- DC
-
dendritic cells
- NK
-
natural killer
Respiratory infections are a leading cause of mortality and morbidity in elderly people. Increased susceptibility to infections is influenced by many factors, including immunosenescence, nutritional status, poor hygiene and living conditions, medical history, medication use and stress. Reduced biodiversity, compromised stability and larger inter-individual variation in the gut microbiota are also commonly reported to be associated with ageing( Reference Claesson, Jeffery and Conde 1 , Reference Biagi, Candela and Turroni 2 ). This review examines how age-related changes in the gut can impact on the immune system, describes evidence suggesting that the gut microbiota influences the immune response to respiratory infections and evaluates whether restoration of gut microbial homoeostasis by probiotics offers an opportunity to modulate the outcome of respiratory infections and vaccination against influenza in older people.
Influence of ageing on the gut microbiota composition
Reduced biodiversity, compromised stability and larger inter-individual variation in the gut microbiota have become widely recognised as a feature of ageing( Reference Claesson, Jeffery and Conde 1 – Reference Tiihonen, Ouwehand and Rautonen 3 ). A reduction in total gene counts in faecal samples from 178 elderly subjects in long-term care compared with those in the community illustrates the reduction in gut microbiota biodiversity, which is associated with frailty, morbidity and poor nutritional status in elderly subjects( Reference Claesson, Jeffery and Conde 1 ). Also commonly reported is an age-related increase in facultative anaerobes, including streptococci, staphylococci, enterococci and enterobacteria( Reference Mueller, Saunier and Hanisch 4 – Reference Biagi, Nylund and Candela 7 ). These are sometimes referred to as ‘pathobionts’; bacteria, which are present at low concentrations in the healthy gut microbiota, but thrive in inflamed conditions and actively promote the inflammation by producing inflammatory stimuli( Reference Biagi, Nylund and Candela 7 ). It has been suggested that reduced intestinal motility contributes to a compromise in the homoeostatic equilibrium between the gut microbiota and the host immune system in older people( Reference Biagi, Candela and Turroni 2 ). However, the relationship may be reciprocal, since impairment of the gut-associated lymphoid tissue resulting in reduced production of secretory IgA, α-defensins, antimicrobial peptides and mucus may lead to failure to control the resident microbiota, facilitating dysregulated growth( Reference Biagi, Candela and Turroni 2 ). Age-related alterations in the composition of the gut microbiota can influence health through a number of mechanisms. Reduced biodiversity may result in increased colonisation of toxin-producing Clostridium difficile, a major nosocomial complication affecting older people( Reference Rupnik, Wilcox and Gerding 8 , Reference Rea, O'Sullivan and Shanahan 9 ). Excessive endotoxin production in the gut can promote inflammation( Reference Schiffrin, Morley and Donnet-Hughes 10 ) and a decrease in butyrate-producing bacterial groups may impair epithelial integrity and remove the protective, trophic and anti-inflammatory effects of butyrate from the colonic epithelium( Reference Barcenilla, Pryde and Martin 11 – Reference Macia, Thorburn and Binge 13 ).
The ageing immune system
The term ‘immunosenescence’ describes a loss of diversity in the T cell repertoire and senescence or unresponsiveness of oligoclonal T cells, resulting in poor antigen-specific cellular responses, skewing of immune effector pathways and ultimately, poor resistance to infection and response to vaccination( Reference Vallejo 14 ). Degeneration of the thymus, the site of T cell maturation, is a hallmark of ageing; by age 50–55 years, the thymus is reduced to approximately 10% of its original capacity and by age 70 years, thymic output of naïve T cells is virtually absent( Reference Vallejo 14 ). However, the number of circulating T cells in older people remains constant because of homoeostatic proliferation of both naïve and memory T cells, much of which is antigen-independent. Thymic involution, combined with increased homoeostatic proliferation and expansion of the memory compartment, dramatically reduce T cell receptor diversity. In addition, repeated antigen exposure (for example, chronic antigenic stimulation by persistent Herpes viruses), proliferation of T cells and telomere shortening lead to replicative senescence and as a result, dysfunctional cells increasingly fill the immunological space. Since a diverse T cell repertoire is essential for an effective immune response to new infections and to immunisation, older individuals face a significant challenge in dealing with both( Reference Effros 15 , Reference Kovaiou, Herndler-Brandstetter and Grubeck-Loebenstein 16 ). It is well established that ageing is associated with an increased susceptibility to infections( Reference Yager, Ahmed and Lanzer 17 ) and that vaccines are considerably less effective in older people( Reference Kovaiou, Herndler-Brandstetter and Grubeck-Loebenstein 16 , Reference McElhaney 18 , Reference Aspinall, Del Giudice and Effros 19 ), presenting a major public health challenge.
