Introduction
Given this is the centenary of the Journal of Helminthology, one might have expected great inroads to have been made into the control, and in some cases elimination, of helminth zoonoses. Unfortunately, this is not the case. Tremendous advances have been made over the last 100 years in the tools at our disposal and our understanding of the diversity, taxonomy, host range, distribution, ecology and public health significance of helminth zoonotic agents. However, this is not reflected in a reduction in their global impact and attempts at controlling the causative agents have largely been impeded by anthropogenic factors (Lindahl & Grace, Reference Lindahl and Grace2015). For example, one of the best-known helminth zoonotic agents is Toxocara canis. The ‘tools’ available to control this parasite in dogs, in terms of prophylactic and therapeutic drugs have been available for several decades. However, whereas control has been readily accomplished in some countries, such as Australia, the parasite is an emerging issue elsewhere (see below). It is principally human behaviour in one way or another that allows the causative agents of helminth zoonoses to thrive. Helminths such as T. canis and Echinococcus granulosus can be effectively controlled in domestic dogs, but this requires education and a willingness by dog owners to administer prophylactic drugs to puppies or ensure dogs do not have access to raw uninspected meat, respectively.
Helminth parasites feature prominently in the World Health Organization's list of neglected diseases (8/17). Most are zoonotic with animal reservoirs playing a significant role in the epidemiology of human infections and thus they are a focus of control efforts. Most of the helminth species were described many years ago, but their zoonotic potential became clear much later. We have known about the zoonotic potential of helminths such as Echinococcus and Trichinella (echinococcosis and trichinosis) for over 150 years because of the pioneering experiments of researchers such as von Siebold, Zenker and Kuchenmeister, whereas the aetiology of visceral larva migrans was demonstrated much more recently by Beaver (see Grove, Reference Grove1990). The history of helminth zoonoses is fascinating and detailed descriptions given by authors such as Calvin Schwabe (Reference Schwabe1969) and David Grove (Reference Grove1990) provide a valuable education on the types of investigations that would not be practical or possible today. The first papers on zoonotic helminths were published in the Journal of Helminthology in the 1920s on Hymenolepis, Opisthorchis, Echinococcus, Trichinella and Toxocara.
Education and public health measures, supported by drugs with some species, remain the main strategies in the control of helminth zoonoses. Considerable research has been undertaken on the development of helminth vaccines, including those with zoonotic potential, but vaccines have had no impact on the global burden. The technological advances that have been made in developing molecular-based vaccines have yet to be put into practice. No vaccines are approved for human or animal use (Bain, Reference Bain2019; Perera & Ndao, Reference Perera and Ndao2021). The only successful vaccine against a helminth infection remains the oral vaccine containing live irradiated lungworm larvae (L3) developed by Jarrett and colleagues at the University of Glasgow to protect calves against infection with the bovine lung worm Dictyocauls viviparus (Jarrett et al., Reference Jarrett, Jennings, McIntyre, Mulligan and Urquhart1960; Poynter, Reference Poynter1963). It is a live attenuated vaccine that allows larval development to the fourth stage in an immunogenic site, the mesenteric lymph nodes, following which there is no development to the adult stage. It is extremely effective in preventing infection and disease (parasitic bronchitis or ‘husk’) in cattle, but the other factor that made this vaccine a success was the clear market need given that there were no effective drugs to treat a potentially fatal infection (Poynter, Reference Poynter1963). In contrast, an effective vaccine developed against canine hookworm using a similar approach was not a commercial success given the availability of cheaper, effective therapeutic approaches (Miller, Reference Miller1978). The attenuated vaccine approach taken in the development of the canine hookworm unfortunately would not be transferable to the development of a similar vaccine for human hookworm for reasons of registration. In the 60 years since the bovine lungworm vaccine was developed, molecular approaches have only resulted in potentially effective vaccines against E. granulosus in sheep and some species of Taenia. These vaccines are not yet used in control programmes, for a variety of reasons, principally economic, but trials have been undertaken and continue (e.g. Larrieu et al., Reference Larrieu, Gavida and Lightowlers2019; Nsadha et al., Reference Nsadha, Rutebarika and Ayebazibwe2021).
Nearly 30 years ago, Calvin Schwabe referred to the dynamic state of flux and new discovery that has always characterized studies on zoonoses (Schwabe, Reference Schwabe1984). Helminth zoonoses are always changing in terms of host range, geographical distribution and the emergence of new or unexpected challenges. This is what makes them so interesting but at the same time so demanding.
In this review, the advances that have been made using molecular techniques in the characterization of the causative agents of helminth zoonotic infections are examined. The knowledge gained has provided comprehensive data on the epidemiology of zoonotic helminthiases yet progress in their control has been thwarted by increasingly problematic anthropogenic factors which are discussed.
Impact of molecular tools
The advent of polymerase chain reaction (PCR)-based techniques and sequencing has had a tremendous impact on our understanding of helminth zoonoses and developing strategies for their control.
Taxonomy
A sound taxonomy of helminths with accurate descriptions of individual species and accepted names is the first step in their control. The formal naming of a species is a prerequisite for effective communication which is particularly important when the species in question are of public health importance and require coordinated control efforts. The molecular characterization of numerous helminths has helped in resolving taxonomic issues, identifying new zoonoses and interpreting epidemiological observations.
Molecular approaches have proved useful where the taxonomic significance of morphological characters was questionable, for example in Echinococcus, or difficult to resolve, as with Ancylostoma ceylanicum (see below). The lack of morphological differences between many inter-specific and intra-specific variants has, in the past, compounded an often-confusing taxonomic picture, which in many cases has taken decades to resolve. For example, with Trichinella, there were no morphological characters that could complement increasing epidemiological evidence that Trichinella spiralis is not a uniform species (Zarlenga et al., Reference Zarlenga, Thompson and Pozio2020).
Echinococcus has a long history of taxonomic and nomenclatural confusion, particularly regarding the species level taxonomy (Thompson, Reference Thompson2017). This has resulted not only from a lack of useful morphological characters, but also when taxonomic uncertainty overshadowed the importance of host occurrence (Thompson, Reference Thompson2017). The application of molecular tools has resolved much of the uncertainty of the past and there is now widespread agreement based on not only molecular but also morphological and ecological criteria that Echinococcus should be split into ten species (Thompson, Reference Thompson2020; table 1). Molecular approaches have been pivotal in resolving taxonomic issues and interestingly have largely confirmed original taxonomic considerations, and importantly, from a practical point of view, the reliability of differential morphological characters. As a consequence of establishing a sound taxonomy, the ecology of transmission of Echinococcus in areas where multiple host species are infected has only recently been interpreted with confidence. The life cycles and host range of all ten species are now well documented (Romig et al., Reference Romig, Deplazes, Jenkins, Giraudoux, Massolo, Craig, Wassermann, Takahashi and de la Rue2017; table 1) and represent an essential foundation for control efforts.
Table 1. Species and genotypes of Echinococcus.
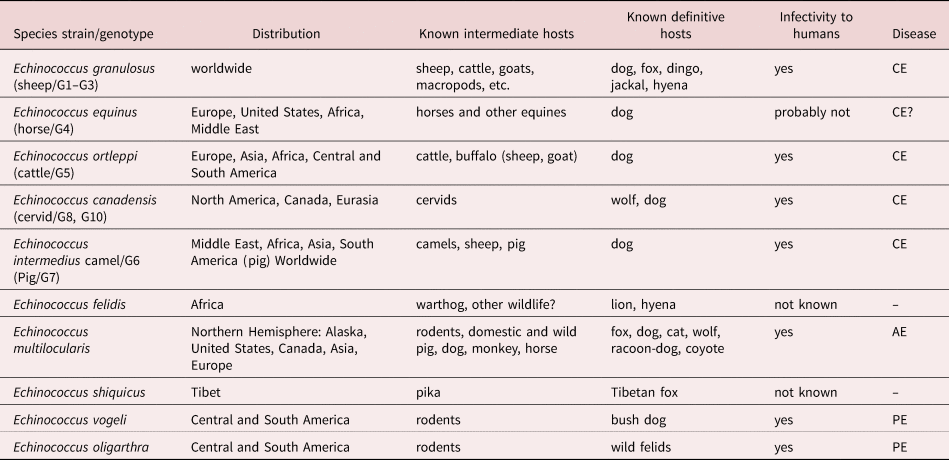
Data updated from Thompson (Reference Thompson2017) and Romig et al. (Reference Romig, Deplazes, Jenkins, Giraudoux, Massolo, Craig, Wassermann, Takahashi and de la Rue2017).
CE, cystic echinococcosis; AE, alveolar echinococcosis; and PE, polycystic echinococcosis.
