Diabetic nephropathy (DN) is one of the major microvascular complications of diabetes mellitus, which affects 30–40% of diabetic patients. It can lead to end-stage renal disease and reduce the quality of life(Reference Gross, De Azevedo and Silveiro1). Increased levels of serum creatinine and urinary albumin excretion are the most significant clinical manifestations of DN(Reference Reutens and Atkins2). The pathophysiology of DN is not understood exactly; however, hyperglycaemia is an important event that develops structural and functional changes such as glomerular hyperfiltration, epithelial hypertrophy and microalbuminuria(Reference Soldatos and Cooper3).
Oxidative stress and inflammation are considered as core factors to be involved in the pathophysiology of DN. In fact, disturbance in the metabolism of glucose, overload of glucose and excessive influx of energy substrates into the mitochondria can lead to increased oxidative stress and inflammation(Reference Duran-Salgado and Rubio-Guerra4). Hyperglycaemia motivates the secretion of angiotensin-II, which arouse haemodynamic, trophic, inflammatory and pro-fibrogenic reactions in the kidneys. Factors involved in the hyperfiltration injury are vascular endothelial growth factor and cytokines such as transforming growth factor-beta (TGF-β)(Reference Wolf, Butzmann and Wenzel5). Previous studies demonstrated that an excess amounts of reactive oxygen species could activate protein kinase C, mitogen-activated protein kinases and transcription factors all of which could lead to kidney fibrosis(Reference Kashihara, Haruna and K Kondeti6). In addition, free radicals modulate the secretion of inflammatory cytokines and thickening of the basal membrane glomerulus(Reference Donate-Correa, Martín-Núñez and Muros-de-Fuentes7).
Regarding the factors involved in the pathophysiology of this complication, it seems that a multi-dimensional treatment is needed for the management of renal function in DN patients. Therefore, dietary intake as an important factor should also be considered in patients with malfunctioning kidneys. In this regard, functional foods and herbal medicine have attracted much attention, as adjunctive treatments to standard drugs(Reference Birch and Bonwick8).
Abelmoschus esculentus L. (Okra) is a yearly plant from Malvaceae or mallow family(Reference De Rosa, Kenny and Puglia9). In traditional medicine, okra was used as an appetite motivator, cooling, anti-diabetic, diuretic and antispasmodic(Reference Roy, Shrivastava and Mandal10,Reference Islam11) . Due to the compounds contained in okra such as bioactive polysaccharides, soluble and non-soluble fibres, antioxidant ingredients and abscisic acid, it is considered a functional food(Reference Dantas, Alonso Buriti and Florentino12). The beneficial effects of okra on oxidative stress, inflammation and blood glucose may be attributed to its antioxidant compounds, abscisic acid, bioactive polysaccharides and fibres. For instance, abscisic acid is a phytohormone in the okra with hypoglycaemic effects in the body(Reference Daliu, Annunziata and Tenore13). Recently, studies on animal models showed that okra extract significantly reduced fasting blood glucose (FBG) and improved the antioxidant enzymes in diabetic rats(Reference Tian, Miao and Zhang14–Reference Sabitha, Ramachandran and Naveen16). Also, another study on type 2 diabetes model mice indicated that okra extract could protect renal function, inhibit the harm of high blood glucose to the kidneys and suppress oxidative stress, as well as inflammation(Reference Liao, Zhang and Wang17). In this regard, it was reported that okra supplementation had beneficial effects on glutathione peroxidase, catalase and TNF-α (Reference Majd, Azizian and Tabandeh18,Reference Freitas, do Val and Fernandes19) . Additionally, some studies showed that okra was effective on the management of blood glucose and oxidative stress in diabetic patients(Reference Tian, Miao and Zhang14,Reference Moradi, Tarrahi and Ghasempour20–Reference Saatchi, Aghamohammadzadeh and Beheshtirouy22) . It was also demonstrated that okra powder had a significant effect in reducing the level of FBG, insulin(Reference Moradi, Tarrahi and Ghasempour20,Reference Saatchi, Aghamohammadzadeh and Beheshtirouy22) and HbA1c(Reference Saatchi, Aghamohammadzadeh and Beheshtirouy22) in diabetic patients. Moreover, in a clinical trial on diabetic patients, it was illustrated that okra fruit could decline the level of FBG(Reference Haryati23).
To the best of our knowledge, although there are numerous in vitro or in vivo studies and few clinical trials that evaluated the effect of okra on metabolic indices in diabetes mellitus, no clinical trial has been conducted to consider the effects of dried okra fruit extract (DOE) on hyperglycaemia, kidney function, inflammation and gene expression in patients with DN; therefore, the present randomised controlled trial aimed to investigate the effect of DOE on glycaemic control, renal function, inflammation as well as the expression of PPAR-α, PPAR-γ, TGF-β and Nrf-2 genes in DN patients.
