Introduction
Usage of antibiotic growth promotors has been a common practice in European poultry farming in order to increase the performance until the phasing out in the year 2006. The raising awareness on the hazard subsequent to antibiotic feed additives in animal farming has led to the ban of such in European poultry production (Regulation (EC) No 1831/2003 chapter II article 11). Nevertheless, poultry meat still carries the highest contamination with ESBL-producing bacteria compared with other meat sources (Geser et al., Reference Geser, Stephan and Hachler2012; Carmo et al., Reference Carmo, Nielsen, Da Costa and Alban2014; De Jong et al., Reference De Jong, Smet, Ludwig, Stephan, De Graef, Vanrobaeys and Haesebrouck2014). Cephalosporins belong to the ß-lactam antibiotics, which can be hydrolyzed by extended-spectrum ß-lactamases and thereby exert a selective pressure on ESBL-producing bacteria (Paterson and Bonomo, Reference Paterson and Bonomo2005). Despite that several countries, such as Sweden and Belgium, do not use cephalosporins for poultry, a high prevalence of ESBL-producing bacteria remains (Smet et al., Reference Smet, Martel, Persoons, Dewulf, Heyndrickx, Catry, Herman, Haesebrouck and Butaye2008; SVARM 2011, 2012). This suggests that there are additional sources for the contamination with ESBL-producing bacteria in poultry farming (Hiroi et al., Reference Hiroi, Matsui, Kubo, Iida, Noda, Kanda, Sugiyama, Hara-Kudo and Ohashi2012a).
Worldwide, chicken was the second most common meat source (35.2%) in 2012, behind pork (36.3%) and followed by beef (22.2%) (http://www.fao.org/ag/againfo/themes/en/meat/backgr_sources.html). Poultry is the meat source with the highest percentage rise between 1990 and 2012 (104.2%) according to the Food and Agriculture Organization of the United Nations (FAO) (http://www.fao.org/ag/againfo/themes/en/meat/backgr_sources.html). ESBL-producing bacteria are able to transmit from poultry and poultry products to humans (Bertrand et al., Reference Bertrand, Weill, Cloeckaert, Vrints, Mairiaux, Praud, Dierick, Wildemauve, Godard, Butaye, Imberechts, Grimont and Collard2006; Leverstein-Van Hall et al., Reference Leverstein-Van Hall, Dierikx, Cohen Stuart, Voets, Van Den Munckhof, Van Essen-Zandbergen, Platteel, Fluit, Van De Sande-Bruinsma, Scharinga, Bonten, Mevius and National2011). The transmission from poultry to humans as food borne diseases or through the environment leads to a major hazard for public health (Apata, Reference Apata2009; Davies and Davies, Reference Davies and Davies2010). Infections with multi-drug resistant bacteria, such as ESBL-producing bacteria, are difficult to treat and cause high morbidity and increased mortality in humans (Davies and Davies, Reference Davies and Davies2010). Trivially, the accountable bacteria are therefore often referred to as ‘superbugs’. Examples for such bacterial species are Escherichia coli, Salmonella enterica and Klebsiella pneumoniae, all common bacteria in the gastrointestinal tract of poultry (Rehman et al., Reference Rehman, Vahjen, Awad and Zentek2007; Apata, Reference Apata2009; Davies and Davies, Reference Davies and Davies2010). Third-generation cephalosporins are frequently used to treat humans with difficult-to-cure infections caused by Enterobacteriaceae (Koga et al., Reference Koga, Scandorieiro, Vespero, Oba, De Brito, De Brito, Nakazato and Kobayashi2015). Currently, a rising occurrence of bacteria resistant to third-generation cephalosporins causes concern. In 2014, the national prevalence in Europe reached from 3.3 to 40.4% leading to an increase of the mean percentage from 9.6% (2011) to 12.0% (2014) in the EU/ EEA (European Economic Area) (Ears-Net, 2015). A major part of these bacteria produces ESBL. The European Antimicrobial Resistance Surveillance System (EARSS) reported a national prevalence in ESBL-producing bacteria in the EU/EEA between 73.6 to 100% in 2013 and 71.1 to 100% in 2014 (Ears-Net, 2014, 2015). In the EU, the growing awareness on multi resistant bacteria and the impact of co- and cross selection has led to a ban on antibiotic feed additives (Regulation (EC) No 1831/2003 chapter II article 11) and new adjustments on recommended mineral contents in feed (EFSA: https://www.efsa.europa.eu/en/press/news/160809a.). According to the World Health organization, ESBL-producing Enterobacteriaceae belong to the most urgent health issues (World Health Organization, 2014). It is therefore crucial to identify and reduce the ESBL load in poultry farming.
To our best knowledge, this is the first review comparing types and prevalence of ESBLs common in poultry. This information is vital hence to the possible transmission of ESBL genes from commensals to pathogens and from poultry to humans.
Classification of ESBL genes
Due to the transmission of plasmids with genes encoding for ESBLs between different species (Apata, Reference Apata2009) and the absence of correlation between ESBL-type and ESBL-producing bacteria, a categorization of ESBL by bacterial species is not considered useful. Hence, these bacteria are classified based on the amino acid sequences of the ESBL. They can be functionally classified into the group of Ambler's class A (TEM, SHV, CTX-M, PER, VEB, GES, TLA, BES) and D (OXA) lactamases according to Ambler et al. (Reference Ambler, Coulson, Frere, Ghuysen, Joris, Forsman, Levesque, Tiraby and Waley1991) (Bush et al., Reference Bush, Jacoby and Medeiros1995; Gniadkowski, Reference Gniadkowski2001). Worldwide, TEM (named after the patient Temoniera), SHV (sulphydryl reagent variable), and CTX-M (hydrolyses cefotaxime) are the most frequently detected ESBL-types (Canton et al., Reference Canton, Novais, Valverde, Machado, Peixe, Baquero and Coque2008). The genes encoding for these enzymes are termed blaTEM, blaSHV and blaCTX-M. These also represent the major ESBL-types observed in poultry and poultry products. Within the groups, there are a number of subtypes.
