Introduction
The potential to store insects for prolonged durations at low temperatures could be beneficial for use in mass rearing of biological control agents (Leopold, Reference Leopold, Hallman and Denlinger1998; Colinet and Boivin, Reference Colinet and Boivin2011; Filho et al., Reference Filho, Leite, Soares, Alvarenga, Paulo, Santos and Zanuncio2014). Long-term storage could supplement, or even replace, expensive continuous rearing practices currently being used in mass rearing facilities (Cagnotti et al., Reference Cagnotti, Lois, Silvia, Botto and Viscarret2018). The ability to store insects could open new opportunities for producers of biological control agents to stockpile insects when levels of production are higher than levels of demand, and then deliver these insects quickly when demand increases (Siam et al., Reference Siam, Zohdy, ELHafez, Moursy and Sherif2019). The two basic strategies for low-temperature storage of insects are (1) the cryopreservation of embryos at cryogenic temperatures, most often in liquid nitrogen at −196°C, and (2) long-term storage at temperatures below the threshold for development, which is typically applicable for insects in diapause but can also be used for insects induced into other types of deep states of dormancy (Leopold, Reference Leopold, Vreysen, Robinson and Hendrichs2007; Denlinger, Reference Denlinger2008). However, prolonged low-temperature storage may result in developmental failures, depletion of energy substrates, loss of metabolite homeostasis, and oxidative damage as potential mechanisms responsible for accumulation of indirect chill injury in insects (Colinet et al., Reference Colinet, Nguyen, Cloutier, Michaud and Hance2007, Hahn and Denlinger, Reference Hahn and Denlinger2007). Methods must be developed to understand and mitigate the stresses of long durations of storage at temperatures below the developmental threshold.
Insects often face harsh environmental factors during their life cycle that must be endured to complete their development and reproduction. Diapause, a programmed state of dormancy, is the principal mechanism by which insects survive non-favorable seasonal conditions in their environment (Koštál, Reference Koštál2006). Diapause takes place in the life cycle of most stored-product Lepidoptera (Bell, Reference Bell1994), and thus may be of use in developing protocols for biological control in stored-product systems. Specifically, for programs wishing to implement biological control of stored-product pests, the ability to keep hosts in a dormant state may be advantageous for the production of parasitoids for augmentative biological control in commodity storage facilities.
Using dormant hosts to rear parasitoids for biological control programs may be advantageous because dormancy may change host physiology in ways that are favorable for parasitoid production (Hallman and Denlinger, Reference Hallman and Denlinger1999; Sanowar et al., Reference Sanower, Mbata and Payton2018). For example, diapause programming is often associated with increases in metabolic reserves of lipids, carbohydrates, and proteins that can be used by the insect to sustain themselves through a long, dormant period (Hahn and Denlinger, Reference Hahn and Denlinger2007; Yocum et al., Reference Yocum, Buckner and Fatland2011; Sinclair, Reference Sinclair2015). Lipids are the primary source of metabolic reserves that most insects use during diapause (Danks, Reference Danks1987; Hahn and Denlinger, Reference Hahn and Denlinger2007, Reference Hahn and Denlinger2011). It has been reported that lipid reserves provide efficient storage of energy and their metabolism can create metabolic water, which may be particularly advantageous in dry environments, like stored grains (Wharton, Reference Wharton, Gilbert, Iatrou and Gill1985; Danks, Reference Danks2000). Similarly, diapause and other forms of environmentally induced dormancy (i.e., thermal quiescence) can alter other aspects of host metabolism besides lipid storage and composition, including changes in protein and amino acid contents or blood and tissue carbohydrate content that can be advantageous for parasitoid production (Hahn and Denlinger, Reference Hahn and Denlinger2007, Reference Hahn and Denlinger2011). Furthermore, inducing diapause or other forms of dormancy with low temperatures may have effects on the host immune system that could make them more favorable for successful parasitoid development. For example, Ferguson et al. (Reference Ferguson, Heinrichs and Sinclair2016) reported that cold acclimation decreased realized immunity at low temperatures. Thus, inducing dormancy may have extended benefits for parasitoid production due to host immune suppression.
The Indian meal moth, Plodia interpunctella (Hübner) (Lepidoptera: Pyralidae), is a cosmopolitan pest of warm-temperate or sub-tropical origin that can now be found on every continent excluding Antarctica (Howe, Reference Howe1965; Bell, Reference Bell1975; Mohandass et al., Reference Mohandass, Arthur, Zhu and Throne2007). Plodia interpunctella is a severe pest of stored food products, including grains and grain-based products, nuts, and fruits (Hamlin et al., Reference Hamlin, Reed and Phillips1931; Mohandass et al., Reference Mohandass, Arthur, Zhu and Throne2007). Aside from direct product loss through feeding, P. interpunctella also causes economic losses from costs of control, quality reduction, and consumer complaints (Phillips and Throne, Reference Phillips and Throne2010). Many populations of P. interpunctella facultatively enter diapause in the last (fifth) larval instar in response to photoperiod and/or temperatures (~20°C or lower), although some populations have either lost or evolved low incidences of diapause (Tzanakakis, Reference Tzanakakis1959; Masaki and Kikukawa, Reference Masaki, Kikukawa, Folett and Folett1981; Kikukawa and Masaki, Reference Kikukawa and Masaki1984; Bell, Reference Bell1994). Diapause is a topic of particular interest in stored-product settings because diapausing P. interpunctella have been found to be more difficult to control when using fumigants such as phosphine, and in modified-atmosphere packaging (Adler, Reference Alder2001; Gourgouta et al., Reference Gourgouta, Agrafioti and Athanassiou2021). The mechanistic basis for diapause or other forms of dormancy reducing the efficacy of fumigants like phosphine in stored-product pests is currently unknown. However, insects that have become dormant either through programmed diapause or environmental factors, like low temperature or low humidity, also frequently have both lower respiration rates that could limit the entrance of gaseous fumigants into the insect's body, and increased expression of a number of stress hardiness mechanisms such as antioxidants that could help reduce intracellular damage due to off-target effects of pesticide metabolism by mixed-function oxidases (Denlinger, Reference Denlinger2002; Hahn and Denlinger, Reference Hahn and Denlinger2011; Sahoo et al., Reference Sahoo, Smith, Perrone-Bizzozero and Twiss2018; Moreira et al., Reference Moreira, Pereira, Mench, Garbisu, Kidd and Castro2021).
