Introduction
Increasing threats of climate variability and change and accelerated soil fertility decline have raised the need for climate-smart cropping systems that adapt to the vagaries of climate change, mitigate its effects, and increase productivity and profitability of agriculture (Lipper et al., Reference Lipper, Thornton, Campbell, Baedeker, Braimoh, Bwalya, Caron, Cattaneo, Garrity and Henry2014). Conservation Agriculture (CA), a cropping system defined by its core principles of minimum soil disturbance, crop residue retention, and crop diversification (Kassam et al., Reference Kassam, Friedrich, Shaxson and Pretty2009), among other complementary good agriculture practices (Thierfelder et al., Reference Thierfelder, Baudron, Setimela, Nyagumbo, Mupangwa, Mhlanga, Lee and Gérard2018), has been promoted in the last three decades to smallholders to provide such benefits in southern Africa (Thierfelder et al., Reference Thierfelder, Chivenge, Mupangwa, Rosenstock, Lamanna and Eyre2017).
Smallholder farm sizes in southern Africa range between 0.5 ha and 3 ha with an average farm size of 1.5 ha per farm household with a few exceeding 3 ha (Giller et al., Reference Giller, Delaune, Silva, van Wijk, Hammond, Descheemaeker, van de Ven, Schut, Taulya, Chikowo and Jens2021). Farming systems in southern Africa are predominantly characterized by mixed crop-livestock systems with cereals such as maize (Zea mays L.) and traditional grain crops e.g. sorghum (Sorghum bicolor L. Moench) and millet (Pennisetum glaucum L.) being grown either as monocrops or integrated with legumes such as cowpea (Vigna unguiculata (L.) Walp.) and groundnut (Arachis hypogaea L.) (Rufino et al., Reference Rufino, Dury, Tittonell, van Wijk, Herrero, Zingore, Mapfumo and Giller2011). The cropping systems in this region are practiced under a unimodal rainfall pattern and usually begin after the effective rains in November and last for approximately six–seven months (Herrmann and Mohr, Reference Herrmann and Mohr2011). Farmers in the region often rely on manual family labor for most field operations such as sowing and weeding but the use of animal draught power is a relatively common alternative especially in Zambia and Zimbabwe (Moyo, Reference Moyo2016). In some cases, labor is hired locally when farmers can afford to pay either in cash or in kind using grain as payment. Inputs such as mineral fertilizers and herbicides are purchased from local agro-dealers or obtained from the government through subsidy programs (Hemming et al., Reference Hemming, Chirwa, Dorward, Ruffhead, Hill, Osborn, Langer, Harman, Asaoka, Coffey and Phillips2018). Farm produce is mostly sold on local markets based on pan-seasonal and pan-territorial floor prices but can also be sold to grain boards managed by governments (Jayne, Reference Jayne2012).
Previous research in the region in the last decades has provided the proof of concept that cropping systems based on the principles of CA can reduce the exposure of crops to drought and heat stress (Komarek, Thierfelder and Steward, Reference Komarek, Thierfelder and Steward2021; Steward et al., Reference Steward, Dougill, Thierfelder, Pittelkow, Stringer, Kudzala and Shackelford2018). Some of the main drivers for increased resilience are increased water infiltration and available soil moisture (Mhlanga and Thierfelder, Reference Mhlanga and Thierfelder2021) in response to reduced soil disturbance and surface protection with mulch (Thierfelder and Wall, Reference Thierfelder and Wall2009), which also minimizes evapotranspiration and moderates soil temperature. By applying adequate nutrients in the form of manure, compost and/or mineral fertilizer, CA systems can gradually improve soil fertility leading to increased crop productivity. However, this may take 2–5 cropping seasons to become significant, depending on the context (Thierfelder, Matemba-Mutasa and Rusinamhodzi, Reference Thierfelder, Matemba-Mutasa and Rusinamhodzi2015a; Thierfelder et al., Reference Thierfelder, Rusinamhodzi, Ngwira, Mupangwa, Nyagumbo, Kassie and Cairns2015b). Soil carbon (C) under CA may gradually increase if adequate amounts and types of residues are retained on the soil surface (Ligowe et al., Reference Ligowe, Nalivata, Njoloma, Makumba and Thierfelder2017). However, there are mixed results on net C increase in CA systems (Cheesman et al., Reference Cheesman, Thierfelder, Eash, Kassie and Frossard2016; Ngwira, Sleutel and De Neve, Reference Ngwira, Sleutel and De Neve2012; Ngwira, Thierfelder and Lambert, Reference Ngwira, Thierfelder and Lambert2013; Rusinamhodzi et al., Reference Rusinamhodzi, Corbeels, Nyamangara and Giller2012) which does not seem as promising as measured in temperate regions (Corsi et al., Reference Corsi, Friedrich, Kassam, Pisante and Sà2012). Critical in C sequestration is the crop diversification component (rotation, intercropping, or agroforestry interventions), which provides additional C through biomass if not burned on the soil surface, grazed, or removed (Powlson et al., Reference Powlson, Stirling, Thierfelder, White and Jat2016).
Several other scholars also highlighted limitations and specific challenges that hamper widespread adoption of CA (Andersson and D'Souza, Reference Andersson and D'Souza2014; Arslan et al., Reference Arslan, McCarthy, Lipper, Asfaw and Cattaneo2014; Giller et al., Reference Giller, Andersson, Corbeels, Kirkegaard, Mortensen, Erenstein and Vanlauwe2015, Reference Giller, Witter, Corbeels and Tittonell2009). Some even claim, based on their existing knowledge of African farming systems, that CA systems will never be adopted by smallholder farmers due to too many implementation challenges (Giller et al., Reference Giller, Witter, Corbeels and Tittonell2009). Warranted or not, we can summarize these challenges as being agronomic (e.g. lack of sufficient crop residues as mulch, weed control, pest and disease carryover through crop residues, delays in increased crop productivity due to nitrogen (N) lockup, termites, etc.), socio-economic (culture, tradition, beliefs on how agriculture should be conducted; mixed messages from extension services, crop/livestock trade-offs, lack of input and output markets for some legumes, lack of machinery, and crop chemicals, etc.), and political (lack of political support to more environmentally friendly, albeit currently unconventional, farming systems). Furthermore, governmental programs that only stress food security initiatives without sustainable agriculture land management may be strong deterrents to widespread CA adoption, among other reasons (Thierfelder, Matemba-Mutasa and Rusinamhodzi, Reference Thierfelder, Matemba-Mutasa and Rusinamhodzi2015a; Thierfelder et al., Reference Thierfelder, Rusinamhodzi, Ngwira, Mupangwa, Nyagumbo, Kassie and Cairns2015b). However, despite these challenges, CA systems are still considered as one of the most appropriate climate-smart agriculture options under the conditions of southern Africa for a large proportion of mixed farming systems (Thierfelder et al., Reference Thierfelder, Chivenge, Mupangwa, Rosenstock, Lamanna and Eyre2017). Conservation Agriculture systems fail where soils are too wet, where organic matter levels are too low, and where the climate is too cool for successful implementation (Baudron et al., Reference Baudron, Thierfelder, Nyagumbo and Gérard2015c). As such, CA systems are at the core of national agricultural policies, and here in particular, climate-smart agriculture (CSA) policies and interventions in the region (Dougill et al., Reference Dougill, Whitfield, Stringer, Vincent, Wood, Chinseu, Steward and Mkwambisi2016; Whitfield et al., Reference Whitfield, Dougill, Dyer, Kalaba, Leventon and Stringer2015).
The challenges of crop residue retention and weed control in CA systems
This paper aims to look deeper into two main challenges that have been identified as critical for the success of CA systems in southern Africa. First, there is an unanswered question on how to deal with trade-offs in the use of crop residue for soil cover or for livestock feeding in mixed farming systems (Mupangwa et al., Reference Mupangwa, Thierfelder, Cheesman, Nyagumbo, Muoni, Mhlanga, Mwila, Sida and Ngwira2020). Should residues be kept on the soil surface or be fed to livestock (and perhaps returned as manure instead)? The trade-offs between the two production strategies usually go in favor of livestock, at least for large parts of southern Zimbabwe and Zambia, leading to overgrazing and insufficient ground cover (Baudron et al., Reference Baudron, Delmotte, Corbeels, Herrera and Tittonell2015a; Valbuena et al., Reference Valbuena, Erenstein, Homann-Kee Tui, Abdoulaye, Claessens, Duncan, Gérard, Rufino, Teufel, van Rooyen and van Wijk2012) (Fig. 1a). Trade-offs are further stretched by using crop residues for other purposes such as fuel for cooking, building material (Fig. 1b). What are the consequences, strategies, and potential ways to overcome this challenge? This is and continues to be one of the most critical questions in CA promotion.
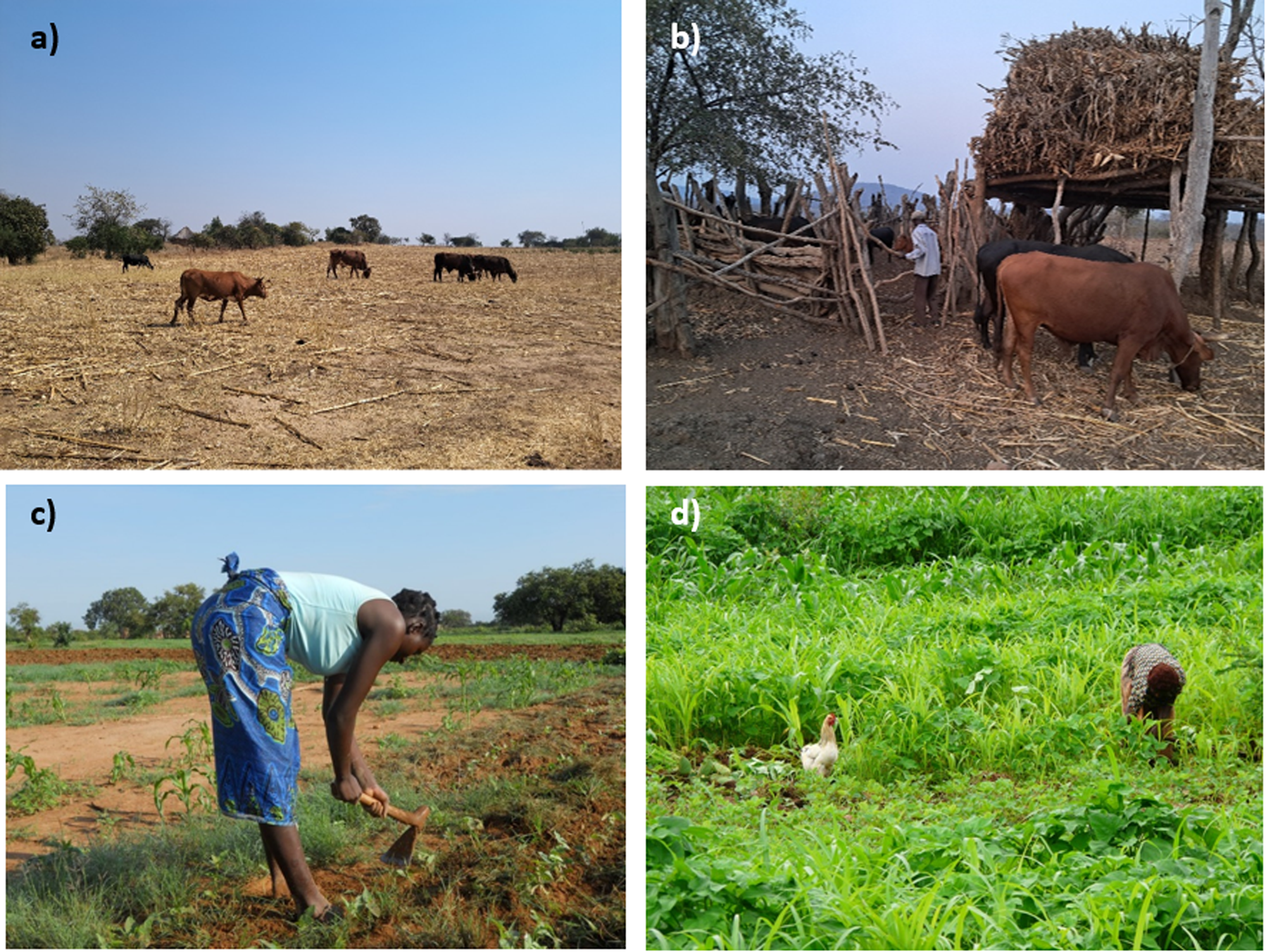
Figure 1. The two major impediments to the widespread adoption of Conservation Agriculture systems: (a) cattle grazing maize residues as soon as farmers finish crop harvesting in Rushinga, Zimbabwe; (b) maize residues that farmers use to feed their cattle during the off-season stacked in the cattle kraal in Rushinga in Zimbabwe; (c) smallholder farmers use hoes to weed their maize fields in Monze, Zambia; (d) farmer controlling weeds in a weedy field during the season, Bindura, Zimbabwe. Photo credits: Mhlanga B (CIMMYT); Thierfelder C (CIMMYT).