Of all the helminth zoonoses, Trichinella provides the best example of the value of molecular characterization in determining the genetic distinctness of inter-specific/intra-specific variants in the genus when differential morphological characters to support epidemiological observations are lacking. All developmental stages of currently recognized species and intra-specific variants/strains/genotypes of the genus (table 2) are morphologically indistinguishable. Until the 1950s, T. spiralis was considered a uniform species. The seminal studies by George Nelson and colleagues on Trichinella in the 1960s proved pivotal in establishing our current understanding of the intra-specific diversity of the genus and the epidemiology of trichinosis (trichinellosis) globally. Their studies in Africa were stimulated by observations that demonstrated differences in infectivity between local Kenyan isolates and ‘typical’ isolates of T. spiralis, particularly low infectivity to domestic pigs (Nelson & Mukundi, Reference Nelson and Mukundi1963). Locally acquired infections were also less clinically severe in humans than with ‘typical’ T. spiralis, and subsequent studies demonstrated that the local strain is principally maintained in a wild animal cycle (Nelson et al., Reference Nelson, Guggisberg and Mukundi1963; Capo & Despommier, Reference Capo and Despommier1996). Nelson's studies ‘shattered many of the preconceived ideas about the parasite’ (Nelson et al., Reference Nelson, Mukundi and Blackie1966) and importantly, complemented earlier observations of Rausch and colleagues in the Arctic who found that the local form of Trichinella was adapted to a wildlife cycle and not introduced but endemic to Arctic regions (Rausch et al., Reference Rausch, Babero, Rausch and Schiller1956). The application of molecular tools has been instrumental in defining the taxonomy of both encapsulated and non-encapsulated forms of Trichinella thus providing a firm foundation on which to interpret the wealth of epidemiological data obtained over a period of more than 50 years since the discovery of what we now know as Trichinella nelsoni. A total of ten species (table 2) and three so far unresolved genotypes T6, T8 and T9, have been recognized in the genus Trichinella (Zarlenga et al., Reference Zarlenga, Thompson and Pozio2020).
Table 2. Species and genotypes of Trichinella.
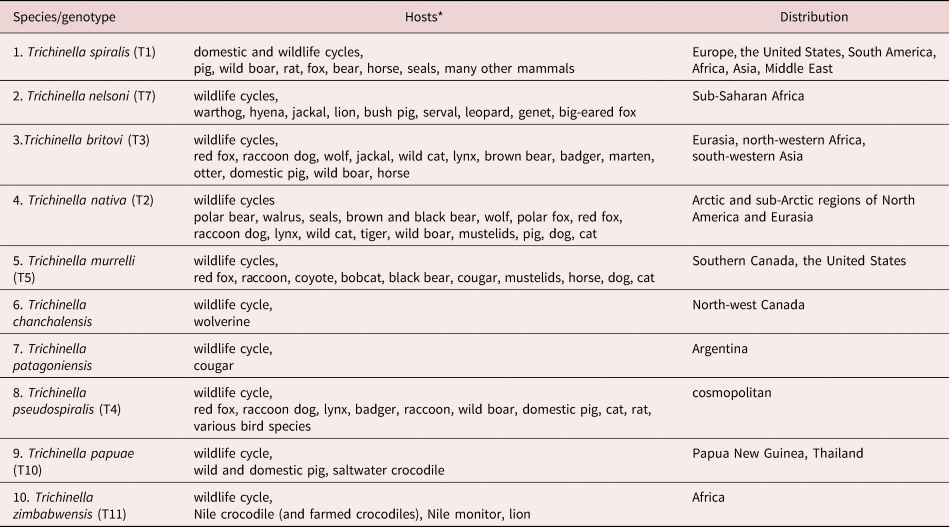
Data from Deplazes et al. (Reference Deplazes, Eckert, Mathis, von Samson-Himmelstjerna and Zahner2016) and Zarlenga et al. (Reference Zarlenga, Thompson and Pozio2020).
*All zoonotic but no human cases reported for T. patagoniensi, T. chanchalensis and T. zimbabwensis.
1–7, encapsulated clade; and 8–10, non-encapsulated clade.
Characterization
The ability to diagnose and characterize a helminth infection based on faecal stages is an important factor both clinically and epidemiologically. However, morphology is often of limited value for the detection and characterization of helminth parasites as well as for the diagnosis of infections. This may be because of insufficient, or poor-quality material; no differential morphological characters between species of the same genus; and the need for staff well trained in microscopy (Singh, Reference Singh1997; Betson et al., Reference Betson, Alonte and Ancog2020), a skill that is sadly very much in decline. Molecular tools have thus provided an important alternative or adjunct to the use of microscopy, providing better sensitivity as well as a means of characterizing helminth parasites genetically.
The eggs of Taenia species are morphologically identical which in the past has been a problem for studies on the epidemiology of taeniid infections where several species may coexist in an endemic area. Such situations require the use of molecular tools to determine the source of infection in humans and any cohabiting non-human definitive hosts. For example, in Laos Taenia solium is an important public health issue (Conlan et al., Reference Conlan, Khamlome, Vongxay, Elliot, Pallant, Sripa, Blacksell, Fenwick and Thompson2012a; Ash et al., Reference Ash, Okello, Khamlome, Inthavong, Allen and Thompson2017). However, in rural areas people may be infected with both, or either, Taenia solium and Taenia saginata and appropriate PCR-based assays are the only way to determine the prevalence of the two species from faecal samples (Conlan et al., Reference Conlan, Vongxay, Khamlome, Dorny, Sripa, Elliot, Blacksell, Fenwick and Thompson2012b). Interestingly, in rural villages in Laos, dogs were found to be infected with Taenia hydatigena using PCR, raising questions about the possible competitive interactions of T. solium and T. hyadatigena in pigs acquiring infections with both species (Conlan et al., Reference Conlan, Vongxay, Fenwick, Blacksell and Thompson2009, Reference Conlan, Vongxay, Khamlome, Dorny, Sripa, Elliot, Blacksell, Fenwick and Thompson2012b).
Similarly, the eggs of the zoonotic trematodes Clonorchis and Opisthorchis are identical which has made it difficult to determine their geographical distribution in endemic areas where both species occur. This is the case in south-east Asia where molecular epidemiological studies have provided a more accurate picture of the distribution of both species (Traub et al., Reference Traub, Macaranas, Mungthin, Leelayoova, Cribb, Murrell and Thompson2009; Saijuntha et al., Reference Saijuntha, Sithithaworn, Kiatsopit, Andrews, Petney, Toledo and Fried2019). Similarly, with the faecal stages of zoonotic nematodes, PCR-based tools have proved valuable in differentiating between species, particularly of Ancylostoma (see below).
Smyth (Reference Smyth1995) identified two nematodes as emerging zoonoses, Angiostrongylus costaricensis and Oesophagostomum bifurcum. Since then, the zoonotic significance of both parasites has increased and molecular tools have proved invaluable in terms of diagnosis, in the case of A. costaricensis (Rojas et al., Reference Rojas, Maldonado-Junior, Mora, Morassutti, Rodriguez, Solano-Barquero, Tijerino, Vargas and Graeff-Teixeira2021) and determining prevalence and genetic diversity of Oesophagostomum (Sirima et al., Reference Sirima, Bizet and Hamou2021).
Surveillance and biosecurity
Otranto & Deplazes (Reference Otranto and Deplazes2019) predicted that the use of DNA-based analyses of clinical material would allow the specific diagnosis of exotic (i.e. unexpected) infections. This was demonstrated in a case of the diphyllobothriid cestode, Adenocephalus pacificus in a child in southern Australia (Moore et al., Reference Moore, Thompson, Jabbar, Williams, Rasiah, Pallant, Koehler, Graham and Weldhagen2016). Infection was traced to a locally caught marine finfish which was unexpected since recent food risk assessments in Australia did not recognize A. pacificus tapeworms as a potential zoonotic threat from locally caught finfish (Moore et al., Reference Moore, Thompson, Jabbar, Williams, Rasiah, Pallant, Koehler, Graham and Weldhagen2016). Morphologically, limited information could be obtained from strobilar material and eggs in the faeces of the infected child, and could only suggest diphyllobothriosis, not the genus or species. However, DNA sequencing confirmed this diagnosis and identified the causative agent as A. pacificus. This is the first human case of diphyllobothriosis reported in Australian waters which is discussed further below.
Opportunities for the introduction of exotic helminth parasites have increased with globalization and the expansion of tourism and illegal wildlife trade (Thompson et al., Reference Thompson, Owen, Puana, Banks, Davis and Reid2003; Thompson, Reference Thompson2018; Betson et al., Reference Betson, Alonte and Ancog2020; Bezerra-Santos et al., Reference Bezerra-Santos, Mendoza-Roldan, Thompson, Dantas-Torres and Otranto2021), emphasizing the need for improved border security and surveillance. From an economic perspective T. spiralis has always been considered a major parasite threat to the biosecurity of many countries in terms of trade (Thompson et al., Reference Thompson, Owen, Puana, Banks, Davis and Reid2003; Thompson, Reference Thompson2018). The importance of demonstrating Trichinella-free status is a significant trade issue for many countries which is exacerbated by the limitations of meat inspection procedures, particularly in free-ranging situations, as well as a lack of appropriate surveillance in wildlife populations (Thompson, Reference Thompson2013). Pig producers need to show negligible risk of their animals becoming infected from wildlife. New molecular and biochemical-based methodologies have improved detection, differentiation and characterization of Trichinella species and genotypes (Zarlenga et al., Reference Zarlenga, Thompson and Pozio2020). Confinement production systems, improvements in biosecurity, surveillance programmes and laws regulating commercial pork production are considered to have ‘essentially eradicated' Trichinella in the United States and Europe (Diaz et al., Reference Diaz, Warren and Oster2020; Shurson et al., Reference Shurson, Urriola and van de Ligt2022). However, this view is not correct because of the numerous wildlife reservoir species in the United States and elsewhere in the world which may impact domestic environments (Pozio, Reference Pozio2013 and e.g. see Reichard et al., Reference Reichard, Sanders, Prentiss, Cotey, Koch, Fairbanks, Interisano, La Rosa and Pozio2021; and see below).
