1. Introduction
Human societies are changing the atmospheric water cycle – evaporation, moisture transport, precipitation – via land-use change, climate change, air pollution, and more recently also via weather modification. Land-use changes directly affect evaporation from land and include everything from replacing tropical forests with rangelands, to irrigating deserts, and converting grasslands to monocultures (Gasparri et al., Reference Gasparri, Kuemmerle, Meyfroidt, le Polain de Waroux and and Kreft2016; Lambin et al., Reference Lambin, Turner, Geist, Agbola, Angelsen, Bruce, Coomes, Dirzo, Fischer, Folke, George, Homewood, Imbernon, Leemans, Li, Moran, Mortimore, Ramakrishnan, Richards and Xu2001; Lambin & Meyfroidt, Reference Lambin and Meyfroidt2011). Climate change influences precipitation patterns profoundly, and increasing air pollution, aerosols in particular, affects atmospheric physics including cloud formation and precipitation (Allan et al., Reference Allan, Barlow, Byrne, Cherchi, Douville, Fowler, Gan, Pendergrass, Rosenfeld, Swann, Wilcox and Zolina2020; Ramanathan et al., Reference Ramanathan, Crutzen, Kiehl and Rosenfeld2001; Rosenfeld et al., Reference Rosenfeld, Lohmann, Raga, O’Dowd, Kulmala, Fuzzi, Reissell and Andreae2008). These modifications can have significant consequences for the timing, magnitude, and duration of evaporation delivery to the atmosphere, and the fate of precipitation elsewhere (Bagley et al., Reference Bagley, Desai, Dirmeyer and Foley2012; Keys et al., Reference Keys, Wang-Erlandsson and Gordon2016; Mahmood et al., Reference Mahmood, Pielke, Hubbard, Niyogi, Dirmeyer, McAlpine, Carleton, Hale, Gameda, Beltrán-Przekurat, Baker, McNider, Legates, Shepherd, Du, Blanken, Frauenfeld, Nair and Fall2014; Staal et al., Reference Staal, Tuinenburg, Bosmans, Holmgren, van Nes, Scheffer, Zemp and Dekker2018; Swann et al., Reference Swann, Longo, Knox, Lee and Moorcroft2015; Tuinenburg et al., Reference Tuinenburg, Hutjes, Stacke, Wiltshire and Lucas-Picher2014; Wang-Erlandsson et al., Reference Wang-Erlandsson, Fetzer, Keys, van der Ent, Savenije and Gordon2018, Reference Wang-Erlandsson, Tobian, van der Ent, Fetzer, te Wierik, Porkka, Staal, Jaramillo, Dahlmann, Singh, Greve, Gerten, Keys, Gleeson, Cornell, Steffen, Bai and Rockström2022; Wei et al., Reference Wei, Dirmeyer, Wisser, Bosilovich and Mocko2012; Zemp et al., Reference Zemp, Schleussner, Barbosa, Hirota, Montade, Sampaio, Staal, Wang-Erlandsson and Rammig2017). Given the actual, and potential, changes globally, we conceptualize these flows of atmospheric water – including the delivery of evaporation, the flow of water vapor, and eventual precipitation – as economic goods.
Historically, flows of atmospheric water could implicitly be considered ‘public goods’ since evaporation flows and atmospheric water vapor were generally neither consumed (rival) nor controlled (exclusive) (Farley & Costanza, Reference Farley and Costanza2010). However, given that people can change this relationship via land-use change, atmospheric water may transition into a ‘common-pool’ good (rival and non-excludable; Rockström et al., Reference Rockström, Mazzucato, Andersen, Fahrländer and Gerten2023), and possibly to a controlled ‘club’ good (non-rival and excludable). Now, with the advent of scientifically verifiable cloud seeding, specifically via recent work on wintertime orographic cloud seeding (French et al., Reference French, Friedrich, Tessendorf, Rauber, Geerts, Rasmussen, Xue, Kunkel and Blestrud2018; Tessendorf et al., Reference Tessendorf, Boe, Geerts, Manton, Parkinson and Rasmussen2015, Reference Tessendorf, French, Friedrich, Geerts, Rauber, Rasmussen, Xue, Ikeda, Blestrud, Kunkel, Parkinson, Snider, Aikins, Faber, Majewski, Grasmick, Bergmaier, Janiszeski, Springer and Bruintjes2019), deliberate atmospheric water manipulation may accelerate in its deployment, in the near future. Along with scientific advancements in the understanding of fog harvesting (Qadir et al., Reference Qadir, Jiménez, Farnum, Dodson and Smakhtin2018; Tu et al., Reference Tu, Wang, Zhang and Wang2018), it is now conceivable for atmospheric moisture to enter the domain of ‘private good’ (i.e. excludable and rival). Yet, there has been no documented effort to seriously consider the implications or future pathways of anthropogenic modification of the atmospheric water cycle, despite the far reaching implications for society (Keys et al., Reference Keys, Wang-Erlandsson, Gordon, Galaz and Ebbesson2017; te Wierik et al., Reference te Wierik, Gupta, Cammeraat and Artzy-Randrup2020). Therefore, we explore the possible implications that could arise if atmospheric water transitions away from a public good, to become a common-pool, club, or private good. In doing so, we aim to identify new pathways forward that could better anticipate and support research and policy needs.