Second, weed control using herbicides in CA systems remains an essentially unquestioned answer. Most farmers who switch from conventional tillage systems to no-tillage agriculture face the challenges of increased weeds and their management especially during the early years after adoption (Fig. 1c and 1d). The change from conventional systems to CA also results in shifts in weed communities which require new knowledge from the farmers to control the new spectrum of weeds (Muoni et al., Reference Muoni, Rusinamhodzi, Rugare, Mabasa, Mangosho, Mupangwa and Thierfelder2014). An immediate approach to weed control is using herbicides and yet these may pose threats to human health and the environment if not used judiciously. Herbicides are often inaccessible to farmers and require knowledge to appropriately use them among other drawbacks. We must question this answer and ask, what could be the potential alternatives to chemical weed control? Besides more judicious use, what strategies can be employed to safely apply herbicides under smallholder farmers conditions? What is the scope for ecological, mechanical, and biological weed control options under smallholder farming conditions?
This paper partly draws lessons from recently completed large on-farm surveys conducted in Malawi, Zambia, and Zimbabwe from March to June 2021 by the International Institute of Tropical Agriculture (IITA) and the International Maize & Wheat Improvement Center (CIMMYT) under the ‘Understanding and Enhancing Adoption of Conservation Agriculture in Smallholder Farming Systems of Southern Africa (ACASA)’ project which was funded by the Royal Norwegian Government. The methodology, sampling procedure and part of the data from this study have already been published by Tufa et al. (Reference Tufa, Kanyamuka, Alene, Ngoma, Marenya, Thierfelder, Banda and Chikoye2023). We also synthesized other published literature to support any propositions.
In the following chapters, we will describe the challenge of crop residue retention and weed control under the conditions of southern Africa in more detail and further discuss different kinds of solutions to these problems. We end with a conclusion and a way forward.
The issue of residue retention in mixed crop/livestock systems
Smallholder farming systems in southern Africa, especially in livestock dominant areas of Zambia and Zimbabwe, are characterized by intensive crop/livestock interactions (Mkuhlani et al., Reference Mkuhlani, Mupangwa, MacLeod, Gwiriri, Nyagumbo, Manyawu and Chigede2020; Valbuena et al., Reference Valbuena, Erenstein, Homann-Kee Tui, Abdoulaye, Claessens, Duncan, Gérard, Rufino, Teufel, van Rooyen and van Wijk2012; Wall et al., Reference Wall, Thierfelder, Nyagumbo, Rusinamhodzi and Mupangwa2019). Predominantly, cattle, goats, and chicken are raised by smallholder farmers in these areas. In dryer locations, goats become more prominent and, in a few instances donkeys and sheep. Livestock plays a critical role in the farming system and society. It is used as (a) source of animal protein; (b) draft power; (c) nutrient supply (animal manure); (d) financial security in times of risk and disaster; (e) currency in remote areas where the banking systems are underdeveloped (barter trade); and (f) a sign of wealth and reputation in the farming community. For example, in Zimbabwe and Zambia, paying bride price using cattle (locally called Lobola) is common when marriage arrangements are negotiated between families (Thierfelder, Matemba-Mutasa and Rusinamhodzi, Reference Thierfelder, Matemba-Mutasa and Rusinamhodzi2015a; Thierfelder et al., Reference Thierfelder, Rusinamhodzi, Ngwira, Mupangwa, Nyagumbo, Kassie and Cairns2015b). The number of animals that must be paid for can be substantial. For example, marrying a woman from the Masvingo area in Southern Zimbabwe will lead to demands of 10–12 cattle as bride price which requires substantial herds or sufficient cash that can be paid in lieu. The amount can even be higher for women who have reached higher levels of education.
However, the contributions of livestock to food and nutrition security in sub-Saharan Africa are also one of the biggest challenges for farmers managing natural resources in rural settings. In southern Africa, the cropping season lasts from November to April, with increasingly shorter seasons due to climate change (Cairns et al., Reference Cairns, Sonder, Zaidi, Verhulst, Mahuku, Babu, Nair, Das, Govaerts, Vinayan, Rashid, Noor, Devi, San Vicente, Prasanna and Sparks2012). There is usually no rainfall from May to October, which leads to the drying up of all pastures and grazing areas and hence limited fodder available for livestock feed. Therefore, a logical strategy for livestock herders is to let all animals loose after harvest to graze crop residues freely (Fig. 1a). For instance, a recent survey conducted in Malawi, Zambia, and Zimbabwe (Tufa et al., Reference Tufa, Kanyamuka, Alene, Ngoma, Marenya, Thierfelder, Banda and Chikoye2023) showed that free grazing of crop fields after harvest is common in all surveyed countries (Table 1). Other farmers in Malawi report challenges in retaining crop residues due to mice hunters burning crop residues, and the burning of maize (Zea mays L.) stalks to cure tobacco nurseries (Bunderson et al., Reference Bunderson, Jere, Thierfelder, Gama, Mwale, Ng'oma, Museka, Paul, Mbale, Mkandawire, Tembo, Kassam, Mkomwa and Friedrich2017; Thierfelder, Matemba-Mutasa and Rusinamhodzi, Reference Thierfelder, Matemba-Mutasa and Rusinamhodzi2015a; Thierfelder et al., Reference Thierfelder, Rusinamhodzi, Ngwira, Mupangwa, Nyagumbo, Kassie and Cairns2015b; Tufa et al., Reference Tufa, Kanyamuka, Alene, Ngoma, Marenya, Thierfelder, Banda and Chikoye2023).
Table 1. Crop residue utilization in Malawi, Zambia, and Zimbabwe (% of households)
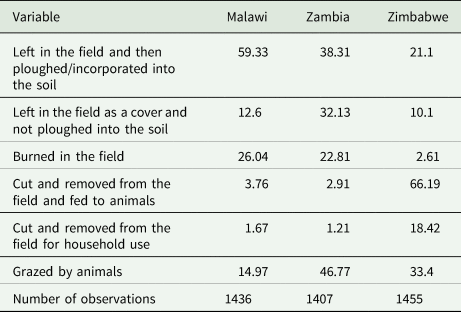
Source: ACASA survey, 2021.
About 68%, 88%, and 98% of farmers interviewed in Malawi, Zambia, and Zimbabwe, respectively, confirmed that open grazing is accepted after harvest in their communities (Table 2). However, more than 80% of the interviewed farmers in the three countries mentioned that traditional rules support farmers guarding their crop fields against free grazing (e.g., by fencing) if they want to retain crop residue as mulch (Table 2). Free roaming of livestock is gradual and increases towards the end of the rainy season. Farmers begin with livestock grazing their own fields after harvest (Fig. 1a). When all farmers have finished harvesting, free-roaming animals become dominant and livestock graze in any community fields without restriction.
Table 2. Free grazing of crop fields after harvest and traditional rules to guard against free grazing (% of yes response)
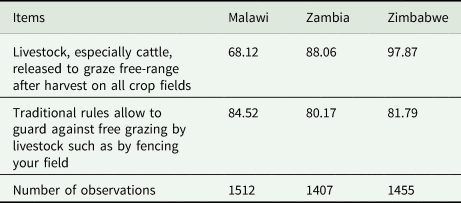
Source: ACASA survey, 2021.
Exclusion from grazing could be enforced through local by-laws but cultural habits and norms often make it challenging to sanction others from grazing their plots. This is the dilemma on communal land in southern Africa where individual farmers do not have exclusive tenure rights to use land parcels and open grazing is the de facto land-use option in the dry season. It leads to the classic problem in natural resource management called the ‘tragedy of the commons’. The impact of free grazing can be substantial, leading to bare fields at the onset of the next cropping season. Due to the competition for residues from roaming livestock, some farmers have resorted to exporting the residues from their fields to stacks in their cattle kraals to use these for feeding livestock and applying the remainder to their fields before the first rains (Fig. 1b).
Another unintended consequence of open grazing is the increased labor burden for land preparation. If farmers prepare basins or rip lines immediately after harvest, these are trampled on by livestock during the dry season and would require redoing the basins just before planting.
Maintaining crop residues in ungrazed areas can have significantly positive effects. In a study done in the Rift Valley of Ethiopia, livestock exclosure from cropping areas positively affected soil fertility and crop yields (Baudron et al., Reference Baudron, Mamo, Tirfessa and Argaw2015b). Exclosure over eight years led to an 80% increase in soil carbon and a 70% increase in teff yield in the ungrazed area, which shows the magnitude of the impact of livestock on soil fertility.
CA farmers, who benefit on one hand from the livestock (e.g., manure and draft power), suffer on the other hand from grazing of their precious crop residues that they intend to retain on the soil surface (Baudron et al., Reference Baudron, Delmotte, Corbeels, Herrera and Tittonell2015a) which is not matched by supplementary fodder production (Mupangwa and Thierfelder, Reference Mupangwa and Thierfelder2014) as traditionally practiced in temperate regions. Besides livestock feed, crop residues are used as sources of energy for cooking and heating, and as straw for fencing. This adds another dimension to the tradeoffs for residue use as mulch.