ESBL-producing bacteria have the ability to interchange ESBL-genes within and across species (Apata, Reference Apata2009). The Genes encoding for ESBLs are located on mobile elements (plasmids, integrons or transposons), but can also be found on the bacterial chromosome. An active transposition from plasmid to genome was suggested by Shahada et al. (Reference Shahada, Chuma, Kosugi, Kusumoto, Iwata and Akiba2013). The ESBL-genes spread vertically through cell division or by gene transfer within one species, but also horizontally to other species and genera by conjugation (Händel et al., Reference Händel, Otte, Jonker, Brul and ter Kuile2015; Yamaichi et al., Reference Yamaichi, Chao, Sasabe, Clark, Davis, Yamamoto, Mori, Kurokawa and Waldor2015; Porse et al., Reference Porse, Schonning, Munck and Sommer2016). In poultry, ESBL-genes are found on a variety of plasmid types where IncI1 and IncFIB are examples for frequently detected types (Supplementary data). Beside ESBL genes, these plasmids often carry genes that encode resistance to other antibiotics or heavy metals and are therefore often co-selected, especially when located close to each other (Silver and Phung, Reference Silver and Phung1996; Meunier et al., Reference Meunier, Jouy, Lazizzera, Kobisch and Madec2006; Liu et al., Reference Liu, Wang, Liao, Sun, Zhu, Chen and Liu2011; Seiler and Berendonk, Reference Seiler and Berendonk2012; Borjesson et al., Reference Borjesson, Bengtsson, Jernberg and Englund2013)
Recent development of ESBL in poultry
Although antimicrobial resistance is an ancient phenomenon, prevalence of ESBL-producing bacteria in poultry became significantly higher after the usage of ß-lactam antibiotics (Dierikx et al., Reference Dierikx, Van Der Goot, Smith, Kant and Mevius2013b). Besides mutations in genes encoding for ESBL, selective pressure influences other genes, promoters and the quantity of ESBL-genes enhancing the resistance against antibiotics. A change of porin proteins through mutation can e.g. alter the outer membrane permeability for antibiotics. The emergence of resistances against other groups of antibiotics can lead to co-selection and stronger promoters can increase the expression of ESBL-genes (Gniadkowski, Reference Gniadkowski2001, Reference Gniadkowski2008).
The exposure of bacteria to ß-lactam antibiotics in poultry farming through feed additives and clinical or preventive treatments leads to the elimination of sensitive strains but spares resistant bacteria. Merely these ‘persisters’ are able to multiply in the presence of ß-lactam antibiotics. They can increase tremendously due to selective pressures leading to an increase in resistance against ß-lactams (Apata, Reference Apata2009; Poole, Reference Poole2012). Furthermore, ESBL-producing bacteria may serve as a gene reservoir for other strains and species (Apata, Reference Apata2009). Another possible way for bacteria to obtain ESBL-genes is through interactions with environmental bacteria, which can hold both ancient or recently developed antibiotic resistance (Galan et al., Reference Galan, Gonzalez-Candelas, Rolain and Canton2013).
Selective pressure, due to the usage of antibiotics, may enhance the emergence and rise of antibiotic resistance. In the case of ESBL-producing bacteria, ß-lactam antibiotics can be at the root of the resistance (Dierikx et al., Reference Dierikx, Van Der Goot, Smith, Kant and Mevius2013b). Declining prevalence of ESBL-producing bacteria in broiler meat and intestinal content were observed subsequent to the ban of antibiotic growth promotors in Denmark, Sweden and the Netherlands (DANMAP, 2015; Swedress-Svarm, 2015; Veldman et al., Reference Veldman, Teeuwsen, Caccarelli, Mevius, Wit and Pelt2016). Correspondingly, the occurrence of bacteria resistant to cefotaximeFootnote 1 in fecal samples from broilers reduced significantly after a national ban on the usage of ceftiofur in Dutch hatcheries in 2010 from about 18% (2010) to under 10% (2011) (Koene et al., Reference Koene, Mevius, Dierikx, Veldman and Wit2012). These data show an immediate effect from antibiotic reduction measurements on the prevalence of antibiotic resistance. Nevertheless, there are several reports on an occurrence of ESBL-producing bacteria in broilers and their products superior to the incidence in other livestock. The high prevalence of ESBL in broilers is remarkable since many countries, such as Denmark, Belgium and Sweden, have banned the usage of cephalosporins for poultry but not for other livestock (Smet et al., Reference Smet, Martel, Persoons, Dewulf, Heyndrickx, Catry, Herman, Haesebrouck and Butaye2008; Bengtsson et al., 2012; Kameyama et al., Reference Kameyama, Chuma, Yabata, Tominaga, Iwata and Okamoto2013). This must lead to the assumption that other factors besides the usage of ß-lactam antibiotics affect the spread and prevalence of ESBL- producing bacteria in poultry.
