INTRODUCTION
Megalithic monuments built during the Neolithic period hold fundamental keys to understanding the processes driving early social complexity (for seminal discussions, see Renfrew, Reference Renfrew and Renfrew1973; Chapman, Reference Chapman, Chapman, Kinnes and Randsborg1981, Reference Chapman1990; Sherratt, Reference Sherratt1990; for recent overviews, see Meller et al., Reference Meller, Gronenborg and Risch2018; Müller et al., Reference Müller, Hinz and Wunderlich2019; Laporte and Cousseau, Reference Laporte and Cousseau2020). However, methodological challenges remain that make that understanding quite difficult. The first and foremost challenge is the establishment of the monuments’ temporality. While burial activity at Neolithic monuments is relatively easy to verify through the radiocarbon dating of human bone, establishing the age of their construction remains an outstanding challenge that has hampered prehistoric archaeology for decades. This problem is particularly pressing for megaliths that were not predominantly used for burial and is further compounded by the fact that, almost invariably, the most important constructions experienced activity well beyond the Neolithic period, into the Bronze Age and Iron Age, and even during historical periods, which often obliterated the evidence of early activity in and around them.
In this paper, an innovative multimethod approach is used to tackle this issue, revealing the age of construction and associated social and cultural context of one of Europe's most fascinating megalithic monuments: the Menga dolmen. Menga is part of the Antequera megalithic site (Málaga, Spain), one of the very few European megalithic complexes included in the UNESCO World Heritage Site List (Fig. 1). Antequera houses four major megaliths and two “natural” monuments closely associated with them. The four megaliths include Menga, which attained great fame throughout the nineteenth century (Sánchez-Cuenca, Reference Sánchez-Cuenca2011) following the first explorations in the 1840s (Mitjana y Ardison, Reference Mitjana y Ardison1847); the Viera dolmen, discovered in 1903 barely 50 m southwest of Menga; El Romeral tholos, discovered in 1904 some 6 km east of Menga and Viera; and the Piedras Blancas megalithic structure, discovered as recently as 2020, as part of the research program this paper stems from. The two natural monuments include El Torcal, a karstic formation located 11 km south of the megaliths, and home to El Toro Cave, occupied since the Early Neolithic (ca. 5400 BCE); and La Peña de los Enamorados, a limestone massif rising 800 m above sea level and presiding over the skyline of the Antequera plain.

Figure 1. (A) Major earthen and megalithic monuments in fourth and third millennium western Europe (UNESCO World Heritage List in red; all others in yellow); (B) location of the Antequera region within the Iberian Peninsula; (C) distribution map of Neolithic sites in the lands of Antequera region (main sites discussed in the text in red; all others in black).
Menga is a remarkable monument. Located on a hilltop (Fig. 2A), its inner space, measuring 24.9 m in length, with a maximum width of 5.7 m and a height rising from 2.5 m at the entrance to 3.45 m at the back of the chamber, is enclosed by 12 uprights on each side plus a massive backstone and covered by five capstones (Fig. 2B). The feat involved in the construction of this dolmen is best reflected by the magnitude of the colossal stones used to build it, with a combined estimated weight of well over 800 tons. Capstone C-5, at the back of the chamber (Fig. 2C), is the largest of all, with an estimated weight of 170 tons, which makes it the largest stone ever moved as part of the megalithic phenomenon in Iberia, and one of the largest in Europe. By comparison, the sarsen stones used in Stonehenge (UK), which were added in ca. 2500 BCE to the already existing ditched enclosure, weigh an average of 25 tons, with the largest of them, the so-called Heel Stone, weighting ca. 30 tons (Parker Pearson, Reference Parker Pearson2012). Another important element in Menga's architectural design is the presence of three large pillars aligned with the chamber's longitudinal axis, below the joints of the four inner capstones (Fig. 2C and D). The mound covering the construction presents a diameter of 48.3 m in the northeast to southwest axis and 44 m in the northwest to southeast axis, covering a surface of ca. 2.730 m2. This stout tumulus has provided protection and stability to the construction for almost 6000 years.

Figure 2. Menga: (A) general view of the mound and entrance form the northeast (photograph: LGS); (B) plan showing the numbering of stones (design: Coronada Mora Molina, Universidad de Sevilla); (C) interior view from the back of the chamber (photograph: Miguel Ángel Blanco de la Rubia); (D) interior view from the back of the chamber with water well and Pillar 3 in the foreground (photograph: Coronada Mora Molina, Universidad de Sevilla).
Three other elements add to the uniqueness of Menga. The first of them is the water well located at the back of the chamber (Fig. 2D). With 19.4 m of depth and 1.5 m of width (slightly narrower at the bottom), this well provides access to fresh water, which makes it a unique hydraulic feature within the context of the megalithic phenomenon worldwide. Indeed, Menga's long biography runs in parallel with the complex history of local water resources (García Sanjuán et al., Reference García Sanjuán, Montero Artús, Mora Molina, Bartelheim, García Sanjuán and Hardenberg2021). The second element to be noted is the landscape aspect of the dolmen. Unlike most Iberian megaliths, which face sunrise between the summer and winter solstices (Hoskin, Reference Hoskin2001), Menga's axis of symmetry points to 45°, about 10° north of the summer solstice. Thus, Menga faces the northern sector of La Peña de los Enamorados mountain, where at the time of its construction intense activity was already taking place around the Matacabras sanctuary, which includes rock art paintings of the “schematic” style (García Sanjuán et al., Reference García Sanjuán, Wheatley, Díaz-Guardamino Uribe, Mora Molina, Sánchez Liranzo and Strutt2015; Rogerio-Candelera et al., Reference Rogerio-Candelera, Bueno Ramírez, De Balbín Behrmann, Dias, García Sanjuán, Coutinho and Lozano Rodríguez2018). The megalithic monument recently discovered at the foot of Matacabras adds to the significance of La Peña's northern sector. However, it is important to note that, although Menga's orientation prioritized a land “target” and was not made to fall between the range of the solstices, as is customary in Iberia, its design also sought to integrate architecture and sunlight (Lozano-Rodríguez et al., Reference Lozano Rodríguez, Ruiz Puertas, Hódar Correa, Pérez Valera and Morgado Rodríguez2014), thus seeking a sunlight effect in a manner best reflected in Newgrange (Ireland) (Patrick, Reference Patrick1974). Third, Menga is quite remarkable because it appears to have been in constant use since its original construction, never falling into oblivion—the inevitable fate awaiting most prehistoric megaliths at one point or another. The stratigraphic deposits and material culture associated with the dolmen reveal clear evidence of its use during the Copper Age, Bronze Age, Iron Age, antiquity, Middle Ages, and modern history (García Sanjuán and Lozano Rodríguez, Reference García Sanjuán, Lozano Rodríguez, Laporte and Scarre2016).
Despite all this, the construction date of Menga and its temporality as a monument have essentially been a mystery until now. The seriousness of this problem is best reflected in the fact that, as late as 2016, not a single radiocarbon date had been published for it. This is all the more striking in light of the set of characteristics that makes it such an exceptional monument (the true “flagship” of the Antequera site, itself only matched by Altamira and Atapuerca as the main “icons” of Spanish Prehistory) and the almost 200 years elapsed since the first explorations, a period that has witnessed a substantial number of archaeological interventions. Paradoxically, although a true quantum leap has been achieved within the last decade, progressing from 0 to 61 radiocarbon dates for Menga, those dates published up to now provide mostly historical ages, thus reflecting activity at the monument and its surroundings in antiquity, the Middle Ages, and modern history (García Sanjuán et al., Reference García Sanjuán, Mora Molina, Lozano Medina, Aranda Jiménez, García Sanjuán and Mora Molina2018a). Such a data set did not provide the kind of precise information needed to date Menga's construction, which occurred in the Neolithic. Needless to say, this in turn prevented any in-depth understanding of the social and cultural circumstances that made such a remarkable monument possible.
Using an innovative multimethod approach, we have obtained 29 new numerical ages over the last 7 years, including 12 radiocarbon, 11 optically stimulated luminescence (OSL), and 6 uranium-thorium series (U-Th) dates. They are presented in this paper for the first time. As will be shown later, this data set provides the basis to build a fresh high-resolution multimethod chronometric model of Menga's construction, which in turn allows for an entirely new analysis of its genesis as a monument. For the first time in almost 200 years of research, a sound understanding of the sociocultural background surrounding the construction of Menga, both locally (including the occupancy, frequentation, and ritual use of other sites known in the region) and regionally (the wider arena of changes occurring in the Late Neolithic period) is possible.