Increased demand for meat products and animal-derived food means that the pressure on land will increase in the coming decades (Herrero et al., Reference Herrero, Havlík, Valin, Notenbaert, Rufino, Thornton, Blümmel, Weiss, Grace and Obersteiner2013). Livestock numbers will surpass the carrying capacity of land and likely lead to further degradation (Springmann et al., Reference Springmann, Clark, Mason-D'Croz, Wiebe, Bodirsky, Lassaletta, De Vries, Vermeulen, Herrero and Carlson2018).
In addition to grazing crop residues by roaming cattle, the region is plagued by uncontrolled veld fires (wildfires). Residues are often considered waste, and, to ‘clean up’ the dirty land, residues are being removed through burning or export. The recent ACASA study results showed that 26% of the sampled households in Malawi and 23% of the same in Zambia reported burning crop residues (Table 1). This net export of carbon from the field has consequences on the soil fertility status (Gentile et al., Reference Gentile, Vanlauwe, Chivenge and Six2011) and the long-term sustainability of southern African landscapes. In the past, this would have been replenished by long fallow periods, which are no longer possible due to increasing population pressure and the need to increase food production at the country level.
Another issue is the source of crop residue used by farmers. If mulch materials are collected from natural forests, this could lead to degradation or deforestation in cases where trees are cut down. Crop residues from maize are the predominant source in Zambia and Malawi. In Zimbabwe, farmers often use Hyparrhenia grass species as the main source of mulch. Other important sources of mulch besides grass are groundnut and soybean residues and shells. This high reliance on maize residue for mulching in the region highlights the significance of crop-livestock trade-offs mentioned earlier.
Previous research shows that a lack of crop residues in smallholder CA systems may be counterproductive in the longer term (Govaerts et al., Reference Govaerts, Sayre, Ceballos-Ramirez, Luna-Guido, Limon-Ortega, Deckers and Dendooven2006, Reference Govaerts, Verhulst, Castellanos-Navarrete, Sayre, Dixon and Dendooven2009) as most soil quality improvements are associated with the retention of crop residues (Wall et al., Reference Wall, Thierfelder, Hobbs, Hellin, Govaerts and Kassam2020). Practicing no-tillage without crop residue retention can lead to soils with very hard and impenetrable soil surfaces resulting in negative outcomes such as soil crusting (Giller et al., Reference Giller, Andersson, Corbeels, Kirkegaard, Mortensen, Erenstein and Vanlauwe2015) and should, therefore, be avoided. This is supported by a study from Zimbabwe that tested yield response to different combinations of CA principles which showed that no-tillage alone without mulching and crop rotation leads to a decline in yields (Mhlanga et al., Reference Mhlanga, Ercoli, Pellegrino, Onofri and Thierfelder2021).
No-tillage and crop residue retention encourage soil life and microbial activity benefiting both above and below ground biodiversity (Mashavakure et al., Reference Mashavakure, Mashingaidze, Musundire, Nhamo, Gandiwa, Thierfelder and Muposhi2019; Mhlanga et al., Reference Mhlanga, Muoni, Mashavakure, Mudadirwa, Mulenga, Sitali and Thierfelder2020; Muoni et al., Reference Muoni, Mhlanga, Forkman, Sitali and Thierfelder2019). Research by Muoni et al. (Reference Muoni, Mhlanga, Forkman, Sitali and Thierfelder2019) and Thierfelder and Wall (Reference Thierfelder and Wall2010) confirmed that no-tillage with residue cover significantly increases the abundance of earthworms which are most important to develop a conducive soil pore structure (Kladviko, Griffith and Mannering, Reference Kladviko, Griffith and Mannering1986a; Kladviko, Mackay and Bradford, Reference Kladviko, Mackay and Bradford1986b). A positive outcome of ground burrowing macro-fauna is improved infiltration into deeper layers and an increase in available soil moisture for plant growth in times of in-season dry spells or droughts (Mhlanga and Thierfelder, Reference Mhlanga and Thierfelder2021). Crop residues have also been identified as being the most critical factor in maintaining yield stability and resilience in fully factorial CA omission trials conducted across multiple locations in southern Africa (Mhlanga et al., Reference Mhlanga, Ercoli, Pellegrino, Onofri and Thierfelder2021).
However, residue retention and its effects on soils may not always be positive: Besides increased water retention and gradually improving soil quality, there can be increased N lockup and waterlogging as negative side effects especially in high rainfall areas. Previous research by Mupangwa et al. (Reference Mupangwa, Nyagumbo, Mutsamba, Mhlanga and Mataruse2018) highlighted the challenges with N lockup in response to an increasing body of soil organisms after crop residues are applied. Issues with N lockup and dynamics in response to residue retention of different quality (e.g. C:N ratio) have also been acknowledged by Gentile et al. (Reference Gentile, Vanlauwe, Chivenge and Six2011) in trials in Kenya and are site-specific to the soil and environmental conditions.
According to Mupangwa et al. (Reference Mupangwa, Nyagumbo, Mutsamba, Mhlanga and Mataruse2018), soil micro-organisms are dependent on organic C and N to form their body structure. If residues are applied, this usually leads to an exponential increase in fauna which requires additional N fertilization to overcome the N-lockup (Fig. 2). This is more pronounced when residues of high C:N ratio such as maize residues are applied. Soil microorganisms have a C:N ratio of about 8:1 and can decompose residues up to a ratio of 24:1 efficiently (Cates et al., Reference Cates, Ruark, Hedtcke and Posner2016). Since maize has a C:N ratio of about 57:1, this means that microbes will scavenge more N from the soil to effectively decompose residues with higher C:N ratio, leading to N immobilization (lock-up).
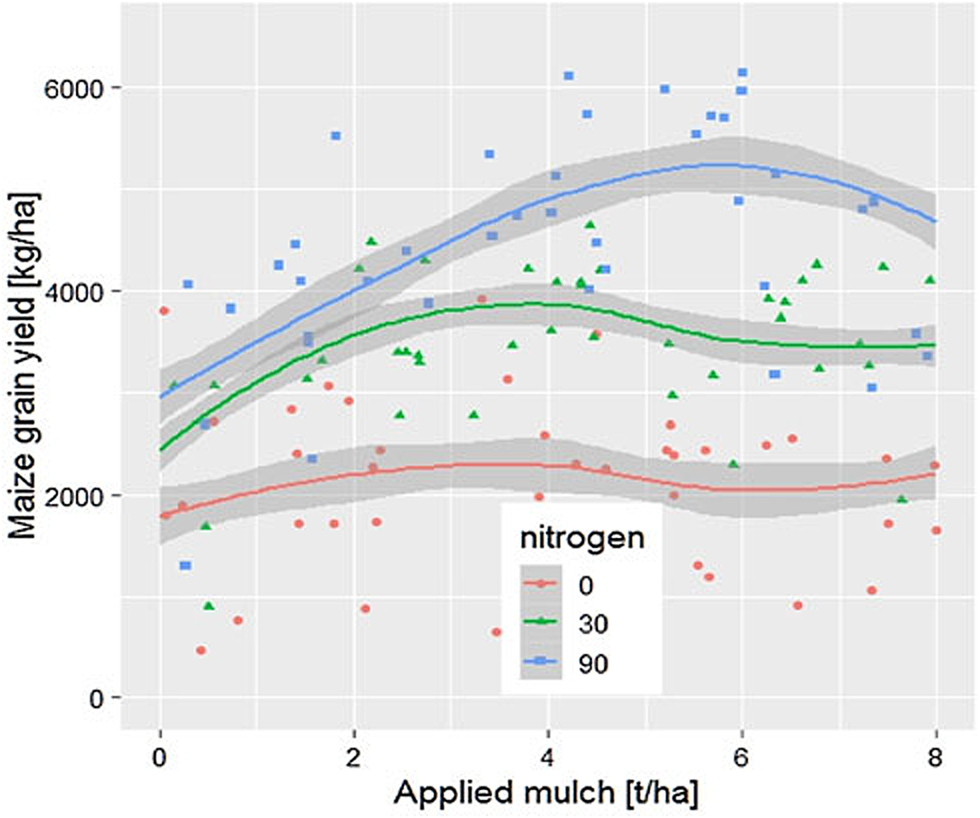
Figure 2. Interaction effects of residue biomass levels and N fertilizer on grain yield at the University of Zimbabwe site in the 2014 growing season. Adapted from Mupangwa et al. (Reference Mupangwa, Thierfelder, Cheesman, Nyagumbo, Muoni, Mhlanga, Mwila, Sida and Ngwira2019).
In trials carried out at the University of Zimbabwe farm, increasing levels of crop residues were applied in combination with increasing N levels. The data shows that with zero application of N, the increase in crop residue quantity did not result in increased yield benefits. Only a marginal increase in the zero N treatment could be attributed to improved soil moisture retention, weed suppression and/or availing other crop nutrients through the residues. However, the application of 30 kg N ha−1 and 90 kg N ha−1 resulted in notable yield increases even without residue application (Fig. 2). The application of 30 kg N ha−1 resulted in higher yield response up to when 4 t ha−1 of crop residues were applied and started to decline as this was probably not adequate to reduce the negative effects of N immobilization. An application rate of 90 N kg ha−1 moved the peak response to 6 t ha−1 of residues that could be supported without yield penalties (Mupangwa et al., Reference Mupangwa, Thierfelder, Cheesman, Nyagumbo, Muoni, Mhlanga, Mwila, Sida and Ngwira2020). This shows that cereal residue retention must go hand in hand with sufficient N fertilization in N deficient environments at least in the early stages of conversion to CA. The application of N helps alleviate the negative effects of retaining cereal residues and promotes the response of crops to the positive effects of these residues such as weed suppression, moisture retention, etc. This was also demonstrated by Kinyua et al. (Reference Kinyua, Mucheru-Muna, Bolo and Kihara2021) in which a change in residue quantity at a threshold of N quantity resulted in an insignificant maize yield increase. In cases where N application through fertilizers cannot be afforded, mixing cereal residues with legume residue with a low C:N ratio may help alleviate the negative effects of N immobilization or improve response to beneficial effects. However, immobilization can also actively preserve soil N, thus reducing losses to processes associated with rapid mineralization.
Crop residues can be a carrier of leaf diseases which have been observed in Malawi, although this can be reduced when cereals are continuously rotated with legume species and the crop residues that are carried over are alternating between species. Increases in gray leaf spot (Cercospora zeae-maydis) which provides an early-stage inoculum for the next maize crop resulting in infections would be a common threat. Similarly, tan spot (Pyrenophora tritici-repentis) and crown rot (Fusarium pseudograminearum) may be carried over to the following season (Giller et al., Reference Giller, Andersson, Corbeels, Kirkegaard, Mortensen, Erenstein and Vanlauwe2015). However, recent breeding efforts in southern Africa have reduced these leaf diseases in germplasm which is a positive research advancement in breeding efforts for CA.