As mentioned, antibiotic resistance against different types of antibiotics can be co-selected (Meunier et al., Reference Meunier, Jouy, Lazizzera, Kobisch and Madec2006; Liu et al., Reference Liu, Wang, Liao, Sun, Zhu, Chen and Liu2011). Examples for co-resistance against ß-lactams and heavy metals are silver and CTX-M-15 and CTX-M-14, mercury and SHV and TEM or mercury and ampicillin among others. Copper and zinc may promote multi resistant bacteria (Sutterlin et al., Reference Sutterlin, Edquist, Sandegren, Adler, Tangden, Drobni, Olsen and Melhus2014; Yazdankhah et al., Reference Yazdankhah, Rudi and Bernhoft2014; Vahjen et al., Reference Vahjen, Pietruszynska, Starke and Zentek2015). Co-resistance also exists among different antibiotic classes, i.e. ESBL-producers may hold co-resistance to fluoroquinolone, tetracycline and/or trimethoprim (Shashwati et al., Reference Shashwati, Kiran and Dhanvijay2014; Tacao et al., Reference Tacao, Moura, Correia and Henriques2014; Bajaj et al., Reference Bajaj, Kanaujia, Singh, Sharma, Kumar and Virdi2016). A high percentage (98%) of the ESBL-producing strains obtained from chicken carcasses in Brazil also expressed resistance to tetracycline (Koga et al., Reference Koga, Scandorieiro, Vespero, Oba, De Brito, De Brito, Nakazato and Kobayashi2015). ESBL-producing E. coli occurred with a high prevalence (34% in 2010 and 54% in 2011) in Swedish broiler flocks. Genes encoding for CTX-M were found on an IncI1 plasmid together with resistance against tetracycline and sulfamethoxazole, suggesting co-selection as a source for the high prevalence (Borjesson et al., Reference Borjesson, Bengtsson, Jernberg and Englund2013). Nevertheless, low usage of not only cephalosporins but also other antibiotics (0.19% of all flocks) (Bengtsson et al., 2012) must lead to the assumption that the resistance against ß-lactam antibiotics growing in poultry is also due to reasons other than antibiotic usage (Borjesson et al., Reference Borjesson, Bengtsson, Jernberg and Englund2013). Especially when compared with the usage of antibiotics in other livestock, which show significantly lower contamination rates with ESBL-producing bacteria despite higher consumption of antibiotics (Bengtsson et al., 2012; Borjesson et al., Reference Borjesson, Bengtsson, Jernberg and Englund2013). The prevalence of ESBL-producing E. coli was compared in three flocks of broilers, one treated with antibiotics (not cephalosporins), one without and one fed antibiotics and kept in laboratories, which never housed poultry before. Surprisingly, the first two flocks showed a high occurrence of ESBL, while the flocks kept in the laboratories showed much lower contamination with ESBL-producing bacteria (Hiroi et al., Reference Hiroi, Matsui, Kubo, Iida, Noda, Kanda, Sugiyama, Hara-Kudo and Ohashi2012a). This implies that the environment plays a major role, even more important than antibiotic co-selection. Correspondingly, high contamination levels with ESBL-producers were observed in the environment close to barns housing poultry (Blaak et al., Reference Blaak, Van Hoek, Hamidjaja, Van Der Plaats, Kerkhof-De Heer, De Roda Husman and Schets2015). The bacteria may spread to the environment by waste products from animal production (Apata, Reference Apata2009). The high prevalence of ESBL-producers in the environment may also explain the high prevalence of ESBL-producing bacteria in organic broiler flocks (Stuart et al., Reference Stuart, Van Den Munckhof, Voets, Scharringa, Fluit and Hall2012).
Antibiotic and heavy metal resistance are often observed simultaneously. Bacteria, which frequently carry plasmids with both genes encoding for biocide/metal resistance and antibiotic resistance genes, are Staphylococcus spp., Klebsiella spp., Salmonella spp., Enterococcus spp. and Escherichia spp. (Pal et al., Reference Pal, Bengtsson-Palme, Kristiansson and Larsson2015). The prevalence of co-resistance plasmids is significantly higher in humans and domestic animals than in other environments such as wild animals, soil, plants or food (Pal et al., Reference Pal, Bengtsson-Palme, Kristiansson and Larsson2015). ESBL-producing bacteria from a hospital environment showed co-resistance to cadmium, copper, mercury and lead, but not zinc (Touati et al., Reference Touati, Zenati, Brasme, Benallaoua and De Champs2010). Silver resistance was associated with CTX-M-15 and CTX-M-14 in humans but not in birds, while mercury resistance genes were found together with SHV and TEM genes in both human and avian samples (Sutterlin et al., Reference Sutterlin, Edquist, Sandegren, Adler, Tangden, Drobni, Olsen and Melhus2014). Hence to these common co- and cross-selection of antibiotic resistance and heavy metal resistance, it is to no surprise that mineral feed can influence the gut resistome (the gathered bacterial genetic pool of antibiotic resistance, regardless if pathogenic or not (Wright, Reference Wright2007)). High levels of zinc and copper supplementation may promote multi resistant bacteria (resistant against three or more antibiotics) in animals and animal excretions (Yazdankhah et al., Reference Yazdankhah, Rudi and Bernhoft2014; Vahjen et al., Reference Vahjen, Pietruszynska, Starke and Zentek2015). Zinc supplementation was associated with evaluated resistance against antibiotics such as ampicillin, piperacillin, doxycycline, penicillin, tetracycline and sulfonamide/trimethoprim in pigs. It also enhanced the prevalence of ESBL-producing E. coli and methicillin resistant Staphylococcus aureus, both causing difficult-to-treat infections in humans and animals (Aarestrup et al., Reference Aarestrup, Cavaco and Hasman2010; Holzel et al., Reference Holzel, Muller, Harms, Mikolajewski, Schafer, Schwaiger and Bauer2012; Bednorz et al., Reference Bednorz, Oelgeschlager, Kinnemann, Hartmann, Neumann, Pieper, Bethe, Semmler, Tedin, Schierack, Wieler and Guenther2013; Vahjen et al., Reference Vahjen, Pietruszynska, Starke and Zentek2015). Copper supplementation led to increased resistance against macrolides, glycopeptides, ampicillin, amoxicillin/clavulanic acid and piperacillin (Hasman and Aarestrup, Reference Hasman and Aarestrup2002; Holzel et al., Reference Holzel, Muller, Harms, Mikolajewski, Schafer, Schwaiger and Bauer2012). Feed supplements, which tend to reduce antibiotic resistance, are mercury (also reducing the prevalence of multi resistant bacteria) and, with less impact, lead. Co-resistance to cadmium and ß-lactams was detected irregularly in pigs (Hustavova et al., Reference Hustavova, Vozarova, Havranekova and Jackova1994; Holzel et al., Reference Holzel, Muller, Harms, Mikolajewski, Schafer, Schwaiger and Bauer2012). Nickel and chrome had no impact on observed resistance in pigs’ excretions (Holzel et al., Reference Holzel, Muller, Harms, Mikolajewski, Schafer, Schwaiger and Bauer2012). Besides from co-selection, an increased uptake of plasmids, due to mineral interaction, may be the reason for co-resistance as a consequence of mineral feed supplementation (Bednorz et al., Reference Bednorz, Oelgeschlager, Kinnemann, Hartmann, Neumann, Pieper, Bethe, Semmler, Tedin, Schierack, Wieler and Guenther2013).