Scenarios are a commonly used tool for exploring future trajectories of science, policy, and culture (Carpenter et al., Reference Carpenter, Bennett and Peterson2006), yet scenarios can vary widely in terms of approach, style, and depth of detail (Kishita et al., Reference Kishita, Hara, Uwasu and Umeda2016). Scenario narratives are commonly used to provide both qualitative interpretations of observed or model-based findings, and to facilitate stakeholder engagement (Carpenter et al., Reference Carpenter, Bennett and Peterson2006; O'Neill et al., Reference O'Neill, Kriegler, Ebi, Kemp-Benedict, Riahi, Rothman, van Ruijven, van Vuuren, Birkmann, Kok, Levy and Solecki2017; Raskin, Reference Raskin2005). Importantly though, scenario narratives are often only descriptions – not stories – of possible future worlds. While such descriptions can be creative, they often fail to foster student curiosity, engage the public, or interest policymakers (Milkoreit, Reference Milkoreit2017). Story-based scenarios have emerged as successful vehicles for impactful scenarios (Calvert, Reference Calvert2019; Johnson & Winkelman, Reference Johnson and Winkelman2017; Merrie et al., Reference Merrie, Keys, Metian and Österblom2018; Nature, 2018) given the ways that stories and storytelling can allow for sense- and meaning-making, reveal assumptions or hidden biases, and create alternate worlds (Bentz et al., Reference Bentz, O'Brien and Scoville-Simonds2022; Riedy, Reference Riedy, Molthan-Hill, Luna, Hall, Puntha and Baden2020). Story-based scenarios represent a deeper exploration of future worlds (Carbonell et al., Reference Carbonell, Sánchez-Esguevillas and Carro2017), and allow participants to explore how their daily lives, values, and habits can be mapped onto different projections of the future.
Despite ongoing recognition of the various ways in which anthropogenic changes actively modify the atmospheric water cycle (Gordon et al., Reference Gordon, Steffen, Jönsson, Folke, Falkenmark and Johannessen2005; Keys et al., Reference Keys, Wang-Erlandsson and Gordon2016; Kleidon, Reference Kleidon2006; Schumacher et al., Reference Schumacher, Keune, Dirmeyer and Miralles2022; Wang-Erlandsson et al., Reference Wang-Erlandsson, Fetzer, Keys, van der Ent, Savenije and Gordon2018), there remain gaps in mainstream water policy, nationally and internationally, for managing changes to the atmospheric water cycle via land-use change (Keys & Wang-Erlandsson, Reference Keys and Wang-Erlandsson2018; te Wierik et al., Reference Te Wierik, Gupta, Cammeraat and Artzy-Randrup2020). The main international policies that do exist guide the use of precipitation enhancement (e.g. to avoid hostile actions) and have existed for decades. The Convention on the Prohibition of Military or Any Other Hostile Use of Environmental Modification Techniques, popularly known as ENMOD, was intended to ban weather modification for warfare (Bonnheim, Reference Bonnheim2010; Wunsch, Reference Wunsch1980). However, weather modification, specifically precipitation enhancement that is not for hostile use, is typically managed on a national basis. For example, in the United States any activities seeking to modify weather must submit a report of their activities to the National Oceanographic and Atmospheric Administration (Charak & DiGiulian, Reference Charak and DiGiulian1974; Simon, Reference Simon2021) and China passed its first national law on weather modification in 2002 (Chien et al., Reference Chien, Hong and Lin2017). Nevertheless, there is no global policy for guiding human actions specifically regarding changes in atmospheric moisture and the effects on the overall global hydrological cycle. Chen (Reference Chen2016) explored the opportunity for updated property rights framings to be incorporated for atmospheric moisture in the United States, and that legal interpretations of atmospheric moisture have led to communal, that is, governmental, rights to management rather than individual or private rights. However, this discussion does not include anthropogenic modification of evaporation, and the associated delivery of moisture to the atmosphere. Thus, there is a gap in the scientific understanding of the potentially diverse sources of weather modification, and the associated policy discourse. This gap between scientific understanding and policy is, in part, due to the lack of framing terrestrial moisture recycling and atmospheric water manipulation in terms that are salient to policy (Keys et al., Reference Keys, Wang-Erlandsson, Gordon, Galaz and Ebbesson2017). Our study responds to this gap by framing this topic in policy terms that are directly related to ownership and control.