Prolonged exposure to TNF, and perhaps other inflammatory cytokines, promotes immunosenescence; indeed young individuals with chronic inflammatory disease exhibit premature immunosenescence, telomere shortening and reduced thymic output (reviewed in( Reference Vallejo 14 )). The term ‘inflamm-ageing’ has been coined to describe the now well-recognised progressive increase in chronic inflammation associated with ageing. Triggers include microbial components, tissue damage and metabolic stress, which activate inflammasome pathways and lead to the production of inflammatory cytokines and chemokines, acute phase proteins and other inflammatory mediators (Fig. 1)( Reference Calder, Ahluwalia and Albers 20 ). A number of factors have been identified to modify inflamm-ageing, including diet and the gut microbiota (Fig. 1)( Reference Calder, Ahluwalia and Albers 20 ). However, paradoxically, a shift towards a more anti-inflammatory profile is not necessarily beneficial, since up-regulation of IL-10 production suppresses interferon γ production and the expression of co-stimulatory molecules on antigen-presenting dendritic cells (DC)( Reference McElhaney, Zhou and Talbot 21 ). This results in a decrease in the interferon γ:IL-10 ratio, which is associated with impaired clearance of the influenza virus from infected lung tissue and poor response to vaccination( Reference McElhaney, Zhou and Talbot 21 ).
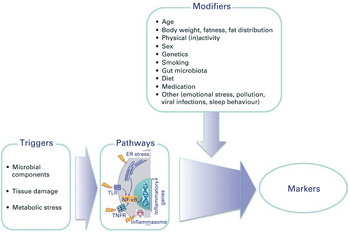
Fig. 1. (colour online) Overview of the triggers and modifiers of inflamm-ageing. ER, endoplasmic reticulum; TLR, Toll-like receptor; TNFR, TNF receptor. Reproduced, with permission, from( Reference Calder, Ahluwalia and Albers 20 ).
Respiratory infections and response to influenza vaccination in older people
Older people experience more frequent and severe respiratory infections and are at higher risk of associated complications, such as pneumonia( Reference Gavazzi and Krause 22 ). As a result, there is greater morbidity resulting from respiratory infections in elderly individuals, and influenza is a major cause of death in the over 65s( Reference McElhaney, Zhou and Talbot 21 , Reference Gavazzi and Krause 22 ). The efficacy of influenza vaccination is also significantly impaired by ageing; it is estimated that influenza vaccination protects only 17–53% of elderly individuals compared with 70–90% of young( Reference Aspinall, Del Giudice and Effros 19 , Reference Goodwin, Viboud and Simonsen 23 ). A direct relationship between poor vaccination and immunosenescence is illustrated by the fact that loss of CD28 (an important co-stimulatory molecule on T cells) is one of the most well-recognised features of immunosenescence and is correlated with a poor response to vaccination( Reference Goronzy, Fulbright and Crowson 24 – Reference McElhaney, Xie and Hager 26 ).