ESBL-producing bacteria and resistance types in broilers
The majority of ESBL producing bacteria in poultry are E. coli and Salmonella spp. (Table 1). ESBL-producing E. coli belong to the phylogenic groups A, B1, B2, D and E (Table 2). While A and B1 are considered part of the commensal intestinal community, B2 and D are linked to pathogenic activity (Herzer et al., Reference Herzer, Inouye, Inouye and Whittam1990; Clermont et al., Reference Clermont, Bonacorsi and Bingen2000). The most frequent detected ESBL type in poultry is CTX-M (De Jong et al., Reference De Jong, Smet, Ludwig, Stephan, De Graef, Vanrobaeys and Haesebrouck2014; Tschudin-Sutter et al., Reference Tschudin-Sutter, Frei, Stephan, Hachler, Nogarth and Widmer2014; Valentin et al., Reference Valentin, Sharp, Hille, Seibt, Fischer, Pfeifer, Michael, Nickel, Schmiedel, Falgenhauer, Friese, Bauerfeind, Roesler, Imirzalioglu, Chakraborty, Helmuth, Valenza, Werner, Schwarz, Guerra, Appel, Kreienbrock and Kasbohrer2014) (Table 5). TEM and SHV are predominant in subclinical infections in poultry while TEM and CTX-M dominate samples taken from poultry with disease-associated symptoms (Olsen et al., Reference Olsen, Bisgaard, Lohren, Robineau and Christensen2014).
Table 1. Recent prevalence of ESBL in Enterobacteriaceae in poultry (2006–2011)

1 Broiler parent flocks.
2 Broiler flocks.
3 Poultry meat.
4 Laying hens.
5 Organic poultry farming.
6 Conventional poultry farming.
7 Broiler grandparent flocks poultry.
Table 2. Recent prevalence of E. coli phylogenic groups in Enterobacteriaceae in poultry (2003–2013)

ESBL producing E. coli were detected in the meconium of 1-day-old chickens showing a tendency of preservation of genotypes throughout the poultry production pyramid. This implies that ESBL genes are transmitted clonally and vertically throughout the entire poultry production pyramid even without antimicrobial selection pressure (Koene et al., Reference Koene, Mevius, Dierikx, Wit and Pelt2009; Dierikx et al., Reference Dierikx, Van Der Goot, Smith, Kant and Mevius2013b; Laube et al., Reference Laube, Friese, Von Salviati, Guerra, Kasbohrer, Kreienbrock and Roesler2013; Agerso et al., Reference Agerso, Jensen, Hasman and Pedersen2014; Olsen et al., Reference Olsen, Bisgaard, Lohren, Robineau and Christensen2014; Zurfluh et al., Reference Zurfluh, Wang, Klumpp, Nuesch-Inderbinen, Fanning and Stephan2014). In the Netherlands, ESBL-producing bacteria are suspected to be introduced to the poultry producing system through imported 1-day-old grandparent chicks. This could be a further explanation for the high prevalence of ESBL-producing bacteria in organic broiler flocks (Stuart et al., Reference Stuart, Van Den Munckhof, Voets, Scharringa, Fluit and Hall2012). The prevalence of ESBL-producing bacteria is hence much higher in 1-day-old grandparent chicks than in 1-day-old parent chicks, who in return show higher contamination than 1-day-old broiler chicks (Koene et al., Reference Koene, Mevius, Dierikx, Wit and Pelt2009; Dierikx et al., Reference Dierikx, Van Der Goot, Smith, Kant and Mevius2013b). Combined with the fact that CTX-M producing Enterobacteriaceae cause food-borne diseases, which are difficult to treat, a hazard to human health arises from poultry production even without the usage of antimicrobial feed additives. Evidence that intraspecies transmission of ESBL encoding genes takes place in Salmonella enterica and E. coli, was found (Shahada et al., Reference Shahada, Chuma, Kosugi, Kusumoto, Iwata and Akiba2013). Besides from the vertical transmission, the contaminated environment seems to be crucial for the infection of poultry. While blaCMY-2 was the only ß-lactamase gene found at the top of the poultry production pyramid, other types were found in older grandparent chickens and in 1-day old parent chicks and broilers. The same enzyme types were isolated in samples taken from the environment, suggesting this to be the source of the infection with the additional ESBL-types (Dierikx et al., Reference Dierikx, Van Der Goot, Smith, Kant and Mevius2013b).
