Introduction
Phenotypic plasticity is defined as the ability of a genotype to produce multiple phenotypes in response to environmental conditions (Roff, Reference Roff2002; Miner et al., Reference Miner, Sultan, Morgan, Padilla and Relyea2005). In organisms with complex and diverse life cycles such as parasites, different environmental conditions include (1) the host's immune system, (2) different host species and (3) the geographical distribution of the definitive hosts (Poulin, Reference Poulin2007). The recent application of molecular markers has helped to define, recognize, delineate and better understand intraspecific variation that can be attributed to differences in the development and phenotypic plasticity of acanthocephalans (Steinauer et al., Reference Steinauer, Nickol and Ortí2007; Rosas-Valdez et al., Reference Rosas-Valdez, Morrone and García-Varela2012, Reference Rosas-Valdez, Morrone, Pinacho-Pinacho, Domínguez-Domínguez and García-Varela2020; Alcántar-Escalera et al., Reference Alcántar-Escalera, García-Varela, Vázquez-Domínguez and Pérez Ponce de León2013; Perrot-Minnot et al., Reference Perrot-Minnot, Špakulová, Wattier, Kotlík, Düsen, Ayogdu and Tougard2018; Pinacho-Pinacho et al., Reference Pinacho-Pinacho, García-Varela, Sereno-Uribe and Pérez Ponce de León2018a; Lisitsyna et al., Reference Lisitsyna, Kudlai, Spraker, Tkach, Smales and Kuzmina2019).
The Neoechinorhynchidae (Ward, 1917) Van Cleave, 1928 is a large, globally distributed family of acanthocephalans, which are typically parasites from the intestine of teleost fishes and freshwater turtles. At present, the family includes 14 genera split into four subfamilies (Gibson and Wayland, Reference Gibson and Wayland2022). The type genus Neoechinorhynchus Stiles and Hassall, 1905 represents a hyperdiverse group of endoparasites of marine, freshwater, brackish water fishes and freshwater turtles, with ~117 species distributed worldwide (Amin, Reference Amin2013; Smales, Reference Smales2013; Pinacho-Pinacho et al., Reference Pinacho-Pinacho, García-Varela, Sereno-Uribe and Pérez Ponce de León2018a; Amin et al., Reference Amin, Chaudhary, Heckmann, Ha and Singh2019, Reference Amin, Sharifdini, Heckmann, Rubtsova and Chine2020). In the Americas, 50 species have been described: 33 in North America, corresponding to the Nearctic biogeographical region, and 17 in Middle and South America, corresponding to the Neotropical biogeographical region (Amin, Reference Amin2013; Pinacho-Pinacho et al., Reference Pinacho-Pinacho, Sereno-Uribe, García-Varela and Pérez Ponce de León2018b). Members of Neoechinorhynchus have been the target of numerous studies related to their ecology, host–parasite relationships, pathogenicity, taxonomy and systematics (see references in Kennedy, Reference Kennedy2006; Pinacho-Pinacho et al., Reference Pinacho-Pinacho, Pérez-Ponce de León and García-Varela2012, Reference Pinacho-Pinacho, Sereno-Uribe and García-Varela2014, Reference Pinacho-Pinacho, García-Varela, Sereno-Uribe and Pérez Ponce de León2018a; Amin, Reference Amin2013; Smales, Reference Smales2013; Amin et al., Reference Amin, Sharifdini, Heckmann, Rubtsova and Chine2020; Koch et al., Reference Koch, Shannon, Detwiler and Bolek2021).
The first morphological and molecular phylogenies inferred with a few species of Neoechinorhynchus revealed that the genus is paraphyletic (Pinacho-Pinacho et al., Reference Pinacho-Pinacho, Hernández-Orts, Sereno-Uribe, Pérez Ponce de León and García-Varela2017). However, one of the most emblematic and enigmatic groups of the genus Neoechinorhynchus from North America associated with emydid turtles (Barger, Reference Barger2004; Barger and Nickol, Reference Barger and Nickol2004) formed a monophyletic assemblage (García-Varela and Pinacho-Pinacho, Reference García-Varela and Pinacho-Pinacho2018; Koch et al., Reference Koch, Shannon, Detwiler and Bolek2021). The taxonomy of the 10 currently described species of Neoechinorhynchus associated with emydid turtles is complex due to their morphological homogeneity. Species delimitation relies entirely on characteristics of the female worm, including the contour of the posterior end and the shape and membrane structure of the fully formed egg, whereas male worms are nearly identical among species (Cable and Hopp, Reference Cable and Hopp1954; Barger, Reference Barger2004; Barger and Nickol, Reference Barger and Nickol2004). In addition, Dezfuli and Tinti (Reference Dezfuli and Tinti1998) and Barger and Nickol (Reference Barger and Nickol2004) mentioned that species of Neoechinorhynchus from turtles exhibit great phenotypic plasticity, which may lead to the misclassification of female specimens and leave males of different species completely indistinguishable. Barger (Reference Barger2004) performed one of the most comprehensive taxonomic reviews of Neoechinorhynchus species that infect emydid turtles from North America, acknowledging that eastern USA represents a biodiversity hot spot for this group of acanthocephalans. Barger reported that Neoechinorhynchus emyditoides Fisher, Reference Fisher1960 has the widest distribution range extending from the eastern USA to southeastern Mexico through the Gulf of Mexico.