Probiotics, ageing and immunity
Probiotics are defined as ‘live microbial feed ingredients that, when ingested in sufficient quantities, exert health benefits on the consumer’( 27 ). Most probiotics consist of lactobacilli or bifidobacteria, but an increase in levels of these bacteria in the gut is not necessarily a health benefit in itself and other parameters need to be considered. The same applies to prebiotics, defined as ‘selectively fermented ingredients that allow specific changes, both in the composition and/or activity in the gastrointestinal microflora that confers benefits upon host well-being and health’( Reference Roberfroid 28 ) and synbiotics (a combination of the two); simply altering numbers of particular bacterial groups is not necessarily considered sufficient evidence for a health benefit. However, there is some evidence for beneficial effects of probiotics on bowel function, including infection- and antibiotic-associated diarrhoea( Reference Ritchie and Romanuk 29 ), on respiratory infection (see later) and on immune function( Reference Tiihonen, Ouwehand and Rautonen 3 , Reference Pae, Meydani and Wu 30 ). Claims relating to positive effects of probiotics on immune function have been difficult to substantiate because many of the immunological markers employed do not in themselves indicate a beneficial physiological effect( Reference Albers, Antoine and Bourdet-Sicard 31 ). Moreover, there is a degree of redundancy within the immune system, such that a reduction in the functional capacity of one component may be compensated for by another( Reference Albers, Antoine and Bourdet-Sicard 31 ). Nevertheless, there is growing evidence that probiotics have immunomodulatory properties and that these properties of probiotics are strain-dependent( Reference Haller, Blum and Bode 32 – Reference You and Yaqoob 41 ). For example, there is consistent evidence that a number of probiotics enhance natural killer (NK) cell activity in vitro ( Reference Dong, Rowland and Yaqoob 40 – Reference Lomax and Calder 43 ) and there is some supportive evidence that this also occurs in vivo ( Reference Ohashi, Nakai and Tsukamoto 44 – Reference Dong, Rowland and Thomas 46 ). Probiotic strains whose cell walls are resistant to digestion appear to be particularly potent enhancers of NK activity; it is suggested that monocytes phagocytose the probiotic bacteria and the insoluble cell wall components induce the production of IL-12, which augments NK cell activity( Reference Shida, Nanno and Nagata 38 , Reference Shida and Nanno 47 ). Animal studies suggest that this may ultimately play a role in modulation of the risk of infection (Fig. 2). For example, neonatal and infant mice administered Lactobacillus casei Shirota by stomach tube for 3 weeks prior to infection with influenza demonstrated a lower rate of accumulated symptoms, a greater survival rate and lower titres of influenza in nasal washings taken a few days after infection( Reference Yasui, Kiyoshima and Hori 48 ). These correlated with an increase in NK cell activity and production of IL-12( Reference Yasui, Kiyoshima and Hori 48 ).
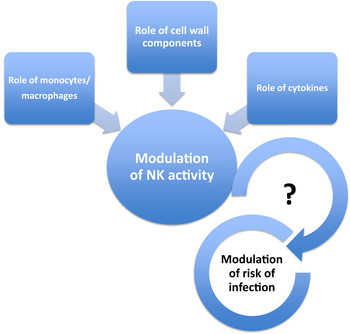
Fig. 2. (colour online) Modulation of natural killer (NK) activity and infection by probiotics. Enhancement of NK activity by probiotics is suggested to require phagocytosis of the bacteria, resulting in induction of cytokines, particularly IL-12. The mechanism involves stimulation by insoluble bacterial cell wall components. Probiotics with cells walls which are particularly resistant to digestion appear to be better inducers of IL-12 and enhancers of NK activity.
Probiotics have also been demonstrated to modulate cytokine production in a strain-dependent manner. Shida et al. ( Reference Shida, Nanno and Nagata 38 ) propose that probiotics fall into two main categories: those which are ‘immunostimulatory’, characterised by their ability to induce IL-12 and therefore to augment host defence via enhancement of NK cell activity and T helper 1 pathways, and those which are ‘immunoregulatory’, characterised by their ability to induce IL-10 and the T regulatory pathway. In general, lactobacilli tend to fall into the immunostimulatory category, whereas bifidobacteria tend to fall in the immunoregulatory category( Reference Shida, Nanno and Nagata 38 , Reference Dong, Rowland and Yaqoob 40 ). Kechaou et al. ( Reference Kechaou, Chain and Gratadoux 49 ) screened 158 bacterial strains for their ability to modulate the induction of IL-12 relative to IL-10 in TNFα-activated HT-29 cells or peripheral blood mononuclear cells and then selected one strain which was strongly pro-inflammatory, one which was neutral, and one which was strongly anti-inflammatory to test in a murine model of influenza infection. The outcome was that the most immunostimulatory strain provided the greatest protection against weight loss and symptoms( Reference Kechaou, Chain and Gratadoux 49 ).