In summary, the functioning of CA systems in southern Africa is strongly linked to and dependent on crop residue retention, which provides soil C, increases infiltration, reduces run-off, regulates temperatures, reduces evaporation, and increases available soil moisture. Besides this, crop residues have significant positive effects on soil quality and health and increase the resilience and yield stability of CA systems. However, crop residues are a scarce resource in the agro-ecologies of southern Africa and are also needed for livestock feed which creates major trade-offs affecting the feasibility of complete CA systems in southern Africa. We will further explore options to reduce this crop/livestock conflict in the section ‘Discussion’.
Weeds and their control - herbicides the ‘Achilles heel’ of CA
Weeds are one of the major constraints and economically important challenges in smallholder cropping systems in southern Africa (Mashingaidze et al., Reference Mashingaidze, Madakadze, Twomlow, Nyamangara and Hove2012; Tibugari, Chiduza and Mashingaidze, Reference Tibugari, Chiduza and Mashingaidze2020). The recent ACASA study, partially published by Tufa et al. (Reference Tufa, Kanyamuka, Alene, Ngoma, Marenya, Thierfelder, Banda and Chikoye2023), identified labor-intensiveness and weed infestation as key constraints that can be drivers of CA dis-adoption in Malawi, Zambia, and Zimbabwe. Data collected confirmed that weeding is the main farm operation for which about half (49%) of the interviewed farm households faced labor constraints (Fig. 3). At the country level, 62% of 1407 farm households interviewed in Zambia cited labor constraints during weeding compared to 43% of 1455 households and 42% of 1512 households in Zimbabwe and Malawi, respectively. Due to their competition with crops for resources such as water, nutrients, and sunlight and their potential to harbor insects and diseases or cause allelopathic effects, weeds can cause substantial crop losses (Ball et al., Reference Ball, Caldwell, DiTommaso, Drinkwater, Mohler, Smith and Ryan2019; Gage and Schwartz-Lazaro, Reference Gage and Schwartz-Lazaro2019). Weeds, for example, have been reported to cause total crop losses worth billions of dollars per annum especially in fields dominated by aggressive and highly competitive species such as the parasitic weed Striga [e.g. Striga asiatica (L.) Kuntze and Striga hermonthica] and couch grass [Cynodon dactylon (L.) Pers.] (Kountche, Al-Babili and Haussmann, Reference Kountche, Al-Babili, Haussmann, Das and Padmaja2016; Lee and Thierfelder, Reference Lee and Thierfelder2017; Vogel, Reference Vogel1995) affecting millions of people in sub-Saharan Africa (Scholes and Press, Reference Scholes and Press2008).
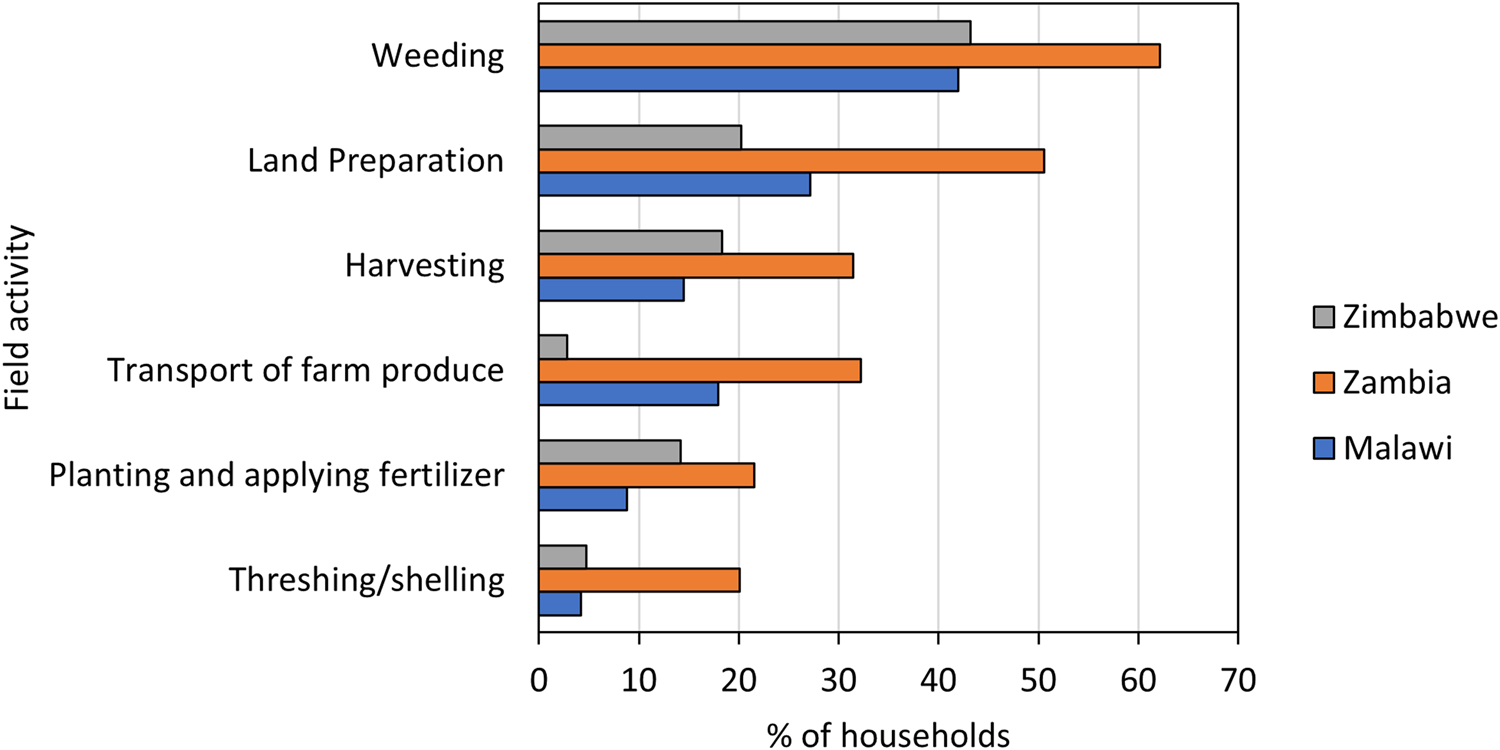
Figure 3. Responses from interviewed farmers to the question, ‘For which farm operation do you face the most labor constraints?’. The values are percentages of households interviewed in Malawi, Zambia, and Zimbabwe. Source: ACASA survey, 2021.
The primary reason to practice soil tillage is to control weeds which has been used as an effective tool since ancient times (Chauhan, Singh and Mahajan, Reference Chauhan, Singh and Mahajan2012). Tillage affects weeds by uprooting, dismembering, burying, and changing the soil environment which prevents germination and establishment of weed seedlings. Tillage is also used to incorporate herbicides into the soil and to remove excess surface residue that may impede crop emergence. CA practices that advocate for reduced intensity or frequency of tillage therefore have serious implications for weed management. Limited soil inversion, the retention of mulch on the soil surface, and diversification of crops often lead to shifts in weed communities, species distribution, densities, and composition and hence require new management strategies for the new weed spectrum (Mhlanga et al., Reference Mhlanga, Cheesman, Maasdorp, Muoni, Mabasa, Mangosho and Thierfelder2015). For example, due to reduced tillage, weeds that propagate through rhizomes such as couch grass (Cynodon dactylon L.) are promoted since they are not disturbed at all and yet such weeds are very difficult to control and affect crop productivity (Malik et al., Reference Malik, Kumar, Yadav and McDonald2014).
Weed control in general is one of the impediments to the adoption of CA in southern Africa and this is because weed communities respond differently depending on the combination of CA principles applied, soil type, and climatic conditions. This calls for a site-specific control strategy which may be difficult for adopting farmers (Mhlanga et al., Reference Mhlanga, Ercoli, Thierfelder and Pellegrino2022). Chemical weed control is the most adopted and effective means of weed management in CA systems (Lee and Thierfelder, Reference Lee and Thierfelder2017), where most farmers, commonly use non-selective desiccant herbicides such as glyphosate [N-(phosphonomethyl) glycine], paraquat (1,10-dimethyl-4,40-bipyridinium), and/or glufosinate [2-Amino-4-[hydroxy(methylphosphonoyl)] butanoic acid]] to control weeds that are present at planting (Lee and Thierfelder, Reference Lee and Thierfelder2017). Selective post-emergence herbicides are used to control weeds that emerge together with or after the crop and these are selective for the crop e.g. Stella star® which is used in maize systems to control grasses.
Continuous and frequent use of the same herbicides induces resistance in weeds against those herbicides or may contribute to offsite pollution and other environmental and health concerns. Hence, the use of alternative and new herbicides with different chemical structures and modes of action is a necessary practice to minimize the evolution of herbicide-resistant weeds. Furthermore, post-emergence weed control with herbicides may not be feasible in intercropping systems which is practiced widely by small-scale farmers as either the cereal or the legume is affected by a specific mode of action.
CA systems became more common when herbicides were made more widely available by the chemical industry. For example, when Monsanto removed its patent on glyphosate (which enhanced the off-patent herbicide formulation production) (Haggblade et al., Reference Haggblade, Minten, Pray, Reardon and Zilberman2017), there was an exponential increase in the adoption of CA systems mostly on large commercial farms due to the reduced cost of the herbicide (Wall, Reference Wall2007). The main reasons for accelerated CA adoption during this time were increased fuel costs for ploughing, which could have been avoided with zero-till equipment and spraying. However, the advent of crop varieties that are resistant to glyphosate has increased the application frequencies and doses of herbicides especially in Brazil, Argentina, and the USA (Dill, Reference Dill2005). Application of glyphosate in ‘roundup-ready’ crops provides a completely clean seed bed after application without damaging the crop of interest, although the recent lack of global public acceptance due to health concerns hinders larger expansion (Dill, Reference Dill2005).
Chemical weed control is commonly referred to as the ‘Achilles heel' of CA in southern Africa (Giller et al., Reference Giller, Andersson, Corbeels, Kirkegaard, Mortensen, Erenstein and Vanlauwe2015). Cash-constrained farmers in this region often do not apply chemical weed control due to a) lack of capital to purchase the products; b) lack of knowledge on the different products; c) education on how to apply them; d) lack of safety measures and protection with potential negative side effects on human health (Lee and Thierfelder, Reference Lee and Thierfelder2017). Increasing environmental concern in the developed world about chemical weed control in Africa has also become more widespread although there is often a limited understanding of the alternatives and their effects on smallholders (Gianessi, Reference Gianessi2009).
Weed control with herbicides saves labor and this benefits mainly women and children who are traditionally assigned to do the laborious tasks of manual weed control. In Malawi, where manual farm labor is common, weed control is done with a hand hoe. The labor demand ranges between 10 and 15 labor days ha−1 (Thierfelder et al., Reference Thierfelder, Bunderson, Jere, Mutenje and Ngwira2016) but can increase substantially if complicated grass weed species (e.g. couch grass) are present. In Zambia, Haggblade and Tembo (Reference Haggblade and Tembo2003) estimated that the use of herbicides reduces labor demand from 50–70 labor days ha−1 to just 10–20 days, providing social benefits to women and children who are mainly involved in the activity. This frees time for other economic activities or allows for resting and/or childcare.