As part of our long-term studies on the biodiversity of helminth parasites of turtles, acanthocephalans belonging to the Neoechinorhynchidae were recovered from the intestines of emydid turtles from five localities in northern and southeastern Mexico. Our extensive sampling allowed us recognize morphologically two species of acanthocephalans, i.e. Neoechinorhynchus schmidti Barger, Thatcher and Nickol, 2004 and N. emyditoides.
The objective of the current study was to combine morphological and molecular characteristics to investigate the phenotypic plasticity of N. emyditoides along its distribution range and explore the genetic structure of populations by using sequences of the cytochrome c oxidase subunit 1 (cox1) from mitochondrial DNA. In addition, we briefly discuss the systematics of Neoechinorhynchus species associated with emydid turtles by using sequences of two molecular markers: the D2 + D3 domain of the large subunit (LSU) and the internal transcribed spacer region, including 5.8S (ITS) from nuclear ribosomal DNA.
Materials and methods
Specimen collection
A total of 47 turtles including 28 specimens of Meso-American slider [Trachemys scripta venusta (Gray)], 6 specimens of ornate slider [Trachemys ornata (Gray)], 2 of red-eared slider [Trachemys scripta elegans (Schoepff)], 4 of yellow mud turtle [Kinosternon flavescens (Agassiz)], plus 7 specimens of Mexican musk turtle [Staurotypus triporcatus (Wiegmann)] were collected from previous field expedition [see Pinacho-Pinacho et al. (Reference Pinacho-Pinacho, Sereno-Uribe and García-Varela2014, Reference Pinacho-Pinacho, García-Varela, Sereno-Uribe and Pérez Ponce de León2018a) and García-Varela and Pinacho-Pinacho (Reference García-Varela and Pinacho-Pinacho2018) in 9 localities across Mexico (Fig. 1; Table S1)]. Turtles were dissected within 2 h after capture; their viscera were placed in separate Petri dishes containing a 0.75% saline solution and examined under a dissecting microscope. The turtles identified as T. scripta venusta were positive for the infection with acanthocephalans, which were washed in 0.75% saline solution and placed in distilled water at 4°C overnight and subsequently preserved in 100% ethanol. Turtles were identified using the field guide of Legler and Vogt (Reference Legler and Vogt2013).

Fig. 1. Map of Mexico showing the sampled sites for the turtles. Localities with a circle were positive for the infection with Neoechinorhynchus emyditoides. Localities with a triangle were negative for the infection. Localities: (1) Huizache, Sinaloa; (2) La Tovara, Nayarit; (3) Tres Palos, Guerrero; (4) Monterrey, Nuevo Leon; (5) Purificación river, Tamaulipas; (6) Tlacotalpan, Veracruz; (7) Catemaco, Veracruz; (8) Pantanos de Centla, Tabasco; (9) Holcá, Yucatán.
Morphological study
For taxonomic identification, specimens were stained with Mayer's paracarmine, dehydrated in a graded ethanol series, cleared with methyl salicylate and mounted on permanent slides with Canada balsam. Mounted specimens were examined under a bright-field Leica DM 1000 LED microscope (Leica, Wetzlar, Germany), and drawings were made using a drawing tube attached to the microscope. Measurements were taken using Leica Application Suite microscope software (Leica) and are given in micrometres (μm).
Vouchers of N. emyditoides from Tlacotalpan, Veracruz (No. 6695) and the new vouchers (Nos. 11647–11653) were examined and deposited in the Colección Nacional de Helmintos (CNHE), Instituto de Biología, Universidad Nacional Autónoma de México (UNAM), Ciudad de México, Mexico. The species identification was conducted following the revision of the genus Neoechinorhynchus by Barger (Reference Barger2004) and the original description (Fisher, Reference Fisher1960). For scanning electron microscopy (SEM), two specimens of N. emyditoides of each locality sampled were dehydrated with an ethanol series, critical point dried, sputter coated with gold and examined with a Hitachi Stereoscan Model S-2469N scanning electron microscope operating at 15 kV from the Instituto de Biología, UNAM.