Australia has long been considered to be free of T. spiralis (Thompson, Reference Thompson2018). Australia's domestic pig population is free from Trichinella pseudospiralis and other Trichinella species (Wildlife Health Australia, 2022). Its discovery would be a significant impediment to trade. With the advent of molecular tools for surveillance the question of Australia's presumed Trichinella-free status has been questioned because in the past surveillance, particularly of potential wildlife reservoirs, has relied on traditional detection techniques (Thompson, Reference Thompson2013). Trichinella spiralis has never been reported in Australia. However, until relatively recently, surveys had not been undertaken on the mainland of Australia (Cuttell et al., Reference Cuttell, Cookson, Jackson, Gray and Traub2012a). Recent surveys detected Trichinella papuae in a wild pig from an Australian island in the Torres Strait which has also been described in pigs in New Guinea (Cuttell et al., Reference Cuttell, Cookson, Jackson, Gray and Traub2012a). Trichinella papuae is not only infective to other mammals, including humans, but also to crocodiles (Pozio et al., Reference Pozio, Hoberg, La Rosa and Zarlenga2009). Trichinella pseudospiralis infection has been detected in marsupials and birds in Tasmania (Obendorf et al., Reference Obendorf, Handlinger, Mason, Clarke, Forman, Hooper, Smith and Holdsworth1990) and in a human from Tasmania (Andrews et al., Reference Andrews, Ainsworth and Abernethy1994). Real-time PCR has been developed as a surveillance tool for the detection of Trichinella in muscle samples from wildlife (Cuttell et al., Reference Cuttell, Corley, Gray, Vanderlinde, Jackson and Traub2012b).
Unlike Australia, Trichinella is present in several South American countries apart from Peru, Colombia and Uruguay which have no documented evidence of Trichinella spp. in animals and humans (Ribicich et al., Reference Ribicich, Fariña, Aronowicz, Ercole, Bessi, Winter and Pasqualetti2020). However, these authors point to the lack of surveillance in other countries. In particular, the growth of the guinea pig meat market in the Andean region, and the high prevalence of Trichinella reported in free range pigs and wild boars, as well as other game animal species, are considered important risks. It is therefore considered important to focus on the role of biosecurity and risk management, while improving meat market regulations, and detection of infection prior to consumption. This will reduce the risk of transmission of Trichinella to humans (Ribicich et al., Reference Ribicich, Fariña, Aronowicz, Ercole, Bessi, Winter and Pasqualetti2020).
Trichinosis in humans is typically related to cultural food practices, including the consumption of raw, undercooked, fermented or cured meat and there has been an increasing reliance on testing to demonstrate food safety (Dorny et al., Reference Dorny, Praet, Deckers and Gabriel2009). As outlined above, in terms of control, much of the emphasis had focused on domestic environments and the role of pigs and synanthropic hosts such as the rat in transmission. However, globally, wildlife is now seen as playing the major role in maintaining cycles of transmission (Rostami et al., Reference Rostami, Gamble, Dupouy-Camet, Khazan and Bruschi2017; and e.g. see Sharma et al., Reference Sharma, Harms and Kukka2021; Gherman et al., Reference Gherman, Boros, Baies, Cozman-Petrut and Cozma2022). The consumption of undercooked commercial pork products is no longer considered the main source of human trichinosis, but in most regions, infection occurs following the consumption of raw or undercooked wild game meats, especially bear, moose, deer, walrus and wild/feral boar/hog (Diaz et al., Reference Diaz, Warren and Oster2020). Thus, although biosecurity and ongoing surveillance is successful in domestic livestock settings, wildlife present an ongoing threat and if a true ‘One Health’ approach is taken it must take into account wildlife reservoirs.
Anthropogenic activities may exacerbate opportunities for spillover from wildlife to domestic foci which may threaten biosecurity for pig producers (Thompson, Reference Thompson2013). There are therefore growing demands for producers, not only to demonstrate that their pigs are Trichinella-free but that there is no risk of Trichinella infection spilling over to their pigs from wildlife. The situation has been exacerbated by the insensitivity of diagnostic assays used in meat inspection, and the growing demand for free-range pork (Thompson, Reference Thompson2014). Although routine screening for Trichinella in meat from endemic regions is mainly done using enzyme-linked immunosorbent assay-based techniques, molecular tools are playing a vital role in the screening of wildlife (Barlow et al., Reference Barlow, Roy, Hawkins, Ankarah and Rosenthal2021). It appears that political manoeuvring associated with market access is serving to artificially inflate this as an issue (Thompson, Reference Thompson2014). This may be the case in the United Kingdom where there is a perceived need for data gathering and assessment of Trichinella in United Kingdom wildlife even though it has rarely been detected in the past (Zimmer et al., Reference Zimmer, Fee and Spratt-Davison2009; Food and Environmental Research Agency, 2013).
Epidemiology – understanding transmission
As discussed above, molecular tools have been invaluable in definitively characterizing many helminths genetically and thus settling taxonomic issues. It follows from the resolution of taxonomic issues that a better understanding of the epidemiology of zoonotic helminth infections, for example those caused by Echinococcus and Trichinella, is possible. However, with some species of helminth that have long been recognized as being distinct species, their zoonotic potential and geographical distribution have been uncertain. This has certainly been the case with the hookworm A. ceylanicum. The A. ceylanicum ‘story’ highlights better than no other how molecular tools led to the taxonomic and epidemiological recognition of a zoonotic hookworm, and in recent times has also demonstrated how widespread the parasite is globally (Traub, Reference Traub2013; Thompson, Reference Thompson2015; Colella et al., Reference Colella, Bradbury and Traub2021; Traub et al., Reference Traub, Zendejas-Heredia, Massetti and Colella2021).
Dogs and cats are the definitive hosts of A. ceylanicum but it has long been known to have the ability to establish patent infections in humans. It is the only hookworm with this zoonotic potential and for many years, infections were considered to be uncommon in humans. This was undoubtedly because of difficulties in specific diagnosis since the eggs of hookworm species are morphologically identical (see above). However, with the development of PCR-based techniques that can differentiate between all species of hookworm in humans, dogs and cats, A. ceylanicum has been shown to have a much broader geographical distribution than previously thought (Palmer et al., Reference Palmer, Traub, Robertson, Hobbs, Elliot, While, Rees and Thompson2007; Conlan et al., Reference Conlan, Khamlome, Vongxay, Elliot, Pallant, Sripa, Blacksell, Fenwick and Thompson2012a; Ngui et al., Reference Ngui, Lim, Traub, Mahmoud and Mistam2012; Traub, Reference Traub2013) and until recently was considered the most neglected of all human hookworm (Traub, Reference Traub2013). As a consequence, the development and application of molecular epidemiological tools is now being undertaken in numerous hookworm endemic regions. Surveys in Asia have shown that A. ceylanicum is the second most common species of hookworm infecting humans and in developing areas of south-east Asia and India, there is now a growing awareness of the role of dogs in the zoonotic transmission of A. ceylanicum (Conlan et al., Reference Conlan, Khamlome, Vongxay, Elliot, Pallant, Sripa, Blacksell, Fenwick and Thompson2012a; Inpankaew et al., Reference Inpankaew, Schar and Dalsgaard2014; George et al., Reference George, Kaliappan, Kattula, Roy, Geldhof, Kang, Vercruysse and Levecke2015). In Australia, a recent study of wild dogs (dingoes and dingo hybrids) in peri-urban areas of northern Australia found that up to 100% were infected with A. ceylanicum thus constituting a zoonotic risk to communities in this tropical area of northern Australia (Smout et al., Reference Smout, Thompson and Skerratt2013). Most recently, molecular tools have confirmed the presence of A. ceylanicum in the West Indies and South America (Sears et al., Reference Sears, Cardenas, Kubofcik, Nutman and Cooper2022; Zendejas-Heredia et al., Reference Zendejas-Heredia, Colella, Macphersopn, Sylvester, Gasser, Macpherson and Traub2022). In many areas where hookworm is endemic, dogs are common in villages and communities where a number of hookworm species may coexist in humans and dogs. Ancylostoma ceylanicum is not usually the dominant hookworm in such settings with Ancylostoma duodenale or Necator americanus more prevalent in humans (Conlan et al., Reference Conlan, Khamlome, Vongxay, Elliot, Pallant, Sripa, Blacksell, Fenwick and Thompson2012a). Mixed infections may occur in both humans (e.g. A. duodenale ± N. americanus + A. ceylanicum) and dogs (Ancylostoma caninum + A. ceylanicum). Dogs therefore represent a reservoir of human infections which must be considered when using mass chemotherapy as a means of controlling hookworm infection in the human population. Studies in Laos suggest that the limited success of mass chemotherapy programmes targeted at humans in rural communities may have resulted from a proportion of human infections being due to A. ceylanicum from dogs (Conlan et al., Reference Conlan, Khamlome, Vongxay, Elliot, Pallant, Sripa, Blacksell, Fenwick and Thompson2012a). The clinical management of hookworm disease in humans in endemic regions where more than one species of hookworm circulate will be optimized by specific diagnosis of the causative species given their different pathogenesis (Conlan et al., Reference Conlan, Khamlome, Vongxay, Elliot, Pallant, Sripa, Blacksell, Fenwick and Thompson2012a).
The zoonotic trematodes Clonorchis and Opisthorchis are common throughout south-east Asia and their distributions may overlap (Traub et al., Reference Traub, Macaranas, Mungthin, Leelayoova, Cribb, Murrell and Thompson2009). It is important to differentiate between the two species because of differences in clinical presentation and the prognosis of infection in humans. There is therefore a need for the surveillance of human populations in endemic and potential new endemic regions. However, Clonorchis and Opisthorchis produce morphologically identical eggs, as do the more common, often co-occurring heterophyid flukes. Fortunately, direct characterization of the causative species of fluke from faecal samples is now possible using PCR-based tools. Recent surveys in Vietnam revealed that infection with several minute intestinal flukes, such as Haplorchis pumilio and Haplorchis taichui, are much more common than infection with Clonorchis sinensis or Opisthorchis viverrini, and they often co-infect humans (Doanh & Nawa, Reference Doanh and Nawa2016). Further, previously reported prevalences of heterophyid infection in Vietnam were likely over-estimated due to misidentification of parasites in faecal examinations (Doanh & Nawa, Reference Doanh and Nawa2016). For many years, Opisthorchis was thought to be the only species occurring in Thailand, but PCR-based tools identified the presence of C. sinensis for the first time in 2009 (Traub et al., Reference Traub, Macaranas, Mungthin, Leelayoova, Cribb, Murrell and Thompson2009).