ESBL-producing bacteria and resistance types in poultry products and their transmission to humans
Poultry meat shows the highest contamination of ESBL-producing bacteria compared with other meat sources (Friese et al., Reference Friese, Schulz, Laube, Von Salviati, Hartung and Roesler2013). Different research groups independently found a high prevalence of ESBL-producing bacteria in products from poultry while meat origin from other livestock showed significantly lower contamination. Contamination of broiler feces and objects in close contact with broiler meat reached up to 100% (Table 1). In a Danish study carried out from 2009 to 2011, poultry meat carried the highest contamination of the examined meat sources (human exposure to ESBL-producing bacteria: 83.8% from broiler meat, 12.5% from pork and 3.7% from beef) (Carmo et al., Reference Carmo, Nielsen, Da Costa and Alban2014). Equally, poultry meat was found to be contaminated with ESBL-producing Salmonella spp. to a higher extent than pork meat (De Jong et al., Reference De Jong, Smet, Ludwig, Stephan, De Graef, Vanrobaeys and Haesebrouck2014). In Germany and Switzerland, feces were collected from healthy poultry, pigs and cattle. Again, samples from broilers showed the highest contamination rate (100% respectively, 63.4%) followed by cattle (60% respectively, 13.7%) and pigs (up to 56.3% respectively, 15.3%) by ESBL-producing E. coli (Geser et al., Reference Geser, Stephan and Hachler2012; Friese et al., Reference Friese, Schulz, Laube, Von Salviati, Hartung and Roesler2013). In Japan, broilers carry the highest contamination with ESBL-producing E. coli (broilers 60.0%, laying hens 5.9%, cattle 12.5% and pigs 3.0%) (Hiroi et al., Reference Hiroi, Yamazaki, Harada, Takahashi, Iida, Noda, Yagi, Nishio, Kanda, Kawamori, Sugiyama, Masuda, Hara-Kudo and Ohashi2012b). In the Netherlands, ESBL-producing E. coli were detected in 79.8% of the examined chicken meat compared with 4.7% in beef and 1.8% in pork (Overdevest et al., Reference Overdevest, Willemsen, Rijnsburger, Eustace, Xu, Hawkey, Heck, Savelkoul, Vandenbroucke-Grauls, Van Der Zwaluw, Huijsdens and Kluytmans2011). Within poultry, broilers are contaminated with ESBL-producing bacteria to a higher extent than laying hens (Hiroi et al., Reference Hiroi, Yamazaki, Harada, Takahashi, Iida, Noda, Yagi, Nishio, Kanda, Kawamori, Sugiyama, Masuda, Hara-Kudo and Ohashi2012b; Blaak et al., Reference Blaak, Van Hoek, Hamidjaja, Van Der Plaats, Kerkhof-De Heer, De Roda Husman and Schets2015; Evers et al., Reference Evers, Blaak, Hamidjaja, De Jonge and Schets2016).
Interestingly, ESBL-producing bacteria show a high prevalence both in conventional and organic poultry farming and retail meat products (Stuart et al., Reference Stuart, Van Den Munckhof, Voets, Scharringa, Fluit and Hall2012) (Table 1). This is surprising considering the strict limitation of antibiotic usage in organic farming. However, when comparing chicken carcasses from free-ranged chicken with those of conventional raised poultry in different countries, the prevalence of extended-spectrum ß-lactamase producers depended on many factors where the production system is not decisive (Blaak et al., Reference Blaak, Van Hoek, Hamidjaja, Van Der Plaats, Kerkhof-De Heer, De Roda Husman and Schets2015; Koga et al., Reference Koga, Scandorieiro, Vespero, Oba, De Brito, De Brito, Nakazato and Kobayashi2015; Mancabelli et al., Reference Mancabelli, Ferrario, Milani, Mangifesta, Turroni, Duranti, Lugli, Viappiani, Ossiprandi, Van Sinderen and Ventura2016). Important variables, such as animal housing, feed or the size of flocks of defined poultry production systems, differ between countries. This complicates the comparison of data on the prevalence of ESBL-producers in different production systems from studies carried out in different countries.
Because products from broilers have the highest ESBL contamination among all animal products, it is not surprising that, when it comes to the ESBL transmission from livestock products to people, poultry is considered as main source. The relationship between ESBL-producing E. coli isolates obtained from poultry and humans was closer than the one between ESBL-producing E. coli from pig and human origin (Cortes et al., Reference Cortes, Blanc, Mora, Dahbi, Blanco, Blanco, Lopez, Andreu, Navarro, Alonso, Bou, Blanco and Llagostera2010). A high similarity was observed between plasmids encoding CTX-M-1 isolated from E. coli and Salmonella spp. strains of poultry origin. Conversely, they showed differences to CTX-M-1-encoding plasmids from other animal origins (Cloeckaert et al., Reference Cloeckaert, Praud, Lefevre, Doublet, Pardos, Granier, Brisabois and Weill2010). This led to the assumption that poultry might be the origin of plasmids encoding CTX-M-1.
