Pyrroloquinoline quinone (PQQ) (4,5-dihydro,5-dioxo H-pyrroloquinoline-2,7,9 tricarboxylic acid) is a three-cyclic helobacterium that acts as a substitute for the role of combined oxidative agents of many bacterial hydrogen derivatives including methanol, ethanol and glucose dehydrogenases( Reference Ahmed and Shahab 1 , Reference Adachi 2 ) also called methotaxin( Reference Alkasrawi, Popescu and Mattiasson 3 , Reference Naveed, Tariq and Sadia 4 ). PQQ, which is a water-soluble, thermo-stable TAG-quinone, is widely distributed in nature( Reference Rucker, Stites and Steinberg 5 ) and characterised as a mammalian vitamin-like redox cofactor( Reference Kasahara and Kato 6 ). Its reducing properties are good candidates for redox control signalling in viable cells( Reference Misra, Rajpurohit and Khairnar 7 ) and are more effective than other bioactive quinones and ascorbic acid( Reference Samuel, Zhang and Wang 8 ). PQQ has powerful antioxidant properties and is found naturally in plant tissues, mammalian organs and biological fluids( Reference Noji, Nakamura and Kitahata 9 ). The antioxidant action of PQQ depends on its ability to catalyse the conversion of superoxide to dioxy. Furthermore, PQQ in oxidised quinone form is reduced to its semi-quinone and hydroquinone forms; and, under appropriate conditions, PQQ can catalyse a continuous redox cycle( Reference Bishop, Gallop and Karnovsky 10 ). The potential number of catalyst cycles is approximately 2000, and four catalytic cycles of ascorbic acid have been reported( Reference Rucker, Stites and Steinberg 5 ). Due to its antioxidant properties( Reference Tsuchida, Yasuyama and Higuchi 11 ), PQQ further exerts a neuroprotective and peritoneal protective action against mammalian oxidative stress( Reference Wang, Zhang and Xu 12 ).
PQQ is ubiquitous in nature and found in many dietary sources such as fermented soyabeans, tea, green peppers, parsley, kiwi and human milk( Reference Mitchell, Jones and Mercer 13 , Reference Kumazawa, Sato and Seno 14 ). However, according to various reports, the PQQ content in food differs even though various PQQ instrumental analysis and bioassay methods have been established. This is because PQQ is chemically reactive and easily forms derivatives or condensation products with other nutrients( Reference Ameyama, Nonobe and Shinagawa 15 , Reference Bergethon 16 ). Although PQQ is not currently classified as a vitamin, PQQ has been implicated as an important nutrient in mammals( Reference Felton and Anthony 17 ). However, PQQ has attracted considerable attention, as it is important for mammalian growth, development, reproduction and immune function. PQQ is also beneficial to animal growth and development( Reference Steinberg, Stites and Anderson 18 ). Current research indicates that mice fed a well-nourished diet but lacks PQQ exhibit decreases in the fertility of both first- and second-generation mice and in the growth rate of newborns when compared with mice supplemented with PQQ( Reference Steinberg, Gershwin and Rucker 19 ). Signs of pregnancy and neonatal PQQ deprivation in severely affected mice usually include friable skin and a humpback posture( Reference Steinberg, Stites and Anderson 20 ).
In Canada, PQQ-containing products have been approved for use as natural antioxidants for health maintenance( 21 ) and in the United States as a dietary supplement for human consumption (e.g. BioPQQ). The most widely used commercial product is pyrroloquinoline quinone disodium (PQQ·Na2) salt( Reference Wang, Zhang and Samuel 22 ). PQQ Na2 is generally used in various experiments due to its high solubility in water( Reference Kuang, Liu and Qiang 23 ), which makes it easy to handle, and this property gives PQQ·Na2 an advantage in comparison with free-form PQQ( Reference Akagawa, Nakano and Ikemoto 24 ). Although PQQ is not biosynthesised in mammals, trace amounts of PQQ have been found in human and rat tissues due to its wide distribution across dietary sources. Nutritional studies in rodents have revealed that a PQQ deficiency exhibits diverse systemic responses, including growth impairment, immune dysfunction and abnormal reproductive performance. However, it is still unknown whether PQQ increases reproduction performance or not. In addition, there are no published data on the effects of maternal dietary treatment and PQQ on the intestinal functioning of their offspring. Therefore, the objective of the present study was to determine whether dietary PQQ·Na2 supplementation is able to increase the number of viable fetuses per litter, and whether the weight of uterine horns with fetuses increased at 1 d of newborn. We also explored whether dietary PQQ·Na2 supplementation was able to improve the antioxidant capacity of the small intestine and the intestinal morphology of weaned rats. In summary, the results of this study could lay a foundation for the further effects of PQQ on maternal reproductive performance and intestinal health.
Methods
Ethics statement
This study was performed in strict accordance with the recommendations of the National Research Council Guide, and all animal experimental procedures were approved by the Ethical and Animal Welfare Committee of Heilongjiang Province, China. All surgeries were performed under anaesthesia using an intraperitoneal injection of sodium pentobarbital, and every effort was made to minimise animal suffering.
Animals and experimental design
Pregnant rats received diets containing different concentrations of PQQ·Na2 (purity, ≥99·5 %). The rats were divided into five groups and received a daily feeding of 0, 0·2, 0·4, 0·8 and 1·6 mg/kg of PQQ·Na2, respectively (Table 1). The animals were given drinking water and food ad libitum.
Table 1 Composition and nutrient levels of the basal diet (air-dry basis)
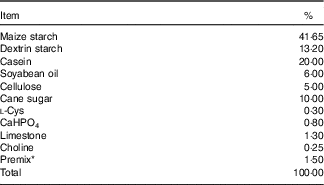
* The premix provided the following per kg of the diet: Fe (as ferrous sulfate) 35 mg, Cu (as copper sulphate) 6 mg, Zn (as zinc sulphate) 30 mg, Mn 10 mg, vitamin A 4·2mg, vitamin D3 0·037mg, vitamin E 80mg, riboflavin 12 mg, nicotinic acid 60 mg, d-pantothenic acid 24 mg, vitamin B12 1·2 mg, biotin 0·2 mg.