SAMPLING AND METHODS
Radiocarbon Dating
In total, 12 samples of charred material and animal bone from Menga (9) and El Toro (3) were submitted for radiocarbon dating to the Centro Nacional de Aceleradores (Seville, Spain) and Beta Analytic. They were pretreated, graphitized, and measured as described by Santos Arévalo et al. (Reference Santos Arévalo, Gómez Martínez and García León2009). The reported δ13C values were measured by accelerator mass spectrometry. All calibrations were made with OxCal 4.4 (Bronk Ramsey, Reference Bronk Ramsey1995) based on the IntCal20 Northern Hemisphere radiocarbon age calibration curve (Reimer et al. Reference Reimer, Austin, Bard, Bayliss, Blackwell, Bronk Ramsey and Burtzin2020).
In the case of Menga, all nine new radiocarbon determinations (Table 1), were drawn from samples collected from Trench 1 of the excavation undertaken by the University of Granada between September 2005 and March 2006. Trench 1 (Fig. 3), 27.4 m long and 3 m wide, was located on the northwestern sector of the dolmen's mound, between its summit and the track opened in the 1940s to facilitate the access of motor vehicles all the way to the dolmen's entrance, which broke a large section of the mound. Trench 1 allowed documentation of the stratigraphy of the mound, which was composed basically of two main layers. The deepest, 1.5 m thick and composed of very dark soil, yielded large amounts of organic material and substantial quantities of hand-thrown pottery and knapped flint. Eight radiocarbon dates were obtained from samples of this layer, which provided in all cases Late Neolithic ages (fourth millennium BCE) as will be described in the “Results.” This layer integrates sediments collected from the very same hill where Menga was built and essentially reflects the intense activity the hill witnessed before and during the construction of the monument. Therefore, the radiocarbon determinations obtained from this layer provide post quem ages for the erection of the dolmen. The upper layer of the mound, some 3 m in depth, was made with alternating layers of carefully locked medium-size sandstone slabs and rammed clay. Undoubtedly, this robust device, enveloping the megalithic chamber, has played a major part in its preservation, protecting it from weathering and geological events. One radiocarbon determination (Beta-526347) comes from a sample taken from the westernmost end of Trench 1 and represents a different event—perhaps the expansion of the mound sometime after the original construction of the dolmen (Mora Molina, Reference Mora Molina2018, p. 737). For this reason, this particular determination has been used as an ante quem age with respect to the primary construction of Menga. Five of these nine dates were obtained from samples of charred material, while the other four are from samples of nonarticulated animal bones (Table 1). All these dates provide very consistent ages between ca. 3900 and 3400 BCE, with the exception of Beta-526347, which provides a slightly later age, which is in keeping with its stratigraphic position.
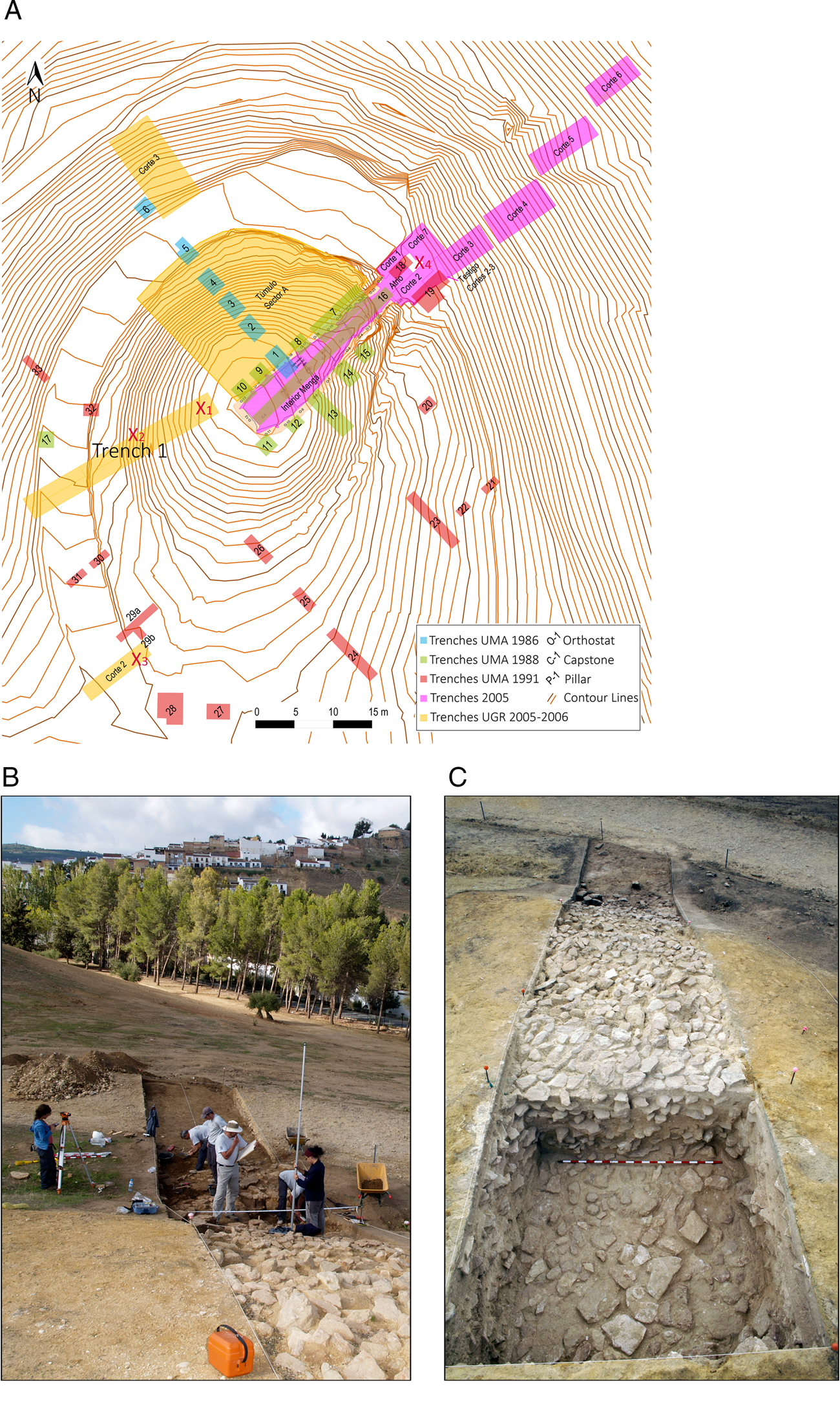
Figure 3. Menga: (A) location of Trench 1 of the 2005–2006 excavation in Menga with reference to other excavated areas of the dolmen (design: Coronada Mora Molina, Universidad de Sevilla); (B) general view of excavation activity in September 2005 at Trench 1, taken from the east (photograph: David García González, CSIC); (C) general view of Trench 1, revealing the construction system of Menga's mound, with alternating layers of medium-sized stone slabs and pressed clay (photograph: David García González, CSIC).
Table 1. New radiocarbon determinations of Neolithic age for Menga.

a NA, not available.
b X1, X2, X3, and X4 refer to the locations of the samples as shown in Fig. 3A (plan of Menga excavations).
