Creatine is essential for normal brain development. We know this because infants with inborn errors in either creatine synthesis or transport develop a consistent series of neurological symptoms, including speech delay, mental retardation and epilepsy(Reference Battini, Alessandri and Leuzzi1–Reference Schulze3). Nevertheless, the great bulk of the body's creatine and creatine phosphate occurs in muscle, and it is clear that these molecules play critical roles in the buffering of ATP levels, the vectorial transport of high-energy phosphates and in the control of the production of reactive oxygen species by mitochondria(Reference Walker4–Reference Guidi, Potenza and Sestili6). The skeletal muscle content of creatine plus creatine phosphate is of the order of 25–30 μmol/g wet weight. The brain content of these molecules is perhaps half that of muscle(Reference Brosnan, Wijekoon and Warford-Woolgar7). The total body content of creatine plus creatine phosphate is quite high, approximately 100 g in an adult human(Reference Wyss and Kaddurah-Daouk8). Creatine and creatine phosphate are spontaneously and irreversibly broken down to creatinine at a rate of 1·7 %/d. Creatinine is excreted in the urine. Therefore, adults have a continuous need to replace creatine, usually by a combination of diet and endogenous synthesis(Reference Brosnan and Brosnan9). In addition to the replacement of this creatine lost as creatinine, creatine must also be supplied to the infant's expanding tissue mass. There are only two ways in which a growing infant may acquire creatine; it is either provided in mother's milk (or in infant formula) or is synthesised de novo by the infant. The situation with formula-fed infants is more complex and depends upon the type of formula used. The present study examines the creatine and guanidinoacetate (GAA, a metabolic precursor of creatine) content of mother's milk and various infant formulas, and estimates the degree to which diet or endogenous synthesis provides creatine to the growing infant. The implications of the quantity of dietary creatine available to infants suffering from creatine deficiency syndromes as well as the burden that creatine synthesis places on an infant's amino acid metabolism are discussed.
Experimental methods
A total of twenty healthy lactating women (31 (sd 5) years of age) donated milk at either 1–2 or 5–6 weeks postpartum (ten subjects at each time period). The milk was collected at the Janeway Children's Health and Rehabilitation Centre, St John's, using electronic breast pumps. Blood was taken from these subjects by nurses at the Janeway Blood Collection Services. None of the subjects was vegetarian. All protocols were approved by both the Memorial University Human Investigation Committee and the Research Approval Committee of the Health Care Corporation of St John's. Informed written consent was obtained before the collection of any human samples.
Furthermore, eight different types of infant formula were purchased from various department stores in St John's. Formulas from six different lots of each type were purchased, except for Isomil, for which samples from only five different lots were available. Each formula was reconstituted according to the manufacturers' instructions and an aliquot of each infant formula, or human milk sample, was centrifuged at 4°C at 150 000 g for 60 min so as to separate the aqueous phase. Blood samples were collected with lithium heparin and centrifuged at 4°C at 3000 g for 10 min to separate the plasma. Samples of the aqueous phase of the milk and formula samples, and of the plasma samples, were deproteinised by the addition of 50 % (v/v) of 0·6 m-perchloric acid, kept on ice for 20 min and centrifuged at 20 000 g for 5 min to sediment the proteins. To 1·5 ml aliquots of the resulting supernatants were added 25 μl of 50 % potassium carbonate and 20 μl of universal indicator before neutralisation with 20 % potassium hydroxide. The deproteinised, neutralised samples were used for the measurement of creatine by the enzymatic method of Lowry & Passonneau(Reference Lowry and Passonneau10) and GAA by the HPLC method of Carducci et al. (Reference Carducci, Birarelli and Santagata11).
Data are represented as means and standard deviations. Statistical significance was determined by means of a one-way ANOVA followed by the Newman–Keuls post hoc test. A P value of < 0·05 was taken to indicate statistical significance.
Results and discussion
Fig. 1 shows the concentrations of creatine and GAA in human milk and plasma. Since there was no difference between the concentrations measured at 1–2 or 5–6 weeks postpartum, these data were combined. Both plasma and milk contained appreciable concentrations (60–70 μm) of creatine, but creatine was not more concentrated in milk than in plasma. The circulating concentration of GAA was much lower than that of creatine and was even lower still in milk. It is clear, therefore, that milk is a source of creatine for the nursing infant but that GAA is not supplied in significant quantities by this route.