In view of the limitations, chemical weed control may not be the answer to CA extension and widespread scaling. Many products that are now banned in Europe are still in use in Africa such as atrazine (active ingredient 2-chloro-4-ethylamino-6-isopropylamino-13,5-triazine) which stays in the soil for a long time, metabolizes, accumulates and whose residual effects may have detrimental effects on the environment and the succeeding crops (Jablonowski, Schäffer and Burauel, Reference Jablonowski, Schäffer and Burauel2011; Liu et al., Reference Liu, Zhou, Guo, Ma and Yang2021). Glyphosate is a different example of a common herbicide that has been associated with health concerns in mammals (Seralini, Reference Seralini2020) and has a widespread negative press due to its use and overuse by commercial farmers around the world. Also, the emergence of resistance to the herbicide by certain grass species requires more use of alternative products, thereby increasing the environmental side effects (Green and Siehl, Reference Green and Siehl2021). Although many chemical companies are working to reduce this resistance to herbicides, the weeds are also always evolving and this is presenting a continued challenge (Matzrafi et al., Reference Matzrafi, Gadri, Frenkel, Rubin and Peleg2014).
To date, the use of herbicides outside the commercial farming sector in Africa is still very low. Farmers rarely use the products due to cash constraints and limited access. This renders the question, ‘what are the alternatives to chemical-based weed control strategies and how can CA systems be made to work under the conditions of smallholder farmers in rural communities?’ Other questions include finding ways to maintain adequate soil cover (at least 30%), establish appropriate crop associations and rotations for CA systems, and the identification of locally adapted cover crops. Thus, there is a need to develop integrated weed management systems adapted to smallholder farming conditions. Alternative practices based on biological, mechanical, and cultural control will be discussed in the subsequent chapters.
Discussion
What are the options to overcome crop residue shortage and to improve its management in mixed crop/livestock systems?
As previously outlined, the retention of crop residues in CA systems for permanent soil cover has been met with multiple challenges spanning from low biomass production to crop-livestock competition and alternative use of crop residues as an energy source and for fencing in the case of maize straw. These challenges have resulted in insufficient soil cover in CA systems of southern Africa. As residue retention or mulching is critical, solutions need to be highlighted and disseminated to farmers to realize the benefits. These solutions are based on the generation of more biomass and developing strategies of preservation. Here, we present some possible solutions.
In situ fodder and biomass production
Increasing biomass production on-farm through in situ fodder or biomass production can relieve the pressure of crop residue competition for livestock feeding and/or use as soil cover as most livestock feed in southern Africa comes from natural pasture grazing and crop residues. The contribution of cultivated fodder to the total dry matter intake is low and it is often restricted to commercial farming. There are however some examples from communities in southern Africa in which cultivated fodder is utilized. For example, a study conducted in Mutandalike, Choma district in Zambia showed that the shares of total dry matter intake from various feed sources were natural pasture (64%), crop residues (30%), collected fodder (3%), cultivated fodder (2%), and purchased feeds (1%) (Mulindi et al., Reference Mulindi, Mwansa, Mwilima and Sikaceya2021). Other similar studies conducted in Masopo in Choma district estimated the contributions of natural pasture, crop residues, collected fodder, cultivated fodder, and purchased feeds at 46%, 27%, 13%, 6%, and 8%, respectively in total dry matter intake (Mwilima, Mulindi and Mwansa, Reference Mwilima, Mulindi and Mwansa2021). These studies also indicated that velvet bean [Mucuna pruriens (L.) DC. var. utilis (Wall. Ex-Wight) Baker ex Burck], Rhodes grass (Chloris gayana Kunth) and Leucaena (Leucaena leucocephala (Lam.) de Wit) were the major cultivated fodder species in the area.
In Malawi, dairy farmers exercise zero grazing with feed coming mostly from established grass pastures and concentrates of maize bran. The main constraints for in situ fodder production, especially pasture improvement in Malawi, are high costs of establishment of the pasture attributed to fertilizer use and fencing; and shortage of land (Kumwenda and Ngwira, Reference Kumwenda and Ngwira2003). From these results, an apparent strategy that emerges to relieve the use of crop residues as livestock feed is to increase the productivity of natural pastures, so these provide more than the 46–64% feed dry matter as estimated by Mulindi et al. (Reference Mulindi, Mwansa, Mwilima and Sikaceya2021) and Mwilima, Mulindi and Mwansa, (Reference Mwilima, Mulindi and Mwansa2021). This can be done via improved natural pasture management by establishing highly productive fodder grass and forage legume species or adjusting (limiting) the number of livestock in these systems.
Intercropping cereals with multipurpose legumes that can be used as both fodder and soil cover material is a possible strategy for reducing competition for biomass. In such a system, there is simultaneous production of biomass from associated crops, thus increasing the amount of biomass per unit area. A study by Azim et al. (Reference Azim, Khan, Nadeem and Muhammad2000) showed that intercropping cowpea into maize using different spatial patterns led to improved biomass production as compared to sole maize cropping (Fig. 4). The increase in biomass was also accompanied by improved fodder quality. Thus, intercropping legumes into cereal systems provides an opportunity to increase biomass and fodder quality while reducing competition for crop residues.
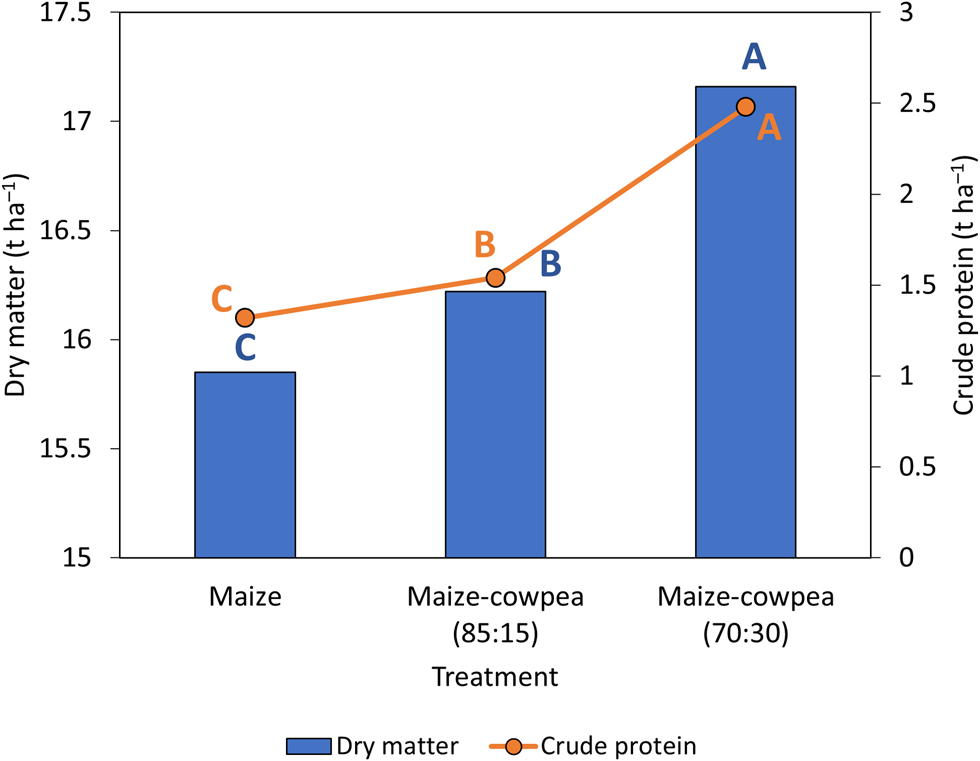
Figure 4. Different spatial arrangements and population ratios of maize-cowpea intercrops on biomass and nutrient yield. Adapted from Azim et al. (Reference Azim, Khan, Nadeem and Muhammad2000).
Studies on the productivity gaps of natural pastures are urgently needed to inform new feeding and grazing strategies. The issue of optimizing natural pasture productivity or of livestock densities (in time and space) remains an unanswered question in our view.
Nevertheless, a bottleneck will continue to be the culture of free grazing which will have to stop in the long run as has happened in most areas of high- and middle-income countries. There is an urgent need to improve grazing regulations so that crop residues on private farmlands are protected and thereby give land operators more control of what happens with the crop residue. We take this up in the next sections below.
Improved grazing systems
There are two grazing systems that are commonly practiced in southern Africa, the continuous unregulated and regulated systems. The continuous unregulated grazing (including on farmlands after harvest) is borne out of traditional ‘communal’ land tenure systems preponderant in most of southern Africa. This is de facto open access. Although this system keeps capital costs at the minimum, it is very inefficient in terms of resource use and profitability. Yet, the imperatives of a modern mixed crop-livestock system require institutional changes to these traditional systems to align them with private and more secure property rights regimes. For example, socializing crop residue (by allowing unrestricted grazing on cropped plots) means that private appropriation of this vital resource is weakened, especially in its use as mulch. The only way for farmers to secure the crop residue for private use (as mulch) is to harvest these, keep them away out of reach of livestock, and return them to the field when the new season starts (Muoni and Mhlanga, Reference Muoni and Mhlanga2014). This is a potentially costly and labor-intensive operation which is also sub-optimal from an agronomic point of view as it goes against the recommendation for a near-permanent soil surface mulch (Muoni and Mhlanga, Reference Muoni and Mhlanga2014). Unrestricted grazing also means that weeds and other perennial plants/crops that grow during the off-season and can potentially add biomass to cropping systems are likely to be grazed. For instance, in agroforestry-based cropping systems that are common in southern Africa that involve perennial shrub and trees such as pigeonpea (Cajanus cajan (L.) Millsp.) and Gliricidia (Gliricidia sepium (Jacq.) Kunth ex Walp), loose livestock tends to browse the leaves and branches, thus compromising productivity and hence more competition for biomass. This has negative consequences on soil organic carbon storage due to high biomass intake, reduced growth period, and increased animal disturbance which reduces photosynthetic activity (Hennessy et al., Reference Hennessy, De Vliegher, Die Dean, Klumpp, Poilane, Richmond, Schönhart, Svoboda and van Rijn2018). If the institutional arrangement (of unrestricted livestock grazing) is due to an insufficient supply of feed resources (especially during the dry season) from designated grazing lands, this still leaves the other question as to what the optimal livestock density needs to be in these communal-based and tightly connected crop-livestock systems. Alan Savory, one of the pioneers of holistic grazing systems development in Zimbabwe clearly outlines this in his work and suggests intensive grazing in small paddocks and shifting animals more frequently between these outlined areas. In his assessment, this will increase sustainability, and reduce the impact on the environment while increasing profits for farmers (Savory and Butterfield, Reference Savory and Butterfield2016; Savory and Parsons, Reference Savory and Parsons1980).