Materials and methods
Participants
DN patients were enrolled in this randomised, triple-blind, placebo-controlled clinical trial, performed between June 2020 and September 2021 in Tabriz, Iran. DN patients were recruited through public announcement, as well as diabetes clinic of Imam Reza hospital. Type 2 diabetic patients with BMI between 25 and 38 kg/m2, having urinary excretion of protein greater than 0·3 g/24 h or a eGFR of at least 45 ml/min per 1·73 m2, but lower than 60 ml/min per 1·73 m² for more than 3 months, and aged between 40 and 70 years were eligible to enter the study. In addition, they had to take anti-diabetic medications for at least 1 year before recruitment and were on stable therapy (i.e. no changes in type or dose of medications) with oral anti-diabetics, angiotensin receptor blockers or angiotensin converting enzyme inhibitor and anti-hypertensive or anti-dyslipidaemia medications for 3 months or more before the trial. Other renal disorders, liver diseases, thyroid or cardiovascular disorders, malignancies, diabetic patients with HbA1c more than 8 mg/dl, use of any anticoagulants and non-steroidal anti-inflammatory drugs, alcohol consumption, smoking, pregnancy or breast-feeding, following unusual diets at least 6 months before the screening or energy consumption of less than 800 kcal or more than 4200 kcal per day, routine consumption of okra in the last 3 months before the trial and allergy to okra were regarded as exclusion criteria. The number of patients estimated for each group was 25 with a power of 80 % and α = 0·05, to detect a difference of 25 mg/dl in the mean value of FBG concentration as a primary outcome with a pooled standard deviation obtained from the study of Saatchi et al. (Reference Saatchi, Aghamohammadzadeh and Beheshtirouy22), according to a formula. Given a 25 % dropout rate, especially because of COVID-19 pandemic, the sample size was increased to thirty-two patients for each group. The primary outcome of this trial was changes in FBG. The secondary outcomes were changes in the expression of PPAR-α, PPAR-γ, TGF-β and Nrf-2 genes in the peripheral blood mononuclear cells (PBMC), inflammatory indices and renal function indices.
Study design
This study was conducted according to the guidelines laid down in the Declaration of Helsinki, and the protocol of the present study was approved by the Ethics Committee of Tabriz University of Medical Sciences (IR.TBZMED.REC.1399·466) and registered at the Iranian registry of clinical trials (IRCT20110530006652N3). At the onset of the trial, the procedure of the trial was explained to each patient and written informed consent was obtained from all participants. The patients were randomly entered either in the DOE or placebo group, according to the block randomisation, stratified by sex (using 15, StataCorp LLC). The patients in the intervention group received one 125-mg capsule of DOE (containing 80 mg DOE, 3·2 mg Avicel and 0·8 mg magnesium stearate), and the placebo group took one 125-mg capsule of carboxymethyl cellulose (containing 87 mg carboxymethyl cellulose) for 10 weeks. Due to the high viscosity of okra extract, Mg stearate was used to increase the dissolution of the extract and Avicel as filler was added to capsules(Reference Paul and Sun24). In addition, all participants received a list of dietary DN recommendations. DOE and carboxymethyl cellulose capsules were similar in shape, colour, taste, smell and size. The bottles of the capsules were encoded as A or B before the study by another person who was not involved in the study. The identity of the capsules and bottles was kept confidential until the statistical analysis was finalised. So, a person outside the clinical team put up the capsules in numbered containers on the basis of random list. Furthermore, an independent person who did not know the type of supplements, randomly assigned the patients to the DOE and placebo group, thus the allocation was concealed from the researchers.
The participants, researchers and statistician were blinded, until the end of the study. The investigator followed the patients every 2 weeks by phone call to be ensured of either the supplement use or any possible side effects. Further, a bottle of DOE or carboxymethyl cellulose containing twenty-four capsules was given to each patient every 3 weeks and they were requested to return the bottle in the next visit to check out the number of – if any – unused capsules. Patients who consumed less than 90 % of the capsules of each bottle were excluded from the trial(Reference Hartman, Lems and Boers25).
Preparation and extraction of plant materials
The dosage of DOE was estimated based on the data of a trial, which demonstrated that the administration of 4 g of dried okra powder had beneficial effects on glycaemic control in patients with type 2 diabetes mellitus(Reference Saatchi, Aghamohammadzadeh and Beheshtirouy22). We performed the following steps for an accurate estimation of the amount of fresh okra fruit required. Initially, we considered thirty-two patients in the intervention group who needed to receive 4 g of dried okra powder for 10 weeks. Therefore, we needed approximately 9000 g of dried okra powder for the extraction and preparation of DOE. In our pilot study, we noticed that 1 kg of fresh okra fruit (from Karaj, Iran) could be converted to 100 g of dried okra powder. As a result, at least 90 kg fresh okra fruit was needed. After the fresh okra fruit was purchased, it was washed, chopped and air-dried. The powder of dried okra was mixed with 50 % ethanol with continuous shaking overnight (24 h) to prepare a crude extract, which equalled to 900 g. Next, okra extract was filtered and concentrated at reduced pressure, using a rotary evaporator and freeze dryer to achieve the crude dried okra extract (DOE), which was nearly 180 g. Finally, capsules of 125 mg (each containing 80 mg DOE, 4 % Avicel and 1 % magnesium stearate) were prepared.
Measurement of sodium and potassium content in DOE supplements
Na and k are the main elements in okra pods. Each 100 g of pod contains 127·6 mg of Na and 337·32 mg of K, and these elements constitute about 17 % of the seed content of this plant(Reference Gemede, Ratta and Haki26,Reference Romdhane, Chahdoura and Barros27) . Due to the limitation of Na and K intake in DN patients, the amount of Na and K in each capsule of DOE was measured using Flame-photometer.
Assessment of anthropometric measures and physical activity
The weight of the participants was measured while they were without shoes and wearing light clothes using a Seca digital scale with 100 g precision (Seca). The height of the patients was assessed in the stand position and barefoot using a metre attached to the wall with 0·1 cm precision. BMI of the subjects was calculated using the following formula: weight (kg)/height (m)2. BMI between range of 18·5–25 kg/m2, 25–30 kg/m2 and higher than 30 kg/m2, respectively, considered as normal, overweight and obese. Physical activity of the patients was evaluated by the International Physical Activity Questionnaire short form (IPAQ-SF)(Reference Vasheghani-Farahani, Tahmasbi and Asheri28). Physical activity of all participants was reported as metabolic equivalent (MET). One MET is the amount of oxygen consumed in the sitting position at rest and is equal to 3·5 ml O2 per kg body weight × min. Based on the instruction of IPAQ, participants who had less than 600 MET/min/week, at least 600 MET/min/week and at least 3000 MET/min/week were scored as low level, moderate level and high level of physical activity, respectively(Reference Moghaddam, Aghdam and Jafarabadi29).