Sprague–Dawley (SD) rats were obtained from the Jilin University Laboratory Animal Centre (Changchun, China) and were acclimated for 1 week before experimentation. The weights of the male rats ranged from 300 to 325 g, and the weights of the female rats ranged from 190 to 210 g (at 9 weeks). The experiment was conducted on 120 female rats divided into five groups that received a daily feeding of 0, 0·2, 0·4, 0·8 and 1·6 mg/kg of PQQ·Na2. Male rats were used as sires only and were not treated. The rats were kept in plastic rat cages and had free access to food and water. The animals were maintained on a 12 h light–12 h dark cycle at 24±2°C in the study room. The relative humidity was maintained at 47–55 %( Reference Wen-Min, Lin-Zi and Zhang 25 , Reference Zhang, Jia and Yin 26 ). Throughout the study, the male and female rats were fed experimental animal rations (University Laboratory Animal Centre). The feed was prepared in an experimental feed mill using high-precision mixers, and all the feedstuffs were subjected to post-processing analytical control. The groupings are presented in Table 2.
Table 2 Pyrroloquinoline quinone disodium (PQQ·Na2) concentrations in feed and the number of rats treated per concentration group
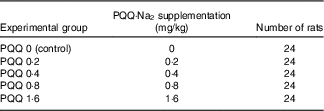
Cohabitation began at approximately 16.30 hours on each mating day (two females per male). The next morning, each female was examined for the presence of sperm using a vaginal lavage. A total of 120 sperm-positive females were presumed to be pregnant on gestational day (GD) 0, and a stratified random procedure was used to assign each animal to a control group or one of the four treatment groups( Reference Zhang, Jia and Yin 26 ). Each pregnant rat was individually maintained in a polycarbonate metabolic cage (Tecniplast). The diet was maintained until rats were weaned on postnatal day (PD) 21. A total of eight pregnant rats within each group were euthanised on GD 20. A total of eight weaning rats per group were euthanised at the time of weaning on PD 21.
Caesarean section, slaughter and collection of samples
The animals were euthanised under anaesthesia using an intraperitoneal injection of sodium pentobarbital (0·5 ml/kg) on GD 20. For blood collection, microcentrifuge tubes were coated with 10 ml of heparin sodium salt (20 U/ml in phosphate buffered saline). Whole blood was collected from the submandibular site as described previously and maintained on ice for 30 min. The samples were centrifuged at 3000 g and 4°C for 10 min. The plasma layers were removed, placed into sterile microcentrifuge tubes, and stored at –40°C until assayed. The placentas were frozen in liquid N2, stored at –80°C and either subjected to RNA or quantitative RT-PCR to analyse their gene expressions. The remaining pregnant rats were fed with the experimental diet until the pups were weaned at 3 weeks of age. A total of eight weaned rats with similar body weights were randomly selected from each group and killed using diethyl ether anaesthetisation. After a laparotomy, their small intestines were dissected and freed from the mesenteric attachment, and all chyme were removed immediately. The jejunum segments were rinsed in a chilled sodium chloride solution (0·9 g/l saline) and divided into three segments of equal length. One part was frozen in liquid N2 and stored at –80°C until RNA extraction. The second part was fixed in 10 % formalin for morphological analysis. The third part was sealed into pockets and preserved at –20°C until used for the evaluation of antioxidant status. The contents of the caecum were immediately frozen in liquid N2 and stored at –80°C until microbial DNA analysis.
Evaluation of antioxidant enzyme activity
The activities of total superoxide dismutase (SOD), glutathione peroxidase (GPx) and catalase (CAT) were analysed using commercial reagent kits( Reference Rongzhu, Suhua and Guangwei 27 ). Analysis of the SOD activity was based on the SOD-mediated inhibition of the formation of nitrite from hydroxylammonium in the presence of O2– generators (xanthine/xanthine oxidase)( Reference Elstner and Heupel 28 ). Activity of SOD was expressed as units/mg of protein and determined by measuring the reduction in the optical density of the reaction solution at 550 nm. GPx is an enzyme that catalyses glutathione oxidation by oxidising the reduced tripeptide GSH into GSSG( Reference Sedlak and Lindsay 29 ). GPx activity was expressed as units/mg of protein, and one unit was defined as the amount required to decrease GSH by 1 mm/min after subtracting the decrease in GSH/min obtained from the non-enzymatic reaction. CAT activity was assayed by the method developed by Aebi( Reference Aebi 30 ) and calculated as nmol H2O2 consumed/min per mg of tissue protein. Approximately 1 g of mucosal scrapings was homogenised after being suspended in 9 ml of saline. After centrifugation at 3000 rpm for 15 min, the supernatant was taken for the measurement of the total SOD, reduced GSH, MDA and CAT. All antioxidant parameters were measured using the methodology of assay kits supplied by Nanjing Jiancheng Bioengineering Institute( Reference Mao, Lv and Yu 31 , Reference Che, Xuan and Hu 32 ).
Real time-PCR (RT-PCR)
Total DNA from the middle section of the caecum samples was extracted using the TIANamp Stool DNA Kit (Tiangen Biotech Ltd), according to the manufacturer’s instructions. The quantity and quality of DNA were assessed using a NanoPhotometer-P330 spectrophotometer (Implen GmbH). Primers for total eubacteria, Lactobacillus spp., Enterobacteriaceae and bifidobacteria spp. were designed according to previously published data( Reference Bartosch, Fite and Macfarlane 33 , Reference Rinttilä, Kassinen and Malinen 34 ). Primers (Table 3) were used for the estimation of select bacterial groups by quantitative real-time PCR using the ABI 7500 Real-Time PCR system (Applied Biosystems Limited). For bacterial groups, real-time PCR used the SYBR® Premix Ex Taq™ II system (TaKaRa® Bio Catalog). The PCR was run in a volume of 20 µl, with 10 µl of SYBR® Premix Ex Taq™, 0·8 µl of each primer (0·4 µm), 0·4 µl of ROX Reference Dye II (TaKaRa), 6·0 µl of double-distilled water and 2 µl of DNA template. The thermal cycling conditions involved an initial denaturation step at 95°C for 5 min, followed by forty cycles of 95°C for 15 s and 72°C for 30 s. Dissociation analyses of the PCR products were carried out to confirm the specificity of the resulting PCR products. Quantification was performed in duplicate, and the mean values were calculated. The results were reported as log10 16S ribosomal DNA gene copies per gram fresh matter.