A shift to a more controlled and holistic grazing system will require enacting local and mutually agreed bylaws to regulate and essentially prohibit cattle grazing on private plots or to promote more intensive (paddock-based) and less communal-based livestock systems. This remains a complex and important unanswered question for sustainability that must be urgently resolved.
Local bylaws
Local bylaws are rules established and enforced by local leadership (headmen or chiefs or village heads). The most common local bylaws are those restricting free grazing of livestock during the rainy season, which is generally accepted by all community members. However, there are also local bylaws that restrict year-round free grazing of livestock and fire outbreaks. For example, a village agricultural committee (VAC) in collaboration with other local institutions has developed bylaws that restrict livestock from free grazing during the wet and dry seasons in Mpokwa Extension Planning Area (EPA) in Zomba district in Malawi (Zulu et al., Reference Zulu, Adams, Chikowo and Snapp2018). However, in other parts of Malawi, the use of bylaws to regulate livestock grazing during the dry season does not exist or is not enforced. Lack of enforcement of the bylaws is because (1) community members within a particular village are related and live as a clan and thus they find it difficult to punish a relative; and (2) conflict of interest by those who are responsible for enforcing (Zulu et al., Reference Zulu, Adams, Chikowo and Snapp2018).
In Eastern Zambia, there are some bylaws that protect improved fallows from grazing and fire outbreaks (Ajayi, Akinnifesi and Ajayi, Reference Ajayi, Akinnifesi and Ajayi2016; Ajayi and Kwesiga, Reference Ajayi and Kwesiga2003). In some parts of Zimbabwe, free grazing is allowed after harvest. However, there are bylaws that define dates for opening crop fields for communal grazing and enable farmers to enclose a maximum of 0.4 ha of a crop field to protect the crop residues from communal grazing (Homann-Kee Tui et al., Reference Homann-Kee Tui, Adekunle, Lundy, Tucker, Birachi, Schut, Klerkx, Ballantyne, Duncan and Cadilhon2013). This leaves another question regarding the social institutions to support CA: in communities with strong kinship ties, why is it difficult to come up with a common understanding that unregulated grazing is not beneficial for the economic and environmental health and sustainability of a community?
Use of non-palatable material
The use of non-palatable residues aims to replace for example maize crop residues as the sole mulching material given the competition from other uses. The sunnhemp variety common rattlepod (Crotalaria grahamiana Wight & Arn) would be such a candidate which is very well adapted to the agro-ecological conditions of southern Africa and is not liked by grazing cattle or goats. This proposition is justifiable where the non-palatable mulch biomass is freely available either from private or communal areas. It still does not answer the question of environmental sustainability. Would it be more sustainable for farmers to grow these? If the suggestion is based on harvesting non-palatable biomass from communal areas, then are we replacing one communal problem (free grazing) with another (harvesting and transfer of biomass from communal lands into cropping areas). This could also lead to another unintended consequence of forest degradation if the mulch materials are obtained from natural forests or indeed deforestation if farmers resort to cutting down trees in the process of trying to access twigs and branches to use as mulch.
Will there be adequate checks and balances (e.g. who assigns and monitors the harvesting quotas among community members)? In other words, how can another version of ‘the tragedy of the commons’ be avoided? The alternative is for farmers to grow these themselves, assuming they have the land, labor, and other resources to do so. The economics of growing non-palatable species for use as mulch or the sustainability (and desirability) of exploiting communal grasslands as sources of unpalatable mulch remains an unanswered research question.
How to overcome the challenges of weed control?
Weeding challenges in CA systems have been addressed worldwide by simply using herbicides and previous success in implementing CA worldwide has been associated with the use of herbicides (Bolliger et al., Reference Bolliger, Magid, Amado, Scora Neto, Dos Santos Ribeiro, Calegari, Ralisch and De Neergaard2006). However, as mentioned before, chemical weeding is often not affordable and, in some cases, inaccessible to the smallholder farmers and at the same time environmentally unfriendly (Rodenburg et al., Reference Rodenburg, Johnson, Dieng, Senthilkumar, Vandamme, Akakpo, Allarangaye, Baggie, Bakare and Bam2019). The use of herbicides, though effective when properly applied, requires knowledge and without basic training this may be an unviable option as they may pose a risk to the health of the farmers (Manda, Reference Manda2021). Thus, possible alternatives need to be identified to overcome this challenge. In the following sub-sections, we discuss some of the possible options that farmers can or should explore in controlling weeds under CA systems.
Alternative weed control options to chemical control (mechanical, biological)
Mechanical weeding (MW) is a more economical alternative to manual weeding although this attracts high initial investments to cover the costs of specialized machinery. It involves the use of implements that range from handheld tools (such as finger weeders) to more sophisticated tools pulled by either animals or powered engines (Lee and Thierfelder, Reference Lee and Thierfelder2017). The aim of these tools is to beat, uproot, or bury weeds growing in between crop rows (Hussain et al., Reference Hussain, Farooq, Merfield, Jabran, Jabran and Chauhan2018). Mechanical weeding can be used in CA systems provided the implements being used do not disturb the surface soil excessively. For example, weeds can be controlled using a slasher, but this is mainly possible at the beginning of the season before sowing or before the crops emerge and is usually only functioning on broadleaved weeds. Mechanical weeding may also be difficult to use when intercropping is practiced in CA systems as maneuvering between crop rows may be difficult. Planting the intercrops in the same row as the main crop can be an alternative to avoid the companion crop being weeded out. Despite being economical, MW often disturbs the superficial soil surface which makes it a less preferred method in CA systems hence the need to identify other means that are less disturbing such as biological control (Abbas et al., Reference Abbas, Zahir, Naveed and Kremer2018).
Biological control in the context of southern Africa involves the use of living organisms such as cover crops, microorganisms, insects, etc., to control weeds (Zachariades et al., Reference Zachariades, Van Der Westhuizen, Heystek, Dube, McConnachie, Nqayi, Dlomo, Mpedi and Kistensamy2021). The most common biological control under cereal-based CA systems is the rotation or intercropping of cereals with vigorously growing legumes such as cowpea (Vigna unguiculata Walp), velvet bean, or lablab (Lablab purpureus L.). Rotating or intercropping cereals with other crops enhances the canopy crop cover which in turn smoothers and compromises weed growth and development and eventually results in death through smothering (Chikoye, Ekeleme and Udensi, Reference Chikoye, Ekeleme and Udensi2001). Growing maize in rotation with vigorously growing cowpea, velvet beans, common rattlepod, or lablab has been shown to reduce weed densities in the subsequent maize crops by 18 to 42% as compared to monocropping in the long-term (Fig. 5) (Mhlanga et al., Reference Mhlanga, Cheesman, Maasdorp, Muoni, Mabasa, Mangosho and Thierfelder2015).
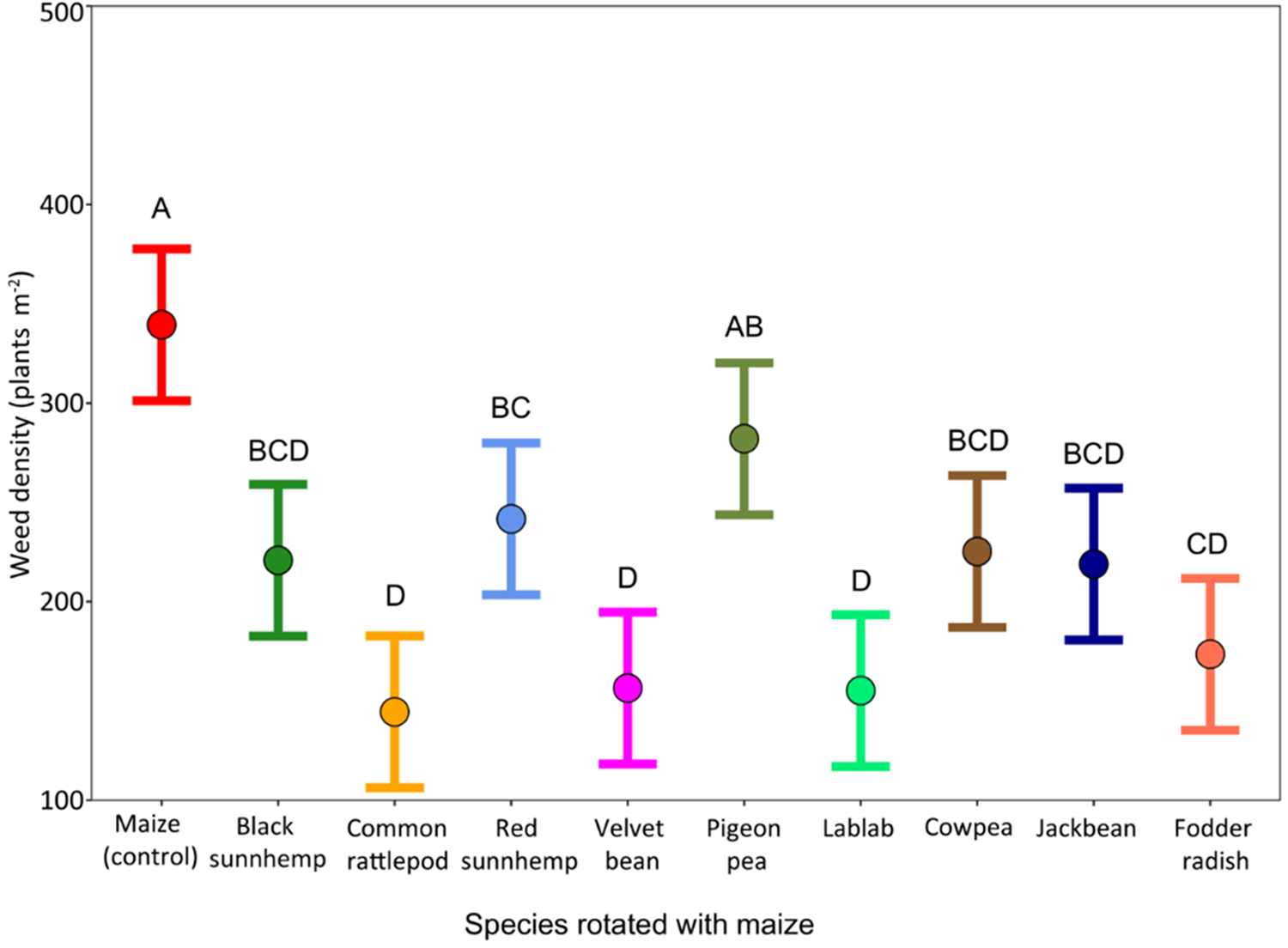
Figure 5. The effects of rotating maize with different grain and cover crops on weed density in a sand soil under Conservation Agriculture in Zimbabwe. Adapted from Mhlanga et al. (Reference Mhlanga, Cheesman, Maasdorp, Muoni, Mabasa, Mangosho and Thierfelder2015).
