-3 fatty acids in slowing cognitive decline( Reference Zhang, Hou and Li 259 ), this has not been well demonstrated( Reference Sydenham, Dangour and Lim 258 , Reference Burckhardt, Herke and Wustmann 260 ). There is a clear need for larger, longer and higher quality human trials in this area of research.
Recommendations for intake of EPA and DHA acid
The demonstration of physiological actions of EPA and DHA that result in improved health outcomes and reduced risk of disease, along with the increased understanding of the molecular and cellular mechanisms of action involved, indicates a need to set recommendations for the intake of these important fatty acids. However, the exact requirement for very long-chain n-3 fatty acids in order to maintain health is not known. Furthermore, there has been a lack of clarity about the extent to which EPA and DHA can be synthesised in human subjects so long as there is sufficient intake of the precursor ALA( Reference Baker, Miles and Burdge 8 ). Nevertheless, the recognition of the health benefits of EPA and DHA has resulted in several recommendations to increase the intake of fish and, more specifically, of EPA and DHA by various governmental, non-governmental and professional agencies( 17 , Reference Kris-Etherton, Harris and Appel 89 , Reference Siscovick, Barringer and Fretts 129 , Reference Simopolous, Leaf and Salem 262 – 267 ). These recommendations are summarised in Table 5.
Table 5. Some of the current recommendations for intake of very long-chain n-3 fatty acids

The future of research in n-3 PUFA
Very long-chain n-3 fatty acids have been a focus of research since the late 1980s, although important pioneering work in the field was performed before then. A PubMed search conducted in late August 2017 identified over 25 500 publications using the search term ‘omega-3’ and 28 500 publications using the search term ‘fish oil’. The earliest publications identified using those terms were from 1922 and 1945, respectively, although the earliest of the almost 1000 publications identified with the search term ‘cod liver oil’ was from 1845. By 1990, there was a publication on the topic of n-3 fatty acids on average every day. By the mid-2010s this had risen to almost five publications every day. Thus, a tremendous effort has been, and is continuing to be, expended on research in the area of n-3 fatty acids. This research effort obviously falls into many categories, not all related to human health and well-being, although much of it does. Research aimed at better defining the roles that very long-chain n-3 fatty acids play in human growth, development, function, health, well-being and disease risk and better understanding the molecular and cellular mechanisms involved will continue for the foreseeable future. Despite the vast amount of knowledge that is currently available about n-3 PUFA, there remain significant gaps and inconsistencies in the literature that impair the ability to make robust recommendations for both the healthy population and specific patient groups. One reason for this is that it is not clear what the extent of the variation in response to very long-chain n-3 fatty acids is within the population or amongst particular subgroups, or what the determinants of such variation might be, although these are likely to include other dietary components, sex, body composition, genetics, stage in the life course, physiological state and the presence of disease. These determinants are likely to be more thoroughly explored in the coming years, with researchers taking advantage of new analytical and data handling technologies to study larger numbers of samples. Surprisingly, there is insufficient information currently available on dose responses of many physiological and pathological outcomes to very long-chain n-3 fatty acid intervention. This hampers the ability to use the full potential of very long-chain n-3 fatty acids in both public health and clinical settings. Most research on very long-chain n-3 fatty acids to date has focused on EPA and DHA, usually studied in combination. This has resulted in many researchers thinking that EPA and DHA have the same biological effects. This seems not to be the case( Reference Mori and Woodman 268 , Reference Wei and Jacobson 269 ), but again this is an area of research that is likely to become better explored in the next period. Related to this, biological actions of DPA have emerged( Reference Kaur, Cameron-Smith and Garg 4 , Reference Weylandt 5 ) but the effects of DPA and its mechanisms of action are poorly described at the moment. It seems likely that DPA will become a greater focus of research in this area. It is also likely that research into the molecular mechanisms of the action of each individual very long-chain n-3 fatty acid will continue, with membrane structure–function relationships, early intracellular signalling pathways, transcription factors, novel membrane receptors and novel lipid mediators all being important candidates for future study. Whatever the mechanism involved, it is evident that EPA, DPA and DHA consumed orally need to be found at the site of their action in the body. As described earlier, it is also clear that the effects of these fatty acids on cell and tissue function are dose dependent( Reference von Schacky, Fischer and Weber 39 , Reference Harris, Windsor and Dujovne 40 , Reference Blonk, Bilo and Popp-Snijders 42 , Reference Rees, Miles and Banerjee 45 ). Thus, it seems likely that strategies for more effective delivery of these bioactive fatty acids will be explored. These might include different chemical( Reference Schuchardt and Hahn 270 , Reference Ghasemifard, Turchini and Sinclair 271 ) or physical( Reference Raatz, Johnson and Bukowski 272 ) formulations.