Amplification and sequencing of DNA
A total of seven specimens identified as N. emyditoides were analysed. The posterior end of four specimens, two male and two female (hologenophores; Pleijel et al., Reference Pleijel, Jondelius, Norlinder, Nygren, Oxelman, Schander, Sundberg and Thollesson2008), were used for DNA extraction, whereas the rest of the body was stained with Mayer's paracarmine and mounted on permanent slides with Canada balsam. In addition, one female of N. emyditoides from Purification River was cut-off into three sections. The anterior and posterior sections were processed for SEM, whereas the middle section was processed for DNA extraction. Finally, other two specimens identified as N. emyditoides were placed individually in tubes and digested overnight at 56°C in a solution containing 10 mm Tris–HCl (pH 7.6), 20 mm NaCl, 100 mm Na2 EDTA (pH 8.0), 1% sarkosyl and 0.1 mg mL−1 proteinase K. Following digestion, DNA was extracted from the supernatant using the DNAzol reagent (Molecular Research Center, Cincinnati, OH, USA) according to the manufacturer's instructions. The domains D2 + D3 and ITS from nuclear ribosomal DNA and the cox1 from mitochondrial DNA were amplified using polymerase chain reaction (PCR). The domains D2 + D3 from LSU of approximately 700 bp were amplified using forward primer 502, 5′-CAA GTA CCG TGA GGG AAA GTT GC-3′ and reverse primer 536, 5′-CAG CTA TCC TGA GGG AAAC-3′ (García-Varela and Nadler, Reference García-Varela and Nadler2005). A fragment of approximately 750 bp from ITS was amplified using the forward primer BD1, 5′-GTC GTA ACA AGG TTT CCG TA-3′ and the reverse primer BD2, 5′-ATC TAG ACC GGA CTA GGC TGT G-3′ (Luton et al., Reference Luton, Walker and Blair1992). The cox1 of approximately 550 bp was amplified using the forward primer 516, 5′-ATT TTT TAG TTT GAG TGT GAG GAG-3′ and the reverse primer 517, 5′-ATGA CGA ATT AAT ATT ACG ATC CA-3′ (see Pinacho-Pinacho et al., Reference Pinacho-Pinacho, García-Varela, Sereno-Uribe and Pérez Ponce de León2018a, Reference Pinacho-Pinacho, Sereno-Uribe, García-Varela and Pérez Ponce de León2018b). The amplification reactions (25 μL) consisted of 1 μL of each primer (10 μ m), 2.5 μL of 10× buffer, 1.5 μL of 2 mm MgCl2, 0.5 μL of dNTPs (10 mm), 16.37 μL of water, 2 μL of genomic DNA and 1 U of Taq DNA polymerase (Platinum Taq, Invitrogen Corporation, São Paulo, Brazil). The PCR cycling conditions for amplification included denaturation at 94°C for 3 min, followed by 35 cycles of 94°C for 1 min, annealing at 50°C for LSU, ITS and 40°C for cox1 for 1 min and extension at 72°C for 1 min, with a final postamplification incubation at 72°C for 10 min. The sequencing reactions were performed using the initial primers for LSU, ITS and cox1, plus two internal primers: 503, 5′-CCT TGG TCC GTG TTT CAA GAC G-3′ and 504, 5′-CGT CTT GAA ACA CGG ACT AAGG-3′ for LSU (García-Varela and Nadler, Reference García-Varela and Nadler2005). Sequencing reactions were performed using ABI Big Dye (Applied Biosystems, Boston, MA, USA) terminator sequencing chemistry, and the reaction products were separated and detected using an ABI 3730 capillary DNA sequencer. Contigs were assembled, and base-calling differences were resolved using CodonCode Aligner 9.0.1 (CodonCode Corporation, Dedham, MA, USA). Newly generated sequences were deposited in the GenBank database under the accession numbers; Hologenophore, male (OM892136 and OM892138 for LSU; OM892142 and OM892144 for ITS; OM891889 for cox1), female (OM892137 and OM892139 for LSU; OM892143 and OM892145 for ITS; OM891888 and OM891890 for cox1). The accession numbers for the female of N. emyditoides cut-off into three sections were OM892146 for ITS and OM892140 for LSU. Finally, the accession numbers of the other specimens were OM892141 for LSU and OM892147-148 for ITS.