Table 3 Primers used for real-time PCR to detect bacterial numbers

F, forward; R, reverse.
Total RNA was extracted from approximately 100 mg of frozen jejunal tissues. The concentration of RNA was estimated based on its absorbance at 260 nm, which was determined using a spectrophotometer. The RNA quality was determined by checking its integrity through agarose gel electrophoresis and by confirming that the A
260 nm:A
280 nm absorbance ratio was between 1·8 and 2·0. Total RNA from each sample was converted into complementary DNA using the Prime Script® RT reagent kit (TaKaRa® Bio Catalog) according to the manufacturer’s instructions and used for RT-PCR. β-Actin was used as a reference gene and was stable and not changed by the different treatments. The real-time PCR process was in accordance with the description in a previous study. All the PCR were performed in triplicate (Table 4). The relative gene expression levels in the intestines and placenta were determined using the
$$2^{{{\minus}\Delta \Delta C_{{\rm t}} }} $$
method(
Reference Livak and Schmittgen
35
).
Table 4 Primers used for real-time PCR
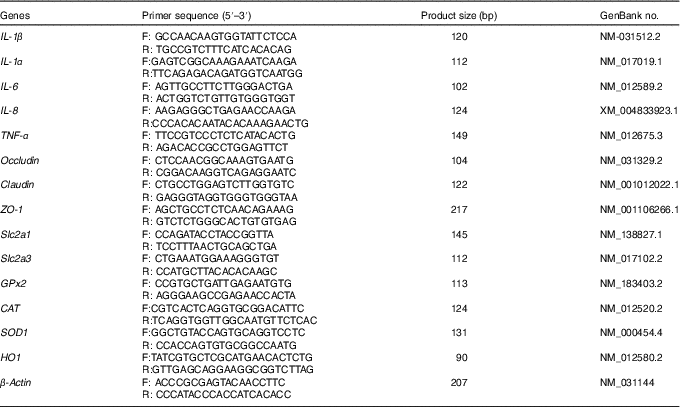
F, forward; R, reverse; ZO-1, zonula occludens-1; Slc2a1, solute carrier family 2 member 1; Slc2a3, solute carrier family 2 member 3; GPx, glutathione peroxidase; CAT, catalase; SOD, superoxide dismutase; HO1, haem oxygenase 1.
Morphological analysis and brush border enzyme activities
Cross-sectional jejunal samples from the formalin-preserved segments were cut into 2 mm sections and fixed by standard paraffin embedding. Samples were sectioned at 5 mm and stained with haematoxylin–eosin. Each slide was divided into three single segments and the microstructures of the jejunum were analysed using an optical microscope (Nikon Eclipse E400). The villous heights and crypt depths of thirty randomly chosen villi were measured.
Other sections (10 cm) of jejunum were dissected from each rat and rinsed with PBS with a pH of 7·2 to measure the specific activity of mucosa enzymes. The mucosa was carefully scraped off with a glass slide placed in Eppendorf tubes and immediately frozen in liquid N2 for later measurement. Mucosa samples were thawed and flushed with isotonic saline at 4°C. Exactly 0·5 g of mucosa was homogenised for 2 min in a chilled sodium chloride solution (0·9 g/l saline) with the ratio 1:9 (w/v), followed by centrifugation at 3000 rpm for 10 min. The supernatant was diluted with a sodium chloride solution (0·9 g/l saline) at a ratio of 1:5 (w/w) for the determination of lactase (β-galactosidase; EC 3.2.1.23) and sucrase (sucrose-α-glucosidase; EC 3.2.1.48) activities and later diluted 1:50 (w/w) for the determination of maltase (β-glucosidase; EC 3.2.1.20).
Hormone levels
The levels of prolactin (PRO), follicle-stimulating hormone (FSH), luteinising hormone (LH), progesterone (PRG) and oestradiol (E2) were determined using an ELISA kit (R&D Systems Inc.), according to the manufacturer’s recommended protocol.
Statistical analysis
All data were analysed using SPSS 19.0 software (SPSS Inc.). All data were analysed using the one-way ANOVA, and means were compared using Duncan’s multiple range test. A Shapiro–Wilk W normality test was performed. All pairwise multiple comparisons of means test for determining significance of differences among the five treatment groups were performed as applicable. The individual rat and her litter were used as the experimental unit. The results were presented as mean values and the standard error of the mean. In all statistical tests used, P<0·05 was considered statistically different.
Results
Reproductive performance
The results pertaining to the reproductive performance of gestational rats are shown in Table 5. The placental wet weights and the weight and length of uterine horns with fetuses at GD 20 in female rats exhibited an increasing tendency, but this difference was not significant (P>0·05). The number of implanted embryos per litter increased significantly (P<0·05) with dietary 0·8 and 1·6 mg/kg PQQ·Na2 during gestation and lactation at GD 20, showing dose dependency. The number of viable fetuses per litter and born alive litter weight increased at 1 d of newborn (P<0·05) with dietary 1·6 mg/kg PQQ·Na2, in a dose-dependent manner, compared with the control. However, weaned rats did not show any effects on these variables with dietary PQQ·Na2 supplementation during gestation and lactation (P>0·05).
Table 5 Effects of dietary pyrroloquinoline quinone (PQQ) disodium supplementation during gestation and lactation on reproductive performance of rats (Mean values with their standard errors)
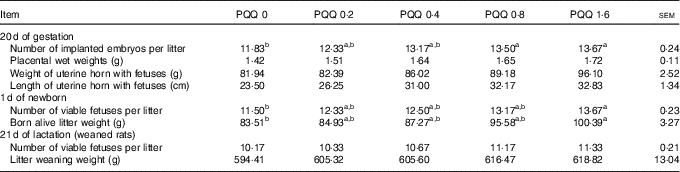
a,b Mean values within a row with unlike superscript letters were significantly different (P<0·05).
Placental antioxidant and glucose transporter gene expression
The mRNA expression levels of antioxidant and glucose transporter genes are presented in Fig. 1. The mRNA expression levels of CAT, GPx2 and SOD1 in the placenta increased (P<0·05) with dietary 0·8 and 1·6 mg/kg PQQ·Na2 supplementation, but the mRNA expression levels of haem oxygenase 1 were not affected (P>0·05). In addition, the mRNA expression of solute carrier family 2 member 1 (Slc2a1) and solute carrier family 2 member 3 (Slc2a3) were also increased (P<0·05) with dietary 1·6 mg/kg PQQ·Na2, in a dose-dependent manner.