One of the most promising and effective biocontrol agents for P. interpunctella in stored-product settings is the Braconid wasp, Habrobracon hebetor (Say) (Hymenoptera: Braconidae), a cosmopolitan, gregarious, and koinobiont ectoparasitoid of a wide range of lepidopteran species (Ghimire and Phillips, Reference Ghimire and Phillips2010; Liu et al., Reference Liu, Liu, Sun, Ma, Jiang and Chen2015; Glupov and Kryukova, Reference Glupov and Kryukova2016; Hasan et al., Reference Hasan, Hasan, Khatun, Hossain, Athanassiou and Bari2020). Habrobracon hebetor also has the potential to be integrated with other biological control agents for the management of pest moth populations (Mbata and Shapiro-Ilan, Reference Mbata and Shapiro-Ilan2005, Reference Mbata and Shapiro-Ilan2010). A major challenge in mass rearing H. hebetor derives from the fact that the parasitoid has a narrow window during host development in which it can successfully parasitize their hosts, which are late instar Pyralidae caterpillars that pupate within few days under optimum conditions (Akinkurolere et al., Reference Akinkurolere, Boyer, Chen and Zhang2009). Efficient mass rearing is one of the prerequisite criteria to be taken into consideration for an augmentative biological control program. A mass rearing protocol for H. hebetor has not yet been established. Rearing of H. hebetor on diapausing host larvae could potentially produce higher numbers of progeny because diapausing host larvae develop very slowly, thus providing a broader window of time for parasitism (Na and Ryoo, Reference Na and Ryoo2000; Sanower et al., Reference Sanower, Mbata and Payton2018). Dormant host larvae can survive for long periods, and once in a state of dormancy, produce less silk than non-dormant larvae further facilitating parasitoid rearing (Williams, Reference Williams1964; Bell, Reference Bell1977; Bell et al., Reference Bell, Bowley, Cogan and Sharma1979; Mbata, Reference Mbata1987; Mohandass et al., Reference Mohandass, Arthur, Zhu and Throne2007). Other characteristics of dormant larvae of P. interpunctella that could potentially enhance progeny production by H. hebetor include alterations in lipid, carbohydrate, and protein metabolism induced by dormancy that may favor parasitoid development, as well as dormancy and cold-induced reductions in host immunity that may favor parasitoid production (Ferguson et al., Reference Ferguson, Kortet and Sinclair2018). Our overarching hypothesis for this study is that storage of P. interpunctella hosts in dormancy for short periods of time would benefit parasitoid production while having little negative effects on host parameters, but that longer term storage would eventually lead to a decline in host quality and subsequently parasitoid production and quality. This investigation had two major objectives. First, we tested the extent to which storing dormant P. interpunctella larvae at 15°C for a variety of durations would affect the ability of larvae to successfully molt to adulthood and subsequent adult reproductive parameters. The ability to keep P. interpunctella larvae in dormancy for prolonged periods could both benefit rearing of parasitoids on those hosts and improve the maintenance of the host colony itself by allowing the host colony to be put in dormancy when parasitoid rearing is not necessary to suit demand. Second, we tested the extent to which rearing H. hebetor on P. interpunctella host larvae that had been held in dormancy for various periods affected parasitoid development.
Materials and methods
Host rearing
The Indian meal moth, P. interpunctella, colony used in the current study was originally collected from local food facilities in 2014 and has been continuously cultured at the Post Harvest Laboratory, Department of Zoology, Rajshahi University, Bangladesh. Moths were reared in 1 liter glass jars on a mixed standardized larval diet (350 g) of corn meal, chick laying mash, chick starter mash, and glycerol (Phillips and Strand, Reference Phillips and Strand1994) at a volumetric ratio of 4:2:2:1, respectively. Cultures were maintained in an incubator (Sanyo MIR-553, South Korea) set at 27 ± 0.5°C, 70 ± 5% relative humidity (RH), with a photoperiod of 16:8 (L:D) h, conditions that clearly maintained non-diapause development.
Parasitoid origin and rearing
Habrobracon hebetor adults were obtained from the Bangladesh Agriculture Research Institute (BARI), Gazipur, Bangladesh in 2014. The parasitoids were cultured and mass-reared on last instar (5th instar) larvae of P. interpunctella in the laboratory at 27 ± 1°C, 70 ± 5% RH, and photoperiod of 16:8 (L:D) h (Mbata and Shapiro-Ilan, Reference Mbata and Shapiro-Ilan2010).
Larval dormancy induction in P. interpunctella
To induce larval dormancy, we shifted larvae from warmer, long-day photoperiodic conditions to cooler, short-day photoperiodic conditions. Specifically, 14-day-old (5th instar) P. interpunctella larvae were transferred from one climate chamber set at 27°C 16:8 (L:D) to another climatic chamber set at 20°C 12:12 (L:D) for one day to provide a brief acclimation period to cooler temperatures, and then the following day larvae were transferred to 15°C and 12L:12D photoperiod to induce dormancy (fig. 1). Throughout this manuscript, we refer to larvae as being dormant rather than as in diapause because while diapause is induced in many P. interpunctella strains (Bell, Reference Bell1976; Wijayaratne and Fields, Reference Wijayarantne and Fields2012) we changed both photoperiod and temperature between our non-dormant and dormant animals and thus cannot distinguish the contributions of programmed diapause vs. thermal dormancy due to exposure to 15°C over the long periods of delayed development observed in our study. Dormant larvae were experimentally kept at 15oC individually in plastic rearing trays (L × W × H: 9.6″ × 4.1″ × 2.0″) (HL-B025, Jiangsu, China) containing 50 small holes (2 ml) filled with food medium (6 g) for one of seven durations: 15, 30, 45, 60, 75, 90, or 105 days (Tzanakakis, Reference Tzanakakis1959; Mohandass et al., Reference Mohandass, Arthur, Zhu and Throne2007), with all treatments and replicates run concurrently. Trays were covered with a transparent plastic sheet with tiny holes to allow exchange of air. The development of larvae was observed every day during different storage periods. If a larva did not pupate during the exposure period at 15°C, the larva was considered to be dormant. Furthermore, some moths emerged early during the induced dormancy period. These early emerging moths were considered to be non-dormant, and 5 days after the last individual emerged from the first clear bout of early emergence, other larvae in the tray that were still clearly in the larval stage showing no sign of metamorphosis into pupae or adults were considered dormant larvae.
The number of pupae and adults per tray was recorded separately for each experiment. The percentage of larvae that successfully survived dormancy and emerged as adults was also recorded. The transition from the dormant larval stage to reinitiate development was made by gradually increasing temperature to avoid possible thermal shock. First, the temperature was increased to 18°C for one day and then increased again on the second day to 23°C, both with a photophase of 14:10 (L:D) and on the third day insects were transitioned to 27°C, RH 70 ± 5%, and a photophase of 16:8 (L:D). Plastic pots (500 ml) containing non-dormant larvae were kept in an incubator set at 27°C, RH 70 ± 5%, and a photophase of 16 h throughout as a control group for comparison. Three replicates were performed, each having 200 larvae in each condition. For this experiment, 18-day-old non-dormant last-instar larvae and dormant larvae stored for different periods of time were used for comparison.