Alignments and phylogenetic analyses
Sequences obtained in the current research for LSU and ITS were aligned with other congeneric species downloaded from GenBank (see Table 1). In addition, sequences of the LSU and ITS of other genera, such as Polyacanthorhynchus Travassos 1920, Acanthosentis Verma and Datta 1929, Floridosentis Ward 1953, Mayarhynchus Pinacho-Pinacho, Hernández-Orts, Sereno-Uribe, Pérez-Ponce de León and García-Varela, 2017, and Atactorhynchus Chandler 1935, were used as outgroup. Sequences of each nuclear molecular marker were aligned separately using the software Clustal W with default parameters implemented in MEGA version 7.0 (Kumar et al., Reference Kumar, Stecher and Tamura2016). The best-fitting nucleotide substitution models for LSU and ITS dataset were GTR + G + I and were estimated with the Akaike Information Criterion (AIC) implemented in MEGA version 7.0 (Kumar et al., Reference Kumar, Stecher and Tamura2016). The phylogenetic analyses were inferred through maximum likelihood (ML) with the program RAxML v7.0.4 (Silvestro and Michalak, Reference Silvestro and Michalak2012) and Bayesian inference (BI) criteria, employing the nucleotide substitution model identified for AIC. BI trees were generated using MrBayes v3.2 (Ronquist et al., Reference Ronquist, Teslenko, Van Der Mark, Ayres, Darling, Höhna, Larget, Liu, Suchard and Huelsenbeck2012), running two independent MC3 runs of four chains for 5 million generations and sampling tree topologies every 1000 generations. ‘Burn-in’ periods were set to 1 million of generations according to the standard deviation of split frequencies values (<0.01). To support each node, 10 000 bootstrap replicates were run with the ML method. Posterior probabilities of clades were obtained from 50% majority rule consensus of sample trees after excluding the initial 20% as ‘burn-in’. The genetic divergence among taxa was estimated using uncorrected ‘p’ distances with the program MEGA version 7.0 (Kumar et al., Reference Kumar, Stecher and Tamura2016).
Table 1. Specimens information, GenBank accession numbers of LSU, ITS and cox 1

Sequences in bold were generated in the current study.
a Source for sequences misidentified as Octospiniferoides sp.
To examine cox1 haplotype frequency among the populations of N. emyditoides, an unrooted statistical network was constructed using the PopART program with the median-joining algorithm (Bandelt et al., Reference Bandelt, Forster and Röhl1999). The degree of genetic differentiation among the populations was estimated using the fixation indices F st (Hudson et al., Reference Hudson, Boos and Kaplan1992), with the program Arlequin v.3.5 (Excoffier and Lischer, Reference Excoffier and Lischer2010). To investigate the population history and demography, Tajima's D (Tajima, Reference Tajima1989) and Fu's F (Fu, Reference Fu1997) tests were calculated using DnaSP v. 5. 10 (Rozas et al., Reference Rozas, Sánchez-DelBarrio, Messeguer and Rozas2003). Values less than 0.05 were considered statistically significant.
Results
Morphological identification
The acanthocephalans were collected from several previous field expeditions in four localities (Fig. 1; Table 1), two from the northern Mexico and two from the southeastern Mexico (Figs 2 and 3). The acanthocephalans exhibited the typical morphological characteristics of N. emyditoides. For example, trunk elongated cylindrical, slender, curved ventrally, body wall with reticular lacunar system, usually five (occasionally three) dorsal giant hypodermic nuclei and one ventral (Fig. 2C–D). Proboscis nearly cylindrical, longer than wide (Figs 2A and 3A–D). Anterior hooks markedly larger than middle hooks not in a perfect circle but at different levels, posterior hooks smaller than middle hooks (Figs 2A and 3A–H). Neck short, wider than longer. Lemnisci appreciably different in length; longer than the proboscis receptacle. Proboscis receptacle single-walled (Fig. 2B). Male with two oblong testes, contiguous or slightly overlapping, equatorial, just behind anterior trunk; anterior testis relatively longer than the posterior testis. Prominent cement gland elongated, longer than anterior testis. Rounded-ovoid cement gland reservoir with two lateral ducts. Saefftingen's pouch beginning at level of anterior end of the cement reservoir duct (Fig. 2E). Posterior end of the female usually rounded with two lobes (Figs 2F and 3I–L), short vagina, slim uterus and uterine bell attached to the anterior body wall (Fig. 2F). Elliptical eggs, slightly inflated at the poles (Fig. 2G). These characteristics of our new morphometric data correspond to those reported previously (see Table 2) (Bravo-Hollis, Reference Bravo-Hollis1946; Fisher, Reference Fisher1960; Barger, Reference Barger2004).