The ability to transfer genes encoding for ESBL from an E. coli strain from poultry origin to an E. coli recipient with human origin has been frequently reported. The mechanism behind this is the transfer of plasmids by conjugation. Because these plasmids often carry additional genes encoding for other types of antibiotic resistance, they are transferred together with the resistance against ß-lactams (Meunier et al., Reference Meunier, Jouy, Lazizzera, Kobisch and Madec2006; Liu et al., Reference Liu, Wang, Liao, Sun, Zhu, Chen and Liu2011). E. coli-strains isolated from broiler feces may both proliferate and become part of the simulated human gastrointestinal tract. Simultaneously, plasmids encoding for ESBL can be transferred from the E. coli poultry strain to E. coli strains of human origin (Smet et al., Reference Smet, Rasschaert, Martel, Persoons, Dewulf, Butaye, Catry, Haesebrouck, Herman and Heyndrickx2011). CTX-M-2 was identified in Belgium and France, samples containing this enzyme were chronologically obtained first from poultry flocks, then from poultry meat and finally from humans. This led to the assumption that poultry was the source of the infection in humans (Bertrand et al., Reference Bertrand, Weill, Cloeckaert, Vrints, Mairiaux, Praud, Dierick, Wildemauve, Godard, Butaye, Imberechts, Grimont and Collard2006). Comparing ESBL-genes, plasmids and strain genotypes in E. coli from poultry, chicken meat and human sources, a close relation between isolates from human and animal origin was observed (Leverstein-Van Hall et al., Reference Leverstein-Van Hall, Dierikx, Cohen Stuart, Voets, Van Den Munckhof, Van Essen-Zandbergen, Platteel, Fluit, Van De Sande-Bruinsma, Scharinga, Bonten, Mevius and National2011). This suggests that poultry might be a reservoir for ESBL producing bacteria (Girlich et al., Reference Girlich, Poirel, Carattoli, Kempf, Lartigue, Bertini and Nordmann2007). Beside food borne infection, flies may function as a possible vector for transmission of ESBL-producing E. coli from poultry to humans (Blaak et al., Reference Blaak, Hamidjaja, Van Hoek, De Heer, De Roda Husman and Schets2014, Reference Blaak, Van Hoek, Hamidjaja, Van Der Plaats, Kerkhof-De Heer, De Roda Husman and Schets2015). A quantitative microbiological risk assessment investigated the exposure of humans to ESBL-producing E. coli originating from poultry at a worst-case scenario in 2013. Comparing chicken fillets and flies as possible sources for the transmission, the evaluation identified a higher public health risk due to ESBL-producing bacteria originating from chicken fillets than from flies (Evers et al., Reference Evers, Blaak, Hamidjaja, De Jonge and Schets2016).
Although ESBL-producing bacteria are classified as food-borne pathogens originating from livestock products, the prevalence of different ESBL types can differ between people and livestock. CTX-M-1 was found the most common ESBL type in livestock (pigs, cattle and poultry) in Germany, followed by a combination of CTX-M-1 and TEM (Valentin et al., Reference Valentin, Sharp, Hille, Seibt, Fischer, Pfeifer, Michael, Nickel, Schmiedel, Falgenhauer, Friese, Bauerfeind, Roesler, Imirzalioglu, Chakraborty, Helmuth, Valenza, Werner, Schwarz, Guerra, Appel, Kreienbrock and Kasbohrer2014). In the same study, blaCTX-M-15 was the main gene found in samples from humans. In the Netherlands, CTX-M-1 and SHV-12 were the most common types of ESBL in bacteria from both humans and poultry (Huijbers et al., Reference Huijbers, Graat, Haenen, Van Santen, Van Essen-Zandbergen, Mevius, Van Duijkeren and Van Hoek2014). Further studies found CTX-M and TEM-52 to be the predominant enzymes in ESBL-producing E. coli from both humans and poultry (Leverstein-Van Hall et al., Reference Leverstein-Van Hall, Dierikx, Cohen Stuart, Voets, Van Den Munckhof, Van Essen-Zandbergen, Platteel, Fluit, Van De Sande-Bruinsma, Scharinga, Bonten, Mevius and National2011; Overdevest et al., Reference Overdevest, Willemsen, Rijnsburger, Eustace, Xu, Hawkey, Heck, Savelkoul, Vandenbroucke-Grauls, Van Der Zwaluw, Huijsdens and Kluytmans2011). In a Dutch study, samples from humans and retail chicken meat were compared for their ESBL-producing E. coli. A high similarity in mobile resistance elements, virulence genes and genomic backbones were detected (Kluytmans et al., Reference Kluytmans, Overdevest, Willemsen, Kluytmans-Van Den Bergh, Van Der Zwaluw, Heck, Rijnsburger, Vandenbroucke-Grauls, Savelkoul, Johnston, Gordon and Johnson2013).
Whether poultry meat is the reason for human infection with ESBL-producing bacteria or only serves as a reservoir for ESBL-producing bacteria is currently under discussion. In humans, ESBL-producing bacteria are often associated with urinary-tract-infections or the nosocomial bacterial community (Dierikx et al., Reference Dierikx, Van Der Goot, Fabri, Van Essen-Zandbergen, Smith and Mevius2013a; Huijbers et al., Reference Huijbers, Graat, Haenen, Van Santen, Van Essen-Zandbergen, Mevius, Van Duijkeren and Van Hoek2014; Valentin et al., Reference Valentin, Sharp, Hille, Seibt, Fischer, Pfeifer, Michael, Nickel, Schmiedel, Falgenhauer, Friese, Bauerfeind, Roesler, Imirzalioglu, Chakraborty, Helmuth, Valenza, Werner, Schwarz, Guerra, Appel, Kreienbrock and Kasbohrer2014). A review of the infection with ESBL-producing bacteria through products of livestock origin identified poultry as a major source (Lazarus et al., Reference Lazarus, Paterson, Mollinger and Rogers2015). ESBL-producing bacteria from poultry may reach the environment with waste products, manure and excretions. They may proceed to humans through surface water, vegetables and fruits as well as when handling the animals. Poultry products, especially when treated with poor hygiene, are suggested to be another major source of human infection with ESBL-producing bacteria (Apata, Reference Apata2009).