The nine new Menga 14C dates were combined with three previously published ones, which were obtained from samples of charred material extracted from a pit (Structure 9) located at the atrium and from the base of the mound (García Sanjuán and Lozano Rodríguez, Reference García Sanjuán, Lozano Rodríguez, Laporte and Scarre2016, p. 5). As mentioned earlier, several other radiocarbon dates have been published for Menga and its surroundings, all of which provided historical ages and therefore are not relevant to establish its construction date.Footnote 1
In the case of El Toro, a cave located at El Torcal karst that holds important keys to understanding the cultural background to early monumentality in the Antequera region, three samples of charred material were obtained for radiocarbon dating. Excavated in the late 1980s and early 1990s (a full description is available in Martín Socas et al., Reference Martín Socas, Cámalich Massieu and González Quintero2004), El Toro is a good example of an Early Neolithic habitat, resulting from the spread of the first farming communities along the Mediterranean coasts of Iberia between ca. 5600 and 5400 BCE. As shown by a robust series of 29 radiocarbon determinations (Egüez et al., Reference Egüez, Mallol, Martín Socas and Cámalich Massieu2016) (Table 2), this cave was used as a habitation and as an animal pen, probably also witnessing some ritual activity (Egüez et al., Reference Egüez, Mallol, Martín Socas and Cámalich Massieu2016; Santana et al., Reference Santana, Rodríguez-Santos, Cámalich-Massieu, Martín-Socas and Fregel2019), since the second half of the sixth millennium BCE and throughout the entire fifth millennium BCE. In their conclusions, the excavators claimed that El Toro Cave was abandoned because of an earthquake that caused a dramatic change in its topographic conditions, including the lasting blockage of its main entrance and the collapse of a major slab from the roof (Martín Socas et al., Reference Martín Socas, Cámalich Massieu and González Quintero2004, p. 297). It is worth noting that Antequera is located in one of the most seismic areas of the Iberian Peninsula (Lario et al., Reference Lario, Zazo, Goy, Silva, Bardají, Cabero, Dabrio, Insua Arévalo and Martín González2010; Grützner et al., Reference Grützner, Ruano, Jabaloy, Galindo-Zaldívar, Becker-Heidmann, Sanz De Galdeano, Rudersdorf and Reicherter2013). This slab, measuring about 12 m in length and 4 m in width, lies at the far end of the cave's main chamber (Fig. 4C and D). However, the chronology and possible causes of the fall of this slab, potentially relevant to understand the abandonment of the cave, were never established. The three newly dated samples provided ages that also fall within the Late Neolithic period (Table 3), as will be discussed later.
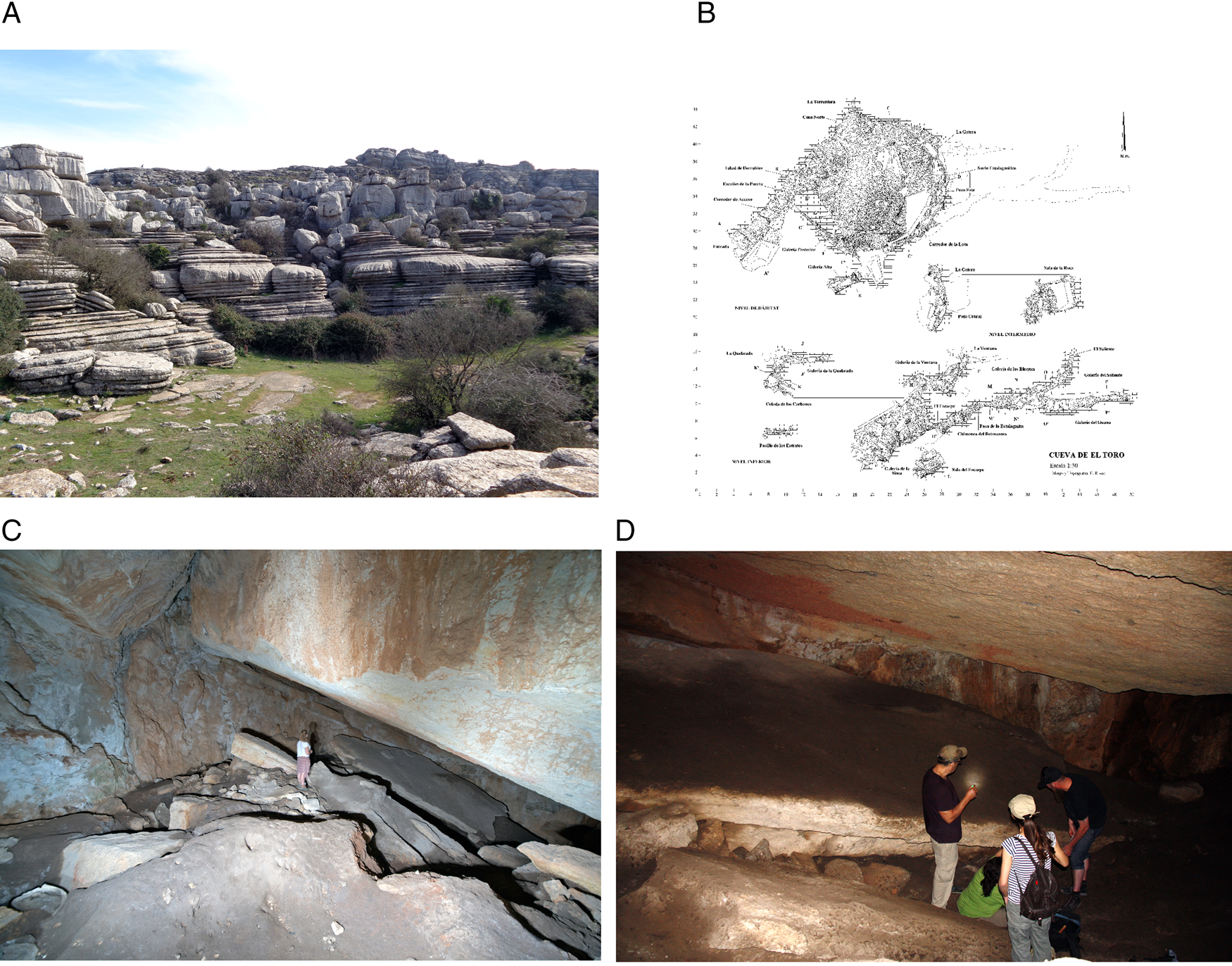
Figure 4. El Toro: (A) general overview of El Torcal karstic formation (photograph: Coronada Mora Molina, Universidad de Sevilla); (B) general plan of the cave (after Martín Socas et al., Reference Martín Socas, Cámalich Massieu and González Quintero2004, p. 29, figure 3); (C) general view of the cave, with large fallen roof slab in the background, next to person standing (photograph: DWW): (D) Detail of the sample extraction for radiocarbon and OSL dating, underneath and above the fallen roof slab in June 2017 (photograph: AM).
Table 2. Radiocarbon determinations for El Toro (after Egüez et al., Reference Egüez, Mallol, Martín Socas and Cámalich Massieu2016).

Table 3. New radiocarbon determinations for collapse of El Toro cave roof slab.

Bayesian chronological modeling was applied to the newly obtained dates for both Menga and El Toro, in combination with already existing ones whenever necessary. Bayesian modeling is based on the principles of Bayesian statistics (Lindley, Reference Lindley1985) and operates by combining calibrated radiocarbon dates with archaeological information using a formal statistical methodology (Buck et al., Reference Buck, Cavanagh and Litton1996; Bronk Ramsey, Reference Bronk Ramsey2009). The resulting chronometric models are more robust and precise than those based exclusively on stratigraphic inferences or scientific dating alone. To produce Bayesian chronologies, alternative models must be created and explored to test different hypotheses (García Sanjuán et al., 2018b).
Thus, several Bayesian models were calculated, based on the following criteria of “chronometric hygiene”: (1) multiphase models were calculated through the incorporation of sequential phases, assuming that time lapses may have existed between them; (2) modeled dates (all of them calibrated) were rounded to 5 years; (3) only radiocarbon determinations with standard deviations smaller than 100 were used; (4) results were calibrated to 1σ and 2σ; (5) for each range, medians (μ) were also estimated; and (6) a Bayesian model was considered statistically robust when its verisimilitude index was equal to or greater than 60 (Amodel).
OSL dating
To obtain a more accurate date for Menga's construction, OSL was used to complement radiocarbon dating. Numerical dating by OSL considers the time elapsed since the last exposure of mineral grains (typically quartz or feldspar) to sunlight or heat (in the case of heated lithics or ceramics) and is equal to the dose received during burial over the rate the dose was delivered (dose rate). The (absorbed) dose (Gy) is obtained by dosimetry, that is, applying luminescence protocols that evaluate the natural dose against its laboratory equivalent (D e), the laboratory radiation dose (accumulated energy) needed to induce “artificial” (OSL) signals equal to the natural signal (Aitken, Reference Aitken1985, Reference Aitken1999; Liritzis, Reference Liritzis2011; Athanassas et al., Reference Athanassas, Rollefson, Kadereit, Kennedy, Theodorakopoulou, Rowan and Wasse2015). The dose rate (D r) represents the rate at which energy is delivered (Gy/ka) from the flux of nuclear radiation (alpha, beta, and gamma radiation) due to the decay of natural radioactive elements (U, Th, K, Rb) as well as from cosmic rays. D r is evaluated by measuring the radioactivity of both the sample and its surrounding burial material through well-established analytical (chemical analyses) or gamma spectrometry methods. Dosimetric gamma spectrometry can be performed either in the laboratory or in the field (in situ gamma spectrometry) (Aitken, Reference Aitken1999; Burbidge et al., Reference Burbidge, Trindade, Dias, Oosterbeek, Scarre, Rosina and Cruz2014). In any case, the cosmic-ray component should be modeled independently and added to the dose rate. The dose rate is corrected relative to water content and granulometry of the studied material (Odriozola et al., Reference Odriozola Lloret, Burbidge, Isabel Días and Hurtado2014; Rodrigues et al., Reference Rodrigues, Dias, Valera, Rocha, Prudêncio, Marques, Cardoso and Russo2019).