Of the zoonotic schistosome species, S. japonicum has the largest number of animal reservoirs. Over 40 species of domestic and wild mammals have been described as definitive hosts of S. japonicum (Carabin et al., Reference Carabin, McGarvey, Sahlu, Tarafder, Joseph, De Andrade, Balolong and Olveda2015; Budiono et al., Reference Budiono, Satrija, Ridwan, Handharyani and Murtini2019; Gordon et al., Reference Gordon, Kurscheid, Williams, Clements, Li, Zhou, Utzinger, Mcmanus and Gray2019). In south-east Asia, animal reservoirs are believed to play a significant role in the maintenance of the parasite and its transmission to humans, making control very difficult (Betson et al., Reference Betson, Alonte and Ancog2020). Surprisingly, few molecular epidemiological studies have been undertaken to confirm the actual role of different species of wild and domestic definitive hosts. These need to be undertaken at a local level in different countries and regions in south-east Asia. The identity of which non-human definitive hosts contribute to zoonotic transmission of S. japonicum is important so that they can be targeted for control (Betson et al., Reference Betson, Alonte and Ancog2020).
In a number of endemic areas, molecular epidemiological studies have been instrumental in identifying and describing sympatric cycles of transmission involving more than one species of Echinococcus (Thompson, Reference Thompson2020). Although morphology can be used to differentiate between adult worms belonging to different species, this is not practical given the difficulties in recovering adult specimens from definitive hosts and the associated public health risks involved. However, molecular tools allow species identification from eggs in faeces. For example, a study of wild canids on both sides of the Canada–United States border in eastern North America, found wolves and coyotes infected with Echinococcus canadensis (Schurer et al., Reference Schurer, Bouchard, Bryant, Revell, Chavis, Lichtenwalner and Jenkins2018). This species comprises two genotypes, G8 and G10, and it was possible using appropriate PCR-based techniques to discriminate between the two genotypes, finding single and mixed infections in both canid species (Schurer et al., Reference Schurer, Bouchard, Bryant, Revell, Chavis, Lichtenwalner and Jenkins2018). In the Southern Italian Alps, a molecular-based faecal survey of. Echinococcus granulosus in wolves and dogs in the Southern Italian Alps, unexpectedly detected Echinococcus multilocularis eggs in four faecal samples from at least two shepherd dogs, and in five wolf faecal samples (Massolo et al., Reference Massolo, Valli and Wassermann2018). Co-infection of Echinococcus ortleppi and E. multiloculalris was also identified in two wolves. Similarly, co-infections of E. multilocularis and E. canadensis were detected by PCR in coyotes and red foxes in Alberta, Canada (Schurer et al., Reference Schurer, Bouchard, Bryant, Revell, Chavis, Lichtenwalner and Jenkins2018).
In some areas where multiple species of Echinococcus are endemic, it is important to determine the species responsible in clinical infections of humans as this will inform public health measures. Using molecular diagnostic techniques, a recent study in China characterized the species of Echinococcus in patients, with 22 isolates of E. granulosus, and E. multilocularis recovered from one patient and Echinococcus intermedius in another (Cao et al., Reference Cao, Chen, Li, Ma, Xiao, Wang and Gao2018). Another study of 42 patients in south-east Iran, found that 18 were infected with E. granulosus and 24 with the G6 genotype (=Echinococcus intermedius) (Lashkarizadeh et al., Reference Lashkarizadeh, Hooshmand, Nasibi, Mohammadi, Shamsaddini, Kamyabi, Rostami and Harandi2019). In one patient, a mixed infection of E. granulosus in the liver and right lung, and E. intermedius in the left lung, was detected (Lashkarizadeh et al., Reference Lashkarizadeh, Hooshmand, Nasibi, Mohammadi, Shamsaddini, Kamyabi, Rostami and Harandi2019). This study showed a significantly high proportion of patients infected with E. intermedius, particularly in the southern parts of the country (Lashkarizadeh et al., Reference Lashkarizadeh, Hooshmand, Nasibi, Mohammadi, Shamsaddini, Kamyabi, Rostami and Harandi2019). In another recent molecular epidemiological study that examined 140 human cases in China, 108 were caused by E. granulosus, one by E. intermedius, and 31 by E. multilocularis (Shang et al., Reference Shang, Zhang and Yu2019). Misdiagnosis between E. granulosus and E. multilocularis was common in these cases (Shang et al., Reference Shang, Zhang and Yu2019) demonstrating the value of using molecular diagnostic procedures to ensure appropriate therapeutic interventions.
Anthropogenic factors
As Schwabe predicted in 1984, there are many situations yet to be discovered about how humans’ changing activities may influence the transmission of zoonoses (Schwabe, Reference Schwabe1984). How such activities will influence wildlife ecosystems as well as public health remain to be uncovered. Nearly 40 years later, anthropogenic factors are increasingly exacerbating the impact of zoonotic helminths on humans and animals. As discussed above, molecular tools have increased our knowledge about the diversity of helminth zoonotic agents which, in turn, has enabled a much better understanding of how anthropogenic factors, directly or indirectly, for example through climate change, are influencing the occurrence and emergence of helminth zoonoses.
Culture, poverty, poor hygiene and diet
The frequency of transmission of many helminth zoonoses is highest in poor and rural, often disadvantaged communities where cultural and educational issues, contact with contaminated water, poor hygiene and poverty, are major impediments to control. Under such conditions children are especially vulnerable to infection. Control is very difficult when it requires changes to long held cultural and community practices. For example, with schistosomiasis in areas where local populations spend much time close to contaminated water sources for washing, collecting water, agriculture and recreation.
Diet is a major factor with many zoonotic helminths and their transmission to humans, particularly in developing regions. The transmission of fish-borne flukes to humans and to intermediate hosts is influenced by human behaviour (Jones, Reference Jones and Gajadhar2015). The distribution of human infections in south-east Asia corresponds well with the availability of snail and fish intermediate hosts and the food consumption habits of local populations. Principally, eating raw or poorly cooked fish and inadequate sanitation leading to contaminated groundwater (Jones, Reference Jones and Gajadhar2015; Betson et al., Reference Betson, Alonte and Ancog2020). In the Arctic, indigenous communities are exposed to infection with Trichinella as a consequence of subsistence hunting and traditional methods of food preparation, particularly raw or poorly cooked food (Jenkins et al., Reference Jenkins, Castrodale and de Rosemond2013). In poor rural communities, for whom livestock are a crucial source of income and economic security, zoonoses affecting livestock represent a double burden, in terms of their economy and health. There are many pig associated zoonoses in Asia and a lack of epidemiological studies may exacerbate their spread (Okello et al., Reference Okello, Burniston, Conlan, Inthavong, Khamlome, Welburn, Gilbert, Allen and Blacksell2015). For example, in Laos, where Taenia solium is endemic in farmed pigs which are a source of income as well as serving as a food source for rural communities (Conlan et al., Reference Conlan, Khounsy, Inthavong, Fenwick, Blacksell and Thompson2008; Okello et al., Reference Okello, Burniston, Conlan, Inthavong, Khamlome, Welburn, Gilbert, Allen and Blacksell2015), the dual routes of infection require a focus on basic hygiene and diet. In such situations, the vaccination of pigs, once available, is likely to have a beneficial impact on local levels of infection in pigs (Okello et al., Reference Okello, Thomas and Inthavong2016).
Conversely, each year thousands of cases of anisakiasis are reported globally, particularly in developed countries with a significant fishing industry and high fish consumption, particularly of dishes comprising raw fish or squid (Adroher-Auroux & Benítez-Rodríguez, Reference Adroher-Auroux and Benítez-Rodríguez2020; Sugiyama et al., Reference Sugiyama, Shiroyama, Yamamoto, Ishikawa and Morishima2022). Using data from 2018 to 2019 health insurance claims, the average annual incidence of anisakiasis in Japan was estimated to be 19,737 cases (Sugiyama et al., Reference Sugiyama, Shiroyama, Yamamoto, Ishikawa and Morishima2022).
The combination of traditional societal behaviours and subsistence hunting enhance the potential for zoonotic transmission. This is particularly the case in Arctic regions where trichinosis remains a public health challenge (Jenkins et al., Reference Jenkins, Castrodale and de Rosemond2013). Unfortunately, ‘spill-over’ of such behaviour to tourists taking part in recreational hunting ‘holidays’ has resulted in local outbreaks of trichinosis when travellers, often illegally, take the meat from hunted animals back to their home countries (Dupouy-Camet et al., Reference Dupouy-Camet, Yera, Dahane, Bouthry and Kapel2016; Rostami et al., Reference Rostami, Gamble, Dupouy-Camet, Khazan and Bruschi2017).