Biology of P. interpunctella developing from dormant larvae
Three replicates of 25 dormant larvae from each storage period and 25 non-dormant larvae of P. interpunctella were placed separately in plastic jars (500 ml) containing 100 g of standard food (Phillips and Strand, Reference Phillips and Strand1994) and allowed to complete development. Jars were kept in an incubator set at 27 ± 0.5°C, 70 ± 5% RH, and 16:8 (L:D). Larvae were weighed at the end of the dormancy holding period to test whether the duration of dormancy had an effect on mass loss. The time from removal from larval dormancy conditions to pupation, the time to adult emergence, and the percent of dormant larvae that yielded emerged adults were recorded for each dormancy duration treatment. The sex of each emerging moth was recorded to test whether the duration of dormancy had an effect on the sex ratio of moths produced, and thus indicated any sex-specific mortality. Five pairs (one male and one female) of newly emerged adults resulting from each duration of dormancy treatment were kept separately in a small plastic container (100 ml) for mating and egg laying. Eggs were counted for each pair in each treatment (fecundity) and kept separately in a plastic petri dish (100 × 20 mm) to record the proportion that hatched (fertility). To test whether the duration of larval dormancy had an effect on host biochemical composition, the total protein content of different dormant and non-dormant host larvae was measured according to Kjeldal method (Jonas-Levi and Martinez, Reference Jonas-Levi and Martinez2017). Percent nitrogen as estimated by the Kjeldal procedure was transformed into protein content by multiplying with a conversion factor of 5.3 (McCarthy and Meredith, Reference McCarthy and Meredith1988; Korel and Balaban, Reference Korel and Balaban2006). Three replicates of pooled larvae (244–672 total larvae per treatment) were sampled for control and each dormancy duration.
Effects of host dormancy history on H. hebetor
To test the extent to which host dormancy duration affects the performance of H. hebetor progeny, ten dormant and ten non-dormant host larvae were placed separately in 500 ml rearing jars containing a pair of newly emerged virgin, naive H. hebetor (one male and one female). Jars were covered with black cloth to encourage wasp mating. Wasps paralyzed host larvae and laid eggs. Experiments were conducted in an incubator maintained at 27 ± 0.5°C, 70 ± 5% RH, and 16:8 (L:D) until the emergence of parasitoid progeny. The number of parasitized host dormant larvae was recorded in each jar. The total number of parasitoid progeny, larval and pupal periods, sex ratios, and body size of male and female adult parasitoids were recorded. Body size measurements (mm) of the head length, total body length from head to tip of abdomen, and wing length of each individual parasitoid were measured using an eyepiece-micrometer (New York Microscope Company, Hicksville, NY, USA). For longevity studies, three pairs of adults of both sexes developing from dormant and non-dormant larvae were kept separately in a plastic container (100 ml) and checked daily until all adults died. Three replicates were conducted for each duration of larval dormancy.
Statistical analysis
Statistical analyses were performed using R software (v.4.0.2). Analysis of variance (ANOVA) procedures were used to determine the effects of storage duration on growth and development of P. interpunctella, as well as on H. hebetor reared on hosts stored at 15°C for different durations. All metrics that were subjected to ANOVA were verified to meet the assumptions of homoscedasticity through the use of Levene's tests. When the assumptions of homoscedasticity were not met due to unequal variances among groups, we used generalized linear models that are robust to departures from homoscedasticity. A linear model was used to estimate the relationship between P. interpunctella pupation duration as storage period at 15°C increased. Means within any of the tests were separated in comparison to the un-stored control using Duncan's new multiple range test (P < 0.05).
Results
Effects of storage on Plodia interpunctella survival and reproduction
Storage at 15°C for any duration of time significantly reduced average larval weight compared to larvae that were not stored (F 7,16 = 137.9, P < 0.001, fig. 2). Although some average weights were statistically significantly different among stored groups, there was no clear pattern with regard to duration of storage (fig. 2). Storage duration significantly impacted the time to pupation after removal of dormant larvae from storage, with larvae stored for 105 days taking significantly more time to begin pupation than any groups stored for less time, 15–90 days (F 6,14 = 56.4, P < 0.001, fig. 3). Duration of the pupal stage was significantly impacted by larval storage duration (F 6,16 = 160.57, P < 0.001), with pupal duration negatively correlated with time stored (R 2 = 0.70, fig. 4). Interestingly, larvae stored for 105 days pupated as quickly as the control group (t = −1.0, P = 0.42). The percent adult emergence was not significantly impacted by storage at 15°C for any of the storage durations in this study (F 7,16 = 1.53, P = 0.23). Similarly, storage duration had no significant impact on the sex ratio of moths (F 7,16 = 2.18, P = 0.09), with an average of 2.5 females per male across all groups. Storage duration also had no effect on moth fecundity (F 1,30 = 0.08, P = 0.779), nor on fertility (F 1,30 = 0.45, P = 0.508), with an average of 202.5 eggs laid by mated females and 43.8% of eggs hatching across all groups. The percent of total protein in the bodies of larval P. interpunctella differed significantly (F 7,16 = 55.92, P < 0.001) among some storage duration groups, but there was no clear pattern with regard to duration of storage in dormancy (fig. 5).
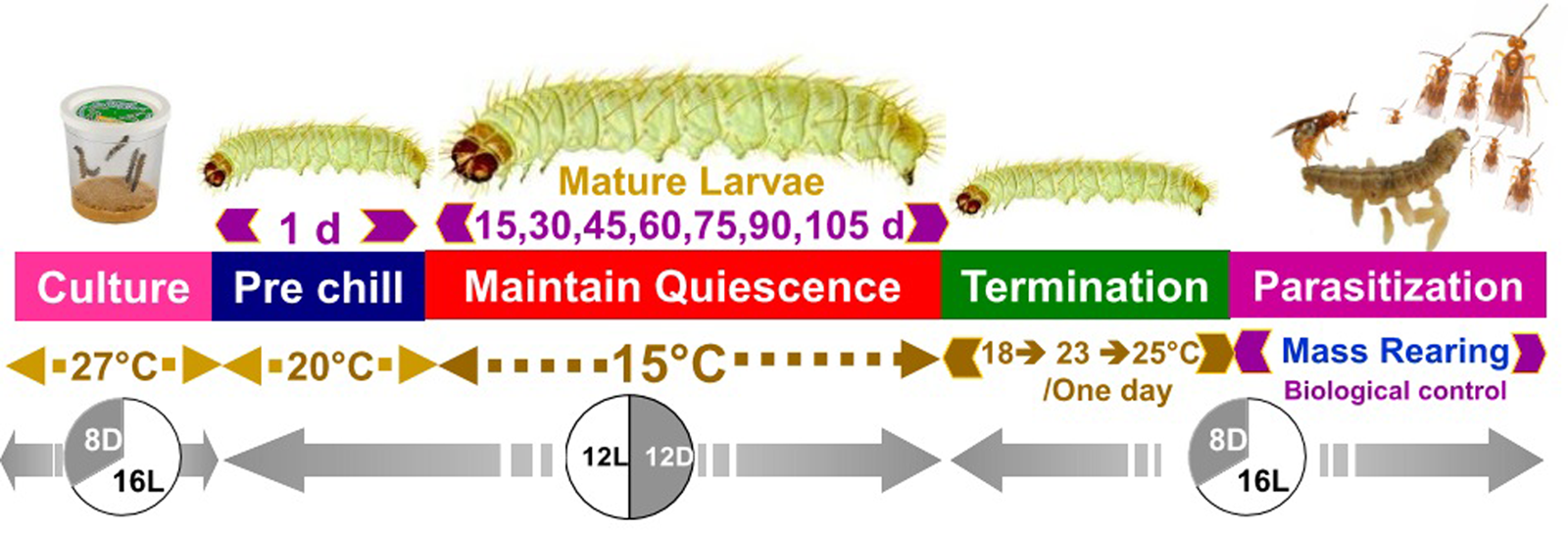
Figure 1. Schematic experimental procedures for our larval dormancy treatments in P. interpunctella and potential for implementation for biological control mass rearing.