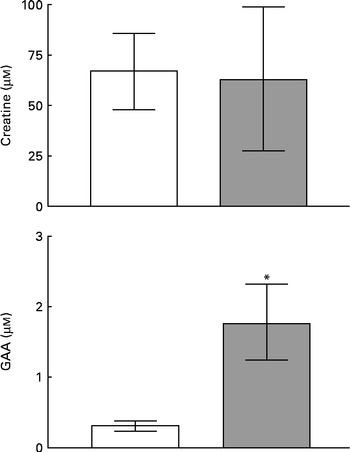
Fig. 1 Concentrations of creatine and guanidinoacetate (GAA) in human milk (□) and plasma (). Values are means of five to twenty observations, with standard deviations represented by vertical bars. * Mean value was significantly different from that for human milk (P= 0·004).
Table 1 shows the creatine and GAA content of the infant formulas. There were appreciable concentrations of creatine in all of the cows' milk-based formulas; indeed, these were substantially higher than the concentrations found in human milk, which is consistent with the very high creatine concentration (550 μm) that we measured in cows' milk. On the other hand, the creatine concentrations of Isomil, Lactofree and Nestlé Alsoy 1 were very low (10–13 μm). Isomil and Alsoy 1 are soya-based. Lactofree is made with modified cows' milk ingredients and is intended for infants that suffer from lactose intolerance. The concentration of GAA in the infant formulas was very low.
Table 1 Creatine and guanidinoacetate concentrations in various infant formulas (Mean values and standard deviations, n 6 (except n 5 for Nestlé Alsoy 1))
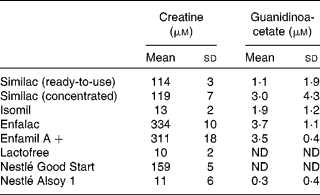
ND, values which could not be determined.
It is of interest to estimate the extent to which mother's milk provides the nursing infant with the necessary creatine. To do this, we need to calculate creatine intake in mother's milk as well as the accretion of creatine by the infant. We have selected as our reference breast-fed infants between 6 and 12 weeks of age. These infants weigh an average of 5·7 kg, ingest an average of 143 ml of milk/kg per d and gain an average of 0·2 kg body weight/week(Reference Motil, Hwai-Peng and Montandon12). Since the muscle mass of infants of this age is about 25 % of body mass(Reference Graystone and Cheek13), this amounts to a gain of 50 g of muscle/week. These infants excrete an average of 62 mg creatinine/d, or 3·8 mmol/week(Reference Coulthard, Hey and Al-Dahhan14). From the data in Fig. 1, we can calculate that these breast-fed infants ingest about 0·4 mmol creatine/week. There are no data on the bioavailability of creatine in infants. However, creatine bioavailability has been determined to be 79 % in adult humans(Reference MacNeil, Hill and MacDonald15). In addition, it is known that the transporter responsible for creatine absorption is elevated in suckling rat pups(Reference Peral, Galvez and Soria16). It is likely, therefore, that the bioavailability of milk creatine is very high in suckling infants.
The accretion of creatine by these infants can also be estimated. First of all, it is necessary to replace the creatine lost to creatinine formation and excretion, 3·8 mmol/week. In addition, it is necessary to provide creatine to the expanding muscle mass. According to Eriksson(Reference Eriksson17), the skeletal muscle content of creatine phosphate in infants of this age is between 12 and 22 mmol/kg wet weight. This gives a mean value of 17 mmol/kg wet weight. We were unable to find any recently published data on the creatine concentration or total creatine content of infant muscle. However, applying a ratio of 3 for creatine phosphate/creatine, which is common in adult human muscle(Reference Kemp, Meyerspeer and Moser18), we estimate creatine concentration in infant skeletal muscle to be approximately 6 mmol/kg wet weight, for a total muscle creatine concentration of 23 mmol/kg wet weight. This is somewhat lower than concentrations found in adult muscle, which is consistent with measurements of creatine in adult and infant muscle reported in the early twentieth century(Reference Denis19). However, since the methodology used in these early studies is not nowadays considered to be very reliable, we have not employed them in our calculations. Applying our new estimates of infant muscle creatine content with an increase in muscle mass of 50 g/week, we calculate that 0·46 mmol creatine/week is required for the expanded muscle mass. Together with the 3·8 mmol creatine lost as creatinine, we estimate that infants of this age require 4·26 mmol creatine/week. Since they only receive about 0·4 mmol/week via milk, almost 90 % of their creatine must be provided by de novo synthesis by the infant. These findings are in accord with our study of creatine accretion in rat pups(Reference Lamarre, Edison and Wijekoon20) and piglets(Reference Brosnan, Wijekoon and Warford-Woolgar21) where we showed that milk provided, respectively, only 12 and 25 % of total creatine accretion, the balance being provided by endogenous synthesis by the neonatal animal. The high rate of endogenous creatine synthesis has important implications both for infants suffering from creatine deficiency syndromes and for the infant's amino acid metabolism.