Fig. 1 Effects of dietary pyrroloquinoline quinone (PQQ) disodium supplementation during gestation and lactation of rats on antioxidant status and glucose transporter in placentas for real-time PCR of female rats. (A) Haem oxygenase 1 (HO1), glutathione peroxidase (GPx2), catalase (CAT), superoxide dismutase (SOD1) and (B) quantitative mRNA expression of solute carrier family 2 member 1 (Slc2a1) and solute carrier family 2 member 3 (Slc2a3) by real-time PCR in rat placentas. Values are means (n 6), with their standard errors represented by vertical bars. a,b,c Mean values with unlike letters were significantly different (P<0·05). , PQQ 0;
, PQQ 0·2;
, PQQ 0·4;
, PQQ 0·8;
, PQQ 1·6.
Concentrations of follicle-stimulating hormone, luteinising hormone, prolactin, progesterone and oestradiol in the plasma of female rats at gestation day 20
The gonadotropin (FSH, LH and PRO) and sex steroid (PRG and E2) concentrations in blood plasma obtained during caesarean section on GD 20 are shown in Table 6. The E2 and PRG concentrations were significantly increased (P<0·05) with dietary PQQ·Na2, and this is the response at all concentrations. The concentration of LH was significantly increased (P<0·05) for the PQQ 0·4 and PQQ 1·6 groups, while the concentration of PRO increased significantly (P<0·05) for the PQQ 0·8 and PQQ 1·6 groups.
Table 6 Concentrations of follicle-stimulating hormone (FSH), luteinising hormone (LH), prolactin (PRO) and oestradiol (E2) in the plasma of female rats on gestation day 20 (Mean values with their standard errors, n 6)

PQQ, pyrroloquinoline quinone; PRG, progesterone.
a,b Mean values within a row with unlike superscript letters were significantly different (P<0·05).
Intestinal morphology of weaned rats
The effects of dietary PQQ·Na2 supplementation during gestation and lactation on villous height, crypt depth and villous height/crypt depth (V/C) in the small intestine of weaned rats are shown in Table 7. Duodenum villous height was significantly higher in the PQQ 0·2 and PQQ 1·6 weaned rat groups (P<0·05). The jejunum villous height increased significantly in a dose-dependent manner in the PQQ 1·6 group of weaned rats (P<0·05), from PQQ 0·2 to PQQ 1·6. The small intestine V/C had an increasing tendency, but this difference was not significant (P>0·05). There were no effects on crypt depth in the small intestine with dietary PQQ·Na2 supplementation during gestation and lactation (P>0·05).
Table 7 Effects of dietary pyrroloquinoline quinone (PQQ) supplementation during gestation and lactation on villous height, crypt depth and villous height/crypt depth (V/C) in small intestine of weaned rats (Mean values with their standard errors, n 6)

a,b Mean values within a row with unlike superscript letters were significantly different (P<0·05).
Intestinal microflora of weaned rats
Fig. 2 shows that dietary supplementation with PQQ·Na2 influenced bacterial numbers in the digesta of the caecum in weaned rats on PD 21. There was a significant increase between the PQQ 0 and PQQ 1·6 groups in regard to the number of Lactobacillus spp. in the digesta of the caecum in weaning rats on PD 21 (P<0·05). The numbers of total eubacteria, Enterobacteriaceae and Bifidobacterium spp. exhibited an increasing tendency, with a concomitant increase in dietary PQQ·Na2 concentration supplementation when compared to the PQQ 0 group, but this difference was not significant (P>0·05).

Fig. 2 Effects of dietary pyrroloquinoline quinone (PQQ) disodium supplementation during gestation and lactation of rats on bacterial numbers of weaned rats. The results were reported as log10 16S ribosomal DNA gene copies/g fresh matter. Values are means (n 6), with their standard errors represented by vertical bars. a,b Mean values with unlike letters were significantly different (P<0·05). , PQQ 0;
, PQQ 0·2;
, PQQ 0·4;
, PQQ 0·8;
, PQQ 1·6.
Antioxidant capacity in the small intestine
The effects of dietary PQQ·Na2 supplementation during gestation and lactation on antioxidant capacity in the small intestine of female rats on GD 20 is shown in Fig. 3. On GD 20, the CAT activity in the ileum of female rats was significantly increased by PQQ·Na2 supplementation (P<0·05), and this is the response at all concentrations. Fig. 4 shows that dietary supplementation with PQQ·Na2 influenced the antioxidant status in the small intestine in weaned rats on PD 21. PQQ·Na2 dietary supplementation in gestating and lactating rats (PQQ 1·6) increased the CAT activity of the jejunal mucosa of weaned rats on PD 21 (P<0·05). The SOD activity of the jejunal mucosa was significantly increased in the PQQ 0·4, PQQ 0·8 and PQQ 1·6 groups of weaned rats (P<0·05). While PQQ·Na2 (in the PQQ 0·4, PQQ 0·8 and PQQ 1·6 groups) induced a significant increase in GPx activity of the jejunum in weaned rats (P<0·05). In addition, PQQ 0·4 and PQQ 1·6 groups exhibited a significant increase in the GPx activity of the ileum mucosa in weaned rats.

Fig. 3 Effects of dietary pyrroloquinoline quinone (PQQ) disodium supplementation during gestation and lactation of female rats on antioxidant status in small intestine on gestation day 20. (A) Catalase (CAT), (B) superoxide dismutase (SOD), (C) malondialdehyde (MDA) and (D) glutathione peroxidase (GSH-Px). Values are means (n 6), with their standard errors represented by vertical bars. a,b Mean values with unlike letters were significantly different (P<0·05). , PQQ 0;
, PQQ 0·2;
, PQQ 0·4;
, PQQ 0·8;
, PQQ 1·6.