Measurment of biochemical parameters
Five millilitres of venous blood samples was collected at baseline (1 d before beginning consumption of capsules), as well as the end of the trial (1–3 d after taking the last capsule of last bottle) subsequent to 10–12-h fasting. Blood samples were centrifuged at 2500 rpm for 10 min to separate the serum samples for measurement of glycaemic variables and high-sensitivity C-reactive protein (hs-CRP(. Fresh whole blood samples were used for isolation of PBMC and RT-PCR. FBG was measured by the enzymatic colorimetric method, using commercial kits (Pars Azmoon Co.). Serum insulin concentration was assessed, using an ELISA kit (Monobind). HbA1c level was measured by chromatography method (Biorex fars). Homoeostatic model assessment for insulin resistance (HOMA-IR) was calculated by the following formula: HOMA-IR = [fasting insulin (µIU/ml) × fasting glucose (mg/dl)]/405(Reference Klishadi, Khosravi and Famouri30). In the present study, hs-CRP concentration was measured by the Immunoturbidimetric method (CardioPhase hsCRP kit, Siemens Healthcare Diagnostics Products GmbH). Furthermore, at the beginning and end of the 10-week trial, the patients were requested to collect their urine for 24 h in the special container given to them for the assessment of protein and creatinine. In the current study, 24-h urinary protein was assessed by turbidity measurement method and 24-h urinary creatinine was measured by Jaffe’s method. CKD-EPI equation was used to calculate eGFR with the following formula:
eGFR = 141 × Min (serum creatinine/kappa, 1)alpha × Max (serum creatinine/kappa, 1).209 × 0·993Age × Sex
Sex: for females = 1·018, alpha = −0·329, kappa = 0·7
Sex: for males = 1, alpha = −0·411, kappa = 0·9
Peripheral blood mononuclear cells and RNA isolation
Immediately after collection of the blood samples in the vials containing EDTA, PBMC were extracted using Ficoll-Histopaque solution gradient centrifugation protocol (Ficoll-Paque, GmbH). Total RNA was isolated using the Ambion Trizol LS reagent (Thermo Fisher Scientific), according to the manufacturer’s protocol. The quality and concentration of extracted RNA were determined using NanoDrop spectrophotometry (NanoDrop Onec, Thermo Scientific). Then, complementary DNA was synthesised through RNA isolated using oligo (dT), random hexamer primer and RT, based on the manufacturer’s instructions (BioFact™ RTase). RNA integrity was determined by gel electrophoresis on a 1 % standard agarose gel.
Real-time PCR
The RT-PCR technique (Roche LightCycler®) was used to determine the genes expression level of PPAR-α, PPAR-γ, TGF-β and Nrf-2 genes. SYBR Green Master mix (Thermo Fisher Scientific) was used for quantitative RT-PCR. The primer sequence of the genes was provided from Primer bank. The primers of PPAR-α, PPAR-γ, TGF-β, Nrf-2 and glyceraldehyde-3-phosphate dehydrogenase genes are presented in Table 1. The amounts of the mRNA were normalised according to the glyceraldehyde-3-phosphate dehydrogenase transcription level, as an internal reference gene, using ΔΔCT comparative method. Fold change of the genes expression was determined by 2–ΔΔCT equation.
Table 1. Sequence of genes primers
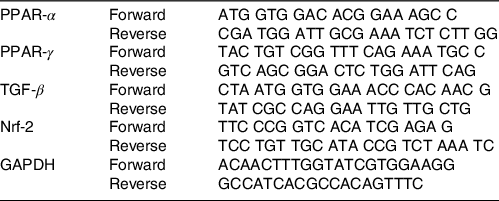
TGF-β, transforming growth factor beta; Nrf-2, nuclear factor erythroid 2-related factor 2; GAPDH, glyceraldehyde 3-phosphate dehydrogenase.
Statistical analysis
Statistical analysis was conducted using IBM SPSS statistics software (IBM SPSS Statistics, version 21) and STATA (StataCorp, version 16), based on both approaches including per-protocol and intention-to-treat. Missing values were imputed via multiple imputation procedures. The normality distribution of data was evaluated using Kolmogorov–Smirnov test, along with graphical methods. Data were reported as mean values and their standard deviations for numerical data and median (25th, 75th) for data with non-normal distribution. The evaluation of differences within groups was assessed using paired samples t test and Wilcoxon signed-rank test for normal and non-normal data, respectively. Between-group comparisons were performed using independent samples t test or Mann–Whitney U test, as appropriate. To adjust the effect of confounders, ANCOVA was applied for normally distributed data. However, quantile regression test was used to control the effect of confounding factors for values with non-normal distribution. All of the variables were analysed based on two models; however, third model was applied just for hs-CRP and kidney function variables. The models were as follows: (model 1): baseline values; (model 2): baseline values, age, changes in BMI, energy intake and physical activity; (model 3 for hs-CRP): baseline values, age, changes in BMI, energy intake, physical activity and change in HbA1c; (model 3 for urinary protein and creatinine excretion): baseline values, age, changes in BMI, energy intake, physical activity and changes in AGE. P-values less than 0·05 were considered as statistically significant levels.