In intercrops, the inter-row spacing is covered by intercropped companion crops. This reduces the light that reaches the weeds and increases the competition for resources which reduces weed proliferation. A global meta-analysis carried out by Gu et al. (Reference Gu, Bastiaans, Anten, Makowski and van der Werf2021) showed that intercrops can reduce weed biomass by 58% as compared to monocrops. Rotating cereals with non-edible or uncommon cover crops such as red sunnhemp (Crotalaria ochroleuca G. Don) or jack bean [Canavalia ensiformis (L.) DC.], respectively, has also been advocated for as a means of weed control and reductions in weed densities (Fig. 5) (Mhlanga et al., Reference Mhlanga, Cheesman, Maasdorp, Muoni, Mabasa, Mangosho and Thierfelder2015).
However, despite the positive effects of rotations, adopting them in CA systems may be limited by landholding size of the smallholder farmers since they would prefer to have cereal crops that have economic value instead of other rotational crops. Thus, a cautious choice of the rotational crop is necessary so that farmers can still benefit from the whole farming system. As for intercrops, the main crop may also suffer competition from the companion crop and end up yielding lower although overall system yields, when all contributions are converted into energy units and nutrition equivalent, may be higher (Madembo, Mhlanga and Thierfelder, Reference Madembo, Mhlanga and Thierfelder2020). Relay cropping, which is the delayed planting of the companion crop until the primary crop has been established or strip cropping, which is growing two or more crop species in defined strips (Kinyua et al., Reference Kinyua, Kihara, Bekunda, Bolo, Mairura, Fischer and Mucheru-Muna2023), may minimize the competition between the primary and companion crop.
The use of microorganisms such as arbuscular mycorrhizal fungi (AMF) as a novel weed control strategy has gained recognition in the past 10 years and more effort is being put into this kind of research. The AMF are mutualistic symbionts that belong to Glomeromycota and form mutual associations with vascular plants and thereby increasing their nutrient uptake (Schüßler, Schwarzott and Walker, Reference Schüßler, Schwarzott and Walker2001; Smith and Read, Reference Smith and Read2010). Despite their positive effects on plants, AMF species such as Glomus intraradices, G. mosseae, and G. claroideum have been shown to reduce the growth of agricultural weeds such as black nightshade (Solanum nigrum L.) and green foxtail [Setaria viridis (L.) P. Beauv.] (Veiga et al., Reference Veiga, Jansa, Frossard and van der Heijden2011). An earlier study also showed that AMF can suppress aggressive agricultural weeds either in the presence or absence of a crop (in this case sunflower) (Rinaudo et al., Reference Rinaudo, Bàrberi, Giovannetti and Van Der Heijden2010). In addition, Fusarium oxysporum isolate Foxy 2, has been shown to control Striga asiatica, a very serious parasitic weed in maize (Elzein and Kroschel, Reference Elzein and Kroschel2004). However, this method still needs to be adapted to the conditions of southern Africa and largely adopted.
Improved agronomic solutions using crop competition and suppression
The manipulation of cropping systems can be a viable option for the control of weeds. For example, the use of crop competition and suppression can be a valuable cultural alternative to the use of herbicides, which involves the shifting of agronomic practices and plant genotypic abilities to suppress weeds or reduce their competition (Mhlanga, Chauhan and Thierfelder, Reference Mhlanga, Chauhan and Thierfelder2016). Crop competition and weed suppression can be increased by increasing plant density or seed rate, reducing crop row spacing, using competitive crop cultivars, and integrating other crops through intercropping. These aspects increase the crop's competition with weeds for resources such as light, moisture, and nutrients. When the crop seed rate is increased, the density of the crops increases, which provides more cover and more light intercepted by the crop and thus reducing the light that reaches the weeds and hence reducing their productivity and competition (Mohammadi, Ghobadi and Sheikheh-Poor, Reference Mohammadi, Ghobadi and Sheikheh-Poor2012). Coupling increased density with other weed control strategies such as hand hoe weeding can have the same effect as herbicide use which means weeds can be controlled in a more environmentally friendly way (Fig. 6a). Weeds, like most plants, need light for photosynthetic activity, and reduced light can reduce their growth and development and their competitive ability (Fig. 6b). Highly competitive weed species such as Cyperus esculentus (L.) and Setaria italia (L.) can be suppressed by increasing the maize population, with positive implications on maize yield (Ghafar and Watson, Reference Ghafar and Watson1983; Stoller, Wax and Slife, Reference Stoller, Wax and Slife1979).
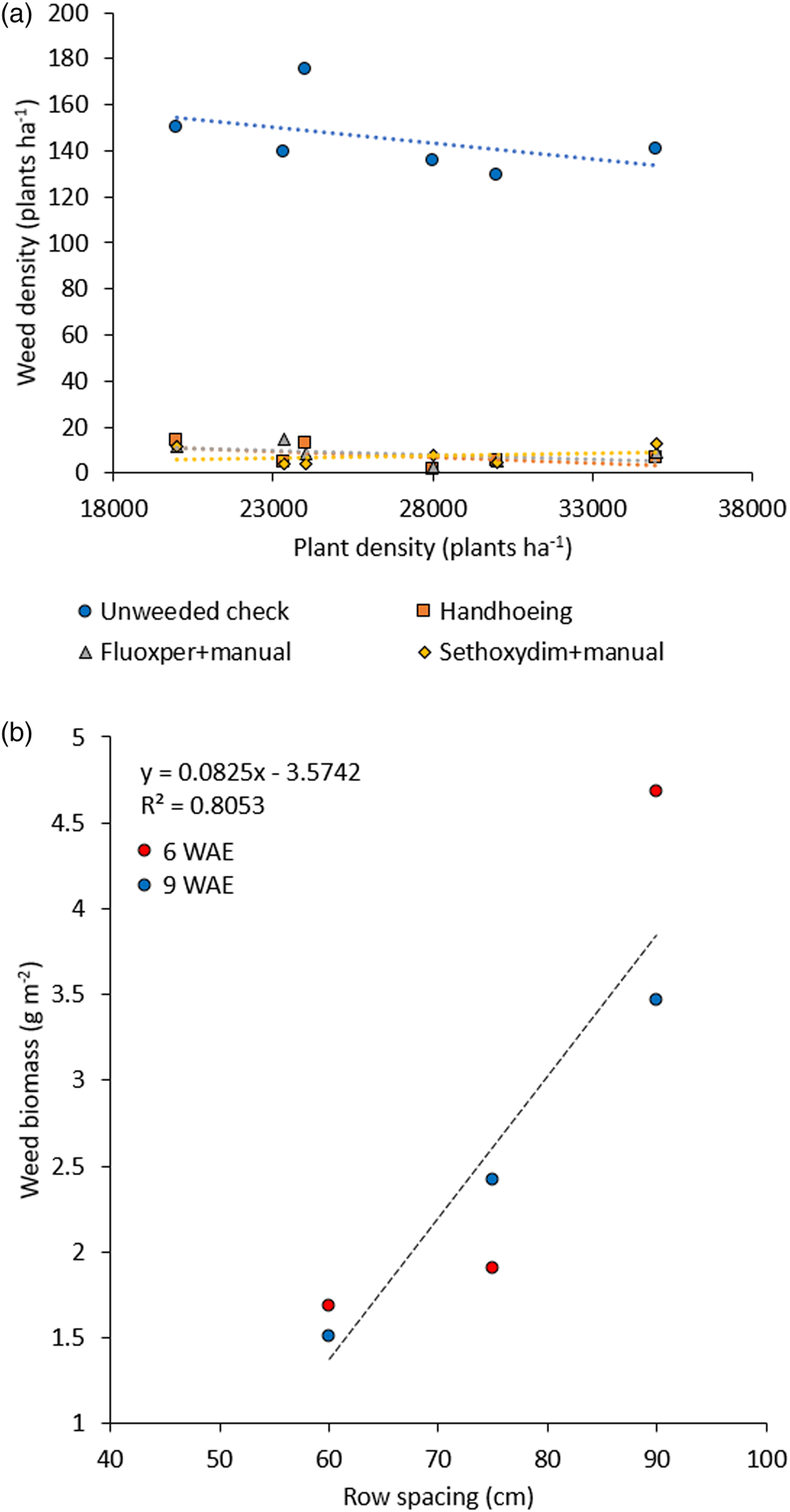
Figure 6. (a) Effect of plant density on weed density; and (b) effect of narrowing maize rows on weed biomass. Adapted from Moswetsi, Fanadzo and Ncube, (Reference Moswetsi, Fanadzo and Ncube2017).
Besides increasing seed rate, narrowing of crop rows can also increase competition for weeds. In soybean, row spacing of 33 cm and 50 cm resulted in 30% less weed biomass than the standard 76 cm row spacing, without any yield penalty (Steve Boahen, personal communication). In other studies, decreasing maize row spacing from 90 cm to 75 cm and to 60 cm reduced weed biomass by 46% and 61%, respectively (Moswetsi, Fanadzo and Ncube, Reference Moswetsi, Fanadzo and Ncube2017) (Fig. 6b). Thus, farmers in southern Africa can adopt this strategy in their cropping systems to control weeds. However, it is highly dependent on the agroecological conditions within which the farmers are located (Williams et al., Reference Williams, Heiniger, Everman and Jordan2014). For example, as mentioned before, increasing crop population means that the intraspecific competition between the crops will also increase and hence this competition needs to be alleviated by ensuring adequate growth resources such as nutrients and moisture. New strategies to alleviate crop competition in CA systems through strip cropping are currently being explored (Kinyua et al., Reference Kinyua, Kihara, Bekunda, Bolo, Mairura, Fischer and Mucheru-Muna2023; Madembo, Mhlanga and Thierfelder, et al., Reference Madembo, Mhlanga and Thierfelder2020), which provides new avenues to manage weeds through agronomic practices. These practices can minimize niches for weed growth.
Since rainfall is highly variable in southern Africa and some farmers are situated on sandy soils of low organic matter, increasing crop population would require moisture conservation techniques and supplementing nutrients in the system either through organic or inorganic sources. Such interventions are possible if mulching is implemented since it gradually improves organic matter if nutrients are supplemented and conserves moisture (Ligowe et al., Reference Ligowe, Nalivata, Njoloma, Makumba and Thierfelder2017; Thierfelder and Wall, Reference Thierfelder and Wall2009). Most of the smallholder farmers in southern Africa cannot afford large amounts of inorganic fertilizers and so their most viable option to supplement nutrients would be through organic materials such as green and brown manuring (Ndambi et al., Reference Ndambi, Pelster, Owino, De Buisonje and Vellinga2019).
Maize breeders may select competitive hybrids with specific traits conducive to increasing competition with weeds. Since crop canopy architecture determines the amount of light that reaches the ground, cultivars that intercept more light are more effective in controlling weeds (Lindquist and Mortensen, Reference Lindquist and Mortensen1999). Such cultivars will also have a high photosynthetic output which means they will outgrow weeds and suppress them more (Huang et al., Reference Huang, Gao, Li, Xu, Tao and Wang2017).
Exploration of the use of allelopathy for weed management
Allelopathy occurs when one plant species releases chemical compounds, either directly or indirectly through microbial decomposition of residues, that affect another plant species (Rice, Reference Rice1984). Some crop cultivars and plants are able to exudate allelochemicals from their roots and these have a negative effect on the growth of weeds (Christensen, Reference Christensen1995; Wu et al., Reference Wu, Pratley, Lemerle and Haig1999), however, this needs to be explored further to commercially exploit it.