Prevalence of ESBL types in poultry farms and products
Plasmids carrying genes encoding for ESBL often carry genes for more than one ESBL-type (Supplementary data). The prevalence of ESBL-producing bacteria in poultry and their products differs between countries, years and products, reaching from 3.3% in retail broiler meat in Denmark (2009) to 100% in conventional farmed flocks (2010) in the Netherlands. In 10 out of 14 cases, ESBL-producing bacteria were observed in more than 50% of the collected samples (Table 1). Furthermore, the prevalence of different ESBL types varies between countries, years and products (Tables 3–5). TEM-52 is the most frequently detected TEM type in poultry (Table 3). SHV-2 and SHV-12 are frequently detected in poultry. In studies comparing the occurrence of SHV-2 and SHV-12, SHV-12 positive samples are usually observed with a higher prevalence (Table 4). CTX-M is widely spread in poultry production. It occurs with high prevalence of up to 100% (UK) (Wu et al., Reference Wu, Day, Mafura, Nunez-Garcia, Fenner, Sharma, Van Essen-Zandbergen, Rodriguez, Dierikx, Kadlec, Schink, Wain, Helmuth, Guerra, Schwarz, Threlfall, Woodward, Woodford, Coldham and Mevius2013). Many different CTX-M-types have been identified in samples from poultry. CTX-M-1 demonstrates to be the most important type of CTX-M enzymes in poultry production. However, a high variation can be observed between different countries (Table 5). While there is a high prevalence for CTX-M in many European countries, it is comparably low in Japan and China. European countries, with a high prevalence for detected CTX-M-1 enzymes from poultry samples, are the UK, the Netherlands and Germany. Spain belongs to the European countries with a low CTX-M-1 prevalence. Conversely, CTX-M-2 was observed with a high prevalence in Japan (51.2%) and a low prevalence in Europe (0–9%). CTX-M-15, being the predominant CTX-M type in humans (Valentin et al., Reference Valentin, Sharp, Hille, Seibt, Fischer, Pfeifer, Michael, Nickel, Schmiedel, Falgenhauer, Friese, Bauerfeind, Roesler, Imirzalioglu, Chakraborty, Helmuth, Valenza, Werner, Schwarz, Guerra, Appel, Kreienbrock and Kasbohrer2014), shows a relatively low prevalence in poultry products. The prevalence reaches from 0% in studies from Germany, the Netherlands and Spain to 17% in a study on poultry products from the UK (Table 5).
Table 3. Recent prevalence of TEM in Enterobacteriaceae in poultry (2006–2013)

1 Organic farming.
2 Conventional farming.
3 Chicken meat.
4 Poultry.
Table 4. Recent prevalence of SHV in Enterobacteriaceae in poultry (2003–2013)

1 Chicken meat.
2 Poultry.
3 Organic farming.
4 Conventional farming.
Table 5. Recent prevalence of CTX-M in Enterobacteriaceae in poultry (2003–2013)
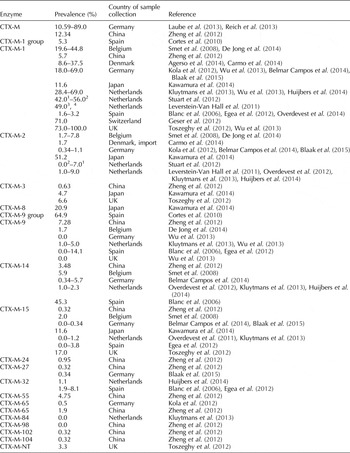
1 Conventional farming.
2 Organic farming.
3 Poultry.
4 Chicken meat.
Suggested strategies to combat ESBL in chicken
By feeding a commercial competitive exclusion product comprising a defined mixture of commercial bacteria, consisting of E. coli strains with susceptibility against antibiotics and other microorganisms, the ESBL-producing E. coli could be reduced in the cecal content of broilers (Nuotio et al., Reference Nuotio, Schneitz and Nilsson2013). To the best of our knowledge, this is the only publication about the influence of probiotics on ESBL-producing bacteria in poultry. The inhibiting effect of probiotic Bifidobacterium spp. strains on the spread of ESBL-genes was demonstrated in an in-vitro experiment and confirmed in gnotobiotic mice. While Bifidobacterium bifidum and Bifidobacterium pseudocatendatum declined SHV-5 and CTX-M-15 gene transfer from a donor to a recipient by around 3 logs, Bifidobacterium longum failed to reduce the transconjugation frequency. Hence to the constant quantities of donors and recipients, the effect was suggested to result from metabolites, inhibiting the transfer of plasmids, rather than from an antibacterial effect of the probiotic bacteria (Moubareck et al., Reference Moubareck, Lecso, Pinloche, Butel and Doucet-Populaire2007). Detection of ß-lactamases (not defined) in the feces of children treated with ceftriaxone reduced from 60% to 30–40% when treated with different mixtures of Bifidobacterium spp. and Lactobacillus spp. (B. bifidicum, Lactobacillus acidophilus and others). Other probiotics were less effective or even increased ß-lactamases (Saccharomyces boulardii and Lactobacillus cusei ssp. rhamnosus GG). Lactulose, a prebiotic, had no impact on the prevalence of ß-lactamases in the examined samples (Zoppi et al., Reference Zoppi, Cinquetti, Benini, Bonamini and Minelli2001). These results demonstrate the importance of detailed research, hence the protective capacities may differ within one species. Regardless of the urgent need of further comprehensive research on this topic, this suggests that probiotic or synbiotic feed additives may reduce antibiotic resistance in poultry production successfully. Research on the impact of feed additives, such as probiotics, prebiotics and organic acids, on the gastrointestinal bacterial community in poultry has been versatile, specific and directed (Van Immerseel et al., Reference Van Immerseel, Russell, Flythe, Gantois, Timbermont, Pasmans, Haesebrouck and Ducatelle2006; Williams, Reference Williams2010; Zalan et al., Reference Zalan, Hudacek, Stetina, Chumchalova and Halasz2010; Huyghebaert et al., Reference Huyghebaert, Ducatelle and Van Immerseel2011; Alloui et al., Reference Alloui, Szczurek and Swiatkiewicz2013). However, research on their impact on antibiotic resistant bacteria has been very sparse.