Urbanization
The importance of increasing urbanization has been neglected until relatively recently. The central driver that attracts wildlife away from their natural habitats into urban areas is food. From an ecological standpoint, this is eloquently discussed by Altizer et al. (Reference Altizer, Becker and Epstein2018) and Becker et al. (Reference Becker, Hall, Forbes, Plowright and Altizer2018) in terms of ‘resource provisioning’, which may be accidental, as when wildlife capitalize on refuse or agricultural by-products, or intentional, through supplemental feeding for management or recreation (in Becker et al., Reference Becker, Hall, Forbes, Plowright and Altizer2018). Environmental encroachment, deforestation and agricultural intensification, also contribute to the movement of wildlife into urban areas (Otranto & Deplazes, Reference Otranto and Deplazes2019; Anon, 2020; Pozio, Reference Pozio2020). All these factors are likely to increase in the future due to human population expansion and increasing demand for resources (White & Razgour, Reference White and Razgour2020).
The most frequent wildlife to have exploited food resources available in urban areas are carnivores, and include the fox, coyote, bears, hyaenas, wolves, raccoons, raccoon dogs, mountain lions, as well as wild boars and stone martins. As a consequence of adapting to urban environments, their ecology has been altered. Such urban invasions are seen throughout Europe, Canada, the United States, Australia and Japan (Otranto & Deplazes, Reference Otranto and Deplazes2019; Pozio, Reference Pozio2020; Waindok et al., Reference Waindok, Raue, Grilo, Siebert and Strube2021). The urbanization of some of these animals, for example raccoons and raccoon dogs, has been exacerbated by translocations (Otranto & Deplazes, Reference Otranto and Deplazes2019). From a zoonotic and public health perspective, the most important parasites infecting such urban wild carnivores are helminths.
Echinococcus multilocularis ‘evolved’ with a successful wild, sylvatic life cycle involving foxes as definitive hosts and microtine, principally arvicolid, rodents as intermediate host. Initially, in natural ecosystems such as the Arctic tundra or Tibetan high-altitude grasslands, and subsequently in highly anthropogenic central European farming landscapes or Japanese city parks (Rausch, Reference Rausch and Thompson1986; Mackenstedt et al., Reference Mackenstedt, Jenkins and Romig2015). It is a cycle now perfectly suited to transmission in an urban environment once its principal hosts successfully adapted to urban environments with the result that spillover to domestic hosts has occurred along with additional wild invaders playing increasingly important roles in transmission, such as the raccoon dog (Nyctereutes procyonoides).
The urbanization of E. multilocularis has now emerged as a significant public health issue, particularly in Europe. Fox densities can build up to be larger in urban than rural areas representing an enhanced contact zone between humans and infected foxes (Mackenstedt et al., Reference Mackenstedt, Jenkins and Romig2015). Echinococcus multilocularis is the cause of alveolar echinococcosis in humans, which is considered one of the most potentially fatal zoonotic diseases in the Northern Hemisphere (Avcioglu et al., Reference Avcioglu, Guven, Balkaya, Kirman, Akyuz, Bia, Gulbeyen and Yaya2021), because of the metastasizing nature of infection with the larval (metacestode) parasite (Thompson, Reference Thompson2017).
In the past, zoonotic transmission of E. multilocularis was principally associated with occupations such as fox trapping (Schantz et al., Reference Schantz, Chai, Craig, Eckert, Jenkins, Macpherson, Thakur, Thompson and Lymbery1995) and other rural activities. However, over the last 40 years it has emerged as a major urban zoonosis as a consequence of various human interventions, most notably vaccinating foxes against rabies which has led to an increase in the numbers of foxes in many European countries, exacerbated by provisioned urban red foxes in Europe (Schweiger et al., Reference Schweiger, Ammann and Candinas2007; Scott et al., Reference Scott, Berg, Tolhurst, Chauvenet, Smith, Neaves, Lochhead and Baker2014; Romig et al., Reference Romig, Deplazes, Jenkins, Giraudoux, Massolo, Craig, Wassermann, Takahashi and de la Rue2017; Altizer et al., Reference Altizer, Becker and Epstein2018). The arvicolid rodent intermediate hosts have also, like the fox, proved to be very successful urban adaptors (Romig et al., Reference Romig, Deplazes, Jenkins, Giraudoux, Massolo, Craig, Wassermann, Takahashi and de la Rue2017). Since the 1980s the autochthonous presence of E. multilocularis has been reported in over 20 European countries that were previously considered non-endemic (Davidson et al., Reference Davidson, Romig, Jenkins, Tryland and Robertson2012; Deplazes et al., Reference Deplazes, Rinaldi and Alvarez Rojas2017; Avcioglu et al., Reference Avcioglu, Guven, Balkaya, Kirman, Akyuz, Bia, Gulbeyen and Yaya2021). In addition, another urban adaptor, the raccoon dog, has successfully invaded Western Europe from the east, and is an excellent definitive host for E. multilocularis, comparable to the fox (Kapel et al., Reference Kapel, Torgerson, Thompson and Deplazes2006; Thompson et al., Reference Thompson, Kapel, Hobbs and Deplazes2006), although its future role in transmission is uncertain given differences in the ecology of foxes and raccoon dogs (Bružinskaitė-Schmidhalter et al., Reference Bružinskaitė-Schmidhalter, Šarkūnas, Malakauskas, Mathis, Torgerson and Deplazes2012; Romig et al., Reference Romig, Deplazes, Jenkins, Giraudoux, Massolo, Craig, Wassermann, Takahashi and de la Rue2017).
The urbanization of the life cycle of E. multilocularis has become a major public health issue not only in Europe but also Japan (Uraguchi et al., Reference Uraguchi, Irie, Kouguchi, Inamori, Sashika, Shimozuru, Tsubota and Yagi2022), and more recently in Canada where both foxes and coyotes are involved in transmission of the parasite (Romig et al., Reference Romig, Deplazes, Jenkins, Giraudoux, Massolo, Craig, Wassermann, Takahashi and de la Rue2017). Anthropogenic landscape changes such as deforestation and overgrazing (White & Razgour, Reference White and Razgour2020) have resulted in an expansion of known intermediate hosts in North America and Tibet (Schantz et al., Reference Schantz, Chai, Craig, Eckert, Jenkins, Macpherson, Thakur, Thompson and Lymbery1995; Giradoux et al., Reference Giradoux, Craig and Delattre2003; Deplazes et al., Reference Deplazes, Gloor, Hegglin and Romig2004; Romig et al., Reference Romig, Thoma and Weible2006, Reference Romig, Deplazes, Jenkins, Giraudoux, Massolo, Craig, Wassermann, Takahashi and de la Rue2017; Wang et al., Reference Wang, Xiao, Vuitton, Schantz, Raoul, Budke, Campos-Ponce, Craig and Giraudoux2007; Davidson et al., Reference Davidson, Romig, Jenkins, Tryland and Robertson2012) and new intermediate hosts, such as the southern red-backed vole (Myodes gapperi) have been identified in urban areas in Canada (Liccioli et al., Reference Liccioli, Duignan, Lejeune, Deunk, Majid and Massolo2012). It has been known for some time that domestic dogs are also capable of acting as suitable definitive hosts of E. multilocularis (Thompson et al., Reference Thompson, Kapel, Hobbs and Deplazes2006). Recently domestic dogs have been found to be an increasing risk as definitive hosts in urban areas in Germany (Schmidberger et al., Reference Schmidberger, Uhlenbruck, Schlingeloff, Maksimov, Conraths, Mayer and Kratzer2022), especially considering the rapidly increasing number of dog owners. Schmidberger et al. (Reference Schmidberger, Uhlenbruck, Schlingeloff, Maksimov, Conraths, Mayer and Kratzer2022) reported that dog ownership in Germany is associated with increased odds of human alveolar echinococcosis. A recent literature review and meta-analysis drew attention to the need to re-assess the role of dogs in the epidemiology of E. multilocularis infections (Toews et al., Reference Toews, Musiani, Checkley, Visscher and Massolo2021).
In other areas of the world such as Canada, the public health significance of E. multilocularis is also an emerging issue in urban areas, and communities with interaction between coyotes, red foxes and most recently wolves, as well as free roaming domestic dogs, with access to abundant populations of intermediate hosts (Davidson et al., Reference Davidson, Romig, Jenkins, Tryland and Robertson2012; Romig et al., Reference Romig, Deplazes, Jenkins, Giraudoux, Massolo, Craig, Wassermann, Takahashi and de la Rue2017). Further, the interaction between wild and domestic cycles raises the possibility of ‘mixing’ of the recently introduced ‘European’ strain of E. multilocularis (Jenkins et al., Reference Jenkins, Peregrine, Hill, Somers, Gesy, Barnes, Gottstein and Polley2012), which may differ in pathogenicity, zoonotic potential and host specificity (Jenkins et al., Reference Jenkins, Castrodale and de Rosemond2013; Deplazes et al., Reference Deplazes, Rinaldi and Alvarez Rojas2017; Romig et al., Reference Romig, Deplazes, Jenkins, Giraudoux, Massolo, Craig, Wassermann, Takahashi and de la Rue2017; Santa et al., Reference Santa, Rezansoff, Chen, Gilleard, Musiani, Ruckstuhl and Massolo2021).
Accidental and deliberate wildlife translocations have also allowed the range expansion of E. multilocularis in different parts of the world. The unfortunate accidental introduction of microtine rodents to Svalbard in the Norwegian Arctic, where foxes were also present, led to the establishment of a successful E. multilocularis cycle (Romig et al., Reference Romig, Deplazes, Jenkins, Giraudoux, Massolo, Craig, Wassermann, Takahashi and de la Rue2017). In contrast, foxes were deliberately translocated within the south-eastern United States to enhance recreational hunting, whereas the movement of foxes from endemic Kuriles to Rebun Island off the north-western coast of Hokkaido, Japan was for rodent control (Davidson et al., Reference Davidson, Appel, Doster, Baker and Brown1992, Reference Davidson, Romig, Jenkins, Tryland and Robertson2012; Schantz et al., Reference Schantz, Chai, Craig, Eckert, Jenkins, Macpherson, Thakur, Thompson and Lymbery1995; Romig et al., Reference Romig, Thoma and Weible2006, Reference Romig, Deplazes, Jenkins, Giraudoux, Massolo, Craig, Wassermann, Takahashi and de la Rue2017).