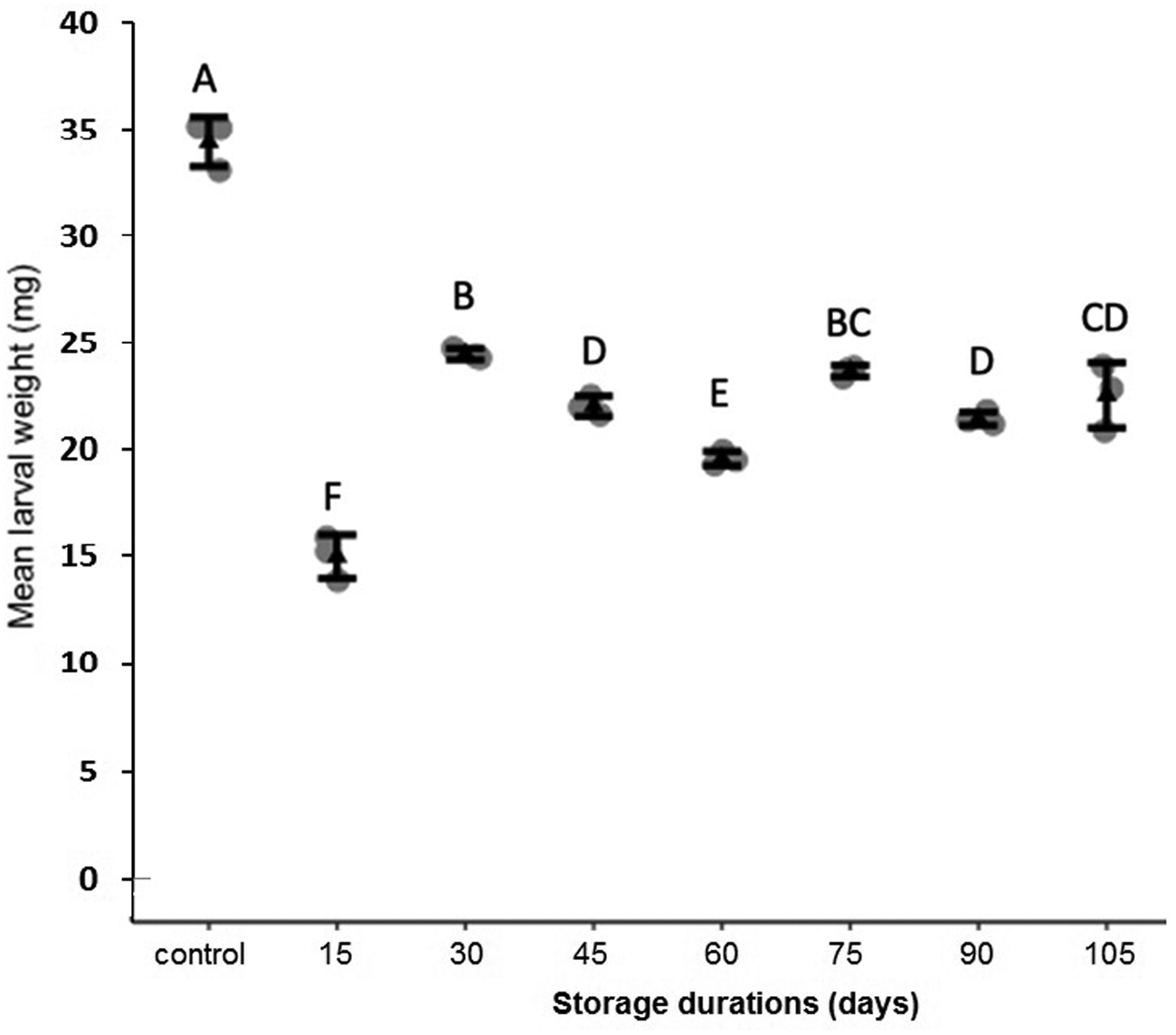
Figure 2. Mean (±SE) weights of P. interpunctella larvae stored at 15°C for a range of exposure periods. Distinct letters for each storage duration indicate statistically significant differences after correction for multiple comparisons with Duncan's multiple-range test.
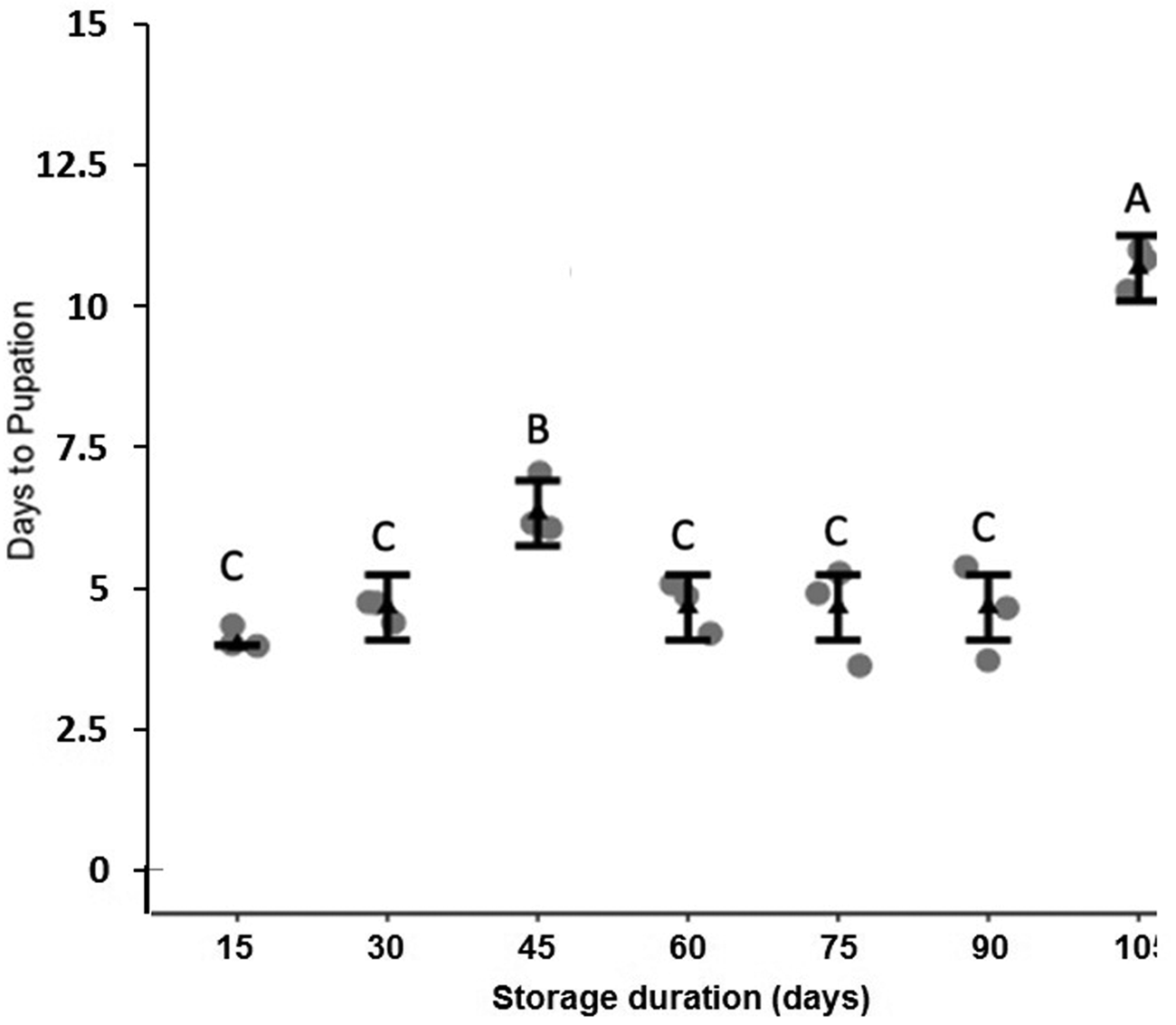
Figure 3. Mean (±SE) time for dormant larvae of P. interpunctella to pupate when exposed to different durations at 15°C. Distinct letters for each storage duration indicate statistically significant differences after correction for multiple comparisons with Duncan's multiple-range test.