The richest dietary source of very long-chain n-3 fatty acids is seafood, especially fatty fish (Table 1). Despite recommendations by many authorities to increase consumption of fish, the majority of the Western population are low consumers. An alternative to eating fish is to use n-3 supplements (fish oil) which give an assured intake of EPA and DHA. A concern is that the supply of fish for human consumption and for the production of oil for use in n-3 supplements is not sustainable. Thus, alternative sources need to be sought and then proven to be beneficial to human health. Such alternatives include algal oils, some of which are already widely used in the infant formula industry and seed oils from plants GM to produce EPA and DHA( Reference Ruiz-Lopez, Usher and Sayanova 273 ). Finally, although the focus of the present paper is the very long-chain n-3 fatty acids EPA, DPA and DHA, plant n-3 fatty acids are widely available and are sustainable. Most research on plant n-3 fatty acids conducted to date has been on ALA as an alternative to very long-chain n-3 fatty acids( Reference Baker, Miles and Burdge 8 ), but there has been some research on SDA( Reference Baker, Miles and Burdge 8 , Reference Walker, Jebb and Calder 274 ). From the research conducted to date, ALA and SDA need to be converted to very long-chain n-3 fatty acids by the pathway shown in Fig. 1 to be biologically effective( Reference Baker, Miles and Burdge 8 ). However, the studies done so far show very poor conversion of ALA and SDA to DHA in human subjects, although SDA is a better precursor for EPA synthesis than ALA( Reference James, Ursin and Cleland 275 ). Future research will attempt to identify strategies to enhance endogenous biosynthesis of very long-chain n-3 fatty acids from their simpler plant precursors. These strategies might include modifying other aspects of the diet or taking advantage of genetic polymorphisms that favour conversion. Thus, there are many important questions yet to be answered and this assures continued research in the field of n-3 fatty acids and human health.
Summary and conclusions
n-3 Fatty acids are a family of PUFA that contribute to human development, health and well-being. Functionally the most important n-3 fatty acids are the very long-chain EPA and DHA found in fatty fish and in supplements; roles for DPA are now emerging. Intakes of EPA and DHA are typically low and much below those that are recommended. Increased intakes of EPA and DHA, either from fish or from supplements, are reflected in greater incorporation into blood lipid, cell and tissue pools. Increased content of EPA and DHA can modify the structure of cell membranes and also the function of membrane proteins involved as receptors, signalling proteins, transporters and enzymes. EPA and DHA also modify the production of lipid mediators and through effects on cell signalling can alter patterns of gene expression. Through these actions EPA and DHA act to alter cellular responsiveness in a manner that seems to result in more optimal conditions for growth, development and maintenance of health. The effects of very long-chain n-3 fatty acids are evident right through the life course meaning that there is a need for all sectors of the population to increase the intake of these important nutrients. EPA and DHA have a wide range of physiological roles, which are linked to certain health or clinical benefits. A number of risk factors for CVD are modified in a beneficial way by increased intake of EPA and DHA; these include blood pressure, platelet reactivity and thrombosis, plasma TAG concentrations, vascular function, cardiac arrhythmias, heart rate variability and inflammation. Thus, there is a key role for these fatty acids in prevention and slowing progression of CVD. Furthermore, some supplementation studies with EPA and DHA have demonstrated reduced mortality in at risk patients, such as post-MI, indicating a therapeutic role, although this is currently disputed by some. A number of other, non-cardiovascular, actions of EPA and DHA have also been documented, suggesting that increased intake of these fatty acids could be of benefit in reducing the risk of (i.e. protecting from) or treating many conditions. For example, they have been used successfully in RA and, in some studies, in inflammatory bowel disease, and may be useful in other inflammatory conditions like asthma and psoriasis. EPA and DHA may also have a role as part of cancer therapy; some recent studies show that they improve the effectiveness of chemotherapeutic agents. DHA has an important structural role in the eye and brain, and its supply early in life when these tissues are developing is known to be of importance in terms of optimising visual and neurological development. For this reason it is very important that pregnant and breast-feeding women have adequate DHA intake. Recent studies have highlighted the potential for EPA and DHA to contribute to enhanced mental development and improved childhood learning and behaviour and to reduce the burden of psychiatric and depressive illnesses in adults, although these areas of possible action require more robust scientific support. There may also be a role for EPA and DHA in preventing neurodegenerative disease of ageing, but evidence for this is currently weak. The effects of EPA and DHA on health outcomes are likely to be dose-dependent, but clear dose–response data have not been identified in most cases. Also in many cases it is not clear whether both EPA and DHA have the same effect or potency and therefore which one will be the most important for a particular indication. Thus, despite several decades of productive research on the health effects of very long-chain n-3 fatty acids and the mechanisms involved, important questions are currently unanswered and many areas remain to be explored in future research.
Conflict of interest
The author serves on the Scientific Advisory Boards of Pronova BioPharma (part of BASF), Smartfish, DSM, FrieslandCampina and Danone Nutricia Research.
Financial Support
None.
Authorship
The author had sole responsibility for all aspects of preparation of this paper.