Results
Of thirty-two patients who entered each group, thirty patients in the DOE group and twenty-five patients in the placebo group completed the study. The flow chart of the study is presented in Fig. 1. In the DOE group, one patient was infected with COVID-19, and another subject did not complete the trial for personal reasons. In the placebo group, five patients discontinued the trial due to the affliction with COVID-19 and two patients cut for personal reasons. Overall, except within-group changes of HOMA-IR, there were no significant differences between the findings of per-protocol, as well as intention-to-treat approaches performed. The outcomes of intention-to-treat approach are presented in the Supplementary file (Tables 2, 3 and 4). Statistical analysis did not show any significant difference in general characteristics between the two groups (Table 2). The mean age of the patients in both groups was over 60 years, most of whom were overweight. According to Table 2, fourteen and thirteen patients in the DOE and placebo groups, respectively, received oral hypoglycaemic agents, anti-hypertensive drugs, and anti-dyslipidaemic drugs. In addition, sixteen and twelve participants in the treatment and placebo groups took insulin, anti-hypertensive and anti-dyslipidaemic drugs, respectively.
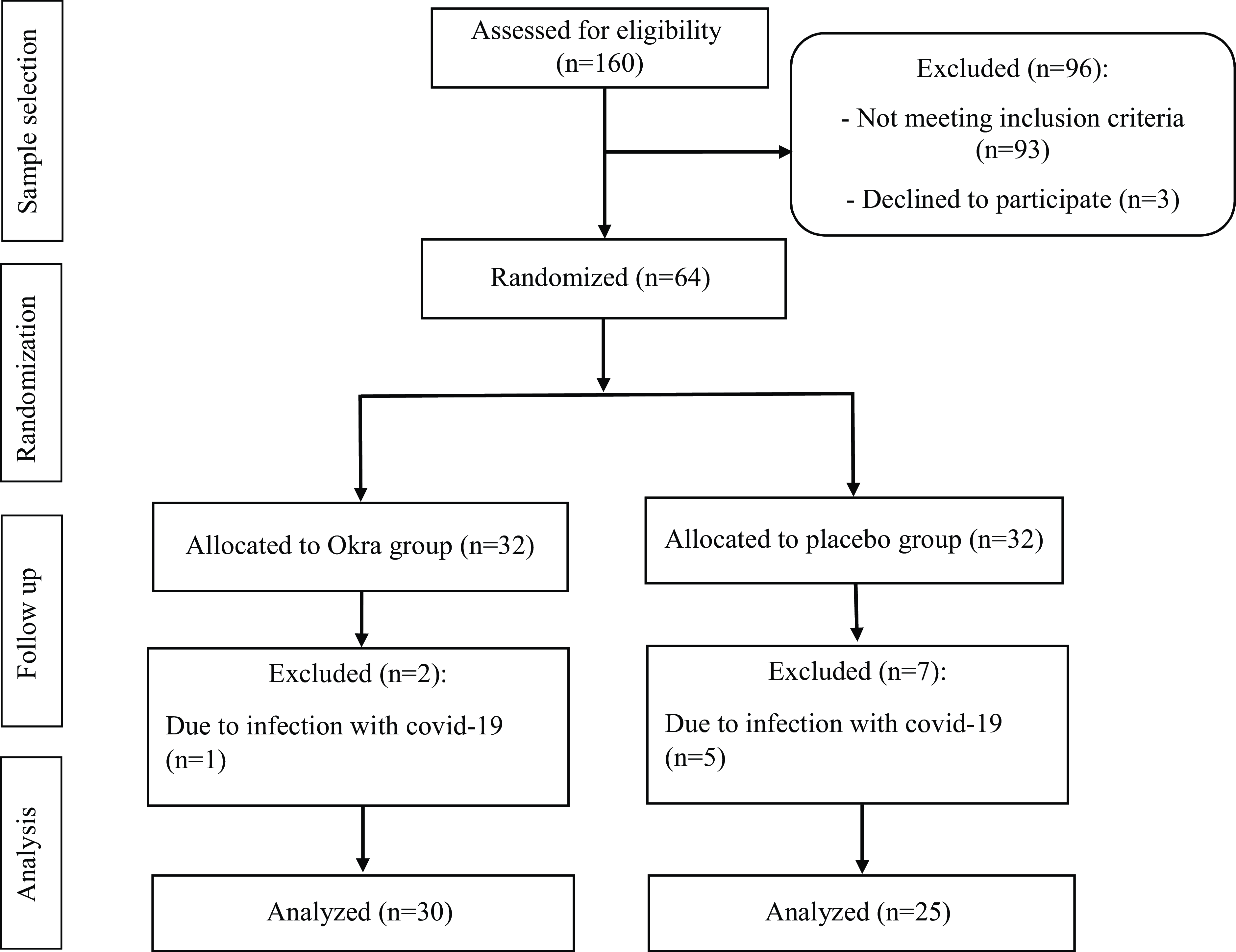
Fig. 1. Flow diagram of the study participants.
Table 2. General characteristics of DN patients in the two study groups (Numbers and percentages; mean values and standard deviations)
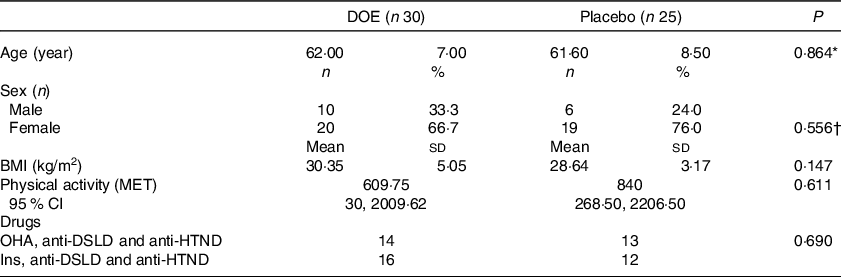
DOE, dried okra extract; DN, diabetic nephropathy; Ins, insulin; OHA, oral hypo-glycaemic agents; anti-HTND, anti-hypertensive drugs; anti-DSLD, anti-dyslipidaemic drugs; MET, metabolic equivalent.