Unlike the urbanization of E. multilocularis from wildlife foci, in Australia, early settlers introduced E. granulosus with their sheep which subsequently resulted in the establishment of E. granulosus in a wildlife cycle involving dingoes and macropod marsupials (Thompson & Jenkins, Reference Thompson and Jenkins2014). Recently, dingoes (increasingly referred to as wild dogs) infected with E. granulosus have been reported in peri-urban areas of Queensland and New South Wales (Harriott et al., Reference Harriott, Gentle, Traub, Cobbold and Magalhaes2019). Dingoes/wild dogs in urban Australia are associated with the transmission of a number of helminth zoonoses, apart from E. granulosus including A. ceylanicum and Toxocara canis, as they can reach higher population densities in urban areas than in their natural habitats (Smout et al., Reference Smout, Thompson and Skerratt2013, Reference Smout, Skerratt, Butler, Johnson, Congdon and Thompson2017; Mackenstedt et al., Reference Mackenstedt, Jenkins and Romig2015; White & Razgour, Reference White and Razgour2020).
Raccoons are native to North America and are the principal definitive host of Baylisascaris procyonis which was highlighted by Smyth (Reference Smyth1995) as a potential emerging zoonosis. There is no doubt now that Baylisascaris has emerged and is a significant public health issue. As a result of introductions of raccoons from North America, principally for hunting, raccoons are now widely distributed across Europe, Russia and Japan. Like the fox, due to its adaptability, the raccoon has been able to colonize urban areas as a habitat where it readily co-exists with humans often making an engaging pet which can readily transmit Baylisascaris to humans via its faeces. The parasite seems to thrive better in terms of prevalence in association with human-dominated landscapes which put paratenic hosts (rodents and other mammals, birds) under pressure and where domestic dogs can become infected and extend the range of environmental contamination (Page, Reference Page2013).
Apart from the public health significance of Baylisascaris in urban areas, the impact of the parasite on conservation is an emerging issue that should not be overshadowed by the public health issue (Otranto & Deplazes, Reference Otranto and Deplazes2019). The low host specificity for paratenic hosts and the pathogenic potential of the migratory larval parasite is considered to be a potential contributor to the extinction of vulnerable species of wildlife (Page, Reference Page2013). For example, B. procyonis is believed to have contributed to local declines and extinctions of the Allegheny woodrat (Neotoma magister) in the United States (LoGiudice, Reference LoGiudice2003), but other wildlife species are also thought to be at risk and not just in the United States, but also in Europe and Japan (Bauer, Reference Bauer2013; Page, Reference Page2013). The fact that raccoons have caused the introduction and spread of ‘exotic’ parasites, such as B. procyonis in Central European countries, with a potential risk of spill-over to other autochthonous species should be considered as a threat to the conservation of biodiversity (Otranto & Deplazes, Reference Otranto and Deplazes2019).
Climate change
It is difficult to predict how a changing climate will affect the structure and functioning of terrestrial, freshwater and marine parasite ecosystems (Jenkins et al., Reference Jenkins, Castrodale and de Rosemond2013), as well as the biology of definitive and intermediate hosts, given the diversity of parasite life cycles (Froeschke et al., Reference Froeschke, Harf, Sommer and Matthee2010). In this context, of most significance perhaps are saprozoonoses such as the taeniids, ascarids and hookworm, which all produce resistant stages which will be directly affected by climate change, increasing or decreasing their survival in the environment. Some require sojourn in the environment to embryonate and they are all capable of prolonged survival under suitable conditions of temperature and humidity. For example, climate change is predicted to favour the survival of Toxocara eggs in the soil (Blum & Hotez, Reference Blum and Hotez2018). Trichinella is unusual among the helminth zoonoses in having one species, T. britovi, that is specifically adapted to cooler climatic zones. Trichinella britovi larvae survive longer in carcasses beneath than in those above the snow (Pozio, Reference Pozio2022). During the past 60 years, there has been a significant reduction in T. britovi prevalence in red foxes from Alpine regions (Pozio, Reference Pozio2022) of between 20 and 30% (Marazza, Reference Marazza1960; Rossi et al., Reference Rossi, Interisano, Deksne and Pozio2019) which correlates with a significant decrease in snow depth and snow cover in the Alps (Scherrer et al., Reference Scherrer, Appenzeller and Laternser2004; Marty, Reference Marty, Rixen and Rolando2013).
Globally, a warming climate and the associated ecological disturbances are modifying the structure of helminth ecosystems (Jenkins et al., Reference Jenkins, Castrodale and de Rosemond2013). For example, with the diphyllobothriid cestodes (Kuchta et al., Reference Kuchta, Serrano-Martínez and Scholz2015), these changes will influence patterns of distribution, seasonal timing of migration and reproduction of their definitive hosts, as well as the development and survival of fish hosts (second intermediate, and paratenic, hosts), as well as invertebrate intermediate hosts and free-living parasite stages (eggs and coracidia). Recently, the first human case of a patent diphyllobothriid infection with A. pacificus was reported in humans in South Australia (see above). Infection was acquired by the ingestion of raw locally caught marine fish (Moore et al., Reference Moore, Thompson, Jabbar, Williams, Rasiah, Pallant, Koehler, Graham and Weldhagen2016). It will be interesting to see if plerocercoids are recovered from more marine fish off the coast of South Australia in the future and determine the possible influence of climate change on this apparent change in distribution.
These effects will be especially felt in the Arctic where a recent study concluded that it has warmed nearly four times faster than the rest of the world since 1979 (Rantanen et al., Reference Rantanen, Karpechko, Lipponen, Nordling, Hyvarinen, Rousteenoja, Vihma and Laaksonen2022). Since the current distribution of E. multilocularis is restricted to the Northern Hemisphere, it is likely to be highly susceptible to the effects of climate change (Jenkins et al., Reference Jenkins, Castrodale and de Rosemond2013). Already, this parasite is emerging (increasing in distribution and prevalence) in wildlife and human hosts in the Arctic and is colonizing new regions through anthropogenic and natural movements of wild and domestic hosts (in Jenkins et al., Reference Jenkins, Castrodale and de Rosemond2013).
As discussed above, the raccoon dog is rapidly expanding in Europe and is likely to exacerbate the already worsening problem caused by the spread of the fox as a definitive host of E. multilocularis. Although the raccoon dog occupies a similar ecological niche to that of foxes, particularly in terms of diet and urbanization, competition has not been observed (Kauhala & Kowalczyk, Reference Kauhala and Kowalczyk2011). However, the raccoon dog has a greater reproductive potential than the fox and this may increase with the effects of global warming (Mustonen & Nieminen, Reference Mustonen and Nieminen2018). This is because climate is likely to have a marked effect on the productivity of the raccoon dog, especially on the proportion of reproducing females and annual birth rate (Kauhala & Helle, Reference Kauhala and Helle1995).
Anisakiasis is an emerging zoonosis where the causative agents have complex multi-host aquatic life cycles. Like the diphylobothriids, all stages of the life cycles will be very sensitive to environmental factors including temperature. Climate change will adversely affect the complex trophic webs that form part of the complex life cycles of anisakid nematodes involving different species of marine mammals, fish and invertebrates which act as definitive and intermediate hosts (Mattiucci & Nascetti, Reference Mattiucci and Nascetti2007). For example, in south-east Asia anisakiasis is an emerging zoonosis and new fish hosts have been identified (Betson et al., Reference Betson, Alonte and Ancog2020). The prevalence of anisakid infection in two species of Croaker fish (Johnius carouna and Dendrophysa ruselli) were found to be 31.7% and 87.5%, respectively (Nuchjangreed et al., Reference Nuchjangreed, Hamzah, Suntornthiticharoen and Sorosjinda-Nunthawarasilp2006), highlighting the potential reservoirs present that could favour zoonotic emergence. Mature adult anisakids have been found in marine animals in Indonesia, the Philippines and Thailand (Wiwanitkit & Wiwanitkit, Reference Wiwanitkit and Wiwanitkit2016). Although cases in humans are not well reported in the literature, examples of anisakiasis have been recorded in Thailand and Malaysia (Hemsrichart, Reference Hemsrichart1993; Amir et al., Reference Amir, Ngui, Ismail, Wong, Ong, Lim, Lau and Mahmud2016).
Wildlife
Some wildlife cycles are a major impediment to the eventual control of certain helminth zoonoses, and in the case of T. spiralis, they can be of economic significance with respect to trade (see above). With T. spiralis, much of the emphasis has been on the control of the parasite in domestic environments, principally involving pigs and synanthropic hosts such as the rat. However, the maintenance of Trichinella spp. in a variety of wildlife transmission cycles and the involvement of humans in these cycles is central to the zoonotic transmission of the parasite (Pozio et al., Reference Pozio, Hoberg, La Rosa and Zarlenga2009).
Globally, most Trichinella infections occur in wildlife (Pozio, Reference Pozio2013). Infections in wildlife have been documented in 66 (33%) countries worldwide compared with 43 (21.9%) countries for domestic animals (Pozio, Reference Pozio2007). Therefore, the spillover from wildlife to domestic foci represents a constant challenge for control in both endemic regions and from a biosecurity viewpoint for those countries which are considered to be Trichinella-free (discussed in detail above).