OSL is regarded as a good alternative to establish the temporality of major monuments when well-contextualized organic remains are scarce or unavailable (López-Romero, Reference López Romero, García Sanjuán, Scarre and Wheatley2011; Rodrigues et al., Reference Rodrigues, Dias, Valera, Rocha, Prudêncio, Marques, Cardoso and Russo2019). This is exactly the situation at Menga, which suffers from a complete lack of Neolithic human bone and a dearth of animal bone associated with its construction phase and early use. In general, the application of OSL to the dating of megalithic monuments started very recently (Galli et al., Reference Galli, Panzeri, Rondini, Poggiani Keller and Martini2020; Shewan et al., Reference Shewan, O'Reilly, Armstrong, Toms, Webb, Beavan and Luangkhoth2021; Parker Pearson et al., Reference Parker Pearson, Pollard, Richards, Welham, Kinnaird, Shaw and Simmons2021; Kim et al., Reference Kim, Choi, Lim, Shin, Yeo, Weon and Heo2022). In Iberia, despite some earlier attempts (see Calado, Reference Calado2003; López Romero, Reference López Romero, García Sanjuán, Scarre and Wheatley2011; Athanassas et al., Reference Athanassas, García Sanjuán, Theodorakopoulou, Jain, Sohbati, Guerin and Lozano Rodríguez2016, Reference Athanassas, García Sanjuán, Stamoulis, Lineros Romero, Carinou and Anglada Curado2017), the use of OSL is still not widespread, and its full potential has not yet been realized.
OSL using the single-aliquot regenerative protocol (Murray and Wintle, Reference Murray and Wintle2000) was measured from a total of three samples collected in 2014 from the foundation sockets (and associated infills) of the uprights and pillars of the dolmen (Fig. 5C and D, Table 4). This assumed that a sufficient amount of grain of the sediment should have time and conditions for an adequate exposure to daylight during collection and transportation from its geological source and trickling into the socket at the time of construction. Therefore, good bleaching conditions are assumed, resetting the geological OSL signal before the construction of the monument. Sampling was based on the following reasoning. Pillar 3 does not stand perfectly vertically, but leans some 4° to the north (Fig. 5B). For this reason, its lower third leans to the northern side of the socket cut as its foundation to a depth of 1.18 m. Thus, although the inner areas of the dolmen, including the sockets of the uprights and pillars, have been extensively excavated since the nineteenth century, we anticipated that undisturbed fractions of the sediments of the infill used originally inside the socket of Pillar 3 would have remained within the narrow space between its northern side and the northern side of the socket. Indeed, infill sediment trapped between Pillar 3 and the socket's northern face was retrieved at three locations (marked in Fig. 5B). This sediment was found to be more cohesive than the surrounding sediment (another evidence of its relatively older age) and allowed the insertion of thin aluminum tubes (Fig. 5D) to capture the samples undisturbed.

Figure 5. Menga: (A) location of Pillar 3 with regard to Capstones 4 and 5 based on the laser-scanning photogrammetry of the monument (design: LGS, after Baceiredo Rodríguez et al., Reference Baceiredo Rodríguez, Baceiredo Rodríguez, García Sanjuán and Odriozola Lloret2014); (B) section of Pillar 3 (P-3) and socket (precise location each dated OSL samples is marked with a red X; design: José Antonio Lozano Rodríguez, CSIC); (C) general view of the excavation process inside the socket of Pillar 3 (photograph: LGS); (D) detail of the sample-extraction process inside the socket of Pillar 3 (photograph: Katerina Theodorakopoulou, University of Athens).
Table 4. Optically stimulated luminescence (OSL) ages for Menga and El Toro.

An earlier attempt at dating the infills from the sockets of Menga (Athanassas et al., Reference Athanassas, García Sanjuán, Theodorakopoulou, Jain, Sohbati, Guerin and Lozano Rodríguez2016) was clouded by large uncertainties due to the infiltration of a considerable amount of unrest grains into the measured multigrain aliquots. The luminescence signal from single grains was then measured to increase the resolution and distinguish the population of grains most likely to be fully reset at the time of construction. This method provided a largely resolved distribution with a well-defined dominant peak from which the burial dose could be estimated by applying the internal–external uncertainty model (IEU; Thomsen et al., Reference Thomsen, Murray, Bøtter-Jensen and Kinahan2007).
At El Toro Cave, two samples of sediment accumulated below and above the fallen slab described earlier were collected for OSL dating. All four samples were retrieved from the southern third of the slab (their positions were only recorded photographically, as no detailed plan of this slab exists). The sample under the slab was not sealed, and therefore it could not be guaranteed that it was deposited before the collapse of the roof.
All OSL samples were collected so as to avoid exposure to daylight and were treated under controlled light conditions. OSL was measured on the quartz fraction of each sample based on multigrain aliquots, which have been shown to provide enough resolution to estimate the true burial dose and the corresponding age of flood deposits (Medialdea et al., Reference Medialdea, Thomsen, Murray and Benito2014). Dose distribution of the sediment under the slab was normally distributed, and the central age model (Galbraith et al., Reference Galbraith, Roberts, Laslett, Yoshida and Olley1999) was suitable for calculating the burial dose, whereas dose distribution of the sediment on top of the slab was largely scattered, so the IEU model (Thomsen et al., Reference Thomsen, Murray, Bøtter-Jensen and Kinahan2007) was considered more appropriate to estimate the burial dose in this case.
For the environmental dose rate estimation, in situ field gamma measurements were performed using a gamma spectrometer (HPI Rainbow MCA with a 3 inch x 3 inch NaI probe). Stripped counts in the windows 1380–1530 keV, 1690–1840 keV, and 2550–2760 keV (designed to obtain signals dominated by 40K, 214Bi, and 208Tl, respectively) were calibrated relative to previous measurements in the Oxford and the Gif-sur-Yvette blocks (Richter et al., Reference Richter, Zink, Przegietka, Cardoso, Gouveia and Prudêncio2003) to obtain apparent parent element concentrations for K, Th, and U, assuming equilibrium in the 232Th and 238U series (Marques et al., Reference Marques, Prudêncio, Russo, Cardoso, Dias, Rodrigues, Reis, Santos and Rocha2021). The dose rate was determined through a combination of techniques. For the three sediment samples from Menga (Menga 2 Socket, Menga 1 Infill, and Menga 1 Contact), the dose rate was derived from in situ gamma measurements using a gamma spectrometer (HPI Rainbow MCA with a 3 inch x 3 inch NaI probe), performed in the sampling contexts. Stripped counts in the windows 1380–1530 keV, 1690–1840 keV, and 2550–2760 keV (designed to obtain signals dominated by 40K, 214Bi, and 208Tl respectively) were calibrated relative to previous measurements in the Oxford and the Gif-sur-Yvette blocks (Richter et al., Reference Richter, Zink, Przegietka, Cardoso, Gouveia and Prudêncio2003) to obtain apparent parent element concentrations for K, Th, and U, assuming equilibrium in the 232Th and 238U series (Marques et al., Reference Marques, Prudêncio, Russo, Cardoso, Dias, Rodrigues, Reis, Santos and Rocha2021). The dose rate for the two sediment samples from El Toro (CET-OSL2 and CET-OSL3) was determined from the radionuclide activity concentration measured by high-resolution gamma spectrometry (Xtra HpGE spectrometer).
In addition, OSL was measured on six ceramic samples from various locations at the dolmen's atrium to further refine Menga's temporality (Table 5). For the ceramic shards, the dose rate was derived from the concentration of radioelements in the pieces (measured by inductively coupled plasma mass spectrometry) used to calculate the beta contribution and in situ gamma measurements for the gamma contribution to the dose rate.