* Obtained from independent samples t test.
† Obtained from Fisher’s exact test.
Table 3. Urine analysis and hs-CRP of DN patients in the two study groups (95 % confidence intervals; mean values and standard deviations)
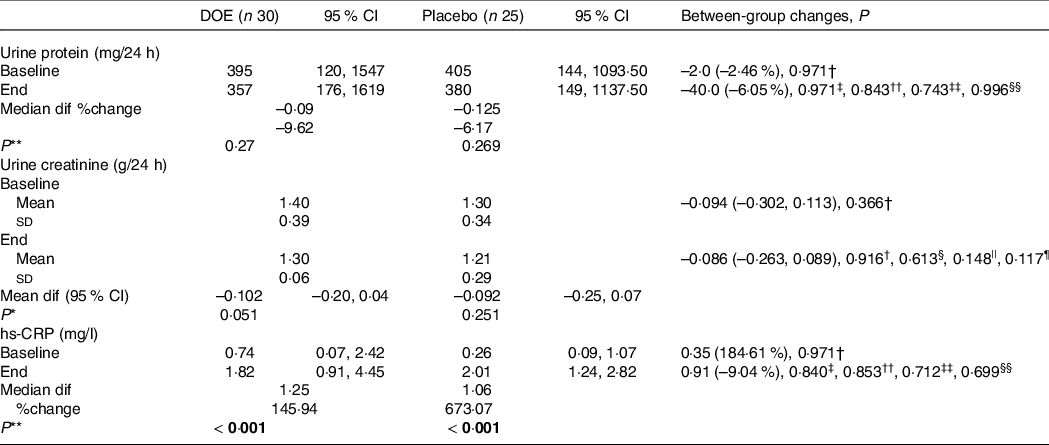
AGE, advanced glycation end products; DN, diabetic nephropathy; DOE, dried okra extract; Dif, difference; hs-CRP, high-sensitivity C-reactive protein.
* Obtained from paired samples t test.
** Obtained from Wilcoxon rank-sum test.
† Obtained from independent samples t test.
‡ Obtained from Mann–Whitney U test.
§ Obtained from ANCOVA, adjusted for baseline values (model 1).
|| Obtained from ANCOVA, adjusted for model 1 plus age, changes in BMI, energy intake and physical activity (model 2).
¶ Obtained from ANCOVA, adjusted for model 2 plus changes in AGE (model 3).
†† Obtained from quantile regression, adjusted for baseline values (model 1).
‡‡ Obtained from quantile regression, adjusted for model 1 plus age, changes in BMI, energy intake and physical activity (model 2).
§§ Obtained from quantile regression, adjusted for model 2 plus changes in AGE (model 3 for urine protein).
|||| Obtained from quantile regression, adjusted for model 2 plus changes in HbA1c (model 3 for hs-CRP).
Table 4. Glycaemic parameters of patients in the two study groups (95 % confidence intervals; mean values and standard deviations)
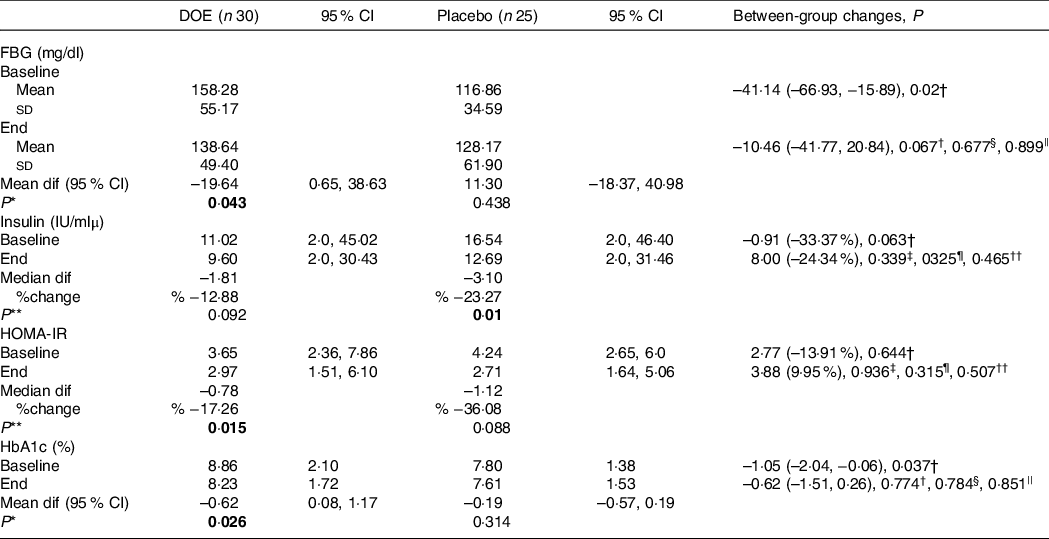
DOE, dried okra extract; Dif, difference; FBG, fasting blood glucose; HOMA-IR, homoeostatic model assessment for insulin resistance.
* Obtained from paired sample t test.
** Obtained from Wilcoxon rank-sum test.
† Obtained from independent samples t test.
‡ Obtained from Mann–Whitney U test.
§ Obtained from ANCOVA adjusted for baseline variable (model 1).
|| Obtained from ANCOVA adjusted for baseline variable, age, change of BMI, change of energy intake and change of physical activity (model 2).