There is particular interest in the positive influence of probiotics in older people, who are subject to alteration in gut microflora composition, as well as immunosenescence. Although probiotics have been proposed as prime candidates for ‘anti-immunosenescence’ therapy( Reference Candy, Heath and Lewis 50 , Reference Sharma, Kapila and Kapila 51 ), and ‘have the potentiality to be involved in the promotion of longevity’( Reference Biagi, Candela and Turroni 2 ), there is limited information regarding their influence on those aspects of immunity, which are particularly susceptible to immunosenescence. A study by Moro-Garcia et al. ( Reference Moro-Garcia, Alonso-Arias and Baltadjieva 52 ) demonstrated that Lactobacillus delbrueckii subsp. bulgaricus 8481 decreased the percentage of CD8+CD28− T cells and increased the proportion of naïve T cells between 3 and 6 months compared with baseline in subjects aged >65 years, although the mechanism is not clear. However, there is some direct evidence that the immune response to probiotics is significantly influenced by ageing. You and Yaqoob( Reference You and Yaqoob 41 ) demonstrated that peripheral blood mononuclear cell from older subjects (age 60–85 years) were more responsive to the immunoregulatory effects (IL-10 induction) of two strains of bifidobacteria than young subjects (age 18–30 years), whereas peripheral blood mononuclear cell from young subjects were more responsive to the immunostimulatory effects (IL-12 induction) of two strains of lactobacilli (Fig. 3). This suggests that the same probiotics might have different effects on health outcomes in young and older subjects. Further studies investigated the effects of the same four probiotics, Bifidobacterium longum bv. infantis CCUG 52486, B. longum SP 07/3, Lactobacillus rhamnosus GG and L. casei Shirota, on human DC function in an allogeneic mixed leucocyte reaction model, using DC and T cells from young and older donors in different combinations. The study demonstrated that ageing increased the responsiveness of DC to probiotics, but this was not sufficient to overcome the impact of immunosenescence in the mixed leucocyte reaction, since pretreatment of young or old DC with lipopolysaccharide or probiotics failed to enhance the proliferation of T cells derived from older donors( Reference You, Dong and Mann 53 ).
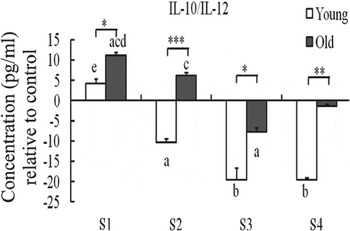
Fig. 3. Effect of probiotics on the IL-10:IL-12 ratio by human peripheral blood mononuclear cells. S1, Bifidobacterium longum bv. infantis CCUG52486; S2, B. longum SP 07/3; S3, Lactobacillus rhamnosus GG; S4, Lactobacillus casei Shirota. Data are means with standard errors for n 8 samples for each group and are relative to a medium only control. There was a significant effect of age (P < 0·001) and treatment (P < 0·001) on the IL-10:IL-12 ratio (two-way ANOVA). Significant differences are denoted as a P < 0·05, b P < 0·01 relative to the medium control for the same age group; c P < 0·05 relative to Lactobacillus rhamnosus GG for the same age group; d P < 0·05 relative to Lactobacillus casei Shirota for the same age group; e P < 0·05 relative to all the other strains for the same age group (post-hoc t tests with Bonferroni correction). Significant age differences are denoted as *P < 0·05, **P < 0·01, ***P < 0·001 for the same treatment (one-way ANOVA followed by post-hoc t tests with Bonferroni correction). Reproduced from( Reference You, Dong and Mann 53 ) with permission.