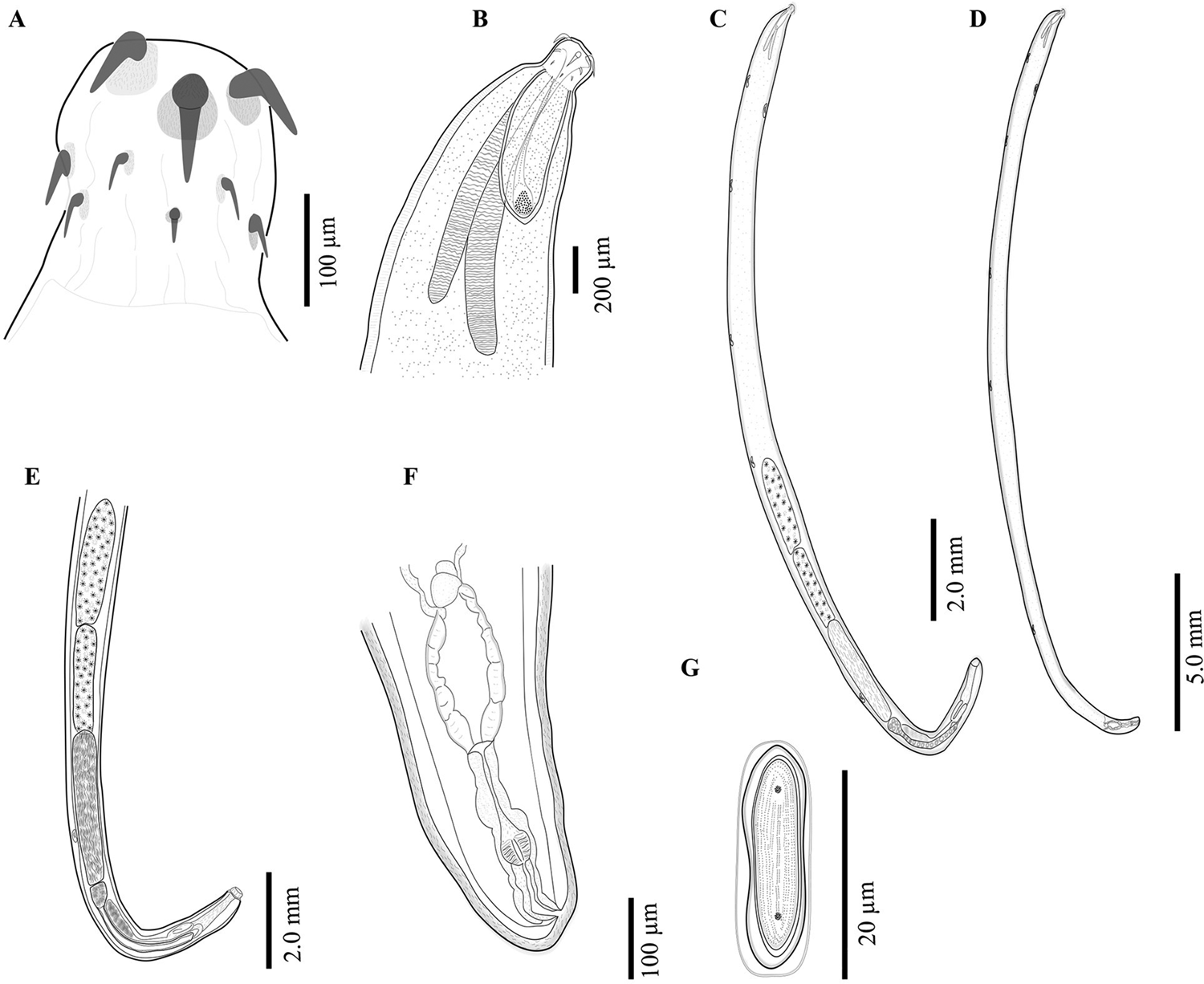
Fig. 2. Neoechinorhynchus emyditoides from Trachemys scripta venusta from Catemaco Veracruz, Mexico. Proboscis (A); male anterior region (B); adult male, whole worm (C); adult female, whole worm (D); male reproductive system (E); female reproductive system (F); egg (G).

Fig. 3. Scanning electron micrographs of proboscis (A–H) and anterior region of adult female (I–L) of Neoechinorhynchus emyditoides of the four populations sampled; Purificación river, Tamaulipas (A, E, I) from Trachemys scripta venusta; Tlacotalpan, Veracruz (B, F, J) from Trachemys scripta venusta; Catemaco, Veracruz (C, G, K) from Trachemys scripta venusta; Monterrey, Nuevo Leon (D, H, L) from Trachemys scripta elegans. Scale bars = 100 μm.
Table 2. Comparative measurements of specimens of Neoechinorhynchus emyditoides

N, number of specimens used; *, immature specimens.
Remarks
Fisher (Reference Fisher1960) recognized two previous described species [Neoechinorhynchus pseudemydis Cable and Hopp, Reference Cable and Hopp1954 and Neoechinorhynchus emydis (Leidy, 1851) Van Cleave, 1919] and described the species N. emyditoides from diverse definitive hosts: Trachemys scripta scripta, T. scripta elegans, T. ornata and Emydoidea blandingii (Holbrook), from Arkansas, Texas, Virginia, Massachusetts, California zoo in the USA, and southeastern Mexico (Bravo-Hollis, Reference Bravo-Hollis1946; Fisher, Reference Fisher1960). Barger (Reference Barger2004) and Barger and Nickol (Reference Barger and Nickol2004) performed one of the most comprehensive taxonomic reviews of the species of Neoechinorhynchus that infects emydid turtles, mentioning that N. emyditoides is the species with the most extensive distribution range including 11 states from eastern USA and single state (Veracruz) from southeastern Mexico. In the current research, we analysed the morphology of specimens from four populations of N. emyditoides from Mexico, exhibiting a wide phenotypic plasticity along its distribution. For instance, newly collected material showed some level of morphological intraspecific variation with respect to previous descriptions performed by Fisher (Reference Fisher1960), Bravo-Hollis (Reference Bravo-Hollis1946) and García-Varela et al. (Reference García-Varela, García-Prieto and Rodríguez2011) (see Table 2). Interestingly, the specimens from Mexico possess lower limits for the following characteristics: body size, receptacle proboscis and proboscis hooks length with respect to original description (see Table 2). Cable and Hopp (Reference Cable and Hopp1954) mentioned that the size and shape of fully developed eggs and the posterior end of the female are morphological traits keys on the differentiation at species level. For instance, the female of N. emyditoides is characterized by possessing a posterior end rounded with two lobes. Our specimens showed phenotypic plasticity on the shape of the posterior end (see Fig. 3I–L) (see Barger, Reference Barger2004).