Table 5. Optically stimulated luminescence (OSL) ages for pottery shards from Menga.

U/Th dating
To further examine the age of the collapse of El Toro roof slab (and, indirectly, the abandonment of the cave and its possible relationship with the construction of Menga), four samples (designated as ET-1, ET-2, ET-3, and ET4 in Table 6) of calcite for U/Th dating were obtained from the present-day roof of the cave, just above the fallen slab. These samples were intended to provide additional ante quem ages for the collapse of the slab. However, these samples failed quality control, as they were all contaminated by detrital Th to a degree that accurate and precise U/Th ages could not be calculated. Detrital, or initial, 230Th can be incorporated into speleothems when they form and may impact on the accuracy of the resulting U/Th age (Hoffmann et al., Reference Hoffmann, Pike, García-Díez, Pettitt and Zilhão2016). Typically, this is identified by measuring levels of 232Th in the sample, and a correction can be applied, provided the isotopic composition of the detrital particles is well known and the contamination is not too severe. However, when (230Th/232Th)A is <20, the detrital Th corrections have a significant effect on the calculated ages, and with the four El Toro U/Th samples being characterized by (230Th/232Th)A ≤ 1 (Table 6), it was not possible to calculate accurate and precise U/Th ages.
Table 6. U and Th concentrations, isotopic activity ratios and U-Th ages for the El Toro and El Aguadero speleothems.a

a Analytical errors are 2σ of the mean. (230Th/238U) = 1 − e-λ230T + (δ234Umeasured/1000)[λ230/(λ230 − λ234)](1 − e−(λ230 − λ234)T), where T is the age. Decay constants are 9.1705 × 10−6 a−1 for 230Th, 2.8221 − 10−6 a−1 for 234U (Cheng et al., Reference Cheng, Edwards, Shen, Polyak, Asmerom, Woodhead and Hellstrom2013), and 1.55125 − 10−10 a−1 for 238U (Jaffey et al., Reference Jaffey, Flynn, Glendenin, Bentley and Essling1971). The degree of detrital 230Th contamination is indicated by the measured [230Th/232Th] activity ratio. Age corrections use an assumed detrital (232Th/238U) of 1.250 ± 0.625, a value typical of upper crustal silicates (Wedepohl, Reference Wedepohl1995), while assuming 230Th and U isotopes are in equilibrium.
Given that U/Th could not be used to directly date the collapse of the El Toro roof slab, a decision was made to take additional samples for U/Th dating from the El Aguadero sinkhole (Periana, Málaga), located some 60 km east of Antequera as the crow flies. A previous radiocarbon date (Beta-222473) on a calcite sample from a stalactite of this cavity had suggested a possible earthquake dating to 5110 ± 70 cal yr BP (4045–3713 BCE [2σ]) (Clavero Toledo, Reference Clavero Toledo2010, p. 136; Bradley and García Sanjuán, Reference Bradley, García Sanjuán, Bickle, Cummings, Hofmann and Pollard2017), which potentially matched the earthquake that, according to the excavators, had led to the abandonment of El Toro.
Thus, six U-Th dates were obtained from three speleothems (stalagmites) of El Aguadero with morphological features indicative of reorientations of growth resulting from possible tectonic movement (Becker et al., Reference Becker, Davenport, Eichenberger, Gilli, Jeannin and Lacave2006; Kagan et al., Reference Kagan, Agnon, Bar-Matthews and Ayalon2005; see Fig. 6 and Tables 6 and 7 in which U and Th concentrations, isotopic activity ratios, and U-Th ages are presented). The El Aguadero stalagmite samples were sectioned using an electric bench saw to reveal fresh surfaces, then individual calcite layers were hand-drilled to produce powders for dating. Chemical preparation and isotopic analysis using a Thermo Scientific Neptune multicollector inductively coupled plasma mass spectrometer was performed at the Ocean and Earth Science analytical geochemistry facilities at the University of Southampton. The analytical methods are detailed in Hoffmann et al. (Reference Hoffmann, Standish, García-Diez, Pettitt, Milton, Zilhão and Alcolea-González2018). Procedural chemistry blank values were always less than 0.01ng 238U, 0.1 pg 235U, 0.01 pg 234U, 0.01ng 232Th, and 1 fg 230Th, respectively. A secular equilibrium standard, uraninite URAN 84.5, demonstrates both accuracy and external reproducibility of the analytical setup. Analyses gave the following activity ratios: (230Th/238U) = 1.0026 ± 0.0007 and (234U/238U) = 1.0001 ± 0.0002 (errors are given as 2σ standard errors of the mean, n = 50 over an ~1.5 year period). This is comparable to the values published for the same solution (Hoffmann et al., Reference Hoffmann, Prytulak, Richards, Elliott, Coath, Smart and Scholz2007). Analyses of a dissolved pristine speleothem sample served as an internal standard solution to further demonstrate external reproducibility. Analyses provided the following values: (230Th/238U) = 0.4335 ± 0.0082, (234U/238U) = 1.0462 ± 0.0053, age = 58.15 ± 1.45 ka (errors are given as 2σ standard deviations of the mean, n = 14 over an ~1 year period).

Figure 6. El Aguadero: (A) Stalagmite C1-1 in situ; (a) Stalagmite C1-1 after cutting; (B) Stalagmite C1-2 in situ; (b) Stalagmite C1-2 after cutting. (Photographs: A and B by JLCT; a and b by CDS.)
Table 7. Summary data for uranium-thorium determinations for possible earthquakes at El Aguadero sinkhole.

RESULTS
Menga
Based on the radiocarbon dates described earlier, two Bayesian models were calculated for the construction of Menga (full data for all Bayesian models presented in this paper are available in the Supplementary Data files). The first combined 11 of the available dates (excluding Beta-526347, as it is much more recent and probably belongs to a later constructive phase) with the only date available for the Viera dolmen (GrN-16067: 4550 ± 140 cal yr BP),Footnote 2 built a few meters to the southwest of Menga. The Viera date acted as an ante quem date for the construction of Menga.Footnote 3 As a result, a robust model was obtained (Amodel = 96) in which the construction of Menga has an early boundary in 3835–3715 BCE (1σ) or 3910–3660 BCE (2σ) (μ = 3785 BCE) and a late boundary in 3575–3500 BCE (1σ) or 3610–3450 BCE (2σ) (μ = 3530 BCE), with a span of 145–275 years (1σ) or 55–335 years (2σ) (Fig. 7A). This model introduced a function interval between Phase 1 (construction of Menga) and Phase 2 (Viera ante quem date) that yielded the following results: 0–215 years (1α) and 0–455 years (2α). The second model used the same 11 Menga dates, but in this case, date Beta-526347 from Sector E of Trench 1, clearly posterior to the rest of the dates, was used as the ante quem limit. As a result, an equally robust model was obtained (Amodel = 97) in which the construction of Menga has an early boundary in 3825–3715 BCE (1σ) or 3895–3660 BCE (2σ) (μ = 3775 BCE) and a late boundary in 3575–3505 BCE (1σ) or 3610–3460 BCE (2σ) (μ = 3535 BCE), with a span of 140–265 years (1σ) or 55–315 years (2σ) (Fig. 7B). This model also introduced a function interval between Phases 1 and 2 with the following results: 0–165 years (1α) and 0–315 years (2α). In summary, the results of these two models set the construction of Menga between ca. 3900 and 3500 BCE (2σ).

Figure 7. Bayesian models for Menga and El Toro: (A) construction of Menga: Menga and Viera dates; (B) construction of Menga: all Menga dates; (C) abandonment of El Toro: published dates; (D) abandonment of El Toro: published dates and new dates for the fallen roof slab. (Design: VBN and LGS.)