Wildlife is essential for maintaining the life cycle of some species of Echinococcus in nature. For E. multilocularis, foxes and microtine rodents serve this role whereas for E. canadensis it is wolves and cervids, Echinococcus oligarthra wild felids and large rodents, and for Echinococcus felidis lions and warthogs (Thompson, Reference Thompson2008; Romig et al., Reference Romig, Deplazes, Jenkins, Giraudoux, Massolo, Craig, Wassermann, Takahashi and de la Rue2017). Throughout most of its geographical range, E. granulosus exists almost invariably under conditions modified by humans (Rausch, Reference Rausch and Thompson1986) perpetuated by domestic animals with infection of humans principally a result of domestic dogs having access to infected livestock. Unfortunately, anthropogenic activities allowing spillover from the domestic sheep/dog cycle, led to the establishment on the Australian mainland of a cycle involving native wildlife, macropod marsupials and dingoes, that not only impacts on public health and livestock industries but also on wildlife health (Jenkins et al., Reference Jenkins, Romig and Thompson2005; Thompson et al., Reference Thompson, Kutz and Smith2009). This artificial, man-made cycle also represents a barrier to the control or eradication of E. granulosus in sheep on the Australian mainland due to infected dingoes contaminating sheep pasture (Jenkins et al., Reference Jenkins, Romig and Thompson2005). There is no evidence of an indigenous species of Echinococcus in Australia or that E. granulosus was present before the arrival of European settlers, with whom E. granulosus was introduced in sheep (Thompson et al., Reference Thompson, Lymbery and Smith2010).
From a conservation perspective, indigenous macropodid marsupials in Australia, particularly small species of wallabies, are highly susceptible to infection with the cystic stage of E. granulosus which may seriously impair respiratory function enhancing their predation (Barnes et al., Reference Barnes, Morton and Coleman2007). As discussed above, the urban encroachment of infected dingoes is also an emerging public health issue.
Echinococcus felidis is confined to Africa and may represent an indigenous form perpetuated in wildlife cycles involving the lion as the principal definitive host and the warthog as an intermediate host. With the introduction and domestication of livestock in Africa, E. granulosus has become widespread in domestic livestock including sheep, cattle and goats, with E. intermedius also occurring in camels (Hüttner et al., Reference Hüttner, Siefert, Mackenstedt and Romig2009; Addy et al., Reference Addy, Alakonya and Wamae2012). Over 18 species of wild herbivores from different parts of southern, central and eastern Africa have been found to be infected with hydatid cysts (Macpherson, Reference Macpherson1983; Romig et al., Reference Romig, Deplazes, Jenkins, Giraudoux, Massolo, Craig, Wassermann, Takahashi and de la Rue2017). These include the most common prey species of the lion although the role of other intermediate hosts is as yet unknown (Schantz et al., Reference Schantz, Chai, Craig, Eckert, Jenkins, Macpherson, Thakur, Thompson and Lymbery1995; Huttner & Romig, Reference Hüttner and Romig2009; Hüttner et al., Reference Hüttner, Siefert, Mackenstedt and Romig2009; Romig et al., Reference Romig, Deplazes, Jenkins, Giraudoux, Massolo, Craig, Wassermann, Takahashi and de la Rue2017). The existence of an independent cycle in wild mammals in Africa has been proposed (Macpherson et al., Reference Macpherson, Karstad, Stevenson and Arundel1983; Rausch, Reference Rausch and Thompson1986), and there was speculation that E. felidis was responsible for infections in domestic livestock and other wildlife intermediate hosts of Echinococcus in Africa. However, without molecular characterization of isolates from intermediate hosts it was not possible until recently, to determine the extent of transmission cycles of E. felidis in African wildlife. Molecular epidemiological studies support an indigenous wildlife cycle in Africa involving the lion and spotted hyaena as definitive hosts, with the warthog and possibly other wild Suidae as intermediate hosts (Romig et al., Reference Romig, Deplazes, Jenkins, Giraudoux, Massolo, Craig, Wassermann, Takahashi and de la Rue2017).
Echinococcus canadensis (formerly genotypes G8 and G10) is also maintained in a wild animal cycle involving wolves and large cervids (moose and caribou), originally referred to as the ‘northern form’ of E. granulosus (Rausch, Reference Rausch2003), and principally occurring in northern Canada, Alaska, parts of Scandinavia, Eastern Europe and the United States (Himsworth et al., Reference Himsworth, Jenkins and Hill2010; Thompson, Reference Thompson2015; Romig et al., Reference Romig, Deplazes, Jenkins, Giraudoux, Massolo, Craig, Wassermann, Takahashi and de la Rue2017; Dell et al., Reference Dell, Newman, Purple, Miller, Ramsay, Donnell and Gerhold2020). Domestic or free-roaming dogs have long been recognized as important ‘bridging hosts' between the wild, sylvatic cycle of E. canadensis and people (Rausch, Reference Rausch2003; Himsworth et al., Reference Himsworth, Jenkins and Hill2010). In northern Canada, interaction between the wildlife cycle and indigenous communities occurs due to subsistence hunting within indigenous communities where domestic dogs have access to offal and carcasses leading to infection with E. canadensis (Lichtenwalner et al., Reference Lichtenwalner, Adhikari, Kantar, Jenkins and Schurer2014; Oksanen & Lavikainen, Reference Oksanen and Lavikainen2015; Schurer et al., Reference Schurer, Bouchard, Bryant, Revell, Chavis, Lichtenwalner and Jenkins2018). A domestic cycle of transmission involving domestic dogs and farmed elk was also identified in western Canada (Thompson, Reference Thompson2013). Recent reports of E. canadensis in coyotes in Canada suggest the emergence of this species in new endemic regions (Santa et al., Reference Santa, Pastran, Klein, Duignan, Ruckstuhl, Romig and Massolo2018; Priest et al., Reference Priest, McRuer, Stewart, Boudreau, Power, Conboy, Jenkins, Kolapo and Shutler2021) and given that, the urbanization of the coyote in Canada is of potential public health significance.
Wildlife also plays an increasingly important role in the epidemiology of schistosome infections. For example, with S. japonicum, wild animals play a very significant role as reservoirs of transmission to humans, making S. japonicum difficult to control. Over 40 species of domestic and wild mammals have been described as definitive hosts (Gordon et al., Reference Gordon, Kurscheid, Williams, Clements, Li, Zhou, Utzinger, Mcmanus and Gray2019). Riley et al. (Reference Riley, Carabin and Belisle2008) explored the rate of transmission of S. japonicum from different mammals using mathematical modelling. The model inferred that rats are key hosts in the infection cycle, while water buffaloes are found to be a less important reservoir host in the Philippines situation than the bovines in China (Riley et al., Reference Riley, Carabin and Belisle2008). However, most emphasis over the last ten years has focused on the role of bovines in zoonotic transmission. A recent study found that rodents are likely to become the main reservoir of ongoing transmission of S. japonicum and a severe risk to not attaining elimination goals in China (Zou et al., Reference Zou, Yu, Qiu, Webster and Lu2020). In West Africa, Catalano et al. (Reference Catalano, Sène, Diouf, Fall, Borlase, Léger, Bâ and Webster2018) reported that rodents may be an important local reservoir for zoonotic schistosomiasis, amplifying transmission to humans and acting as natural definitive hosts of schistosome hybrids.
Emerging issues
Aquaculture
Whenever animals are farmed, increased density will enhance the transmission of parasites. As with livestock industries, this applies to aquaculture for which the impact of parasite infections has long been recognized. In particular, fish-borne zoonotic trematodes are an emerging and rapidly growing concern in developing countries due to associated economic losses and public health concerns (Labony et al., Reference Labony, Abdul Alim, Muhammad Mehedi Hasan, Hossain, Islam, Alam, Tsuji and Anisuzzaman2020). The diseases of most concern include those caused by opisthorchid, heterophyid and echinostomid flukes (Betson et al., Reference Betson, Alonte and Ancog2020; Caffara et al., Reference Caffara, Gustinelli, Mazzone and Fioravanti2020). The situation is not improving as the global demand for farmed fish continues to rise and developing countries seize opportunities to develop their aquaculture capabilities to serve export markets. For example, Vietnam is a densely populated developing country and aquaculture forms part of their economic development strategy, with fisheries accounting for 3.7% of its gross domestic product (Nguyen et al., Reference Nguyen, Dorny, Nguyen and Dermauw2021). It has become one of the largest aquaculture producers worldwide, accounting for 4.5% of the global production, and the third largest world fish exporter after China and Norway (United Nations Food and Agriculture Organization, 2018; Nguyen et al., Reference Nguyen, Dorny, Nguyen and Dermauw2021). As such, the ability to rapidly detect zoonotic trematodes in farmed fish is very important but has been difficult as the infective metacercariae are hard to detect visually in fish (Caffara et al., Reference Caffara, Gustinelli, Mazzone and Fioravanti2020). An important advance has been the development of a rapid and cost-effective multiplex PCR for the simultaneous detection of opisthorchid and heterophyid metacercariae in fish or fish products (Caffara et al., Reference Caffara, Gustinelli, Mazzone and Fioravanti2020).
Dracunculus (Guinea worm)
Dracunculus has been known since early recorded history, undoubtedly because of the female's spectacular behaviour when releasing L1 larvae from infected humans into fresh water which are infective to Cyclops crustaceans. Intensive global eradication efforts spanning several decades which focused on education and clean drinking water raised optimism that this zoonotic helminth would be the first to be eradicated (Callaway, Reference Callaway2016). However, in Africa, dogs are playing an increasingly important role as reservoirs of infection contaminating water sources and thus enhancing transmission to humans. In addition to increasing reports of the role of dogs in the epidemiology of dracunculiasis in humans, has been the recognition of novel paratenic hosts such as fish and amphibians. This has raised awareness of the potential for Dracunculus medinensis to emerge in new geographical areas (Molyneux & Sankara, Reference Molyneux and Sankara2017; Hopkins et al., Reference Hopkins, Weiss, Roy, Yerian and Sapp2020).