¶ Obtained from quantile regression adjusted for baseline variable (model 1).
†† Obtained from quantile regression adjusted for baseline variable, age, change of BMI, change of energy intake and change of physical activity (model 2).
Comparison of renal function variables and hs-CRP between the two study groups is provided in Table 3. Within-group analysis did not show any significant difference between baseline and endpoint values for urine protein and urine creatinine, although hs-CRP concentration was increased at the end of the trial, compared with baseline values in both groups (P < 0·001). However, between-group analysis did not illustrate any significant difference in the urine protein, urine creatinine and hs-CRP between the two groups, even after adjusting for the effect of confounders.
Glycaemic indices of both groups are presented in Table 4. At the end of the trial, the mean values of FBG, HOMA-IR and HbA1c reduced significantly, compared with baseline levels, in patients who received DOE; based on the intention-to-treat analysis, only the level of HOMA-IR in the placebo group cut down at the end of the trial in comparison with the beginning. However, changes in glycaemic indices were not significantly different between the two groups. The results made no significant changes after controlling the role of the confounders, based on different models. In addition, mRNA expression levels of PPAR-α (P = 0·659), PPAR-γ (P = 0·721), TGF-β (P = 0·357) and Nrf-2 (P = 0·521) did not differ significantly between the DOE and placebo groups, even after adjusting the effects of possible confounders (Fig. 2).
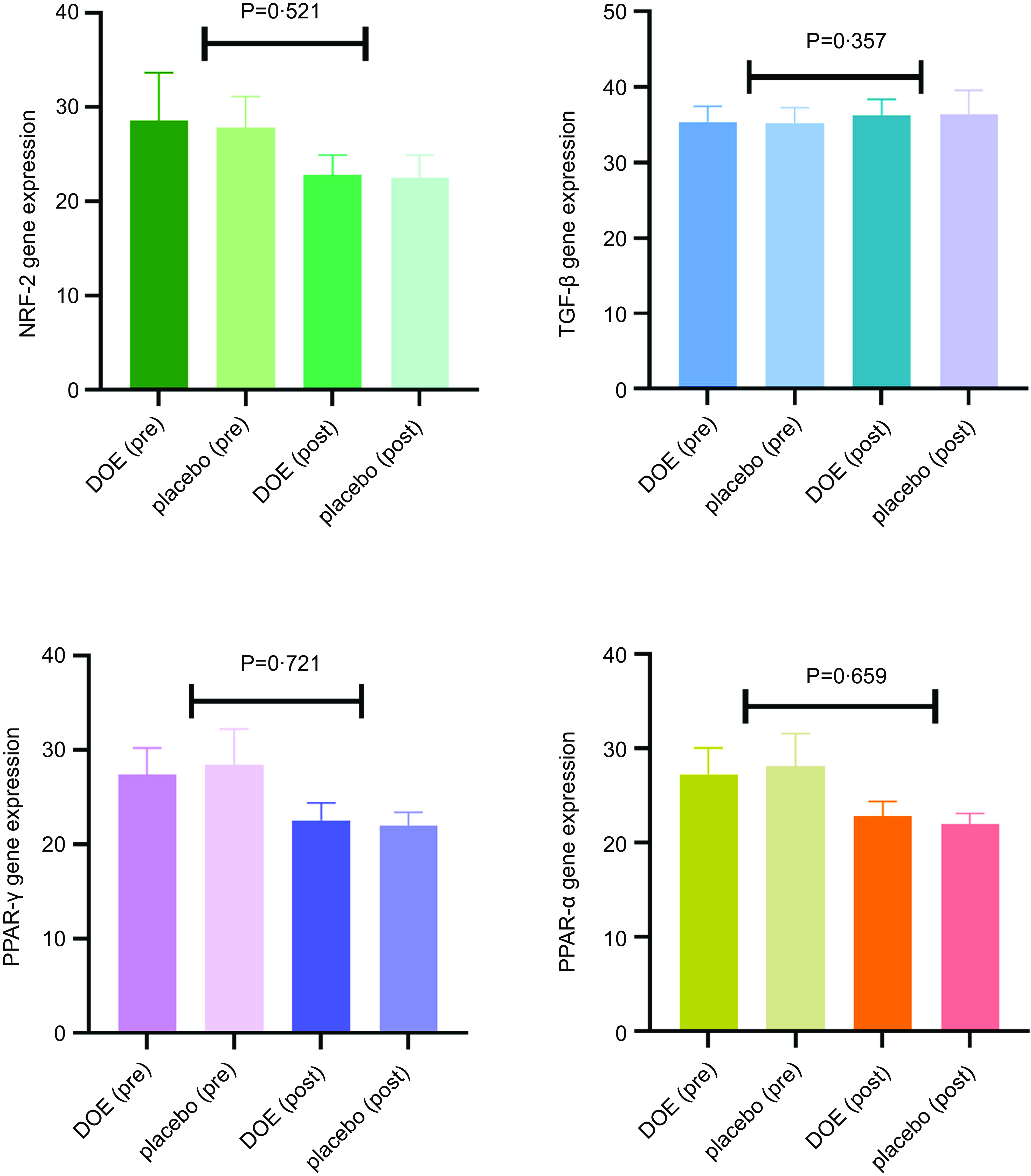
Fig. 2. Effects of DOE on genes expression level of PPAR-α, PPAR-γ, TGF-β and Nrf-2 in DN patients. DOE, dried okra extract; TGF-β, transforming growth factor beta; Nrf-2, nuclear factor-erythroid factor 2-related factor 2; DN, diabetic nephropathy.