The choice of probiotic, particularly for older individuals, is a matter of debate. An interesting point of view put forward by Dominguez-Bello et al. ( Reference Dominguez-Bello, Blaser and Ley 54 ) is that attempting to improve the gut microbiota composition of older people by introducing probiotic strains characteristic of a young microbiota is not necessarily the best strategy, and it may be more appropriate to identify ‘successfully aged’ donors of probiotic strains, which might survive better in an older host and achieve a more suitable equilibrium with the resident microbiota. B. longum bv. infantis CCUG 52486 is an example of a strain which was identified to be present in particularly healthy subjects aged >90 years( Reference Silvi, Verdenelli and Orpianesi 55 ). It has subsequently been demonstrated to have particular ecological fitness and anti-pathogenic effects in vitro ( Reference Likotrafiti, Manderson and Fava 56 ) and immunomodulatory effects which are strongly influenced by the age of the host( Reference You and Yaqoob 41 , Reference You, Dong and Mann 53 ).
Probiotics and respiratory infections
Emerging evidence suggests that the resident gut microbiota plays an influential role in shaping antiviral defences and modulating the outcome of viral infections( Reference Pang and Iwasaki 57 ). Evidence from animal models indicates that germ-free mice are more susceptible to a number of infections, including influenza( Reference Mazmanian, Liu and Tzianabos 58 ), and adult mice treated with antibiotics under pathogen-free conditions before challenge with influenza have a critically impaired immune response and delayed clearance of the virus compared with those not treated with antibiotic( Reference Mazmanian, Liu and Tzianabos 58 ). The suggested mechanism is via Toll-like receptors and nod-like receptor 3, which activate the nod-like receptor 3 inflammasome in the gut, eliciting indirect stimulation of respiratory immunity( Reference Pang and Iwasaki 59 , Reference Mortaz, Adcock and Folkerts 60 ). There may be additional, as yet uncharacterised, functional links between mucosal surfaces in the gut and respiratory system. Evidence that gut-resident bacteria play a role in shaping immune defences forms the basis for the hypothesis that pre- and probiotics may modulate responses to infection or vaccination. This hypothesis is supported by a number of animal studies, which demonstrate that several probiotic strains prevent dissemination and improve clearance of pathogen in the lung, and increase phagocytic and NK activity in respiratory mononuclear cells( Reference Yasui, Kiyoshima and Hori 48 , Reference Kechaou, Chain and Gratadoux 49 , Reference Hori, Kiyoshima and Shida 61 – Reference Maruo, Gotoh and Nishimura 66 ).
Probiotic bacteria may reduce the incidence and/or severity of respiratory infections in children( Reference Hatakka, Savilahti and Ponka 67 ), adults( Reference de Vrese, Winkler and Rautenberg 68 ) and in the elderly( Reference Guillemard, Tondu and Lacoin 69 ), although evidence is limited and studies investigating prevention of common respiratory illnesses have produced mixed results( Reference Pang and Iwasaki 59 ). A recent systematic review evaluated ten randomised controlled trials with a total of 3451 participants investigating the effects of probiotics for the prevention of upper respiratory tract infections( Reference Hao, Lu and Dong 70 ). The review concluded that there was a 42% reduction in the number of participants experiencing at least one episode of acute upper respiratory tract infection, a 47% reduction in the number of participants experiencing three or more episodes of acute upper respiratory tract infection and a 33% reduction in antibiotic usage( Reference Hao, Lu and Dong 70 ). However, there was no effect on mean duration of upper respiratory tract infection and there was no data for older people, despite the fact that respiratory infections and associated secondary complications are a significant cause of death in individuals aged over 65 years. Furthermore, since ageing is associated with reduced biodiversity and compromised stability of the gut microbiota( Reference Biagi, Candela and Turroni 2 ), older individuals may benefit more from intervention with probiotics.