Phylogenetic analyses
The LSU dataset was conformed with 830 characters and 82 sequences, including 23 sequences of 10 Neoechinorhynchus species parasitizing teleost fishes and 46 sequences of five Neoechinorhynchus species associated to emydid turtles plus other sequences from diverse genera that were used as outgroups (see Table 1). The phylogenetic analyses inferred with ML and BI yielded that the genus Neoechinorhynchus is polyphyletic, due to the fact that several genera that were used as outgroups such as Mayarhynchus and Atactorhynchus from the Neoechinorhynchidae were nested inside of Neoechinorhynchus (Fig. 4A). The five species of Neoechinorhynchus analysed associated with emydid turtles formed a clade, which was subdivided into five subclades that correspond with each species analysed as N. pseudemydis, Neoechinorhynchus chrysemydis Cable and Hopp, Reference Cable and Hopp1954, N. emydis, N. schmidti and N. emyditoides with high bootstrap values and Bayesian posterior probabilities (Fig. 4A). In addition, all the samples (including six newly generated sequences) identified as N. emyditoides recovered in the four localities from northern and southeastern Mexico formed a subclade together with other sequence identified previously as N. emyditoides available in the GenBank (MK238158) from Oklahoma, USA (Fig. 4A). The intraspecific genetic divergence among the isolates of N. emyditoides was low that ranged from 0 to 1.6%. Two juvenile worms previously identified as N. emyditoides from Monterrey, Mexico (KR086331–332) were nested in a subclade together with other two sequences available in GenBank (MK238159–160) identified as N. pseudemydis, a parasite of the red-eared slider turtle from Oklahoma, USA. This subclade was supported with high bootstrap values and Bayesian posterior probabilities (Fig. 4A).

Fig. 4. Maximum likelihood tree and consensus Bayesian Inference tree inferred with LSU dataset (A) and ITS dataset (B); numbers near internal nodes show posterior probabilities (BI) and ML bootstrap values. Sequences in bold were generated in this study.
A second dataset with the ITS was conformed with 886 characters and 120 sequences. This alignment included 27 sequences of 12 Neoechinorhynchus species parasitizing teleost fishes and 84 sequences of five Neoechinorhynchus species associated with emydid turtles plus other sequences from diverse genera that were used as outgroups (see Table 1). The phylogenetic analyses inferred with ITS dataset agreed with the LSU tree with respect to polyphyly of Neoechinorhynchus due to the fact that the several genera that were used as outgroup taxa namely Mayarhynchus, Atactorhynchus and Floridosentis from the Neoechinorhynchidae were nested inside Neoechinorhynchus (Fig. 4B). The five species of Neoechinorhynchus associated with emydid turtles formed a single clade, which was subdivided into five subclades that correspond with each species analysed as N. pseudemydis, N. chrysemydis, N. emydis, N. schmidti and N. emyditoides with high bootstrap values and Bayesian posterior probabilities (Fig. 4B). All samples (including seven newly generated sequences) identified as N. emyditoides recovered in the four localities from northern and southeastern Mexico formed a subclade together, with other three sequences identified previously as N. emyditoides available in the GenBank dataset (MW520495–497) from Oklahoma and Texas, USA (Fig. 4B). The intraspecific genetic divergence among the isolates of N. emyditoides was low that ranged from 0 to 0.5%. Two juvenile worms previously identified as N. emyditoides from Monterrey, Mexico (MG870860–861) were nested in a subclade together with other seven sequences available in GenBank (MK238090–901, MW520486–491) identified as N. pseudemydis, plus other nine sequences of Neoechinorhynchus sp. (MW520472–480), recovered from the ostracods of the genus Physocypra from Oklahoma, USA (Fig. 4B).