Disscusion
Given that DN finally develops into end-stage renal disease, therefore, any effort to retard the progression of the disease will improve the renal function and reduce the costs of the health system. To our knowledge, this is the first clinical trial that investigated the effect of DOE supplementation on renal function, hyperglycaemia, systemic inflammation and expression of PPAR-α, PPAR-γ, TGF-β and Nrf-2 genes in patients with DN. Previous studies reported a direct relationship between hyperglycaemia, inflammation and oxidative stress in DN(Reference Joven and Anderson31–Reference Miranda-Díaz, Pazarín-Villaseñor and Yanowsky-Escatell33). Therefore, any intervention aimed at managing hyperglycaemia in this condition is supposed to affect oxidative stress as well as inflammation. In addition, the limitation of Na and K in these patients because of reduced kidney function is very crucial for the management of the disease(Reference Ikizler, Burrowes and Byham-Gray34). Based on the outcomes of the current study, DOE supplementation could reduce significantly only the level of FBG, HbA1c and HOMA-IR at the end of the trial, compared with baseline values. However, no significant between-group changes were observed in the level of urine creatinine and protein, hs-CRP, FBG, insulin, HbA1c, HOMA-IR, as well as the xpression of PPAR-α, PPAR-γ, TGF-β and Nrf-2 genes in the PBMC of the DN patients.
The effects of okra on renal function, inflammatory and glycaemic indices were previously evaluated in animal and in vitro models. Furthermore, although there are few clinical trials that examined the effect of okra on glycaemic control, no previous clinical trial existed about the effects of okra on inflammatory variables and kidney function in humans. For instance, Laio et al. showed that supplementation with okra powder at 200 and 400 mg/kg in diabetic rats reduced the level of urine creatinine and protein and blood urea nitrogen(Reference Liao, Zhang and Wang17). An in vivo study also indicated that okra polysaccharides could significantly decline blood urea nitrogen and creatinine in diabetic rats(Reference Husen, Wahyuningsih and Ansori35). Another in vivo study illustrated that the administration of okra lectin in a rat model of zymosan-induced temporomandibular joint inflammatory hypernociception reduced the leukocyte influx into the synovial membrane and decreased the levels of TNF-α and IL-1β in the temporomandibular joint tissue(Reference Freitas, do Val and Fernandes19). It seems that the disagreement observed between the results of the current study and those of the previous ones is due to the type of samples (DN patients v. diabetic rats), as well as the type of the supplements (okra extract v. okra ingredients).
The hypoglycaemic effect of okra in animal models has been evaluated in the following studies. For example, Emanuel et al. showed that okra extract supplementation at 100 and 200 mg/kg could reduce FBS in diabetic rats. Another study reported that aqueous extract of okra at 500 mg/kg significantly declined blood and urine glucose, compared with the control group, but did not have any effect on FBS level(Reference Wu, Weng and Zheng36). Further, an in vivo study demonstrated that supplementation with 2·5 %, 5 % and 10 % okra powder could significantly decrease FBS in rats feeding with high-fat diet(Reference Zhang, Zhao and Ren37). Okra is considered a functional food, as it is a source of mucilage, fibres, bioactive polysaccharides and antioxidant compounds(Reference Nikpayam, Safaei and Bahreini38). It seems that the hypoglycaemic effects of this vegetable may be due to the above ingredients. Moreover, phenolic ingredients in okra such as catechins, epigallocatechins, isoquercetin and quercetin-3-o-gentiobioside have inhibitory effects on digestive enzymes involved in the metabolism of carbohydrates and lipids such as α-amylase, α-glucosidase and pancreatic lipase(Reference Shen, Li and Qin39). Further, abscisic acid, a phytohormone in the okra mentioned earlier, has beneficial effects on hyperglycaemia(Reference Daliu, Annunziata and Tenore13). The beneficial effects of okra are also attributed to the antioxidant ingredients such as quercetin glycosides, which suppresses the activation of the Nf-κb and release of the inflammatory cytokines(Reference Peng, Lin and Lin40).
Based on the evidence, there are four studies on human samples that evaluated the effects of okra on hyperglycaemia whose findings are inconsistent. Moradi et al. in a double-blind randomised clinical trial on type 2 diabetes mellitus patients showed that supplementation of 10 gr okra powder for 8 weeks could significantly reduce fasting blood sugar (FBS) and HOMA-IR but had no effect on HbA1c in patients with type 2 diabetes(Reference Moradi, Tarrahi and Ghasempour20). In addition, another quasi-experimental study demonstrated that administration of 250 ml of okra fruit immersion was effective on FBS, blood glucose and HbA1c in type 2 diabetic patients(Reference Haryati23). Furthermore, Saatchi et al. indicated that supplementation with 4 g/d of whole okra fruit powder for 8 weeks had a beneficial effect on FBG, blood glucose and HbA1c in patients with type 2 diabetes(Reference Saatchi, Aghamohammadzadeh and Beheshtirouy22). However, fresh okra consumption did not have any effect on FBG, insulin and HbA1c in overweight subjects with impaired glucose tolerance in a crossover study(Reference Taniguchi-Fukatsu, Yamanaka-Okumura and Naniwa-Kuroki41).
The discrepancy between the results of the present study and animal models has several reasons. Most of the studies, already conducted, are in vitro, which have a different methodology and study design. In such studies, animals were kept in the laboratory where various conditions like dietary intake, sleep, physical activity, environment, temperature, etc. were under the control of the researcher, while the living conditions of the participants especially in the present study are affected by numerous situations, though the possible confounding factors were controlled. Further, it seems that the bioavailability of okra products in human is different from animals, due to intestinal absorption, intestinal microflora metabolism, hepatic and intestinal metabolism, cell uptake, and urinary and biliary excretion that all can affect its efficacy.