Probiotics and the immune response to influenza vaccination
Response to vaccination is increasingly being used as a surrogate for the response to infection and can therefore provide information on the immunomodulatory effects of dietary components, including probiotics, in human subjects( Reference MacDonald and Bell 71 ). Four out of eight of the studies investigating the impact of probiotics on responses to influenza vaccination were conducted in healthy adults( Reference Olivares, Diaz-Ropero and Sierra 72 – Reference Rizzardini, Eskesen and Calder 75 ), while the remaining four were conducted in elderly subjects( Reference Bunout, Barrera and Hirsch 76 – Reference Van Puyenbroeck, Hens and Coenen 79 ); three of these were conducted in institutionalised individuals( Reference Boge, Remigy and Vaudaine 77 – Reference Van Puyenbroeck, Hens and Coenen 79 ) (Table 1). The largest of the trials conducted in elderly subjects (n 362 and 375 on probiotic and placebo, respectively) demonstrated no effect of L. casei Shirota on respiratory infections, seroprotection, seroconversion or mean antibody titres( Reference Van Puyenbroeck, Hens and Coenen 79 ). However, a much smaller trial (n 14–19 per group)( Reference Bosch, Mendez and Perez 78 ) reported a significant increase in influenza-specific IgG and IgA in subjects consuming L. plantarum CECT7315 and CECT7316. Boge et al.,( Reference Boge, Remigy and Vaudaine 77 ) conducted an intervention trial of a probiotic drink containing Lactobacillus paracasei ssp. paracasei (Actimel®) on the response to influenza vaccination in healthy elderly volunteers (age >70 years). This trial was conducted in two phases: a pilot study in 2005–2006 (probiotic/placebo consumed for 7 weeks), followed by a confirmatory study in 2006–2007 (probiotic/placebo consumed for 13 weeks), with the inactivated influenza virus vaccine being administered during the fourth week of intervention. H1N1 was the only vaccine strain common to both phases of the study, with the H3N2 and B strains being different between vaccination seasons. In both phases of the trial, the probiotic group exhibited higher virus-specific antibody titres post-vaccination compared to the control group, although these differences were only statistically significant within the confirmatory phase( Reference Boge, Remigy and Vaudaine 77 ). The intensity of the probiotic effect was vaccine subtype-dependent, with the most pronounced enhancement for the influenza virus H3N2 strain in the pilot and the B strain in the confirmatory study. Seroconversion rates within the probiotic group in the confirmatory phase were significantly higher for the B strain at 3, 6 and 9 weeks postvaccination compared to the placebo group (P = 0·02), but there was no effect of the probiotic on seroconversion for the H1N1 or H3N2 strains( Reference Boge, Remigy and Vaudaine 77 ). It is perhaps pertinent to note that the B strain is known to show major human variability, and the effects on this subtype therefore need to be interpreted with caution. Bunout et al.,( Reference Bunout, Barrera and Hirsch 76 ) examined the effects of a complete nutritional formula containing a range of nutrients and vitamins plus the probiotic Lactobacillus paracasei (NCC 2461) and the prebiotic fructo-oligosaccharide for 6 months on the response to influenza and pneumococcal vaccines (given at 4 months) in free-living Chilean subjects aged over 70 years. At 12 months there was a significantly lower incidence of infection, in particular, respiratory infection, in the treatment group compared with the control group, but there was no effect on antibody responses to either vaccine( Reference Bunout, Barrera and Hirsch 76 ). A fifth study in elderly subjects is not included in Table 1 because of an unusual study design, which makes the data very difficult to interpret( Reference Namba, Hatano and Yaeshima 80 ). In this small study, twenty-seven elderly subjects consumed a test food containing B. longum BB536 for 5 weeks, with an influenza vaccination (2004/2005 campaign) being given at 3 weeks. At 5 weeks, the subjects were then randomised to either continue on the probiotic, or to consume a placebo for a further 14 weeks. The randomisation was stratified for sex and H3N2 titres, but not for overall protection, so that the proportion of subjects with effective titres was 53·8% in the BB536 group and 28·6% in the placebo group( Reference Namba, Hatano and Yaeshima 80 ). Although the paper reports significantly lower incidence of influenza and fever in the probiotic group, the subject numbers are extremely small, and this data needs to be interpreted with caution. Thus, it is too early to draw any conclusions regarding the potential influence of probiotics on the response to influenza vaccination in elderly subjects; more research is required.