Haplotype network
A haplotype network was built with the cox1 alignment of 546 bp and contained sequences of 29 specimens (including three newly generated sequences) identified as N. emyditoides from four populations two from northern Mexico (localities; Monterrey, Nuevo León and Purificación river, Tamaulipas) and other two from southeastern Mexico (localities; Catemaco, Veracruz and Tlacotalpan, Veracruz). The haplotype network revealed the presence of 13 haplotypes, two of them (H1, H3) were shared between the localities of Catemaco, Veracruz and Monterrey, Nuevo León and 11 were unique haplotypes (Fig. 5). The level of haplotype diversity (Hd = 0.913) was very high, and nucleotide diversity was low (pi = 0.03728) among the populations (Table 3). We detected low values of F st between the two populations from southeastern Mexico [localities; Catemaco, Veracruz and Tlacotalpan Veracruz (F st = 0.1603)] and between the two populations from northern Mexico [localities; Monterrey, Nuevo León and Purificación river, Tamaulipas (F st = 0.1848)] (see Table 4).

Fig. 5. Haplotype network of samples of Neoechinorhynchus emyditoides, built with the gene cytochrome c oxidase subunit 1 (cox1) from mitochondrial DNA. Each circle represents a haplotype, with size proportional to the haplotype's frequency in the populations.
Table 3. Molecular diversity indices and neutrality tests calculated for cox 1 datasets among the populations of Neoechinorhynchus emyditoides used in this study

n, number of sequences; H, number of haplotypes; S, number of segregating sites; Hd, haplotype diversity; Pi, nucleotide diversity; K, average number of nucleotide differences; TV, Tlacotalpan, Veracruz; CV, Catemaco, Veracruz; ML, Monterrey, Nuevo León; RT, Purificación river, Tamaulipas
Table 4. Pairwise F st values estimated for cox (under the diagonal) and F st P values (above the diagonal)

TV, Tlacotalpan, Veracruz; CV, Catemaco, Veracruz; ML, Monterrey, Nuevo León; RT, Purificación river, Tamaulipas.
Significance level = 0.05.
Discussion
To the best of our knowledge, two species of the genus Neoechinorhynchus (N. schmidti and N. emyditoides) associated with emydid turtles have been recorded in Mexico (Bravo-Hollis, Reference Bravo-Hollis1946; García-Varela et al., Reference García-Varela, García-Prieto and Rodríguez2011; Pinacho-Pinacho et al., Reference Pinacho-Pinacho, García-Varela, Sereno-Uribe and Pérez Ponce de León2018a, Reference Pinacho-Pinacho, Sereno-Uribe, García-Varela and Pérez Ponce de León2018b). The current records suggest that the species do not have a sympatric distribution; for example, N. emyditoides has been recorded from northern Mexico to the Papaloapan River basin, which is considered the second largest hydrobiological system in southeastern Mexico, whereas N. schmidti has been recorded in the Centla Swamps, which is one of the largest wetlands in southeastern Mexico and is formed by the delta of the Grijalva and Usumacinta rivers (González-Ramírez and Parés-Sierra, Reference González-Ramírez and Parés-Sierra2019). The species N. emyditoides was described in red-eared slider turtles (T. scripta elegans) from the St. Francis River, Arkansas, USA (Fisher, Reference Fisher1960). This acanthocephalan is considered one of the biogeographical core parasite fauna of emydid turtles in the eastern USA (Barger, Reference Barger2004; Barger and Nickol, Reference Barger and Nickol2004). In the current study, adults recovered from several emydid turtles from northern and southeastern Mexico were identified as N. emyditoides. Our observations and morphometric data found remarkable morphological differences among the populations from Mexico (see Table 2), including the contour posterior end of the females (Fig. 3). The inclusion of molecular characteristics in this study was key to achieving a better understanding of the phenotypic plasticity of N. emyditoides along its distribution range. We demonstrated that the posterior shape of females exhibits great phenotypic plasticity, which is essential for delimitation of the 10 recognized species associated with freshwater turtles. Therefore, the addition of molecular data should be necessary on the description and delimitation of species of the genus Neoechinorhynchus associated with emydid.
The haplotype network analysis of cox1 sequences inferred with 29 sequences revealed the presence of 13 haplotypes, two of which (H1, H3) were shared between the localities of Catemaco, Veracruz and Monterrey, Nuevo León, and 11 were unique haplotypes (Fig. 5). The two populations from southeastern Mexico (Catemaco and Tlacotalpan, Veracruz) had low F st value (0.1603), suggesting genetic flow between both populations, which can be explained by the fact that both localities belong to the same hydrological basin (González-Ramírez and Parés-Sierra, Reference González-Ramírez and Parés-Sierra2019). In contrast, the two localities from northern Mexico (Monterrey, Nuevo León and Purificación river, Tamaulipas) did not belong to the same freshwater system. However, the value of F st was also low (0.1848), which suggests that definitive or intermediate hosts have the capacity to disperse and maintain the connection between the two localities.