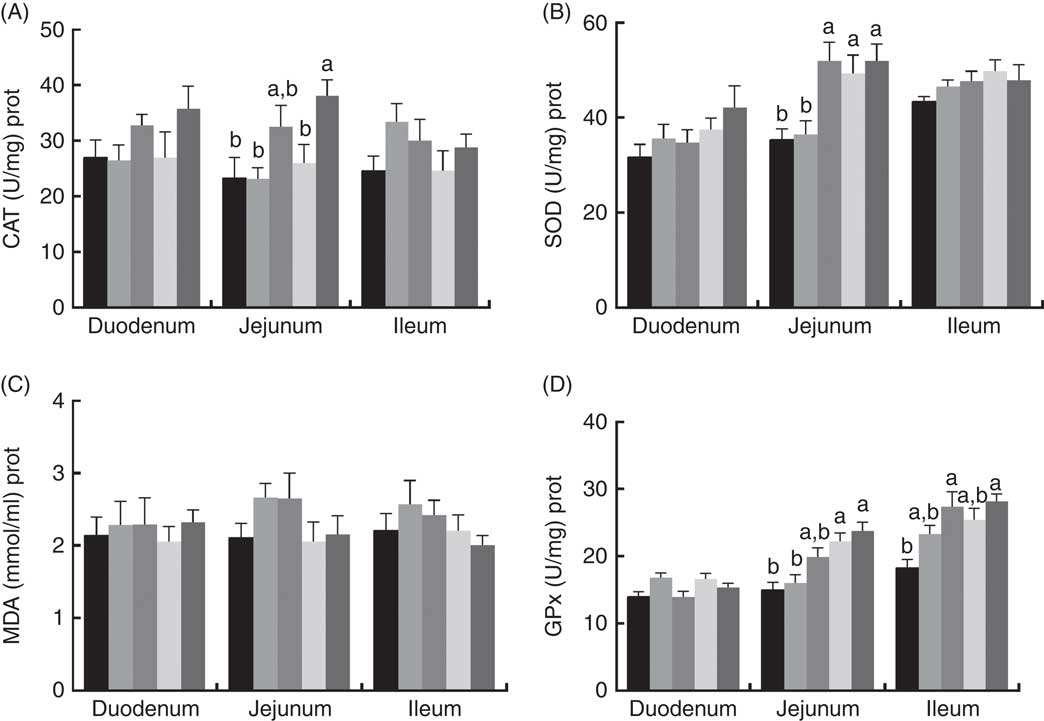
Fig. 4 Effects of dietary pyrroloquinoline quinone (PQQ) disodium supplementation during gestation and lactation of weaned rats on antioxidant status in small intestine. (A) Catalase (CAT), (B) superoxide dismutase (SOD), (C) malondialdehyde (MDA) and (D) glutathione peroxidase (GSH-Px). Values are means (n 6), with their standard errors represented by vertical bars. a,b Mean values with unlike letters were significantly different (P<0·05). , PQQ 0;
, PQQ 0·2;
, PQQ 0·4;
, PQQ 0·8;
, PQQ 1·6.
Gene expression of tight junction proteins and concentrations of cytokines in jejunal of weaned rats
Immune responses in the intestinal mucosa are partly controlled by cytokine release in response to environmental stimuli. We therefore investigated the gene expression of cytokines in the jejunum. The results are shown in Fig. 5. The mRNA expression of pro-inflammatory cytokines including IL-8, TNF-α and IL-1α exhibited a reducing tendency, but these differences were not significant (P>0·05). The IL-6 and IL-1β cytokines were not affected (P>0·05) by dietary PQQ·Na2. Fig. 6 shows that dietary supplementation with PQQ·Na2 influenced the expression of tight junction proteins in the jejunum of weaned rats. The results of the expression of tight junction proteins suggest that dietary supplementation with PQQ·Na2 in gestating and lactating rats (PQQ 0·4 and PQQ 0·8 groups) increased the expression of zonula occludens-1 (ZO-1) mRNA in the jejunal mucosa of weaned rats (P<0·05). In addition, the expression of claudin mRNA in the jejunal mucosa in the PQQ 0·8 and PQQ 1·6 groups was significantly increased in weaned rats (P<0·05). The expression of occludin mRNA showed an increasing trend with an increase in PQQ·Na2, but this was not significant (P>0·05).
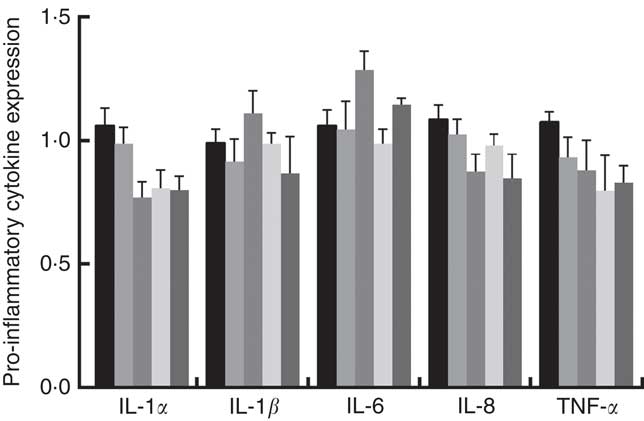
Fig. 5 Effects of dietary pyrroloquinoline quinone (PQQ) disodium supplementation during gestating and lactating rats on the concentrations of cytokines in the jejunal mucosa of weaned rats. , PQQ 0;
, PQQ 0·2;
, PQQ 0·4;
, PQQ 0·8;
, PQQ 1·6.
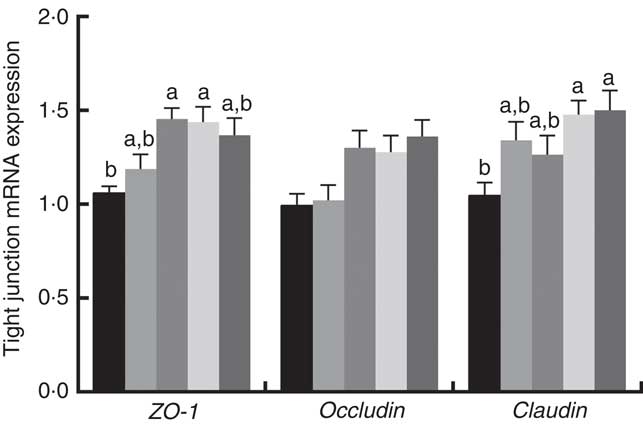
Fig. 6 Effects of dietary pyrroloquinoline quinone (PQQ) disodium supplementation during gestating and lactating rats on tight junction mRNA expression in the jejunal mucosa of rats weaned from their offspring. ZO-1, zonula occludens-1. Values are means (n 6), with their standard errors represented by vertical bars. a,b Mean values with unlike letters were significantly different (P<0·05). , PQQ 0;
, PQQ 0·2;
, PQQ 0·4;
, PQQ 0·8;
, PQQ 1·6.