The use of allelopathic plants in a crop rotation or as part of an intercropping system may provide a non-herbicide mechanism for weed control. For example, intercropping of sorghum (Sorghum bicolor L.), sesame (Sesamum indicum L.), and soybean (Glycine max L.) in a cotton (Gossypium hirsutum L.) field produced greater net benefits and a significant inhibition on purple nutsedge (Cyperus rotundus L.) in comparison with the cotton alone (Iqbal, Cheema and An, Reference Iqbal, Cheema and An2007).
The allelochemicals from living plants or decomposed straws can suppress weed growth in farmlands. For example, in laboratory bioassays and pot culture experiments, Rugare, Pieterse and Mabasa, (Reference Rugare, Pieterse and Mabasa2020) studied the allelopathic potential of aqueous extracts of green manure cover crops [jack bean (Canavalia ensiformis L.) and velvet bean], on the germination and seedling development of goosegrass (Eleusine indica L.) and blackjack (Bidens pilosa L.) and maize and found that the germination of both weeds was inhibited by these extracts. Soil amended with the green manure of jack bean and velvet bean reduced the emergence and growth of weed seedlings but had little adverse effects on maize. Allelopathic cultivars represent the most promising application of allelopathy. Several crops, such as alfalfa, barley, black mustard, buckwheat, rice, sorghum, sunflower, and wheat, demonstrate strong weed suppression ability, either by exuding allelochemical compounds from living plant parts or from decomposing residues (Tesio and Ferrero, Reference Tesio and Ferrero2010). Plants with allelopathic potential are a more sustainable alternative for weed control and are a means to minimize reliance on herbicides, hence reducing the selection pressure for herbicide resistance.
Designing a push-pull approach for an integrated management of weeds is another avenue to explore (Hassanali et al., Reference Hassanali, Herren, Khan, Pickett and Woodcock2008; Khan et al., Reference Khan, Pickett, Hassanali, Hooper and Midega2008). This entails integrating plants that are antagonistic to weed growth and development and those that promote the growth of other species. For example, if a weed community is dominated by certain species, the introduction of plants with allelopathic effects to the dominant species is likely to assist in reducing the density of the dominant plant leading to a more diverse and less competitive system. More diverse systems can also be achieved by introducing plants that promote the germination of less abundant weeds.
Reducing seed production and the weed seed bank in the soil
The weed seedbank in the soil and annual recruitment are the main sources of weed infestation in crops. The long-term weed management strategy in CA systems should focus on depleting the soil weed seed bank and reducing seed production in each season. Weeds that have survived pre- and post-emergence weed control measures must not be allowed to produce any seed because as an old saying tells us: ‘one year of seeding is seven years of weeding’. Prevention of weed seed production can be accomplished by intercropping the primary crop with smother crops such as sweet potatoes (Ipomoea batatas (L.) Lam.), application of sublethal dosages of herbicides, slashing the weeds, or burning the dried weeds to kill seeds. (Ekeleme et al., Reference Ekeleme, Akobundu, Isichei and Chikoye2003) demonstrated that tropical kudzu [Pueraria phaseoloides (Roxb.) Benth.] fallow was more effective in shading weeds and probably reducing the quantity of light reaching them than the natural bush and planted L. leucocephala fallow systems, and this may have been the basis of the significantly lower seed amounts in P. phaseoloides plots. The planting of self-seeding cover crops can also assist in ensuring continuous ground cover on- and off-season.
Depleting the weed seedbank might be beneficial for cropping but will have negative side effects on ecological diversity and maybe ‘living with some weeds’ could be a compromise between full control and harnessing agro-ecological control mechanisms if some weeds are still present. Most plants classified as weeds in southern African farming systems offer ecosystem, social, and human services, and this further calls for a holistic management of weed communities such that they are less competitive while being beneficial (Blaix et al., Reference Blaix, Moonen, Dostatny, Izquierdo, Le Corff, Morrison, Von Redwitz, Schumacher and Westerman2018). Leaves of weeds such as blackjack [Bidens pilosa L. (Asteraceae)] and pigweeds (Amaranthus spp.) are common vegetables in southern Africa where they are usually served with starches (Norman, Reference Norman1994). The same weeds also have medicinal properties, e.g. the extracts of blackjack have been shown to have anti-inflammatory properties (Mtenga and Ripanda, Reference Mtenga and Ripanda2022). Diverse weed communities can promote crop health and diversity of beneficial arthropods such as bees and natural predators of insect pests (Bretagnolle and Gaba, Reference Bretagnolle and Gaba2015). Weeds can also act as hosts of beneficial obligate microbes such as arbuscular mycorrhizal fungi (AMF) and this is important in southern Africa characterized by dry seasons in which no crops grow (Mhlanga et al., Reference Mhlanga, Ercoli, Thierfelder and Pellegrino2022). Since weeds are more adapted to both the seasonal and off-season conditions, they can thrive through the dry winter periods in which most crops do not thrive and hence providing alternative hosting of AMF.
Research gaps and needs
There is a considerable body of evidence from the agricultural and environmental sciences carried out in southern Africa in the last two decades to suggest that CA-based systems offer promising sustainability and yield dividends, especially when applied in the longer term (Komarek, Thierfelder and Steward, Reference Komarek, Thierfelder and Steward2021; Thierfelder, Matemba-Mutasa and Rusinamhodzi, Reference Thierfelder, Matemba-Mutasa and Rusinamhodzi2015a). It is apparent that there is a strong proof of concept for extension systems and farmers to scale this to larger farming communities and environments. However, this does not suggest that the scientific enquiry into CA is concluded. There are many areas of biophysical research that remain unresolved, and these areas also include AMF, manipulating plant populations and spatial arrangements, and inter-species competition as well as specific cereal–legume rotation sequences.
Additionally, and critically, the social science research into the success of CA as part of a complex (crop-livestock) farming system has lagged the biophysical science. There is a need to enhance the social science research into sustainable intensification of the farming systems of southern Africa with CA as part of the portfolio of paradigm shifts needed to achieve sustainable intensification (Tufa et al., Reference Tufa, Kanyamuka, Alene, Ngoma, Marenya, Thierfelder, Banda and Chikoye2023).
Further research questions relate to the social institutions to support CA mainstreaming and scaling. For example, and for illustration as mentioned before, in communities with strong kinship ties, why is it difficult to come up with a common understanding that unregulated grazing is inimical to the agricultural and economic health of the community in the long run? Why are kinship ties and related norms counterproductive to the modernization of crop-livestock systems in southern Africa? Is this driven by an ‘egalitarian’ approach and prioritization of social harmony over economic efficiency?
Therefore, five areas of social science research merit attention:
-
First, research on institutional innovations to facilitate local community governance and entrenching norms of private property in consonance with modern economic systems.
-
Second, studies on macro (national level) policies may create a broad impetus for economic and social changes and an avenue towards the envisaged paradigm shifts in CA-based or other nature and climate-positive farming systems.
-
Third, there is a need to do more scientific research on behavioral change aspects of farmers and their communities to understand how to use existing social norms for social change towards more private and market-oriented property systems without undermining the social and collective fabric needed for collective action in other areas where that is needed.
-
Fourth, research on the economics of residue use (alternative uses and the economics of N-supplementation to deal with N-immobilization, smallholder in-situ fodder production), long-term soil resource management, improving the extensive nature of the crop/livestock systems, and how to achieve sustainable intensification of such needs to be strengthened.
-
Fifth, how can markets be more effectively used to increase the supply of technical solutions, be it legume species, environmentally benign herbicides, and even mechanical tools for weed control?
Conclusion and way forward
This opinion paper highlights that there are unanswered questions (e.g. how to improve existing crop-livestock systems of southern Africa to become optimal, and how alternative sources of crop residues can be made available instead of using maize stover as residue). There are also unquestioned answers on CA implementation in southern Africa (e.g. ‘herbicides are the only solution for weed control in CA systems’). Related to that we highlight how local norms and institutions continue to perpetuate unsustainable communal resource use.
A range of options have been proposed for both types of questions: Residue trade-offs in crop-livestock systems can be minimized by applying several strategies ranging from increased in situ and ex situ fodder production; controlled and more holistic grazing systems and community agreements and/or enforcements that may overcome unsustainable grazing practices. Besides chemical weed control there are viable biological and mechanical options that need to be further explored and integrated into current smallholder farming systems of southern Africa.
Some additional way-forward principles can be highlighted. First, to implement CA properly (using the most current available scientific information) requires high levels of agronomic knowledge and standards of practice on the part of extension agents and farmers. This places serious responsibility on public extension and research systems (including the CGIAR) to adapt CA systems to local context and to disseminate the existing body of information to key stakeholders (mostly farmers). Second, it will be important to facilitate farmer-research interaction using the existing knowledge and continue to engage decision-makers. Finally, there is a need to launch a new generation of research agenda that tackles the unanswered questions and unquestioned answers.
This new research agenda should focus on the social and institutional innovations needed to mainstream CA as well as strengthen and expand the research on agronomic weed management alternatives and finally focus on the science of communal grazing land management to enhance their productivity. Research on alternative biomass production and where this is technically and economically feasible is warranted. In short, many of the crop residue and weed challenges are bigger than weeds and crop residues themselves and appear to be symptoms of the broader systemic complications that cannot be resolved without understanding the interactions between the current scientific recommendations, private incentives, social norms, institutions, and government policy.
Data availability statement
No applicable.
Author contributions
CT elaborated the idea wrote the first draft, revised the manuscript several times and finalized the manuscript; BM supported drafting of narrative, analyzed some of the data, and reviewed the manuscript several times; HN provided socio-economic insights, contributed to the narrative, and reviewed the manuscript; PM, AM, AA, and AT provided useful comments and contributed to the narrative; DC provided the first idea of the paper title and contributed to the narrative.
Funding statement
This work was financially supported by the project ‘Understanding and Enhancing Adoption of Conservation Agriculture in Smallholder Farming Systems of Southern Africa (ACASA)’ which was funded by the Royal Norwegian Government through NORAD (grant P1925). Financial and logistical support was further received by the USAID-funded Africa Research in Sustainable Intensification for the next Generation (Africa RISING) project (AID-BFS-G-11–00002) and the Ukama/Ustawi Regional CGIAR Initiative Diversification for Eastern and Southern Africa.
Competing interests
The author declare no conflicts or competing interests of any sorts in publishing this article.
Ethics approval/declarations
The regional survey was ethically cleared by the Internal Research Board of the International Institute of Tropical Agriculture (dated 10.12.2020) and the National Ethics Boards of Zambia (Ref. No. 2021-Jan-21) and Malawi (NCST/RTT/2/6). For Zimbabwe, no clearance was required. Waivers can be provided upon request.
Consent to participate
All participants who were interviewed during surveys routinely sign a consent form before any questions were asked.
Consent for publication
Not applicable.
Code availability
Not applicable.