Table 1. Studies investigating the effects of probiotics on the response to influenza vaccination
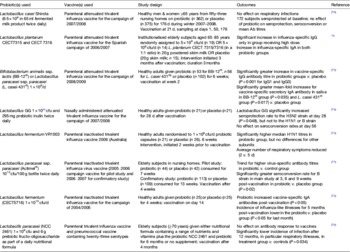
In healthy adults, Bifidobacterium animalis ssp. lactis (BB-12®) or Lactobacillus paracasei ssp. paracasei (L. casei 431®) taken for 6 weeks increased influenza vaccine-specific serum IgG and vaccine-specific salivary sIgA titres after vaccination at 2 weeks in the 2008/2009 campaign( Reference Rizzardini, Eskesen and Calder 75 ). There was no effect of either probiotic on vaccine-specific serum IgA or IgM on plasma cytokine concentrations, or on parameters of innate immunity, although the authors acknowledge that sampling at 4 weeks after vaccination may have missed changes in immune response to the vaccine( Reference Rizzardini, Eskesen and Calder 75 ). Lactobacillus GG taken for 28 d immediately after receiving a nasally administered trivalent live attenuated influenza vaccine from the campaign of 2007/2008 significantly increased seroprotection (haemagglutinin inhibition antibody titre = 40) to the H3N2 virus strain, but not to the H1N1 or B strain at day 28( Reference Davidson, Fiorino and Snydman 74 ). However, at day 56 the rates of seroconversion (at least a fourfold rise in HAI antibody titre) were not significantly different. French & Penny( Reference French and Penny 73 ) reported significantly higher antibody titres to H1N1 following intervention with Lactobacillus fermentum VRI 003 for 6 weeks in total (vaccination after 2 weeks), but there were no differences for other subunits. The average number of days of respiratory symptoms was reduced from 5 to 2 d( Reference French and Penny 73 ). Lactobacillus fermentum CECT5716, taken for 4 weeks, significantly increased titres of influenza virus-specific plasma IgA (but not IgM or IgG) to the inactivated trivalent influenza vaccine for the vaccine campaign of 2004/2005, administered 2 weeks into the intervention( Reference Olivares, Diaz-Ropero and Sierra 72 ). Additionally, the incidence of influenza-like illnesses for 5 months post-vaccination were lower in the probiotic group compared to the control group( Reference Olivares, Diaz-Ropero and Sierra 72 ). Overall, there is some evidence for adjuvant effects of probiotics in influenza vaccination, but this is currently limited, most of the studies are very small, and there may be strain-specific differences which need to be considered( Reference Dong, Rowland and Yaqoob 40 , Reference Shida and Nanno 47 ). In selecting probiotics with potential adjuvant activity specifically targeted towards older individuals, Dominguez-Bello et al. ( Reference Dominguez-Bello, Blaser and Ley 54 ) suggest that ‘the healthy old rather than the healthy young are the best donors of probiotic species for old individuals’. This argues against the common perception that modulating the microbiota composition of an aged gut to resemble that of a younger individual will be beneficial for intestinal health and immunity. Instead, it is suggested that the aim should be to establish a new equilibrium with a suitably aged microbial community in an older human host( Reference Dominguez-Bello, Blaser and Ley 54 ).
Future perspective
In their recent review, Kau et al. ( Reference Kau, Ahern and Griffin 81 ) propose that ‘the time is right and the need is great to understand better the relationships between diet, nutritional status, the immune system and microbial ecology in humans at different stages of life.’ They suggest that a combination of metagenomics methods for describing the gut microbiome and the use of gnotobiotics (rearing of animals under germ-free conditions with subsequent exposure to single species) represents a potentially powerful approach to examine relationships between nutritional status and gut microbial communities. It may also provide a mechanistic understanding of the influence of the gut microbiota on the immune system that could be used to inform the design and execution of human studies. One of the challenges in this area is that the effects of probiotics on human health may be strain-specific and, as this review illustrates, dependent on the age of the host. Hypothesis-led research is therefore critical in evaluating the potential use of probiotics in respiratory infections and vaccination in the ageing population.
Acknowledgements
Some of the work described in this review was funded by a Dorothy Hodgkin postgraduate award in association with Yakult UK, and by a research grant (no. BBH00470X/1) from the Biotechnology and Biological Sciences Research Council Diet and Health Research Industry Club (BBSRC-DRINC).
Financial support
P. Y. has received research funding for some of the work described in this review from Yakult UK and from the Biotechnology and Biological Sciences Research Council Diet and Health Research Industry Club (BBSRC-DRINC).
Conflicts of interest
None.