The radiocarbon-based chronometric models obtained for Menga were then compared with the three OSL ages obtained from samples of the infill of the socket of Pillar 3 (Fig. 4, Table 2), according to the reasoning described earlier. The sediments from inside the socket of Pillar 3 yielded the following OSL ages: 5.8 ± 0.3, 5.5 ± 0.4, and 5.5 ± 0.8 ka (or 3769 ± 201, 3517 ± 325 and 3543 ± 510 BCE) (Table 2). These three ages are very consistent with each other within 1σ and with the results of the Bayesian models described earlier, giving robustness to the results of the radiocarbon dating and making it possible to establish that the construction of Menga took place between c. 3800 and 3600 BCE. These three new OSL dates are the first ever obtained for the Antequera megalithic site, and save for a “para-megalithic” structure located in Carmona, Sevilla, dating to antiquity (Athanassas et al., Reference Athanassas, García Sanjuán, Stamoulis, Lineros Romero, Carinou and Anglada Curado2017), they are the first ones ever published for an Iberian megalithic chamber.
In addition, the estimated ages for the six ceramic fragments obtained at various locations in Menga's atrium (Table 3) show rather disparate ages variously set in the Late Neolithic, Bronze Age, antiquity, and Middle Ages. While reflecting the long biography of the monument, these ages are quite important, precisely because they demonstrate how consistent and tight the radiocarbon chronology of the materials recovered from the mound is, which is of crucial value to ensure the reliability of the proposed date for Menga's construction.
Altogether, combining the results of the radiocarbon and OSL dates, it is possible to narrow down the probable construction date of Menga to between ca. 3800 and 3600 BCE. Those freshly obtained numerical ages provide a high-resolution empirical base to understand the social and cultural background surrounding the construction of this exceptional dolmen, as will be discussed later.
El Toro Cave
Establishing the degree of synchronicity between the abandonment of El Toro and the building of Menga was also a major aim of this study. To this end, three Bayesian models were made to establish the time of the collapse of El Toro Cave's roof slab. Several determinations already published for this cave (Egüez et al., Reference Egüez, Mallol, Martín Socas and Cámalich Massieu2016) were discarded, including those dating Early Neolithic activity, which are not relevant in this case (Beta-174305, UGRA-194, GrN-1544, Beta-174308, Beta-341132, Beta-341131, GrN-15444, and GrN-15440) and those dating sporadic frequentation after its abandonment. Other dates were discarded on account of the low quality of the samples or standard deviations above 100 years (GrN-15446, UGRA-189, I-17533, I-17532, Gak-8060, and Gak-8059).
The first model, based on the selected 15 radiocarbon determinations, was not statistically robust (Amodel = 38). A second model, calculated on the basis of 14 determinations, excluding Beta-174307, which is later than the rest of the series, was statistically robust (Amodel = 95). This model shows that the end of the cave's occupation had an early chronological boundary set between 4175 and 4050 BCE (1σ) or between 4250 and 4045 BCE (2σ) (μ = 4125 BCE) and a late chronometric boundary set between 4030 and 3965 BCE (1σ) or between 4050 and 3935 BCE (2σ) (μ = 3995 BCE), with a span of 20–155 years (1σ) or 0–240 years (2σ) (Fig. 7C). According to the 2σ intervals, the abandonment of the cave would have occurred between 4250 and 3935 BCE.
Next, we used the three newly obtained radiocarbon determinations to try to establish the date of the collapse of the roof slab (Table 5). Date Beta-428896, obtained from a sample from underneath the slab, provided an age for the latest activity in the cave before the fall of the slab. The two dates from above the slab (Beta-429656 and Beta-428897) were not statistically identical. For this reason, a model was calculated with two sequential phases: the first phase incorporates date Beta-428896 and latest of the two dates from above the slab (Beta-336259), while the second phase includes both dates from above the slab. The resulting model is on the threshold of being statistically robust (Amodel = 60), and for the phase before the fall of the slab (Phase 1), it provides an early boundary between 5000 and 4705 BCE (1σ) or between 5630 and 4620 BCE (2σ) (μ = 4955 BCE) and a late boundary set between 4140 and 3980 BCE (1σ) or between 4160 and 3965 BCE (2σ) (3955 BCE), while for the phase after the collapse of the roof slab (Phase 2), it yields an early boundary set between 4025 and 3970 BCE (1σ) or between 4110 and 3855 BCE (2σ) (μ = 4010 BCE) and a late boundary set between 3960 and 3795 BCE (1σ) or between 3980 and 3510 BCE (2σ) (μ = 3825 BCE), with an interval between Phase 1 and Phase 2 of 0–40 years (1σ) or 0–120 years (2σ). Therefore, if we take the late boundary of Phase 1 and the early boundary of Phase 2, the roof slab fell between 4160–3965 and 4110–3855 BCE (2σ), which is highly consistent with the results of the model based on the already published dates for El Toro, as described earlier.
Finally, a third Bayesian model was calculated in which the published dates for the occupation of the cave (Phase 1) were combined with the new dates for the time after the collapse of the roof slab (Phase 2). The resulting model (Fig. 7D) was robust (Amodel = 79.3) and set the early boundary of the cave's abandonment (Phase 1) between 4160 and 4050 BCE (1σ) or between 4180 and 4000 BCE (2σ) (μ = 4095 BCE) and the late boundary for it between 4045 and 4000 BCE (1σ) or between 4155 and 3975 BCE (2σ) (μ = 4030 BCE). The sporadic activity after the collapse of the roof slab has an early boundary between 4020 and 3975 BCE (1σ) or between 4070 and 3955 BCE (2σ) and a late boundary between 3970 and 3870 BCE (1σ) or 3985 and 3685 BCE (2σ), with an interval of 0–35 years (1σ) or 0–105 years (2σ). Choosing to err on the side of caution, we combined the early boundary of the cave's abandonment with the late boundary of the sporadic activity after the collapse of the slab: in that case, the cave was abandoned between 4160 and 3870 BCE (1σ) or between 4180 and 3685 BCE (2σ).
In summary, the Bayesian model calculated on the basis of the previously published dates, shows that the end of El Toro's sequence of permanent occupation occurred between 4250 and 3935 BCE (2σ). A Bayesian model based on the three new radiocarbon determinations obtained by us from sediments below and above the fallen slab showed that the fall of the slab occurred between 4110 and 3510 BCE (2σ), whereas a mixed model combining the previous two models (including both published and new dates) revealed the cave was very probably abandoned between 4180 and 3685 BCE (2σ).
These results were then compared with the two OSL ages from sediments from below and above the El Toro's fallen roof slab (Fig. 8, Table 2). The sample from underneath the slab (CET-OSL3) provided an age of 5.1 ± 0.3 ka (or 3113 ± 164 BCE), while the one from above the slab (CET-OSL2) returned an age of 4.8 ± 0.3 ka (or 2781 ± 166 BCE). The corresponding dose distributions are shown in Figure 8. Although congruent between themselves, these ages do not consistently match the ones provided by the radiocarbon samples. This may have been caused by unaccounted-for postdepositional activity in those sediments. Despite the failure of U-Th samples and lack of accordance between the OSL ages and the radiocarbon ages, the radiocarbon models reveal that the collapse of El Toro's roof slab and subsequent end of the stable occupation of the cave were coeval, both having occurred shortly before (or at the time of) the construction of Menga.

Figure 8. (A–C) Menga: dose distributions of samples from Menga derived from optically stimulated luminescence (OSL) measurements of quartz single grains. The plots show the individual dose values and their uncertainties. (D) El Toro: dose distributions of samples derived from OSL measurements of quartz multigrain aliquots.
To explore whether the El Toro roof slab fell as a result of an earthquake, six samples from two stalagmites collected from El Aguadero sinkhole were dated. Three samples from stalagmite C1-1 (Fig. 6Aa) produced ages from 57.25 (+4.64, −4.12) ka (C1-1a) to 3.59 (+0.52, −0.51) ka (C1-1c). Growth therefore commenced in or before the late Middle Paleolithic and continued to some degree well into the Holocene. Sample C1-1c formed during later prehistory, around the Middle Bronze Age and Late Bronze Age transition. Sample C1-1b, located 19 mm nearer to the base of the stalagmite, dates to 20.96 (+0.82, −0.76) ka, and with no clear shift in the axis of growth between these two samples, there is no clear evidence of stalagmite realignment due to tectonic activity in the Neolithic. Three samples from stalagmite C1-2 (Fig. 6 Bb) produced ages from 13.17 (+1.38, −1.29) ka (C1-2a) to 8.18 (+0.63, −0.59) ka (C1-2c). Sample C1-2c is located 22 mm from the top of the stalagmite; therefore, the vast majority of growth occurred before the Neolithic. The obvious shift in the growth axis of this stalagmite (seen toward the bottom of the image) occurred between samples C1-2a and C1-2b, so between 11.29 ka and 14.55 ka. The results show that none of the six El Aguadero U/Th ages obtained as part of this study record an earthquake in the Antequera region matching the results of radiocarbon date Beta-222473 published by Clavero Toledo (Reference Clavero Toledo2010, p. 136).