2. Conceptual development
2.1 Rivalry and excludability for atmospheric water
In economics, the concept of ‘goods’ (e.g. timber, theater tickets, parking spaces) can be categorized into four quadrants based on how use and control of goods affects their status. First, if the use of a good reduces its available supply for others, it is called rival, while if use does not reduce availability it is considered non-rival (Kaul et al., Reference Kaul, Grunberg and Stern1999). Second, if a good can be intentionally or unintentionally controlled such that others can be prevented from access, it can be considered excludable, while if such control is not possible it is considered non-excludable (Kaul et al., Reference Kaul, Grunberg and Stern1999). This classification produces a four quadrant space, including: ‘public’ goods (non-rival, non-excludable), ‘common-pool’ goods (rival, non-excludable), ‘club’ goods (non-rival, excludable), and ‘private’ goods (rival, excludable) (Figure 1). Many natural resources are considered ‘public’ goods, especially spatially extensive phenomena such as clouds, air, timber, or even ice caps. This classification is appropriate, human intervention being absent. However, there are now few if any actual ‘public’ goods remaining, given the pervasive and widespread anthropogenic impacts on temperature, ocean heat budgets, and other planetary changes (Steffen et al., Reference Steffen, Broadgate, Deutsch, Gaffney and Ludwig2015) that drive and perpetuate unequal access to ecosystem services within societies and across generations (Hamann et al., Reference Hamann, Berry, Chaigneau, Curry, Heilmayr, Henriksson, Hentati-Sundberg, Jina, Lindkvist, Lopez-Maldonado, Nieminen, Piaggio, Qiu, Schill, Shepon, Tilman, van den Bijgaart and Wu2018; Thiery et al., Reference Thiery, Lange, Rogelj, Schleussner, Gudmundsson, Seneviratne, Andrijevic, Frieler, Emanuel, Geiger, Bresch, Zhao, Willner, Büchner, Volkholz, Bauer, Chang, Ciais, Dury and Wada2021).

Figure 1. Conceptual overview of atmospheric water as an economic good. The horizontal axis indicates excludability of a resource. The vertical axis indicates whether the resource is rivalrous. Within each, there is a brief detail of the key requirement for atmospheric water to exist in that domain.
We classify atmospheric water into the four quadrants, based on how humans modify rivalry and excludability (Figure 1). For atmospheric water to be categorized as a ‘public’ good, anthropogenic activities that could modify atmospheric water would need to steward atmospheric water availability elsewhere in an undirected way. If anthropogenic activities change the quantity of moisture that is available elsewhere, then atmospheric water moves toward becoming a ‘common-pool’ resource. If anthropogenic activities exert control over the distribution of moisture but do not change the availability of moisture, then atmospheric water moves toward a ‘club’ good. Finally, if anthropogenic activities modify both the quantity and exert control over atmospheric water, then it moves toward a ‘private’ good.
The core objective of this study is to introduce the conceptual exploration of atmospheric water as an economic good, with story-based scenarios of how such human modifications of the atmospheric water cycle may unfold in the future. To do this we blended multiple methods, incorporating computational text analysis, expert participation, and creative science fiction storytelling. Our paper is organized as follows. First, we will explain our methods, which blend a structured literature search, with computational text analysis, with expert training and collaboration, and storytelling. We then interpret these story-based scenarios in the context of improving our understanding of the future of human modification of the atmospheric water cycle. Further, we discuss both policy and research frontiers that need to be explored given the findings of this scenario exercise.
3. Methods
There were five key steps in this research, which we highlight here and explain in detail below. First, a structured literature search was performed, and we collected a large set, or corpus, of scholarly abstracts on the topic of human modification of the atmospheric water cycle. Second, we employed a machine learning-based computational text analysis (called latent Dirichlet allocation, LDA), which is a method for revealing the latent topics and keywords that exist across a corpus of documents. Third, the topics and keywords were mapped to the economic goods framework, based on a subjective interpretation of each topic's keywords. Fourth, we used the results of the LDA to inform a structured futuring exercise among a group of global water experts. This collaborative process involved guiding the experts through a set of creative exercises to develop imaginative visions of future worlds, where humans are (or are not) modifying the atmospheric water cycle. Fifth, a subset of the expert collaborators took these imaginative worlds, and were guided through a creative story-telling process. The results of this study are ten story-based scenarios. We provide a detailed explanation of each step below.
3.1 Structured literature search
The first task was to create a structured literature query to collect scholarly abstracts related to human modification of the atmospheric water cycle (Table 1). After a preliminary exploration of various search terms, phrases, and exclusions, we used the search query below, which was intended to maximize the literature collected, while avoiding false returns. We exclusively used the Web of Science search function, which permits a sophisticated search query and automated download process for collecting scholarly abstracts. Throughout the query, we used asterisks (*), which allowed a word to be completed by numerous suffixes, for example, ‘human*’ indicates that it would include ‘human’, ‘humanity’, ‘humans’, or ‘humanities’. Similarly, metadata about the abstracts was downloaded in a tabular format permitting easy cross referencing. The query is represented in Table 1, indicating that these words and phrases would need to be present in any of the searchable fields within Web of Science, including the article title, keywords, abstract, or other searchable text.
Table 1. Words and phrases that comprised the structured literature query in Web of Science

Using the table of metadata, we performed a manual quality control to ensure that the abstracts included the necessary relevant information, specifically the full text of the abstract, and were, for example, not erroneously blank. Additionally, the quality control checks involved identifying whether a given document was associated with an abstract, searching for duplicate abstracts, and manually searching for abstracts that were not automatically retrieved with the Web of Science search. These abstracts (i.e. documents) were then used in our computational text analysis forming the corpus of analyzed literature.
The abstracts collected in the structured literature search above were prepared for the computational text analysis by batch converting them into plain text format, so that they would be machine readable. Similarly, additional information, such as author or other watermarks, were removed from the text so that only the abstract content was included in each document.