The controversy between the results of our work and previous human studies(Reference Moradi, Tarrahi and Ghasempour20,Reference Saatchi, Aghamohammadzadeh and Beheshtirouy22,Reference Haryati23,Reference Taniguchi-Fukatsu, Yamanaka-Okumura and Naniwa-Kuroki41) , regarding the effects of okra on glycaemic indices, is justified as below. First, the type of the supplements differs; in our study, okra extract was used, while in other studies okra was administered as powder or it was submerged in water(Reference Moradi, Tarrahi and Ghasempour20,Reference Saatchi, Aghamohammadzadeh and Beheshtirouy22,Reference Haryati23) . Second, the dosage of the supplement was also different. For example, Moradi et al. administrated 10 g of okra powder(Reference Moradi, Tarrahi and Ghasempour20); however, the dried extract in our capsules was equivalent to 6 g of okra powder. Though our dosage estimation was based on Saatchi et al. study. Third, the type of the disease of the participants differed. The patients in our study were afflicted with DN who had more complicated situations due to the progressive nature of the disease, compared with diabetic patients without nephropathy(Reference Moradi, Tarrahi and Ghasempour20,Reference Saatchi, Aghamohammadzadeh and Beheshtirouy22,Reference Haryati23,Reference Taniguchi-Fukatsu, Yamanaka-Okumura and Naniwa-Kuroki41) . Also, the mean duration of diabetes in the participants of our reaserch was more than 15 years, compared with 5 years in Moradi et al. study(Reference Moradi, Tarrahi and Ghasempour20) Further, the mean age of the participants in our study was older than 60 years, while the patients in other studies aged less(Reference Moradi, Tarrahi and Ghasempour20). Finally, the mean glycaemic indices, including FBS and insulin, were basically much lower in our participants, compared with other studies(Reference Moradi, Tarrahi and Ghasempour20,Reference Haryati23) .
Regarding the effects of okra on PPAR-γ, PPAR-α, TGF-β and Nrf-2 genes level, we could not achieve significant effective findings. It was already demonstrated that the expression of PPAR-γ and PPAR-α genes is altered in diabetes and leads to disturbances in the metabolism of nutrients(Reference Jia, Kim and Jun42). Diabetes and consequent increment of free radicals increase the TGF-β expression and Nrf-2 inactivation(Reference Kasai, Shimizu and Tatara43). Fan et al. reported that the administration of ethanolic extract of okra could significantly reduce the expression of PPAR-γ in rats fed with high-fat diet(Reference Fan, Zhang and Sun44). Another study showed that okra polysaccharides could decline PPAR-γ expression in obese rats(Reference Fan, Guo and Zhang45). Further, an in vitro study indicated that sub-fractions of okra could reduce the elevated expression of TGF-β gene in HK-2 cells, treated with high concentration of glucose(Reference Peng, Chyau and Wang46). Bioactive polysaccharides of okra including rhamnose and galacturonic acid, as well as polyphenol compounds of okra, were shown to have useful effects on glycaemic indices and obesity through the regulation of the expression of PPAR-γ and liver-X-receptors(Reference Shen, Li and Qin39,Reference Durazzo, Lucarini and Novellino47) . It seems that there is a need to perform further research to determine the effects of okra on serum and gene expression of metabolic parameters.
Strengths and limitations of the study
The present clinical trial had several strengths. One of the most important strengths was the preparation of dried okra and supplements by researchers. In addition, all of the participants received a dietary recommendation based on their disease condition. There were some limitations, too. First, the present clinical trial was conducted during the COVID-19 pandemic; therefore, the dropout rate was a bit higher in the placebo group. Though the given power of the study was maintained. Second, the patients who participated in this study were receiving several drugs for the management of their disease, which may affect the net effects of okra, though drug use did not differ between the two groups and we could not prevent the patients from using their drugs ethically. In addition, the participants in the present trial had to receive medications for the management of their disease. However, we could not request them to stop their medication ethically. Therefore, we checked the type of their drugs, commonly administrated, in DN conditions between the two groups. We also excluded those who changed the type and dosage of their drugs during the intervention to minimise their effects as confounders. Third, we could not measure the effective components of DOE in the present trial.
Conclusion
The present randomised, triple-blind, placebo-controlled, clinical trial, for the first time, showed that supplementation of DN patients with 125 mg DOE for 10 weeks did not have any significant effects on renal function, inflammation, glycaemic control and genes expression level. Given that some of the previous studies showed the beneficial effects of okra and its products on metabolic factors in diabetic patients, as well as in animal models, it seems that further clinical trials are needed to determine the effects of different forms of okra on metabolic parameters in DN patients.
Acknowledgements
The authors would like to thank the Vice Chancellorship for Research, Tabriz University of Medical Sciences.
This work was financially supported by the ‘Research Vice Chancellor’ of Tabriz University of Medical Sciences (TBZMED), Tabriz, Iran, Grant agreement no: 64859.
O.N. and M.S.A. designated the study. O.N., E.S., N.B. and V.S. were involved in sampling and data collection. P.A. involved in supplement preparation. O.N. and M.S.A. involved in statistical analysis and drafted the manuscript. O.N. revised the manuscript. All authors read and approved the final manuscript.
All authors declare that there is no conflict of interest.
All analyses conducted based on previous published studies; therefore, no ethical approval and patient consent are required. All previous published studies were approved by ethics committee, respectively.
The protocol of the present study was approved by the Ethics Committee of Tabriz University of Medical Sciences (IR.TBZMED.REC.1399.466) and registered at the Iranian registry of clinical trials (IRCT20110530006652N3).
Supplementary material
For supplementary material/s referred to in this article, please visit https://doi.org/10.1017/S0007114523002180