DISCUSSION: BIRTH OF A GIANT
Once a reliable date for the construction of Menga is available, it becomes possible, for the first time, to analyze the social and cultural background of its genesis, at both the local and regional scales. Synthetic ages summarizing the data described earlier are provided in Table 8.
Table 8. Summary results of chronometric models for El Toro cave and Menga.

In terms of the local scale, the construction of Menga between ca. 3800 and 3600 BCE is set against the background of major social and cultural changes at the start of the Late Neolithic period (spanning ca. 4200 to 3200 BCE). One of those changes was undoubtedly the abandonment of the “ancestral” El Toro cave between 4180 and 3685 BCE (2σ). While the data presented here cannot falsify the “earthquake hypothesis” and future speleo-seismological research dedicated to cave surveys and speleothem sampling across the region could provide further evidence to establish whether or not a major earthquake occurred ca. 4100–3900 BCE, other explanations can be found to account for the collapse of the roof slab at El Toro. Within El Torcal, the limestone massif in which El Toro is located, a very intense fracturing is recognized in relation to two conjugated systems whose directions are 40°N–60oE and 110°N–120oE respectively (Burillo Panivino, Reference Burillo Panivino, Durán and López Martínez1998). This formation is based on a pseudo-horizontal stratigraphy, which clearly conditions the circulation of water, helping the dissolution of the various materials that form it (well-stratified oolithic, nodular, or pseudobrechoid limestones arranged in boards or banks ranging from a few centimeters to 3–4 m). Karstic corridors, sinkholes, and caves, among other geomorphological features, are thus formed. Due to their different textures and carbonate content, the limestones of the massif have allowed differential action of external agents (dissolution, gelation, and perhaps wind action) on the strata, with alternating and well-defined characteristics in the upper part of the massif (Burillo Panivino, Reference Burillo Panivino, Durán and López Martínez1998). This alternation of limestones with different responses to dissolution and tectonic fracturing within the karstic massif generates differentiated blocks on the roof of its caves. Over time, they may fall due to gravity, which may, in some cases, be helped by seismic activity. In the case of El Toro, it is important to add that the very same anthropogenic activity starting inside the cave at the onset of the Early Neolithic (ca. 5400 BCE), with an obvious increase of CO2 concentrations (caused by hearths and other combustion activities) must have helped the dissolution of carbonate blocks, leading to the potential collapse of roof slabs by gravity.
Altogether, the sudden abandonment of the cave, most likely caused by the catastrophic collapse of the roof slab, must have resonated across the region. At that time, intense human activity was already taking place at various locations on the Antequera plain. This included the northern sector of La Peña de las Enamorados, where the Matacabras rock art sanctuary, the exact spot to which Menga's axis of symmetry is oriented, was in use before ca. 3800 BCE (Rogerio-Candelera et al., Reference Rogerio-Candelera, Bueno Ramírez, De Balbín Behrmann, Dias, García Sanjuán, Coutinho and Lozano Rodríguez2018), and Arroyo Saladillo, located 6 km to the west of Menga, where an individual inhumation (Structure 94) was made in 4037–3805 BCE (2σ) and covered by a cairn of medium-to-large stones, probably in combination with a ditch (García Sanjuán et al., Reference García Sanjuán, Fernández Rodríguez, Balsera Nieto, Mora Molina, Lozano Rodríguez, Cisneros García and López Sáez2020). This tomb is one of Iberia's earliest proto-megalithic graves, which is of the greatest interest in the context of Menga's genesis. Large amounts of knapped lithics and grinding tools found at both Arroyo Saladillo and Huerta del Ciprés (located barely 700 m to the north of Menga) also reveal an intensification of farming activity across the region, with a growing anthropogenic impact (García Sanjuán et al., Reference García Sanjuán, Fernández Rodríguez, Balsera Nieto, Mora Molina, Lozano Rodríguez, Cisneros García and López Sáez2020). Therefore, the construction of Menga was preceded by a buildup or formative phase in which expanded farming, exploitation of abiotic resources (particularly salt, flint, and volcanic rocks, abundant in the region; Lozano Rodríguez et al., Reference Lozano Rodríguez, Morgado Rodríguez, Puga Rodríguez and Martín Algarra2010, Reference Lozano Rodríguez, Ruiz Puertas, Hódar Correa, Pérez Valera and Morgado Rodríguez2014, Reference Lozano Rodríguez, García Sanjuán, Mora Molina, Masclans Latorre, Martínez-Sevilla, Gibaja Bao, García Sanjuán and Mora Molina2018a, Reference Lozano Rodríguez, Martínez-Sevilla, Sánchez Liranzo, Gibaja Bao, Masclans Latorre, Mora Molina, García Sanjuán, García Sanjuán and Mora Molina2018b; Morgado Rodríguez and Lozano Rodríguez Reference Morgado Rodríguez and Lozano Rodríguez2011, Reference Morgado Rodríguez, Lozano Rodríguez and García Alfonso2012; Lozano Rodríguez and Morgado Rodríguez, Reference Lozano Rodríguez, Morgado Rodríguez and Peinado Herreros2012; Morgado Rodríguez et al., Reference Morgado Rodríguez, Martínez Sevilla, Lozano Rodríguez, Palomo, Piqué and Terrada2013), exchange (based on a privileged network that relied on Antequera's geographic position at a major crossroads), and ritual activity at La Peña's northern sector played a major part.
While entirely consistent with the construction of a monument as massive as Menga, this activity does not appear to have included any major stone structures. In other words, a striking paradox becomes apparent: no precedents for an architecture as colossal as Menga existed in the Antequera region, or indeed in Iberia as a whole, in the centuries leading up to its construction. The only megalithic monument known locally before the construction of Menga is Structure 94 at Arroyo Saladillo, which is far smaller and undoubtedly required a very different scale of labor and technological expertise. On the basis of the newly available chronology for its construction, Menga appears to have sprung as an incredible feat of engineering from a background of very little or no previous megalith-building tradition. This raises an important question: where did the builders of Menga draw their formidable expertise from? Two possible hypothetical answers can be given to this question.
The first hypothetical answer lies in the production and emplacement of menhirs. In numerous regions of Iberia there is consistent evidence of “open” monumental sites with menhirs that predate the construction of megalithic burial chambers. This is especially the case in the Alentejo and Algarve regions, in southern Portugal (Calado, Reference Calado2004, Reference Calado, Joussaume, Laporte and Scarre2006; Cerrillo Cuenca et al., Reference Cerrillo Cuenca, Bueno Ramírez and Balbín-Behrmann2019), but also in northern Spain (Peñalver Iribarren, Reference Peñalver Iribarren1989, Reference Peñalver Iribarren2011; Martínez Torres, Reference Martínez Torres2015). These monuments offer a wide variety of situations, ranging from a large number of medium-sized stones, as is the case in the Almendres cromlech, with about 100 stones, or a smaller number of bigger stones, as occurs at Meada (Portalegre, Alentejo), where a single menhir is 7.5 m in height and weighs an estimated 18 tons, most likely the largest of its kind in Iberia. Although they are generally dated to the fifth millennium BCE, their chronology of foundation and use is quite poorly known. There are two reasons for this: first, very little or no burial activity took place around them, and therefore no radiocarbon dates on human bone are available; second, no OSL dates dating their construction have been obtained, save for some very specific exceptions, which have high standard error margins or await confirmation.
Did the builders of Menga gain their expertise in the handling of massive stones through earlier menhir-making? The possible presence of menhirs was suggested for the site of Piedras Blancas, at the northern sector of La Peña de los Enamorados (García Sanjuán et al., Reference García Sanjuán, Wheatley, Díaz-Guardamino Uribe, Mora Molina, Sánchez Liranzo and Strutt2015), but while recent excavations have ruled out this possibility for some of the stones lying there, good-quality dating is still needed for others. The same applies to suggestions that some of Menga's stones were first used as menhirs or stelae, probably at the very same location of the dolmen, and then reused as uprights and capstones when Menga was built (Bueno Ramírez et al., Reference Bueno Ramírez, De Balbín Behrmann, Barroso Bermejo and Ruiz González2009, p. 191). In principle, this hypothesis is consistent with the recent discovery of wine among the residues preserved in the pottery shards found in the mound of the dolmen, which, as was explained earlier, predate its construction (Garnier et al., Reference Garnier, García Sanjuán, Dodinet, Cintas-Peña, Guilaine and García Sanjuán2022). Wine was likely associated with practices of feasting, conviviality, and commensality, which are typical of societal gatherings such as those taking place at monumentalized sites in the Late Neolithic. In this “menhir hypothesis,” the construction date of Menga as a dolmen would have been different (i.e., later) than that of the making of some of its stones, which would add another layer of difficulty to the reconstruction of its complex biography.