3.2 Latent Dirichlet allocation
The computational text analysis was performed using Python-based software including the natural language toolkit, the GENSIM package, and other common computational packages. The specific Python software versions used for the analysis were Python v3.7.7, Natural Language Toolkit (i.e. NLTK), v3.4.4, and Gensim Python package v3.8.0. Following the methods of Keys and Meyer (Reference Keys and Meyer2022), the LDA was performed sequentially including: pre-processing the documents (including tokenization, stopword removal, and lemmatization), sensitivity testing to identify the most suitable number of topics for the LDA, and visualization of the final text analysis. First, during tokenization each word across all documents in the corpus was converted into an individual unit (i.e. token) of analysis (e.g. ‘all of these words’ becomes four tokens ‘all’, ‘of’, ‘these’, ‘words’). Second, stopwords, which are words that appear in texts but convey little to no information (e.g. ‘I’, ‘we’, ‘and’, ‘as’, ‘the’, etc.), were removed. Third, words were lemmatized, which means similar words were collapsed into a common form (e.g. ‘connections’ becomes ‘connection’). This process allowed a greater number of unique tokens to be represented in the analysis.
LDA was a process of iteratively identifying the underlying topic structure across the entire corpus of documents. First, a target number of topics was assigned for the analysis (e.g. 15 topics). Then, the LDA was performed aiming to find the most representative set of 15 topics (with the corresponding keywords). Thus, by varying the number of topics, a different set of topics and keywords could have been revealed. Several methods exist to find the most statistically robust set of topics, such as ‘coherence’. The idea of ‘coherence’ refers to a statistical representation of how each topic makes interpretable sense for a machine, such that the keywords included within each topic have some common meaning or connection. The coherence metric c_v (Röder et al., Reference Röder, Both and Hinneburg2015) was used in a sensitivity analysis to identify the most statistically robust number of topics, by iteratively varying the number of topics in the LDA from 5 to 35. Then, we identified the top three topics with the highest coherence scores, to determine whether the resultant topics and keywords were interpretable. To do this, the keywords corresponding to each topic for each LDA analysis were manually examined for interpretable coherence by the lead author. This primarily involved identifying whether keywords within a cluster exhibited some common characteristics, and that the clusters themselves were distinct from one another.
Finally, if the keywords for a topic represented <1% of total terms across the entire corpus, then that topic was removed from the analysis. Topics with low term representation were not coherent enough for the story-based scenario process (Keys & Meyer, Reference Keys and Meyer2022).
3.3 Map themes onto economic goods framework
The topics produced from the text analysis were interpreted based on the semantic content of the keywords. This interpretation was then mapped onto the economic goods quadrants, as a way to connect the text analysis explicitly to the economic goods classification. This process was subjective and led by the lead author of this study. Multiple interpretations of this mapping are possible, and we discuss the implications of this in Section 5.4.
3.4 Expert participatory science fiction prototyping
The next step was to work with a set of global water experts to collaboratively interpret the thematic output of the computational text analysis and systematically develop science fiction prototypes. Water experts were recruited from a group of scientists, scholars, and practitioners with broad expertise on global water topics who attended a virtual water resilience symposium hosted by the Stockholm Resilience Centre. A series of two, virtual workshops (February 22, 2022 and March 3, 2022) were convened to train the water experts in story-based scenario development, specifically a workshop on worldbuilding using futures methods (e.g. science fiction prototyping), and a workshop on storytelling. The lead author of this article prepared the materials and conducted the workshops for the participants.
3.5 Workshop no. 1: worldbuilding
Worldbuilding is defined as ‘the creation of imaginary worlds with coherent geographic, social, cultural, and other features’ (von Stackelberg & McDowell, Reference von Stackelberg and McDowell2015). The expert participants were led in individual, guided exercises that began with each participant reviewing a specific topic and its associated keywords. Participants were assigned an economic good quadrant, which provided some constraints to the worldbuilding while also ensuring each of the quadrants was examined by at least some participants. Participants were then prompted to visualize a future beyond 2050 that was inspired by the keywords and topic. After taking notes on these visualizations each participant was led in a structured Futures Wheels exercise (Pereira et al., Reference Pereira, Hichert, Hamann, Preiser and Biggs2018). Futures Wheels permit an exploration of direct interactions and consequences, flowing from a focal event or item in the future (Hamann et al., Reference Hamann, Biggs, Pereira and Ziervogel2020).
After the individual exercises, groups were formed for each economic good quadrant, with two participants each representing public good and private good and three participants each representing club good and common pool. Each group was then guided through three exercises: (1) three horizons framework; (2) probe reality; and (3) make-it-weird. The three horizons framework is a structured exercise to systematically describe historical transitions from the present status quo, through a transition period toward a futuristic transformed third horizon (Sharpe et al., Reference Sharpe, Hodgson, Leicester, Lyon and Fazey2016). The first, second, and third horizons are often characterized as paradigms that shift in dominance over time. The three horizons exercise involves putting the visualized futures (e.g. from the Futures Wheels exercise) at the end of the third horizon and describing the first and second horizons. After the three horizons exercise, participants were encouraged to ‘Probe reality’ and discuss what broader cultural forces might shape the broader future world. To do this, we employed the heuristic of the ‘cultural iceberg’ (Hall, Reference Hall1989), which is used to reflect on deeper, less visible aspects of culture. Lastly, groups were encouraged to make it weird by pushing their ideas to ridiculous domains (Dator, Reference Dator1993). This included probing social norms, technological surprises, and more.