Brush border enzymes activities
There was a significant increase (P<0·05) between PQQ 1·6 and PQQ 0 groups regarding the activities of sucrase in the jejunum of the pregnant rats on GD 20 (Fig. 7(A)). Furthermore, PQQ 0·4 and PQQ 1·6 groups increased (P<0·05) the activities of maltase in the jejunum of the pregnant rats on GD 20 (Fig. 7(A)). Fig. 7(B) shows the enzyme activities in the jejunum of the weaned rats. PQQ 0·4 and PQQ 1·6 groups had a significant increase (P<0·05) in the activity of sucrase in the jejunum on PD 21 (Fig. 7(B)). The activity of maltase in the jejunum was significantly increased (P<0·05) in the PQQ 0·8 and PQQ 1·6 groups of weaned rats on PD 21, showing dose dependency.
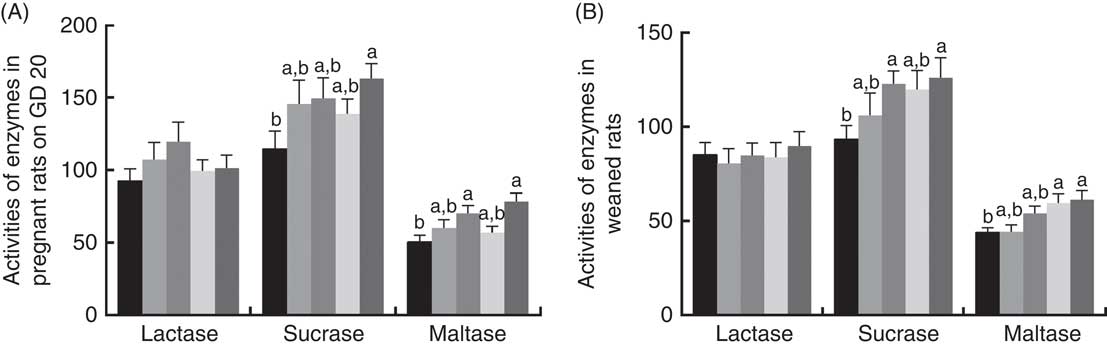
Fig. 7 Effects of dietary pyrroloquinoline quinone (PQQ) disodium supplementation during gestating and lactating rats on brush border enzyme activities in the jejunal. (A) Activities of brush border enzymes in the jejunal of female rats on gestation day (GD) 20; (B) activities of brush border enzymes in the jejunal of weaned rats. Values are means (n 6), with their standard errors represented by vertical bars. a,b Mean values with unlike letters were significantly different (P<0·05). , PQQ 0;
, PQQ 0·2;
, PQQ 0·4;
, PQQ 0·8;
, PQQ 1·6.
Discussion
The use of PQQ in nutrition is increasingly being discussed in the literature( Reference Samuel, Zhang and Wang 8 , Reference Wang, Zhang and Xu 12 ). There are several studies that have found beneficial effects of PQQ supplementation on the growth performance or body composition of growing rats( Reference Steinberg, Stites and Anderson 18 , Reference Steinberg, Gershwin and Rucker 19 ). Jonscher et al. ( Reference Jonscher, Stewart and Alfonso-Garcia 36 ) reported that PQQ treatment led to a significant increase in placental weight and placental surface area. The major objective of the present study was to investigate the influence of dietary PQQ·Na2 supplementation during gestation and lactation on reproductive performance in SD rats and on antioxidant gene expression in the placenta and the effect of the intestinal barrier functions of their offspring. To our knowledge, this is the first study to examine the effects of dietary PQQ·Na2 supplementation during gestation and lactation in SD rats and to investigate the effect of dietary treatment and PQQ·Na2 during gestation and lactation in female rats on the intestinal barrier functions of their offspring.
Oxidative stress, an imbalance between pro-oxidative and antioxidative forces in biological systems, may cause both lipid and protein oxidation and impair normal cell function, which is considered to be responsible for the initiation or development of pathological processes affecting female reproductive processes(
Reference Finkel
37
,
Reference Serdar, Gür and Colakoethullarý
38
). Studies have reported that lipid peroxidation and oxidative stress are higher during normal pregnancy than during non-pregnancy in women(
Reference Mihu, Sabău and Costin
39
). In addition, oxidative stress has been suggested as a causative agent in human and animal pregnancy-related disorders, including embryonic reabsorption, recurrent pregnancy loss, pre-eclampsia, intra-uterine growth restriction and fetal death, many of which are predictive of an elevated risk of the metabolic syndrome in postnatal life and may be a common pathway in developmental metabolic programming(
Reference Pereira and Martel
40
,
Reference Sánchez-Aranguren, Prada and Riaño-Medina
41
). The activity of SOD is known to serve a protective function in the elimination of reactive free radicals, and, therefore, it represents an important antioxidant defence in nearly all cells exposed to oxygen. GSH forms an important part of the non-enzymatic antioxidants(
Reference Cheung, Zheng and Li
42
). Similar to other sulfhydryl-containing products, GSH also has regulatory and protective roles in the body as it establishes the defence line of the body against tissue injury due to chemicals through its reactive oxygen species (ROS) scavenger, cell viability and membrane-stabilising effects(
Reference Szabó
43
). In our study, the antioxidant gene expressions of CAT, SOD and Gpx in the placenta were increased (P<0·05) with dietary PQQ·Na2 supplementation. The antioxidant enzymes SOD and CAT are considered the first-line defence against ROS. In our study, an increase in CAT and SOD was observed (P<0·05) with dietary PQQ·Na2 supplementation in the placenta. A previous study has demonstrated that SOD activity can be up-regulated by PQQ·Na2 treatment(
Reference Zhang, Ding and Gao
44
), and this is consistent with our study results. GSH is one of the most important molecules in mammalian cells for scavenging ROS. Thiol-containing compounds such as GSH and thioredoxin are oxidised by free radicals and rapidly regenerated(
Reference Yang, Lii and Lin
45
). GSH is the most abundant endogenously synthesised, thiol-containing, small-molecule tripeptide that can directly scavenge ROS in mammalian cells(
Reference Meister and Anderson
46
). The mRNA expression levels of GPx2 in the placenta were also increased (P<0·05) with dietary PQQ·Na2 supplementation. PQQ acts as an antioxidant by scavenging
$${\rm O}_{2}^{{\minus}} $$
and protects mitochondria from oxidative stress-induced damage. Therefore, the number of implanted embryos per litter were significantly increased (P<0·05) with dietary PQQ·Na2 during gestation and lactation at GD 20. The number of viable fetuses per litter and weight of uterine horns with fetuses were increased at 1 d of the newborn (P<0·05). Glucose and amino acids are the primary energy substrates utilised by the developing fetus and are transported across the placenta by glucose (Slc2a1 and Slc2a3). In our study, the mRNA expression of Slc2a1 and Slc2a3 were increased (P<0·05) with dietary PQQ·Na2
Reference Briffa, Hosseini and Tran
(47
). Many nutrient carriers and growth factors and fetal placenta from birth mothers decrease as the dam is exposed to stress. Because the number of implanted embryos per litter was significantly increased (P<0·05) with dietary PQQ·Na2 at GD 20, more glucose and amino acids were transported across the placenta by Slc2a1 and Slc2a3
(
Reference Robertson and Karp
48
).