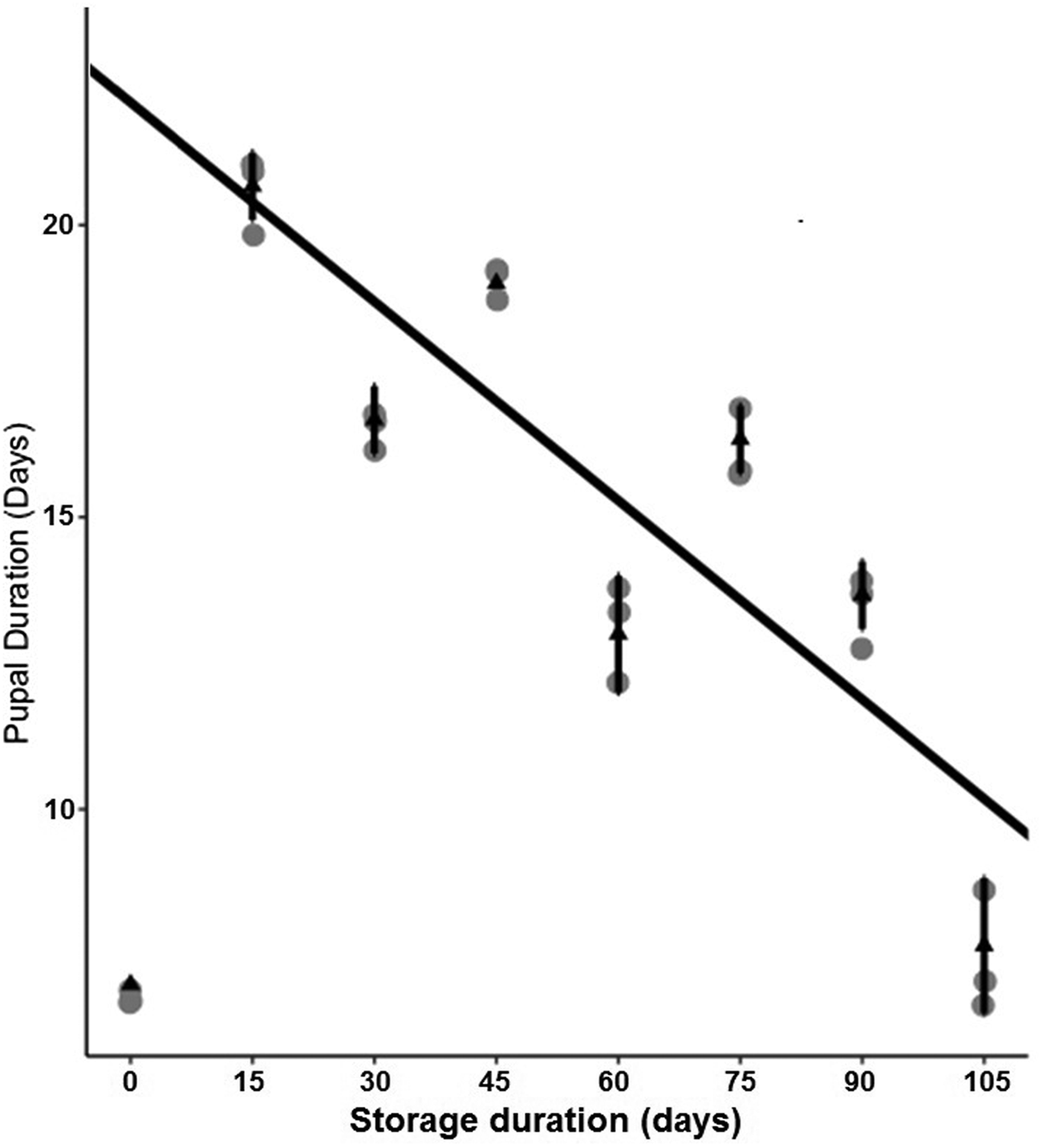
Figure 4. Mean (±SE) duration of P. interpunctella pupal periods that developed from larvae exposed to different durations of storage at 15°C.