The fact that infants receive almost all of their creatine by endogenous synthesis is entirely consistent with the speed with which symptoms become apparent in infants suffering from genetic defects in creatine synthesis. Symptoms become apparent at an early age, regardless of whether the defect is due to an enzyme or a transporter deficiency. These symptoms include highly abnormal developmental scores, mental retardation, speech impairment and epileptic seizures(Reference Battini, Alessandri and Leuzzi1–Reference Schulze3).
It is important to analyse the metabolic burden that the synthesis of approximately 3·9 mmol creatine/week places on these infants. Creatine synthesis takes place by means of a very simple pathway, employing only two enzymes, l-arginine:glycine amidinotransferase and GAA methyltransferase (see Fig. 2). l-Arginine:glycine amidinotransferase catalyses a reaction between arginine and glycine, to produce GAA and ornithine:

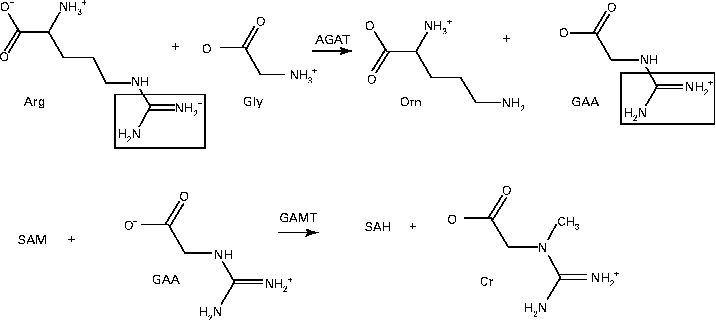
Fig. 2 Creatine (Cr) synthesis. l-Arginine:glycine amidinotransferase (AGAT) first catalyses the transfer of an amidino group from arginine (Arg) to glycine (Gly) to produce ornithine (Orn) and guanidinoacetate (GAA). Guanidinoacetate methyltransferase (GAMT) then catalyses the methylation of GAA from S-adenosylmethionine (SAM) to produce S-adenosylhomocysteine (SAH) and Cr.
GAA methyltransferase uses S-adenosylmethionine (SAM) to methylate GAA to creatine and S-adenosylhomocysteine (SAH):

In this pathway, the entire glycine molecule is incorporated into creatine as well as a guanidino group from arginine and a methyl group from SAM.
Using the data provided by Davis et al. (Reference Davis, Nguyen and Garcia-Bravo22) on the amino acid composition of human milk, we calculate that 6–12-week-old infants ingest an average of 14·3, 10 and 5·2 mmol/week, respectively, of glycine, arginine and methionine. Therefore, creatine synthesis consumes 27 % of dietary glycine. However, glycine is a non-essential amino acid and may be produced endogenously by the infant. Creatine synthesis also consumes guanidino groups corresponding to 39 % of those in dietary arginine and methyl groups corresponding to 75 % of those in dietary methionine. l-Arginine:glycine amidinotransferase converts arginine to ornithine, and this ornithine may be reconverted to arginine by the combined actions of carbamoyl phosphate synthetase I, argininosuccinate synthetase and argininosuccinate lyase. We are aware of no data on the rate of this reconversion in infants. SAH, produced by GAA methyltransferase, is hydrolysed by SAH hydrolase to adenosine and homocysteine; homocysteine may be remethylated to methionine either by methionine synthase or betaine:homocysteine methyltransferase. We are aware of no data on the rate of remethylation in 6–12-week-old infants. However, Thomas et al. (Reference Thomas, Gruca and Bennett23) have shown that remethylation is quite active in newborn infants; therefore, it is likely that much of the SAM required for creatine synthesis is regenerated via the remethylation pathway.
In summary, these calculations reveal that the great bulk of the nursing infant's need for creatine must be met by endogenous synthesis and that this imposes a considerable metabolic burden on the growing infant, particularly with regard to amino acid metabolism. Formula-fed infants receiving cows' milk-based formula receive two to four times as much creatine as nursing infants depending upon the formula of choice. Therefore, the metabolic burden of endogenous creatine synthesis may be reduced, yet remains an appreciable burden on amino acid requirements even in these infants.
Acknowledgements
The present study was supported by grants from the Canadian Foundation for Health Research (to J. T. B. and M. E. B.) and from the Janeway Research Foundation (to J. T. B., M. E. B. and K. A.). J. T. B., E. E. E., M. E. B. and K. A. designed the research; E. E. E. conducted the research; E. E. E. analysed the data; E. E. E. and J. T. B. wrote the paper and had primary responsibility for the final content. All authors read and approved the final manuscript. The authors have no conflicts of interest.