The mechanisms responsible for the antagonistic effect of probiotics towards pathogens are versatile and often strain-specific. Possible inhibitory effects on ESBL-producing bacteria are probably directed towards the bacteria, regardless the ability to produce ESBL or not. Nevertheless, antagonizing a bacterial family or genus like E. coli, which commonly harbor ESBL-genes, the prevalence of ESBL-producing bacteria might reduce subsequent to treatment with probiotics. This leads to the assumption, that antibacterial activity, competitive exclusion and the modulation of the immune system by probiotic strains may reduce ESBL-producing bacteria in broilers. Secretion of microbial substances such as organic acids, bacteriocins or hydrogen peroxide are examples for antibacterial activity. Organic acids, such as lactic acid, acetic acid and propionic acid, may contribute to a lower pH and thereby decrease the number of pathogenic bacteria (Williams, Reference Williams2010; Alloui et al., Reference Alloui, Szczurek and Swiatkiewicz2013). By rivaling for nutrition and attaching to the intestinal mucosa, probiotic strains may counteract the advancement of pathogens in the gastrointestinal tract of poultry. Lactobacilli commonly apply this mechanism of competitive exclusion against pathogens like E. coli, Klebsiella pneumoniae and Pseudomonas aeruginosa (Williams, Reference Williams2010; Alloui et al., Reference Alloui, Szczurek and Swiatkiewicz2013). Increased production of antibodies and cytokines by immune cells as well as enhanced local immune response and morphologic changes in the intestines may contribute to the antagonistic effect by probiotics, enhancing the immune system (Smith, Reference Smith2014). Treatment with lactobacilli might result in stabilized tight junctions and stimulate the expression of mucins, reducing the adherence of pathogens to the epithelial cells in the intestines. A lower permeability and an enhanced local barrier, subsequent to these morphological alterations, may antagonize the uptake of pathogens (Otte and Podolsky, Reference Otte and Podolsky2004; Doron and Gorbach, Reference Doron and Gorbach2006). Furthermore, goblet cells, liable for local defense and reparation of the epithelium, might increase in the presence of probiotics as well (Smith, Reference Smith2014). Prebiotics and synbiotics, a combination of pro- and prebiotics, may enhance the antagonistic effects of probiotics even further (Awad et al., Reference Awad, Ghareeb, Abdel-Raheem and Bohm2009; Huyghebaert et al., Reference Huyghebaert, Ducatelle and Van Immerseel2011; Alloui et al., Reference Alloui, Szczurek and Swiatkiewicz2013). As these mechanisms to combat pathogens may implement potential lethal threats on ESBL-producing bacteria the consequence to such stress must be considered (Boor, Reference Boor2006). Genes encoding for ESBL are frequently located on plasmids, which may be transferred to other Enterobacteriacea by conjugation (Händel et al., Reference Händel, Otte, Jonker, Brul and ter Kuile2015; Yamaichi et al., Reference Yamaichi, Chao, Sasabe, Clark, Davis, Yamamoto, Mori, Kurokawa and Waldor2015; Porse et al., Reference Porse, Schonning, Munck and Sommer2016). Whether the stress induced by potential reduction measurements may induce higher conjugation frequencies has yet to be investigated.
Due to the correlation between antibiotic, zinc and copper resistance, a reduction of zinc and copper contents in animal feed may help to combat the prevalence of ESBL-producing bacteria in poultry. Already, the European Food Safety Authority (EFSA) recommended lower maximum copper contents in piglet and cattle feed in August 2016 (https://www.efsa.europa.eu/en/press/news/160809a). Correspondingly, the Committee for Medicinal Products for Veterinary Use (CVMP) recommended to withdraw veterinary medicinal products containing zinc oxide from the market in December 2016 (http://www.ema.europa.eu/ema/index.jsp?curl=pages/news_and_events/news/2016/12/news_detail_002661.jsp&mid=WC0b01ac058004d5c1).
Nutrition has a major impact on the gastrointestinal composition and antibiotic resistance in people and animals. The diversity and quantity of genes encoding for antibiotic resistance obtained from intestinal bacteria from obese children decreased due to an alteration of the dominant microbial fermentation source from protein to carbohydrates. Especially mechanisms for target alteration and efflux pumps were affected (Wu et al., Reference Wu, Zhang, Wang, Zhang, Wang, Shen, Wang, Pang, Zhang, Zhao and Zhang2016).
Besides from optimizing feed and feed additives, an ESBL-free environment is necessary to keep poultry flocks free from new infections. However, this is almost impossible due to the high prevalence and spread of ESBL-producing bacteria (Hiroi et al., Reference Hiroi, Matsui, Kubo, Iida, Noda, Kanda, Sugiyama, Hara-Kudo and Ohashi2012a). Nevertheless, high biosecurity, a low number of persons entering the stables as well as thorough cleaning and disinfection, may reduce the prevalence of ESBL-producing bacteria in poultry farms. Limiting the number of chicks’ suppliers may also reduce prevalence and diversity of ESBL types in poultry farms (Mo et al., Reference Mo, Kristoffersen, Sunde, Nodtvedt and Norstrom2016). In order to avoid international carryover, traded animals must not carry ESBL-producers. Therefore, an ESBL-reduction strategy should include all levels of the poultry production pyramid to be successful (Stuart et al., Reference Stuart, Van Den Munckhof, Voets, Scharringa, Fluit and Hall2012). Producers of grandparent chicks should aim for ESBL-free flocks and regular controls.
Conclusions
CTX-M-1, TEM-52 and SHV-12 are the extended-spectrum ß-lactamases most frequently detected in poultry. The high prevalence of ESBL-producing bacteria in poultry provides a global challenge, which should be addressed with preventive reduction measurements on all levels of the poultry production system, the environment and dietary factors.
Supplementary material
The supplementary material for this article can be found at https://doi.org/10.1017/S1466252317000020.