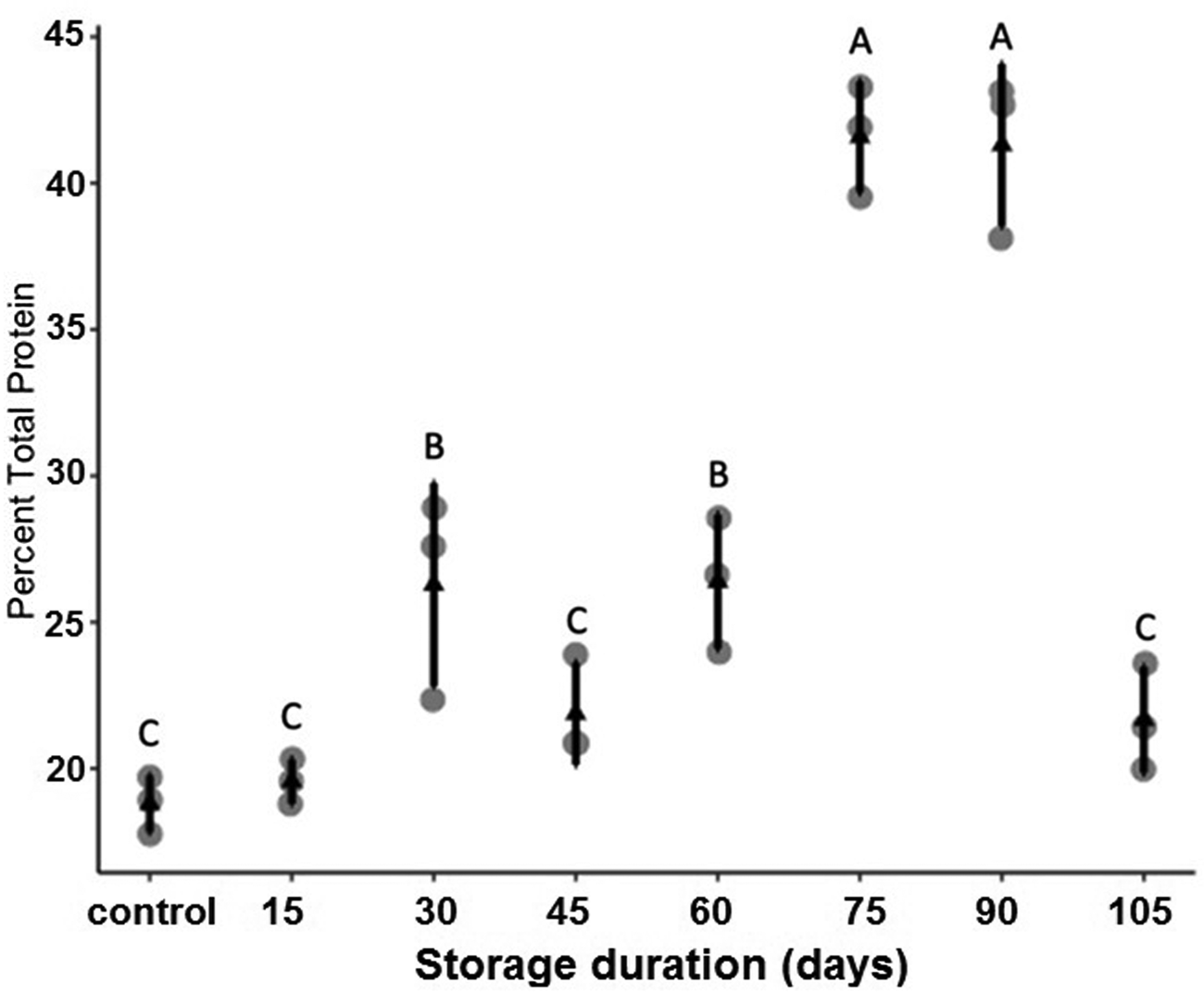
Figure 5. Mean (±SE) percent total protein content of P. interpunctella larvae exposed to different durations of storage at 15°C. Distinct letters for each storage duration indicate statistically significant differences after correction for multiple comparisons with Duncan's multiple-range test.
Effects of host storage on Habrobracon hebetor
There were no significant differences in parasitism percentages across hosts stored for different durations (F 7,16 = 1.90, P = 0.14), with an average of 82.0% hosts parasitized (fig. 6). Host storage in dormancy at 15°C for any duration significantly increased the number of parasitoids per host compared to hosts that did not undergo storage (F 7,16 = 11.57, P < 0.001, fig. 7). Percent parasitoid pupal formation (F 7,16 = 2.31, P = 0.080) and adult emergence (F 7,16 = 1.59, P = 0.209) were not impacted by the duration of host storage in dormancy. Parasitoid larval development was significantly longer by ~2 days in hosts that were stored at 15°C for any duration compared to the control (F 7,16 = 11.29, P < 0.001, fig. 8). There was no impact of host storage duration on parasitoid sex ratio (F 7,16 = 1.59, P = 0.21), with an average of 0.52 females per male across all host dormancy duration groups. With respect to effects of host storage on parasitoid size, there was no effect of host dormancy duration on any of the three traits. However, females had significantly larger head lengths and wing lengths, with the sex effect on body length only marginally significant (two-way ANOVAs, head length: host dormancy duration F 7,70 = 0.01, P = 0.99, sex F 1,70 = 32.39, P < 0.001, wing length: host dormancy duration F 7,70 = 0.21, P = 0.65, sex F 1,70 = 10.49, P = 0.002, body length: host dormancy duration F 7,70 = 0.10, P = 0.74, sex F 1,70 = 3.3, P = 0.074).

Figure 6. Mean percent (±SE) of P. interpunctella parasitized by H. hebetor after exposure to different durations of storage at 15°C.

Figure 7. Mean (±SE) number of H. hebetor produced per infected P. interpunctella larva for each storage duration treatment at 15°C. Distinct letters for each storage duration indicate statistically significant differences after correction for multiple comparisons with Duncan's multiple-range test.
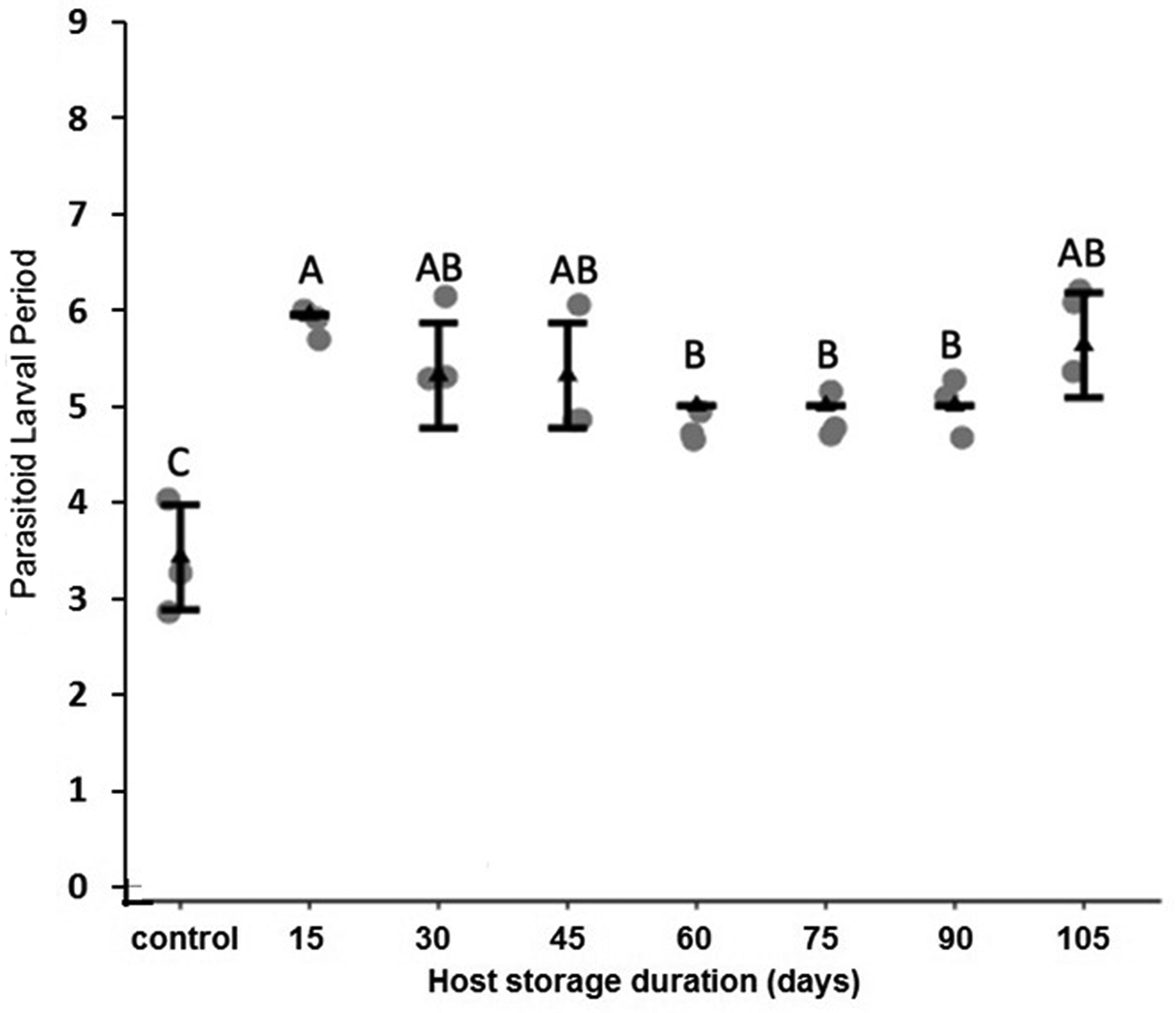
Figure 8. Mean (±SE) duration of H. hebetor larval periods when larvae were reared on the dormant or non-dormant P. interpunctella host larvae. Different letters indicate statistically significant differences after correction for multiple comparisons with Duncan's multiple-range test.