Our phylogenetic analyses inferred with the LSU and ITS datasets confirmed that Neoechinorhynchus is polyphyletic, with most of its members nested in several independent clades that did not share a common ancestor. However, a main clade was formed with the five species of Neoechinorhynchus associated with emydid turtles. This clade contained three species distributed in the eastern USA (N. emydis, N. pseudemydis and N. chrysemydis), one in southeastern Mexico (N. schmidti) and one species (N. emyditoides) distributed from the eastern USA to southeastern Mexico (Fig. 4A and B). All the samples identified as N. emyditoides recovered from the four locations from northern and southeastern Mexico formed a subclade together with other sequences previously identified as N. emyditoides available in GenBank for LSU (MK238158) and ITS (MW520495–497) from Texas and Oklahoma, USA (Fig. 4A and B). Two juvenile worms from red-eared sliders in Monterrey, Mexico (KR086331–332 and MG870860–861 for LSU and ITS, respectively) previously identified as N. emyditoides were nested in a subclade together with other sequences identified as N. pseudemydis. Koch et al. (Reference Koch, Shannon, Detwiler and Bolek2021) performed one of the most extensive studies of morphological, ecological and molecular data of the snail, ostracod and turtle hosts of Neoechinorhynchus species from the eastern USA. Their analysis found that the sequences from northern Mexico originally identified as N. emyditoides (KR086331–332 and MG870860–861) corresponded to N. pseudemydis. The intraspecific genetic divergence estimated on the current study among the isolates of N. emyditoides ranged from 0.3 to 1.6% and from 0 to 0.5% for LSU and ITS, respectively. These values of intraspecific genetic divergence are similar to those previously reported for isolates of N. chrysemydis, N. schmidti and N. emydis, which ranged from 0.3 to 1.6% for ITS and from 0 to 0.01% for LSU (García-Varela et al., Reference García-Varela, García-Prieto and Rodríguez2011; Koch et al., Reference Koch, Shannon, Detwiler and Bolek2021).
Members of the Emydidae family have been divided into two monophyletic lineages recognized as the subfamilies or complexes, Deirochelyinae and Emydinae (Gaffney and Meylan, Reference Gaffney, Meylan and Benton1988; Spinks et al., Reference Spinks, Thomson, McCartney-Melstand and Shaffer2016). Deirochelyinae includes six genera broadly distributed in North America but also contains a few taxa that extend across the Greater Antilles, Mexico, Central America and South America (Parham et al., Reference Parham, Papenfuss, van Dijk, Wilson, Marte, Rodriguez-Schettino and Simison2013, Reference Parham, Papenfuss, Buskirk, Parra-Olea, Chen and Simison2015). Four of the six genera from Deirochelyinae (Trachemys, Graptemys, Pseudemys and Chrysemys), which share a common ancestor (Thomson et al., Reference Thomson, Spinks and Shaffer2021), have been found to harbour Neoechinorhynchus species (Barger and Nickol, Reference Barger and Nickol2004). The fossil record suggests that Deirochelyine originated in North America approximately 20.91 Ma prior to the Miocene (Spinks et al., Reference Spinks, Thomson, McCartney-Melstand and Shaffer2016). Barger (Reference Barger2004) suggested that the eastern USA represents a biodiversity hot spot for Neoechinorhynchus species (and the same for emydids; see Lindeman, Reference Lindeman2013) that infect emydid turtles. The presence of N. emyditoides in northern and southern Mexico could have resulted from ancestral populations of emydid turtles that inhabited the eastern USA and colonized new habitats along the coasts to the south due to the relatively warm and wet temperatures during the mid-Miocene (Spinks et al., Reference Spinks, Thomson, McCartney-Melstand and Shaffer2016), which is mirrored in the diversity of both the hosts and their parasites.
Supplementary material
The supplementary material for this article can be found at https://doi.org/10.1017/S003118202200049X.
Acknowledgements
We thank Luis García Prieto for providing material from the CNHE and Laura Márquez and Nelly López Ortiz from LaNabio for their help during the sequencing of the DNA fragments. We also thank Berenit Mendoza Garfias for her help in obtaining the scanning electron microphotographs. A. L. J. and M. T. G. G. are thankful for the support of the Programa de Posgrado en Ciencias Biológicas, UNAM and CONACYT (A. L. J. CVU. No. 706119; M. T. G. G. CVU No. 956064), for granting a scholarship to complete her Ph.D. and his Master's programme, respectively.
Author contributions
A. L. S. U. and M. G. V. conceived and designed the study. M. G. V., A. L. J. and M. T. G. G. conducted data gathering. C. D. P. P. and R. M. R. performed statistical analyses. A. L. S. U., M. G V., C. D. P. P. and R. M. R. wrote and edited the article.
Financial support
This research was supported by grants from the Programa de Apoyo a Proyectos de Investigación e Innovación Tecnológica (PAPIIT-UNAM) IN201122. Specimens were collected under the Cartilla Nacional de Colector Científico (FAUT 0202) issued by the Secretaría del Medio Ambiente y Recursos Naturales (SEMARNAT), to M. G. V.
Conflict of interest
None.
Ethical standards
The sampling in this work complies with the current laws and animal ethics regulations of México.