Dietary substances that can alter hormone levels are of concern during pregnancy and are hormone sensitive for both mother and fetus( Reference Pasqualini, Kincl and Sumida 49 ). An increased feeding level PRG concentrations of gestation have been reported to be dependent on LH secretion( Reference Peltoniemi, Easton and Love 50 , Reference Tast, Love and Clarke 51 ), an increased LH secretion could therefore positively influence PRG concentration. The concentrations of PRG and oestrogen during pregnancy are high in mammals. PRG is critical for the establishment and maintenance of pregnancy, as its functions support ovulation and uterine as well as mammary gland development. The ovarian corpus luteum is the major source of PRG during pregnancy( Reference Petra, Hansen and Biserka 52 ). Oestrogen not only plays an essential role in the maintenance of pregnancy( Reference Albrecht, Aberdeen and Pepe 53 ) but also participates in the implantation process and stimulates the development of the mammary gland( Reference Topper 54 ). Generally, oestrogen and PRG perform in synergy. In our study, we observed that the E2, LH and PRG increased significantly (P<0·05) with dietary PQQ·Na2 in the blood plasma on GD 20. E2 and PRG act on the central nervous system, ovary and uterus and are important for ovulation, fertilisation, implantation of embryo, maintenance of pregnancy, mammary gland development and lactation( Reference Ahmed, Yeh and Tang 55 ). So PQQ·Na2 supplementation significantly improved the serum E2, LH and PRG, which suggested that PQQ·Na2 improved reproductive performance in rats, which in turn is associated with higher levels of hormones.
A maternal effect is a situation where the phenotype of an organism is determined not only by the environment it experiences and its genotype but also by the maternal environment and the genotype. The nutrient environment experienced by the mother is one of the most important factors affecting the growth and development of offspring in addition to heredity. The nutrient environment experienced by the mother can not only change physiological parameters but also affect the growth and development of the offspring by increasing or decreasing its sensitivity to the maternal transmission factor. In our study, the results of antioxidant capacity by PQQ·Na2 in the small intestine were not significant (P>0·05) on GD 20 (except for the CAT activity in the ileum). However, dietary supplementation with PQQ·Na2 in gestating and lactating rats (PQQ 1·6 group) increased the CAT activity of the jejunal mucosa of weaned rats on PD 21 (P<0·05). The SOD activity of the jejunal mucosa was significantly increased in the PQQ 0·4, PQQ 0·8 and PQQ 1·6 groups of weaned rats (P<0·05). PQQ (PQQ 0·4, PQQ 0·8 and PQQ 1·6 groups) also induced a significant increase in GPx activity of the jejunum in weaned rats (P<0·05). These results show that there is no significance in the antioxidant capacity of the small intestine by PQQ·Na2 in female rats. However, because of the maternal effect, PQQ can be transported to the offspring through the placenta and milk to improve the antioxidant capacity in the small intestine as a result of maternal supplementation with PQQ·Na2.
The gastrointestinal tract acts as the primary immune organ because it must respond to pathogenic stimuli and maintain resistance to co-immunisation and dietary antigens. Intestinal immunity is also closely related to other intestinal functions. Previous studies have shown that reduced expression of inflammatory cytokine genes may promote a more stable ecosystem and interfere with the growth of certain bacterial populations( Reference Walsh, Sweeney and O’Shea 56 ). Accelerated inflammatory cytokine factor overdose production can increase gut mucosal injury partially( Reference Liu, Huang and Hou 57 ). In a recent study, most of the pro-inflammatory cytokines such as TNF-α, IL-1α, IL-1β, IL-6 and IL-8 induced a pathological opening of the intestinal tight junction barrier and the intestinal epithelium was shown to increase permeability( Reference Alsadi, Boivin and Ma 58 ). In the present study, our results show that the mRNA expression of pro-inflammatory cytokines including IL-8, TNF-α and IL-1α exhibited a reducing tendency, which is consistent with that of Steinberg et al. ( Reference Steinberg, Gershwin and Rucker 19 ). Omata et al. ( Reference Omata, Fukatsu and Murakoshi 59 ) reported that PQQ is not included in clinically treated parenteral nutrient solutions because it has not been proven to be an essential nutrient for the body. However, in patients receiving parenteral nutrition, the possibility of PQQ supplements preventing gastrointestinal mucosal immune disorders exists( Reference Omata, Fukatsu and Murakoshi 59 ).