3.6 Workshop no. 2: storytelling
This second virtual workshop focused on guiding participants through the storytelling process, namely designing a character for a story and then systematically drafting a plot. For the character design section, participants were encouraged to imagine a character that would inhabit their world from the first workshop. Then, progressive activities focused on defining internal and external characteristics, motivations of what make the character act, obstacles that are relevant to their character, and thinking about the wants of a character versus what they might need for a satisfying story arc. In the second part of the workshop, participants designed a plot set in their world that their characters would navigate (Keys & Meyer, Reference Keys and Meyer2022). The exercises here were built around identifying ‘Story beats’, specifically:
• Act I
◦ Once upon a time…
◦ Every day…
◦ Until one day…
• Act II
◦ Because of this…
◦ Because of that…
• Act III
◦ Until finally…
◦ Ever since then…
The story beats were nominally split into three acts, as a way to distribute action across a given story. This structure provided a basic scaffold on which a story could be built that would allow a character to navigate the created world, and work through obstacles to ultimately reveal something about the particular economic goods quadrant. Following the two workshops, participants were invited to contribute scenarios based on their workshop output. These scenarios were collaboratively edited by different members of the author team, with all stories edited by the lead author. We note that there was an explicit emphasis on creativity and strangeness in the development of the story-based scenarios. An implication of this is limited control of bias or subjectivity. In the particular context of creative and generative scenario development, this is viewed as both necessary and an advantage for exploring novel possibilities.
4. Results
4.1 Literature query
The structured web query returned 2573 abstracts. Following quality control checks, 2274 articles remained.
4.2 Latent Dirichlet allocation
Based on the coherence metric c_v, we identified LDA models with topics numbering 11, 15, and 17 as good candidates for our analysis (Supplementary Figure 1). Ultimately, while 11 topics yielded the highest coherence value, we selected 17 topics as the most suitable LDA model given it had the highest (subjective) interpretability for its words and topics. We also adjusted the pyLDAvis ‘relevance metric’ slider to 0.6, which corresponds to the recommended value for maximum novelty and coherence in a given topic analysis (Sievert & Shirley, Reference Sievert and Shirley2014).
The overall topic visualization is shown in Figure 2, and shows the distribution of the 17 topics, the terms (i.e. keywords) corresponding to the overall corpus, and the representativeness of these terms across the corpus. We ended up excluding topics 13–17 because they each represented less than 1% of terms in the corpus, and had quite low interpretability. While this removal could have led to missing some weakly represented ideas in the dataset, we considered this appropriate given that the remaining topics covered a substantial range of disciplines and ideas. A full set of visualizations for topics 1–12 are available in the Supplementary pyLDAvis figures.

Figure 2. Visualization of topic distribution (left), and full list of keywords for the overall topic analysis (right). This visualization was created using the pyLDAvis Python package. Note that visualizations for each individual topic are included in the Supplementary material.
A filtering of topics 1–12 was performed by the lead author, to ensure the keywords formed interpretable clusters. Several topics were obviously focused on issues that were only tangentially connected to the topics we aimed to better understand in the scenario and storytelling work. Ultimately, of the 12 topics, topic 2 was removed (focused on carbon uptake, and nutrient loss, but not on atmospheric water) and topic 10 was removed (focused on organic sediment and river discharge, but not atmospheric water). Thus, we were left with ten total topics to be explored in the workshops and structured futuring (Table 2; the full table of all topics and keywords is available in the Supplementary material).
4.3 Map themes onto economic goods framework
Next, we took the remaining topics and mapped them directly onto the economic goods framing (Figure 3). This step allowed for a direct connection of certain topics and keywords to be explicitly explored in the context of particular economic goods framings. For each topic, the keywords were considered how and where they might exist in the spectrum of excludability and rivalry. For example, topic 11 included keywords that refer to cloud microphysics, aircraft, cloud condensation nuclei (CCN), mountains, and ice. All of these would be relevant for glaciogenic cloud seeding, that is, the deliberate enhancement of wintertime precipitation. Thus, this topic and keywords were assigned to the ‘private good’ quadrant. We note here that our classification is but one of many potential classifications. An opportunity exists to classify the topics into different quadrants, which would eventually lead to different types of story-based scenarios, which we explore in Section 5 (Table 3).
Table 3. Summary of scenario story names, economic good quadrant, and corresponding descriptions of worlds and story plots

4.4 Expert workshops overview
The first workshop included ten participants, with specific activities for each workshop participant to complete individually, and then as a group. The disciplinary backgrounds included hydrometeorology, land-use change, urban water infrastructure, comparative governance, water resources management, and ecology. Both the first and second workshops were conducted virtually, which enabled broad geographic collaboration. The second workshop included five participants, and was explicitly focused on story-based development informed by the results from the first workshop.