Most of the focus on controlling Guinea worm has, understandably, been on D. medinensis. However, the application of molecular tools has also revealed more definitive data on the diversity of species in the genus Dracunculus and the limitations of past knowledge based on morphology. These emerging concerns recently culminated in the report of a human patient in Vietnam infected with Dracunculus (Thach et al., Reference Thach, van Doorn, Bishop, Fox, Sapp, Cama and Van Duyet2021). Molecular characterization demonstrated that infection was not with D. medinensis, but with a previously undescribed species, in a different clade phylogenetically to D. medinensis. It clustered with the animal infective Dracunculus insignis and Dracunculus lutrae, (Thach et al., Reference Thach, van Doorn, Bishop, Fox, Sapp, Cama and Van Duyet2021) and thus is potentially of zoonotic significance.
Toxocara canis
There is increasing concern that T. canis and its impact on human health have been neglected. For many years, anthelmintics have been available to prevent T. canis infection in domestic dogs and the veterinary profession has done much to educate dog owners about the public health significance of T. canis and how it can be readily controlled. This has had dramatic effects in countries such as Australia where the prevalence in domestic dogs has fallen to less than 1% (Palmer et al., Reference Palmer, Thompson, Traub, Rees and Robertson2008; Massetti et al., Reference Massetti, Wiethoelter, McDonagh, Rae, Marwedel, Beugnet, Colella and Traub2022). Surprisingly, this has not been the case in other Western countries (Deplazes et al., Reference Deplazes, van Knapen, Schweiger and Overgaauw2011; Mathison & Pritt, Reference Mathison and Pritt2018) where the parasite is still persisting in large endemic areas despite the availability, as in Australia, of effective anthelmintics (Deplazes et al., Reference Deplazes, van Knapen, Schweiger and Overgaauw2011), perhaps due to human complacency or variable levels of dog owner education.
Hotez (Reference Hotez2020) considers that insufficient attention is given to the potential emergence of toxocariasis in developing countries where increasing urbanization in tropical and sub-tropical areas coupled with large numbers of stray dogs and cats will lead to increasing levels of environmental contamination with Toxocara eggs (Hotez, Reference Hotez2017). In addition, increasing urbanization of the red fox in many parts of the developed world with a reproductive cycle giving rise to large numbers of cubs with high T. canis worm burdens, will contribute significantly to environmental contamination with eggs (Otranto & Deplazes, Reference Otranto and Deplazes2019). In addition, climate change in some areas such as the Arctic may extend the range of Toxocara through increasing numbers of wildlife hosts such as the red fox, leading to a spillover into local wildlife such as wolves and Arctic foxes (Jenkins et al., Reference Jenkins, Castrodale and de Rosemond2013).
Thelazia callipaeda
Thelazia callipaeda is a zoonotic filarial nematode transmitted by lachryphagous drosophilids that was first described in a dog from India in 1910 (Railliet & Henry, Reference Railliet and Henry1910). Seven years later the first case of infection in the eye of a human in China was reported (Stuckey, Reference Stuckey1917). Subsequently, occasional human cases have been reported throughout south-east Asia and India (Sillman, Reference Sillman1953; Otranto & Deplazes, Reference Otranto and Deplazes2019). However, since the late 1980s when it was first reported in dogs in Italy, T. callipaeda has rapidly expanded its distribution throughout Europe with infections in domestic and wild animal species as well as increasing numbers of cases reported in humans (Otranto & Deplazes, Reference Otranto and Deplazes2019). Not surprisingly, canine thelaziosis is considered an emerging vector borne helminth with significant zoonotic potential. The reason why it has emerged, and continues to do so, in Europe is not clear but may be associated with the increasing importance of wildlife, such as the fox, but also animals such as bears and wolves (Papadopoulos et al., Reference Papadopoulos, Komnenou, Karamanlidis, Bezerra-Santos and Otranto2021) and mustelids (Ionică et al., Reference Ionică, Deak and D'Amico2019) in maintaining infection in their natural habitats which increasingly encroach into domestic environments. Another zoonotic filarial nematode, Brugia malayi, has re-emerged in Sri Lanka after a quiescent period of four decades with dogs and cats the potential reservoirs for human infections (Mallawarachchi et al., Reference Mallawarachchi, Chandrasena, Wickramasinghe, Premaratna, Gunawardane, Mallawarachchi and de Silva2018). Overall microfilaraemia rates of over 80% in dogs and 75% in cats were found with higher levels in rural than urban areas.
Haycocknema perplexum
The nematode, H. perplexum, cannot be considered an emerging zoonotic helminth but remains a curiosity in terms of its zoonotic potential. Haycocknema perplexum is a minute muspiceoid nematode with related species in native wildlife including koalas, bats and kangaroos (Spratt, Reference Spratt2005; Ward et al., Reference Ward, Krishnan, Iyengar, Robertson, White and Urkude2022). However, the natural host of H. perplexum remains unknown. It has been identified in 12 human patients, who have contracted infection in Australia (Northern Australia and Tasmania) since 1998 (Dennett et al., Reference Dennett, Siejka, Andrews, Beveridge and Spratt1998; Spratt, Reference Spratt2005; Basuroy et al., Reference Basuroy, Pennisi, Robertson, Norton, Stokes, Reimers and Archer2008; McKelvie et al., Reference McKelvie, Reardon, Bond, Spratt, Gangell, Zochling and Daffy2013; Vos et al., Reference Vos, Robertson and Binotto2016; Koehler et al., Reference Koehler, Leung, McEwan and Gasser2018; Pritt et al., Reference Pritt, Mathison and Bradbury2022; Ward et al., Reference Ward, Krishnan, Iyengar, Robertson, White and Urkude2022). Clinical features common to infection are limb wasting and weakness associated with myositis, dysphagia and persistent eosinophilia, suggesting a long period of subclinical infection (Basuroy et al., Reference Basuroy, Pennisi, Robertson, Norton, Stokes, Reimers and Archer2008; Ward et al., Reference Ward, Krishnan, Iyengar, Robertson, White and Urkude2022). Diagnosis based on symptoms alone is difficult given that they are similar to those of muscular dystrophy and inflammatory myopathy (Pritt et al., Reference Pritt, Mathison and Bradbury2022). The source of infection with H. perplexum is not known and its zoonotic potential remains to be proven, although some of the cases were associated with the consumption of bush meat (Spratt, Reference Spratt2005; Ward et al., Reference Ward, Krishnan, Iyengar, Robertson, White and Urkude2022).
Identifying zoonotic potential
Recently, Majewska et al. (Reference Majewska, Huang, Han and Drake2021) developed a predictive model from a global dataset to identify factors contributing to spillover of helminths from wildlife to domestic animals and humans. By doing this with well recognized zoonotic helminths, their model identified several non-zoonotic helminths with zoonotic potential. Among these were Paramphistomun cervi, followed by Schistocephalus solidus and Strongyloides papillosus. Predisposing factors for zoonotic potential included the nature of the intermediate hosts, involvement of wildlife and livestock in the life cycles, free-living environmental stage in the case of Strongyloides and likely scenarios of transmission to humans. These authors concluded that their results highlight the importance of the often-changing interface between wildlife, companion animals and humans in determining the risk of zoonotic helminth infections (Majewska et al., Reference Majewska, Huang, Han and Drake2021).
One Health
Finally, I would like to mention ‘One Health’. It seems mandatory nowadays to advocate taking a One Health approach in the context of controlling zoonotic infections, including helminths. Unfortunately, the term is increasingly mentioned in papers, often in just the title or abstract, without qualification or explanation for its relevance to the published study. It is therefore losing its significance in ecological and epidemiological studies of infectious diseases. This is a shame as there are important elements, particularly with respect to parasite zoonoses (Thompson, Reference Thompson2013), that form part of ‘One Health’ as originally proposed (Gibbs, Reference Gibbs2014). In terms of zoonotic helminths, effective control must take a One Health approach, and this has been clearly demonstrated in the successful control programmes of Echinococcus infections in different parts of the world. Although implemented before the term One Health was adopted, their control by necessity included collaborative strategies encompassing environmental, human and animal health as envisioned by the ‘father’ of One Health Calvin Schwabe (Schwabe, Reference Schwabe1969; Zinsstag et al., Reference Zinsstag, Schelling, Waltner-Toews and Tanner2011).
Concluding comments
We have the ‘tools’ that have enabled the characterization of the causative agents of helminth zoonotic infections and significant inroads have been made into understanding their ecology and epidemiology. Molecular tools and improved surveillance continue to provide information on emerging zoonoses and to identify ‘new’ zoonoses. Importantly, we now better understand transmission patterns more than ever before and know from past experience that breaking the cycles of transmission is the key to control. So, theoretically, we are in the best position possible to control helminth zoonoses. However, this is not the case. For example, T. canis remains a problem in developed countries and is an emerging issue in developing countries, even though effective cheap, prophylactic drugs have been available for decades. Unfortunately, we cannot rely on vaccines to control helminth zoonoses but even if they were available, would they really have an impact? They would still have to be administered, which, in regions where they would be most effective would require financial support. This would need to come from governments and aid agencies, given the likely lack of commercial interest. We need to act now, not procrastinate, with well utilized, successful approaches that require education and government support. If not, the situation will only worsen due to anthropogenic factors such as urbanization and climate change which are exacerbating the problem of helminth zoonoses.