Parenteral nutrition can be used to prevent and reverse malnutrition and to maintain the nutritional status of patients who are unable to receive adequate enteral nutrition. During weaning, the intestinal morphology often undergoes large changes characterised by a decrease in the height of the villi and an increase in the depth of the crypt. This finding suggests that due to nutrient absorption, the surface area present on the villi is small, and the increase in immature fluff formed in the crypt may reduce the overall growth performance of the animal. In the present study, dietary supplementation with PQQ·Na2 on duodenum villous height was significantly higher in weaned rats (P<0·05). The villous height of the jejunum was significantly increased in the PQQ 1·6 group of weaned rats (P<0·05). Variations in both villous height and the ratio of villous height and crypt are good indicators of improved nutrient digestion and absorption capacity of the small intestine. Previous studies have shown that lower villus height and crypt depth is related to microbial challenges and the composition of animal feed( Reference Sayan, Assavacheep and Angkanaporn 60 ). Hedemann et al. ( Reference Hedemann, Eskildsen and Laerke 61 ) reported that the increases in villus length can inhibit the growth of many pathogens. The intestinal epithelial layer acts as a barrier to the infiltration of harmful intestinal contents into the bloodstream. In the jejunum, when the microvilli become more sparse and shorter, the permeability of the intestinal epithelial layer increases, the inflammatory response increases and intestinal motor dysfunction increases( Reference Collins 62 ). Therefore, we have further studied the gene expression of tight junction proteins and cytokine concentrations in the jejunum of weaned rats on PD 21. Tight junction proteins (claudin 1, ZO-1 and occludin) have a crucial role in intestinal barrier integrity and permeability by sealing the paracellular space between epithelial cells, thus preventing the paracellular diffusion of intestinal bacteria and other antigens across the epithelium( Reference Ulluwishewa, Anderson and Mcnabb 63 ). Friedman et al. ( Reference Friedman, Dobrinskikh and Alfonso-Garcia 64 ) reported that continuous exposure to PQQ improved the barrier dysfunction. Yin et al. ( Reference Yin, Ming and Bai 65 ) suggested that the expression of the jejunal tight junction protein ZO-1 was significantly higher in pigs with increase in PQQ·Na2. In our study, the results of the expression of tight junction proteins showed that dietary supplementation with PQQ·Na2 increased the expression of ZO-1 and claudin mRNA in the jejunal mucosa of weaned rats (P<0·05). Pro-inflammatory cytokines have been shown to down-regulate tight junction protein gene expression( Reference Alsadi, Boivin and Ma 58 , Reference Chen, Mao and He 66 ) through the dysregulation of tight junction proteins, resulting in an increase in intestinal permeability( Reference Berin, Yang and Ciok 67 ). This is part of the reason for increased higher expression of ZO-1 and claudin mRNA in weaned female rats supplemented with PQQ·Na2. Furthermore, the high concentration of PQQ in the offspring of female rats supplemented with PQQ can exert an antioxidant property, decreasing lipid peroxidation and ROS formation, possibly resulting in the increased expression of ZO-1 and claudin mRNA. The present study indicates that supplementation with PQQ·Na2 in female rats could enhance intestinal barrier integrity by up-regulating the tight junction protein mRNA in weaned rats.
The activity of brush border enzymes (such as lactase, sucrase and maltase) is closely related to the digestion of nutrients. Disaccharides (e.g. sucrose, maltose and lactose) can be hydrolysed by sucrase, maltase and lactase to the corresponding monosaccharide to be absorbed by the small intestine( Reference Pappenheimer 68 ). Disaccharides can only be absorbed by the disaccharidase of the small intestine. Improving disaccharide activity in the small intestine provides sustained energy storage that promotes carbohydrate degradation and also growth and development. The disaccharidase (i.e. brush edge enzyme) released by epithelial cells after shedding is determined by the height and maturation of chorionic cells( Reference Chen, Zhang and Zheng 69 ). In the present study, there was a significant effect (P<0·05) of increasing PQQ·Na2 supplementation on the activities of sucrase and maltase in the jejunum of the pregnant rats on GD 20. There was also a significant effect (P<0·05) of increasing PQQ·Na2 supplementation on the activities of sucrase and maltase in the jejunum of the weaned rats on PD 21. These results indicate that PQQ can improve the activities of sucrase and maltase. Simultaneously, this also indicates that PQQ can be passed through the mother to improve the activities of sucrase and maltase in weaned rats. One of the most important beneficial effects of the primary plexus is the creation of a barrier. Barriers are needed, as exogenous pathogens can cause colonisation of the digestive tract to be more difficult and may cause a disease called microbial colonisation resistance( Reference Brassart and Schiffrin 70 ). Therefore, one aim of the present study was to detect whether the PQQ diet of gestating and lactating female rats can affect the composition of the intestinal flora in their offspring. As the results show, dietary supplementation with PQQ·Na2 increased the number of Lactobacillus spp. in the digesta of the caecum in weaned rats.
Conclusions
In conclusion, our results have shown that dietary 1·6 mg/kg PQQ·Na2 supplementation during gestation and lactation of female rats can significantly increase the number of viable fetuses per litter, born alive litter weight and the mRNA expression levels of CAT, GPx2 and SOD1 in the placenta, thereby increasing the reproductive performance. The duodenum villous height, jejunum villous height, the activities of maltase and sucrase, the number of Lactobacillus spp. and the expression of ZO-1 and claudin mRNA were significantly increased by 1·6 mg/kg PQQ·Na2 supplementation. It suggested that PQQ can improve intestinal health by enhancing the intestinal morphology, tight junction function and improving the antioxidant status. The dietary supplementation of 1·6 mg/kg PQQ·Na2 is the best functional dose to improve reproductive performance of female rats and the intestinal barrier functions in the progeny based on the results from the present study.
Acknowledgements
The authors wish to acknowledgement the assistance of Yinguo Li, Chunliang Zhao and Xiaoyu Li.
This work was supported by the National Key Research and Development Plan of China (2016YFD0501207), the China Agriculture Research System (CARS-36). The funders had no role in the design, analysis or writing of this manuscript.
All authors participated in the development of the study concept and design. B. Z., W. Y. and H. Z. were responsible for the execution of the study. B. W., S. H. and Q. M. were involved in the animal experiments, analysis and data collection. Q. M. and Z. C. were responsible for the statistical analysis. B. Z. wrote the draft of the manuscript. Q. M., A. S. and Z. C. reviewed and revised the manuscript.
None of the authors has any conflicts of interest to declare.