During the first workshop, the participants first worked individually using exercises including a guided visualization and a Futures Wheels exercise (an example of a Futures Wheels diagram for the ‘private good’ category is shown in Supplementary Figure S2). Second, participants were then grouped together based on each of the four economic goods quadrants, and collaboratively participated in exercises using the three horizons framework (Supplementary Figure S3), the cultural iceberg (Supplementary Figure S4), and the ‘make-it-weird’ framework (Supplementary Figure S5). During the second workshop, five of the original ten participants were led through a set of guided exercises, and produced outlines of characters and plots for their scenario worlds that they designed in the first workshop.
4.5 Collaborative scenario writing
Ten story-based scenarios were generated that are representative of the original ten topics identified in the computational text analysis. The full set of stories is available in the Supplementary material. The lead author provided editing and guidance for all of the scenarios. The final scenarios are short, written stories ranging in length from approximately 1000 to 2000 words and include a range of forms including oratory, journal entries, internal reflections, third-person narrative, conversations and dialogues, and a podcast transcript. Similarly, the scenarios are set on all populated continents, in Myanmar, India, Australia, United States, Brazil, Nigeria, Germany, and China.
4.6 Scenarios illustrate strange possibilities
Based on the final story output, we analyze the patterns and the possibilities that illustrate important aspects of the future of human modification of the atmospheric water. First, geophysical realities, such as proximity to coastlines, may confer some resistance of atmospheric moisture becoming anything other than a public good, given both the constant replenishment (i.e. non-rival) and limited potential for control (i.e. non-excludable) of oceanic sources of moisture. Second, technology change could lead to surprising social and legal problems related to transboundary decision making and liability related to manipulation of weather. Third, climate changes will modulate every aspect of human society, and thus lead to an ever-broadening incentive to control and manage precipitation. Fourth, social norms that are radically different from the present day may emerge from transformations caused by increasingly frequent Anthropocene crises. We summarized key characteristics of these scenarios in Table 4.
Table 4. Comparison of scenario characteristics among economic goods quadrants

The scenarios allowed us to explore relationships among water-related sectors, as well as draw connections among disciplines and topics that are unconnected to water science. Table 5 includes excerpts from four of the ten scenarios that were developed in this study. The public good quadrant is represented by an excerpt from ‘The Palash’. The excerpt references how historical weather modification led to Earth system consequences (e.g. transformation of South Asian monsoon dynamics) and was later globally banned as a result. The story explicitly mentions human rights consequences that could arise resulting from human modification of the atmospheric water cycle. This ‘assault’ led to atmospheric water being recognized and protected as a public good.
Table 5. Brief excerpts from the story scenarios that discuss aspects relevant to the economic goods framing
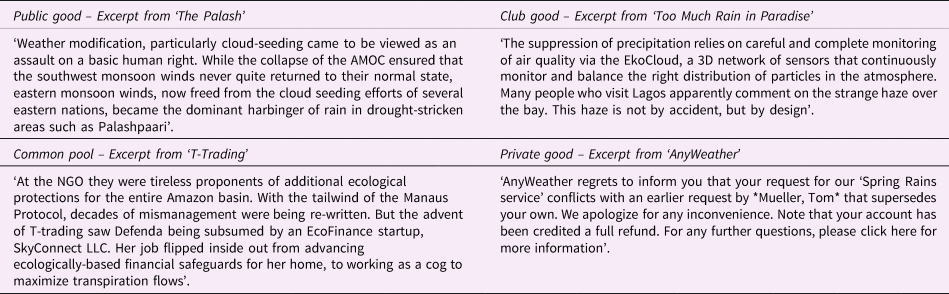
The common-pool quadrant includes an excerpt from the scenario ‘T-Trading’. The excerpt mentions how a comprehensive conservation and preservation effort for the Amazon led to a different perspective on how to profit from the intact forest cover. This story explores unexpected implications of successful conservation efforts, such as the financialization of the Amazonian atmospheric water cycle. While financialization is already discussed in the contexts of deforestation and commodities in the Amazon (Galaz et al., Reference Galaz, Crona, Dauriach, Jouffray, Österblom and Fichtner2018), financialization of the atmospheric water cycle is a new topic.
The club good quadrant is represented by ‘Too much rain in paradise’, and the excerpt highlights the role of weather modification in community-scale efforts to avoid flooding or unwanted rain. This story could be viewed as a technologically advanced descendent of existing weather modification efforts that seek clear skies, particularly efforts throughout China (Bluemling et al., Reference Bluemling, Kim and Biermann2020). The story also draws connections among new topics, including advances in drone-based, three-dimensional monitoring of atmospheric conditions as well as the manipulation of conditions with the continuous management (and curation) of desired atmospheric aerosol conditions.
The private good quadrant is represented by ‘AnyWeather’, and the excerpt highlights how weather services are now ‘on demand’, similar to other delivery services. While this requires non-existent technological advancements in microscale weather modification, the story is especially about the implications of pay-to-play services in the context of competing user interests. In this case, the story explores how a for-profit company might create different types of solutions for managing competing requests, including letting those with more money have greater levels of priority for their desired weather.