Discussion
Performance of dormant P. interpunctella larvae was surprisingly resilient to storage in dormancy at 15°C for prolonged durations. Despite the fact that all groups held in dormancy had less mass than non-dormant control larvae, all P. interpunctella stored at 15°C survived to adulthood at similar proportions and maintained reproductive potential not different from control moths that were never put into dormancy. Dormant insects, either in diapause or cold storage, typically lose substantial mass as the dormancy period increases due to expenditure of nutrient reserves (Hahn and Denlinger, Reference Hahn and Denlinger2007). Prolonged durations in dormancy conditions have often been found to increase mortality and decrease a number of life-history traits from lifespan and fat reserves to fertility and fecundity, particularly in females of some species (Ellers and Van Alphen, Reference Ellers and Van Alphen2002; Williams et al., Reference Williams, Shorthouse and Lee2003; Munyiri et al., Reference Munyiri, Shintani and Ishikawa2004; Matsuo, Reference Matsuo2006; Hahn and Denlinger, Reference Hahn and Denlinger2011; Margus and Lindström, Reference Margus and Lindström2020). Thus, in our study, we expected to find that hosts held longer periods of time were less suitable than those held for only short durations in dormancy. In our study, P. interpunctella larvae do have less total mass after dormancy than larvae that did not undergo dormancy (control larvae), but there appears to be no major loss of host quality for either parameters important to mass rearing of hosts or parasitoid rearing and production with the time hosts spent in dormant conditions from 15 days to over 100 days. Some insects are capable of severely suppressing their metabolic rates to limit loss of resources over time (Pullin, Reference Pullin1996; Hahn and Denlinger, Reference Hahn and Denlinger2011). The initial decrease in wet mass between control larvae and larvae stored for 15 days may be indicative of a lag between being placed in dormancy conditions and the larvae initiating a reduction in metabolism (Sinclair, Reference Sinclair2015), after which depletion of stores may be very slow. Interestingly, the lowest weights were observed in the group of larvae held only 15 days and larvae held in longer durations of storage were all intermediate between the heavy weights seen in control animals and the lightest weights seen at 15 days. One possible explanation for this unexpected pattern is that the differences in weights observed among groups held dormant for different periods of time are reflective more of body water content than dry mass differences. While we do not know whether dormant P. interpunctella larvae are capable of taking up water from their environment, we do know that other diapausing insects are capable of gaining body water from water vapor in the air around them (Yoder and Denlinger, Reference Yoder and Denlinger1991; Danks, Reference Danks2000; Benoit et al., Reference Benoit, Zhang, Jennings, Rosendale and Denlinger2015; Doherty et al., Reference Doherty, Guay and Cloutier2017). Given that P. interpunctella has evolved to live in relatively dry conditions found in stored grains (Bell, Reference Bell1975; Mbata, Reference Mbata1987), it seems possible that dormant individuals may be able to gain body water content from water vapor in the air, but rigorous testing of this idea will require substantial further work.
Perhaps our most important finding is that P. interpuntella larvae emerging from dormancy served as better hosts for H. hebetor parasitoids than moths that had not undergone any dormancy, at least based on the parameters tested so far. Hosts exiting dormancy produced more parasitoids with no impacts on parasitoid size, whether hosts were held dormant for 15 or 105 days. While others have previously shown that dormant P. interpuntella hosts produce more H. hebetor (Sanower et al., Reference Sanower, Mbata and Payton2018), our work stands out as a novel contribution because we have shown that this pattern of dormant hosts being better for parasitoid production is not just true for hosts early in dormancy, but that hosts can be stored for more than 3 months and still provide improved parasitoid yields. Body size is an important correlate of parasitoid fitness in general and a very important trait for biological control agents because size affects flight ability, parasitism efficiency, longevity, and female fecundity and thus efficacy of the control agent (Visser, Reference Visser1994; West et al., Reference West, Flanagan and Godfray1996; Ellers and Jervis, Reference Ellers and Jevis2003; Gao et al., Reference Gao, Tang, Wei, Wang, Yang and Zhang2016). We had expected parasitoid body size might decline with extended dormancy of hosts, but we found no effect of host storage duration on parasitoid body size in this study. We hope that these results combined with several other studies that have shown improved performance of parasitoids on dormant hosts (e.g., Leopold, Reference Leopold, Hallman and Denlinger1998; Colinet and Boivin, Reference Colinet and Boivin2011; Filho et al., Reference Filho, Leite, Soares, Alvarenga, Paulo, Santos and Zanuncio2014; Sanower et al., Reference Sanower, Mbata and Payton2018) will encourage mass rearing programs for biological control agents, like H. hebetor, to incorporate host dormancy into their workflows.