Another hypothetical explanation of the apparent lack of local precedents for the construction of Menga is human mobility. This hypothesis sets this monument against the background of the various cultural phenomena occurring in the Iberian Peninsula in the early fourth millennium BCE, that is to say, at the onset of the Late Neolithic period, including the abandonment of caves for open-air settlements, the generalization of ditched enclosures, and the spread of megalithic chambers. A phenomenon clearly associated with this period is an increase in supraregional human interaction. Recent studies have revealed the presence of variscite from Huelva (southwest Spain, 200 km west of Antequera) in the Carnac region of French Brittany since ca. 4500 BCE and from Palazuelo de las Cuevas (Zamora, in the Spanish central plateau) since 4300–4000 BCE (Querré et al., Reference Querré, Calligaro, Cassen, Querré, Cassen and Vigier2019). From ca. 4700 BCE, evidence of large-scale megalithic monumentality exists in various regions of western France (Guilaine, Reference Guilaine, García Sanjuán, Scarre and Wheatley2011, pp. 79–81). Throughout the Atlantic façade, and more particularly in Brittany, “low” burial mounds with block enclosures including small, closed chambers (called coffres in French literature) are known, although the most impressive monuments are undoubtedly the gigantic burial mounds from the Carnac region (Saint-Michel, Le Moustoir, and Tumiac), ranging between 100 and 200 m in length, which in all cases cover a central tomb built in stone blocks, sometimes with corbelled roofing. In Brittany from ca. 4500 BCE, there is also evidence of large menhirs, stelae, and menhir-statues, some of which were later reused in megalithic burial chambers. In the Orleans region, single or double tombs covered by imposing stone slabs, attributed to the Cerny-Videlles cultural complex, were built around 4600–4500 BCE. According to the currently available radiocarbon data, all these cases predate by several centuries the appearance of the first proto-megalithic burial chambers in the Iberian Peninsula, around 4200–4000 BCE (García Sanjuán et al., Reference García Sanjuán, Sánchez Díaz and Morell Rovira2022), and certainly the construction of the Menga dolmen between 3800 and 3600 BCE. In the absence of better data on Portuguese and North African megalithic chambers, for which there are still no precise radiocarbon series to help understand their oldest developments, if one is to seek a “foreign inspiration” for the constructive genius implicit in the dolmen of Menga, the most obvious reference is Atlantic France.
Whether the fifth millennium BCE direct contacts between France and Iberia suggested by the variscite occurred by land or sea is at present unknown. The possibility of long-range maritime voyaging along the Atlantic Seaboard during the Late Neolithic has been suggested repeatedly (Callaghan and Scarre, Reference Callaghan and Scarre2017; Cassen et al., Reference Cassen, Fábregas Valcarce, Grimaud, Pailler and Schulz Paulsson2019; Schulz Paulsson, Reference Schulz Paulsson2019). A recent analysis of the painted boats at Laja Alta (Cadiz) suggests sailing seafaring during the first half of the fourth millennium BCE (Morgado Rodríguez et al., Reference Morgado Rodríguez, García-Alfonso, García del Moral, Benavides, Rodríguez-Tovar and Esquivel2018). This interaction between various Atlantic regions of Iberia and France was also the background to the “arrival” of the Neolithic in the British Isles, recently dated to ca. 4050 cal BCE (Whittle, Reference Whittle2018, p. 5), which obviously required seaworthy boats. Interestingly, recent aDNA analyses have shown that the genetic ancestry of early Neolithic settlers in the British Isles was closer to that of Iberian and Mediterranean populations than central European populations (Brace et al., Reference Brace, Diekmann, Booth, Van Dorp, Faltyskova, Rohland and Mallick2019; Rivollat et al., Reference Rivollat, Choongwon, Schiffels, Küçükkalipçi, Pemonge, Rohrlach and Alt2020).
Therefore, within a supraregional scale of analysis, the construction of Menga occurred at a time of increased mobility and interaction between regions in Atlantic Europe, which may have led to the spread of proficiency in megalith-building techniques. Can the lack of precedents for a monument as fine and colossal as Menga be explained by the participation of “foreign” experts? At this time, and with the evidence available, this cannot be tested, but the newly established date for the inception of the monument opens up this possibility for future analysis. Only further research will help us to understand the apparent contradiction posed by Menga's uniqueness and perfection on the one hand, and the lack of obvious local precedents on the other.
What is beyond doubt, however, is that, upon its construction, Menga gained huge fame. Over time, it would act as a permanent focus of monumentality, ritual practice, and social action in the region. The Viera dolmen, as well as the recently discovered Piedras Blancas megalithic structure, both very likely built at the end of the fourth millennium, and El Romeral tholos, built sometime during the third millennium would have acted as “responses” provided by the local communities to the glorious past represented by the great Menga dolmen as a part of a powerful sense of place-keeping.
CONCLUSION
Through a careful combination of geological and archaeological observations, coupled with a multimethod approach based on 29 new radiocarbon, OSL, and U/Th numerical dates, an innovative and robust approach is made to a problem that for a long time was deemed as almost intractable: the establishment of the construction date of major megalithic monuments. Monumentality is one of the most challenging subjects in the study of early social complexity. The erection of monuments is closely linked to the rise and consolidation of the Neolithic way of life and associated phenomena, such as sedentarization, demographic growth, agricultural intensification, surplus accumulation, craft specialization, increased social and gender differentiation, ritual central places, political hierarchization, and supraregional connectivity. In Europe, major sites of ritual character involving massive earthen monuments (such a ditched enclosures and henges) and megaliths were built between the fifth and third millennia BCE, in what represents a remarkable era in human social evolution. In the case study examined here, not only is a fairly reliable date obtained for the construction of Menga, one the largest megalithic monuments in Europe, but significant information is also gathered regarding the circumstances that surrounded its construction almost 6000 years ago.
In addition to the importance this study has for our understanding of the Antequera monumental landscape, there are significant methodological lessons to be drawn from it. Systematic multimethod dating may and will encounter problems. In the case study presented here, this is reflected in the impossibility of dating calcite crusts from El Toro, the lack of consistency between the radiocarbon and OSL dates obtained for the fall of the El Toro roof slab, and the chronometric “noise” produced by the estimated ages of hand-thrown pottery from Menga's atrium. In addition, the “synchronization” of multiple ages based on a wide array of methods demands time, resources, and a significant amount of multidisciplinary expertise. Despite these problems, however, a multimethod approach can successfully contribute to the establishment of construction dates of major prehistoric monuments, which in turn is a sine qua non condition to comprehend the social and cultural backgrounds they emerged from and were part of.
Acknowledgments
This research presented in this paper was funded by two projects of the National R&D Plan of the Spanish Government: “Megalithic Biographies: The Antequera Megalithic Landscape in Its Temporal and Spatial Context” (HAR2017-87481-P, 2018-2021), and “Nature, Society and Monumentality: High-Resolution Archaeological Investigations of the Antequera Megalithic Landscape” (HAR2013-45149-P, 2014-2017). We would like to thank Francisco Crespo Fuillerat, Charo Moreno, Andrés Muñoz Martínez, Emilia Pérez Díaz, and Domingo Ruiz Canto of the Underground Exploration Group of the Sociedad Excursionista de Málaga for their contribution in obtaining the El Aguadero sinkhole speleothems. Grateful acknowledgments are also made to Fundação para a Ciência e a Tecnologia (FCT, Portugal), through the UID/Multi/04349/2020 and post-doctoral grant SFRH/BPD/114986/2016. We also thank Francisco Jiménez Espejo and José Antonio Lozano Rodriguez for their invaluable help while we were writing this paper, and Francisco Carrión Méndez for generously granting access to the records of his 2005-2006 excavations at Menga.