5. Discussion
5.1 Improved anticipatory capacity
The scenarios depict a range of social, technological, and physical changes – many of which seem implausible or even impossible in the present. Yet, climate change, together with technological progress, is rapidly rendering the impossible, possible; and the unimaginable, a current event (Pettit et al., Reference Pettit, Wild, Alley, Muto, Truffer, Bevan, Bassis, Crawford, Scambos and Benn2021; Samenow & Patel, Reference Samenow and Patel2022). Thus, prudence dictates that we take seriously the highly consequential ideas depicted in these scenarios. In this vein, we recommend several relevant anticipatory practices that also apply to water policy governance:
(1) Anticipate changes in regulatory regimes that may be needed at multiple levels for atmospheric water modification.
(2) Anticipate substantial improvements in the diagnostic and monitoring capability of atmospheric moisture, precipitation, and cloud microphysics and the risks of unequal access.
(3) Anticipate overlapping crises, that relate to atmospheric water flows in surprising ways, and that will motivate unexpected social, economic, and geopolitical surprises.
(4) Anticipate technological surprises that are disruptive, transformative, and seemingly impossible in the present, which will change what is plausible regarding modification of atmospheric water flows.
(5) Anticipate changes in power dynamics (e.g. geopolitical, social, cultural), that could lead to new configurations of who is able to exert disproportionate control over atmospheric water flows.
Intentionally adopting these and perhaps other anticipatory approaches, will improve the responsiveness of both science and policy toward addressing a rapidly changing human relationship with the atmospheric water cycle.
5.2 Implications for the governance of atmospheric water
Another important dimension of thinking about this study is how existing governance and management of atmospheric water fit into this futures-oriented discussion. Existing research has discussed the broad outlines of atmospheric water governance (Keys et al., Reference Keys, Wang-Erlandsson, Gordon, Galaz and Ebbesson2017; te Wierik et al., Reference te Wierik, Gupta, Cammeraat and Artzy-Randrup2020) and others have discussed manipulation of the weather more broadly (Bonnheim, Reference Bonnheim2010; Chien et al., Reference Chien, Hong and Lin2017). Depending on the types of technologies that are deployed and the spatial scales over which atmospheric water changes operate, the administrative responsibility could shift. For small-scale situations, as depicted in the scenarios ‘AnyWeather’ and ‘Helping Heaven’ (where weather conditions are manipulated on a local basis), it could be that regulations remain a national issue, and governance need not interact with transboundary discussions. Indeed, there are some contemporary organizations which seek to directly modify regional evapotranspiration to moisture recycling patterns, despite the absence of transboundary governance mechanisms (Rose, Reference Rose2021). However, scenarios that involve large-scale transpiration modification, such as ‘T-Trading’ and ‘The Fertilizer Incident’ (which depict very large-scale modifications of land-use and corresponding large changes to evaporation and transpiration) would necessarily involve transboundary negotiations. The scenario ‘T-Trading’ specifically lists several international treaties and agreements which create the operational guardrails for financial instruments to emerge that manage transpiration. While fictional, this is analogous to other traded commodities that are regulated (to varying extents) at national and international levels (e.g. staple food crops, housing) (Clapp & Helleiner, Reference Clapp and Helleiner2012; Smith, Reference Smith2010). Future research could advance the understanding of both practical examples of analogous governance structures that would be relevant for different quadrants of the economic goods framing, as well as to describe theoretical governance structures that could meet an as-yet non-existent activity (e.g. what types of regulatory schemes would be necessary to govern Transpiration Futures trading?).
5.3 Limitations
This is an initial exploration of atmospheric water as an economic good, specifically focusing on how humans are modifying this water globally. Given that we have aimed to be brief in the introduction regarding the conceptual framing, there is a substantial discussion that should yet be had regarding the economic goods classification. This discourse, however, is likely to be most fruitful when examined from multiple disciplinary perspectives, as well as inter- and trans-disciplinary perspectives. In this study, we have aimed to introduce the concept, and highlight how a specific approach (i.e. hybrid futures methods blending computational text analysis and expert creativity) can advance our perception of key issues. In this way, our study should be viewed as a horizon scanning exercise that can begin to enumerate the ideas, pitfalls, and potential challenges associated with thinking about human modification of the atmospheric water cycle.
The mapping of topics and keywords from the LDA onto the economic goods quadrants was conducted prior to the expert workshops by the lead author, and we emphasize that different subjective interpretations of this mapping could lead to different creative outcomes. Future efforts could explore the implications of different subjective mappings, which could lead to entirely different types of story scenarios being developed. Similarly, future research could aim to develop an objective procedure for interpretation of the LDA results into an economic goods framework. Yet, given that the entire research effort aims to embrace creativity, it may be more worthwhile to lean into the opportunity for numerous creative interpretations of the empirical LDA results to inform options for more flexible and responsive policy and governance environments.
Also, while the group of experts collaborating in this study is highly interdisciplinary (with expertise spanning resource economics, engineering, urban water systems, environmental humanities, biodiversity conservation, ecohydrology, and more), there are yet more disciplines that could be engaged. In particular, it could be worthwhile to engage weather modification personnel and practitioners to think about the future of their industry to better understand the perspective of ‘insiders’ for this topic.