In our study, we do not know precisely why hosts that experienced dormancy allowed for greater parasitoid production, but several broad possibilities seem likely. One possibility is that female H. hebetor laid more eggs per host larva when the host larva was in dormancy than were laid in non-dormant hosts. There are many factors, from host density to parasitoid density to host quality and more, that affect both how many larvae are laid in each host and downstream parasitoid larval performance (Harvey et al., Reference Harvey, Harvey and Thompson1995; Glupov and Kryukova, Reference Glupov and Kryukova2016). Another non-mutually exclusive possibility for the improvement in parasitoid production from dormant hosts we observed is that dormant hosts could have increased nutritional quality, an important feature for this gregarious parasitoid species. Many insects have been documented to increase lipid reserves prior to or during dormancy (Lefevere et al., Reference Lefevere, Koopmanschap and de Kort1989; Joanisse and Storey, Reference Joanisse and Storey1996; Atapouret al., Reference Atapour, Moharramipour and Barzegar2007; Rozsypalet al., Reference Rozsypal, Koštál, Berková, Zahradníčková and Šimek2014, Sinclair and Marshall, Reference Sinclair and Marshall2018). Exposure to lower temperatures has also been found to increase fat body protein content while maintaining high lipid content in other tropical insects (Chowanski et al., Reference Chowanski, Lubawy, Spochacz, Ewelina, Grzegorz, Rosinski and Slocinska2015). Sanower et al. (Reference Sanower, Mbata and Payton2018) also found increased H. hebetor production in dormant P. interpunctella. These authors proposed that the extended duration of the 5th larval instar of P. interpuntella (the stage that adult H. hebetor attack) combined with an increase in nutritional quality made dormant larvae better hosts, although Sanower et al. (Reference Sanower, Mbata and Payton2018) did not directly measure any facets of host nutritional quality. The reduction in weight observed in dormant larvae relative to non-dormant in this study may simply be due to dehydration that many insects undergo during dormancy(Wharton, Reference Wharton, Gilbert, Iatrou and Gill1985; Danks, Reference Danks2000), but some of the weight loss may be due to depletion of host nutrient reserves (Hahn and Denlinger, Reference Hahn and Denlinger2011; Marshall and Sinclair, Reference Marshall and Sinclair2018).
We measured total body protein content as one potential facet of host nutritional quality through time in dormant larvae. While there was no difference in body protein content between non-dormant controls and P. interpuntella larvae held dormant for 15 days, longer periods of dormancy showed higher total body protein content with the highest body protein contents occurring after 75 and 90 days of storage. But interestingly, between 90 and 105 days of storage body protein content dropped sharply. These data also agree with previous work on host protein content from our group, wherein the protein content of our 15-day dormant larvae (~18% when held at 15°C, 12:12 LD) is very similar to larvae early in a previous paper with similar conditions (~21% body protein for 15-day-old diapausing larvae held at 17°C, 12:12 LD in Hasan et al., Reference Hasan, Hasan, Khatun, Hossain, Athanassiou and Bari2020). While we do not know what other changes in body content or metabolism may have accompanied changes in total body protein content that we observe in this study, we speculate that perhaps body protein content initially increased as dormant larvae catabolized fat or other stores, but that once other stores had reached very low levels, dormant larvae may have begun catabolizing protein, leading to the precipitous decrease in protein content between 90 and 105 days of dormancy. Because P. interpuntella enters dormancy at temperatures well above freezing, it is highly unlikely that they expend resources on the synthesis of large quantities of cryoprotectants, such as glycerol, that can consume substantial energy reserves in other insects (Adedokun and Denlinger, Reference Adedokun and Denlinger1985; Storey and Storey, Reference Storey and Storey1986). Carbohydrates, such as glycogen or trehalose, could also be the major source of energy for dormant larvae (Becker et al., Reference Becker, Schlöder, Steele and Wegener1996; Zhou and Miesfeld, Reference Zhou and Miesfeld2009). Future studies should investigate total neutral lipid content, assumed to be indicative of stored triacylglycerides, and carbohydrate substrates within dormant and non-dormant P. interpuntella held under these or similar conditions.
It is also possible that dormancy impacts the immune response of P. interpunctella, making it more susceptible to parasitism. Although dormant insects have been found to maintain an innate immune response, lower temperatures and dormancy can impact behavioral defenses in host–parasitoid interactions (Nakamura et al., Reference Nakamura, Miyado, Takezawa, Ohnami, Sato, Ono, Harada, Yoshida, Kanai, Miyado and Umezawa2011; Le Lann et al., Reference Le Lann, Lodi and Ellers2014; Ferguson et al., Reference Ferguson, Heinrichs and Sinclair2016, Reference Ferguson, Kortet and Sinclair2018; Wu et al., Reference Wu, Hoffmann and Thomson2016; Warsi and Mbata, Reference Warsi and Mbata2018). It is important to note H. hebetor larval development is significantly longer when being reared from hosts that were previously dormant. This may simply be due to competition among the parasitoid larvae, because an increase in developmental time with higher density of H. hebetor larvae developing in a single host has previously been noted (Milonas, Reference Milonas2005). Aside from the slightly longer development time, there appear to be no other changes in larval development or adult size in H. hebetor developing from previously dormant hosts.
In conclusion, the absence of detrimental effects of storage on P. interpunctella combined with the increased production of H. hebetor from stored larvae indicates that prolonged storage of 5th instar P. interpuntella larvae for mass rearing of H. hebetor is a viable option. Furthermore, because H. hebetor oviposit on 5th instar P. interpuntella and dormancy extends the duration of the 5th larval instar, increasing the time that the moths are susceptible to parasitoid attack would be a clear benefit to parasitoid mass rearing programs (Akinkurolere et al., Reference Akinkurolere, Boyer, Chen and Zhang2009; Warsi and Mbata, Reference Warsi and Mbata2018). The ability to produce and maintain a large supply of host insects is a major barrier in parasitoid mass rearing programs (Murai and Loomans, Reference Murai and Loomans2001; Saleh et al., Reference Saleh, Allawi and Ghabiesh2010; Ovruski and Schliserman, Reference Ovruski and Schliserman2012; Sanower et al., Reference Sanower, Mbata and Payton2018). Thus, we join other authors in advocating for using host dormancy to improve the efficacy and cost efficiency of biological control (Mohandass et al., Reference Mohandass, Arthur, Zhu and Throne2007; Li et al., Reference Li, Zhang, Zhang, Chen and Denlinger2014; Sanower et al., Reference Sanower, Mbata and Payton2018).
Acknowledgements
This work was supported by FAO/IAEA joint Coordinated Research Project on Dormancy Management to Enable Mass-rearing grant 18133 to M. M. H. as well as the US National Science Foundation (IOS 1257298 and DEB 1639005), the Florida Agricultural Experiment Station, and the joint FAO/IAEA Coordinated Research Project on Dormancy Management to Enable Mass-rearing to D. A. H. Biochemical analyses were carried out at BCSIR, Bangladesh. The parasitoids were provided by the Bangladesh Agriculture Research Institute (BARI), Gazipur, Bangladesh. The authors would like thank Dr Asif Ahsan, College of Life Science, Zhejiang University, China for assisting with data analysis. The authors are grateful to the Department of Zoology, Rajshahi University for making laboratory facilities available for this work.
Author contributions
M. M. H. and C. G. A. designed the study, M. M. H. and A. S. M. S. R. conducted lab work, M. M. H. and D. A. T. analyzed the data. M. M. H. and C. G. A. wrote the first draft of manuscript, D. A. H. completed the final draft of the manuscript and all authors contributed to the final version.
Conflict of interest
None.