5.4 Research frontiers
Given that this is among the first efforts to discuss human modification of atmospheric water using an economic goods framing (Keys, Reference Keys2021), there is much yet to do to understand its dimensions, critique the framing, and deploy empirical tools to test hypotheses. First, there is already considerable scholarship surrounding water as an economic good more broadly (Rogers et al., Reference Rogers, De Silva and Bhatia2002; Savenije, Reference Savenije2001; Savenije & van der Zaag, Reference Savenije and van der Zaag2002), and these ideas could be brought to bear more fully to think about atmospheric water specifically. This could include reflection from similar types of ‘source–sink’ relationships such as watersheds that incorporate upstream and downstream ideas into their study (Jansson et al., Reference Jansson, Folke, Rockström, Gordon and Falkenmark1999), as well as the payment for ecosystem services literature (Jack et al., Reference Jack, Kousky and Sims2008). Second, the notion of water as an economic good presupposes that concepts such as ‘valuation’ and other ideas that enable management and control are tenable in the discourse of atmospheric water (Jenerette et al., Reference Jenerette, Marussich and Newell2006; Schaubroeck et al., Reference Schaubroeck, Deckmyn, Giot, Campioli, Vanpoucke, Verheyen, Rugani, Achten, Verbeeck, Dewulf and Muys2016). Future research ought to more deeply engage other topics that have managed to examine valuation of diffuse economic goods, such as air quality (Levinson, Reference Levinson2012), or more broadly through cultural ecosystem services (Sukhdev, Reference Sukhdev2009).
Third, a tremendous amount of opportunity exists to use these scenarios as springboards for empirical analysis. This could take many forms and span numerous disciplines, such as mechanical engineering, ecohydrological modeling, theoretical economics, and weather modeling. We provide a snapshot of questions that emerged from discussion among the group, which could guide future research and policy into aspects of human modification of the atmospheric water cycle:
• What are the theoretical limits of land-based weather modification? What is the theoretical maximum evaporative contribution of the terrestrial biosphere? How does this vary around the planet? Are there biological restrictions to evapotranspiration that could be lifted with genetic modification? What are the barriers to this type of change?
• What are the technological limits of drone technology and its use in weather modification? How small could drones theoretically be constructed, while carrying a payload? What types of payloads could drones carry that are relevant to the weather modification conversation, including aerosols, CCN, etc.?
• What are the smallest scales of weather modification? What are the smallest spatial- and time-scales at which weather could be modified? What prevailing regional and large-scale weather conditions would be more or less permissible for this type of micro-manipulation?
• What types of financial instruments could emerge to manage the flow of atmospheric water flows? Are there existing financial mechanisms (e.g. trade rules, stock exchange listing agreements) which indirectly manage atmospheric moisture? Could there ever be a ‘futures’ or ‘options’ market to modify atmospheric water flows?
6. Conclusions
Humanity is transforming the atmospheric water cycle not least through climate change and large-scale land-use change. The advent of scientifically verifiable cloud seeding may accelerate the uptake of atmospheric water manipulation in the near future to an even larger extent. Moreover, scientific policies, legal frameworks, and governance strategies will need to anticipate this expanded manipulation and its effects on further altering the global hydrological cycle. Thus, in this study we have introduced an economic goods framework for classifying the types of human modification of the atmospheric water cycle including atmospheric water as a public good, a club good, a common-pool resource, and a private good. Moreover, we combined computational text analysis and collaborative expert visioning, to create ten story-based scenarios of the future. Specifically, this blending of objective and subjective approaches provided a rapid method for efficiently summarizing a large amount of information for a creative scenario workshop.
Our scenarios depict both familiar and strange futures. These include detailed worlds with characters that explore issues such as an Amazon basin dominated not by deforestation, but by Transpiration Futures trading; privatized on demand weather that can be called up via an app; transformed drylands to provide continental moisture bridges; and, international efforts coordinating the management of the atmospheric water commons. Our approach effectively blended a broad literature survey into a rapid, expert-driven scenario development process that resulted in ten creative visions of the future. To be very clear, these scenarios are not meant to be prognostic. They are, instead, intended to provide a clearer perspective on present-day scientific and social changes that require outside-the-box thinking. As the world changes around us, these scenarios can help ignite new policy questions and lead to novel scientific inquiry to better understand the future of humanity's modification of the atmospheric water cycle.
Supplementary material
The supplementary material for this article can be found at https://doi.org/10.1017/sus.2024.9.
Acknowledgments
The authors acknowledge the facilitation provided by the Stockholm Resilience Centre during the ‘Water resilience workshop’ held in February 2022, and the contribution of Dr. E.H. Krueger during the workshop.
Author contributions
P. W. K. led this study, including conceptualization, data curation, formal analysis, investigation, methodology, project administration, visualization, and writing. L. W.-E. contributed to conceptualization, investigation, and writing. M.-L. M., A. P., F. S., O. V., R. W., and R. B. W. all contributed to investigation and writing. C. F. and P. D. contributed to the investigation.
Funding statement
L. W.-E. and A. P. are funded by FORMAS, project number 2019-01220. F. S. is funded by the Global Challenges Foundation via Future Earth. C. F. is funded by the Beijer Foundation and the Marianne and Marcus Wallenberg Foundation.
Competing interests
None.