1. Introduction
Scarce Triassic outcrops in the circum-Black Sea region outline three facies belts (Fig. 1). In the west in the Balkans the Triassic sediments were deposited on a continental to shallow marine platform passing to a deep shelf or platform margin at Dobrugea and Istanbul (Gedik, Reference Gedık1975; Muttoni et al. Reference Muttoni, Gaetani, Budurov, Zagorchev, Trifonova, Ivanova, Petrounova and Lowrie2000; Seghedi, Reference Seghedı, Ziegler, Cavazza, Robertson and Crasquin-Soleau2001; Derman, Reference Derman2002; Bedi et al. Reference Bedı, Vasılev, Dabovskı, Ergen, Okuyucu, Doğan, Tekın, Ivanova, Boncheva, Lakova, Sachanskı, Kuşçu, Tuncay, Demıray, Soycan and Göncüoğlu2013). They rest unconformably on the eroded remnants of the Variscan orogen, which include deformed Carboniferous sedimentary rocks and Permo-Carboniferous granitoids. In the north between the Caspian Sea and the Crimea is a Triassic magmatic belt (Nikishin et al. Reference Nikishin, Ziegler, Panov, Nazarevich, Brunet, Stephenson, Bolotov, Korotaev, Tikhomirov, Ziegler, Cavazza, Robertson and Crasquin-Soleau2001, Reference Nıkıshın, Zıegler, Bolotov and Fokın2012; Alexandre et al. Reference Alexandre, Chalot-Prat, Saintot, Wijbrans, Stephenson, Wilson, Kitchka and Stovba2004; Natal’in & Şengör, Reference Natal’in and Şengör2005). This is known almost solely from subsurface data (Tikhomirov, Chalot-Prat & Nazarevich, Reference Tıkhomırov, Chalot-Prat and Nazarevıch2004) but is also inferred from abundant Triassic clastic zircons in the Mesozoic sediments in the Pontides (Karslıoğlu et al. Reference Karslıoğlu, Ustaömer, Robertson and Peytcheva2012; Okay et al. Reference Okay, Sunal, Sherlock, Altıner, Tüysüz, Kylander-Clark and Aygül2013; Ustaömer et al. Reference Ustaömer, Ustaömer, Gerdes, Robertson and Zulauf2014) and in Crimea (A. Nikishin, pers. comm.). South of the magmatic belt and extending westward to the Aegean Sea are strongly deformed, thick turbidite sequences, which are associated in the Pontides with Upper Triassic eclogites and blueschists (Fig. 1; Okay, Reference Okay, Bozkurt, Winchester and Piper2000; Nikishin et al. Reference Nikishin, Ziegler, Panov, Nazarevich, Brunet, Stephenson, Bolotov, Korotaev, Tikhomirov, Ziegler, Cavazza, Robertson and Crasquin-Soleau2001, Reference Nıkıshın, Zıegler, Bolotov and Fokın2012; Okay & Göncüoğlu, Reference Okay and Göncüoğlu2004). These include the Karakaya Complex in western Turkey (Okay & Göncüoğlu, Reference Okay and Göncüoğlu2004), the Küre Complex in the Central Pontides (Ustaömer & Robertson, Reference Ustaömer and Robertson1994), the Tauric series in Crimea and the Dizi series in the Caucasus (Adamia et al. Reference Adamıa, Zakarıadze, Chkhotua, Sadradze, Tseretelı, Chabukıanı and Gventsadze2011; Fig. 1). The significance and origin of this deformation and metamorphism, known as the Cimmeride orogeny, is poorly understood; it is either related to the collision of a ‘Cimmerian continent’ with the Laurasian margin (Şengör, Reference Şengör1984) or to accretional processes along the active Laurasian margin (Okay, Reference Okay, Bozkurt, Winchester and Piper2000).

Figure 1. Tectonic map of the circum-Black Sea region showing the outcrops of Triassic strata north of the İzmir–Ankara suture (modified from Okay & Tüysüz, Reference Okay, Tüysüz, Durand, Jolivet, Horváth and Séranne1999). Abbreviations: CPS – Central Pontide Supercomplex; Permo-Carboniferous granitoids: G – Gebze; Gm – Gümüşhane; I – Istanbul; K – Kürek; S – Söğüt.
Stratigraphic and geochronological data are critical in constraining the origin and timing of the Cimmeride orogeny in the circum-Black Sea region. Here we present new palaeontological and isotopic data on the Triassic series and its basement in the Central Pontides. The data include characterization of a late Variscan metamorphic–plutonic basement, a Middle to Upper Triassic pelagic limestone sequence and pre-Middle Jurassic serpentinites, which occur as tectonic slices in the Upper Triassic turbidites. The Cimmeride deformation is envisaged as an accretionary rather than collisional orogeny involving latest Triassic attempted subduction and accretion of oceanic edifices.
2. Geological setting
The Central Pontides include two Pontic terranes: the Istanbul Zone in the west and the Sakarya Zone in the east (Fig. 1). They share a common Upper Jurassic shallow marine limestone cover in the Central Pontides but show different pre-Jurassic development (Fig. 2). The Istanbul Zone is characterized by a well-developed Palaeozoic (Ordovician to Carboniferous) sedimentary sequence, including Carboniferous coal measures (Görür et al. Reference Görür, Monod, Okay, Şengör, Tüysüz, Yığıtbaş, Sakınç and Akkök1997; Dean et al. Reference Dean, Monod, Rıckards, Demır and Bultynck2000), which rests on a late Neoproterozoic granitic basement (Chen et al. Reference Chen, Sıebel, Satır, Terzıoğlu and Sak2002; Ustaömer, Mundil & Renne, Reference Ustaömer, Mundıl and Renne2005). The Palaeozoic sequence is intruded by Permian granitoids (Şahin et al. Reference Şahin, Güngör, Aysal and Öngen2009; Okay et al. Reference Okay, Sunal, Sherlock, Altıner, Tüysüz, Kylander-Clark and Aygül2013) and is unconformably overlain by Permo-Triassic red beds and by the Middle Jurassic lacustrine limestone and shale (Fig. 2).

Figure 2. Stratigraphic section of the Istanbul and Sakarya zones in the Central Pontides (modified from Okay et al. Reference Okay, Sunal, Tüysüz, Sherlock, Keskin and Kylander-Clark2014). The geological time scale is after Cohen et al. (Reference Cohen, Finney, Gibbard and Fan2013).
The crystalline basement of the Sakarya Zone in the Central Pontides is represented by poorly exposed Permo-Carboniferous granitoids and metamorphic rocks close to the Black Sea margin (Fig. 1; Nzegge et al. Reference Nzegge, Satır, Sıebel and Taubald2006; O. M. Nzegge, unpub. Ph.D. thesis, Univ. Tübingen, 2008). The large area of metamorphic rocks in the southern part of the Central Pontides, the Central Pontide Supercomplex (Figs 1, 3), which is shown as Triassic and older basement on the geological maps and in publications (e.g. Yılmaz & Şengör, Reference Yılmaz and Şengör1985; Tüysüz, Reference Tüysüz1990; Ustaömer & Robertson, Reference Ustaömer and Robertson1994, Reference Ustaömer and Robertson1999; Yılmaz et al. Reference Yılmaz, Tüysüz, Yiğitbaş, Can Genç, Şengör and Robinson1997; Yiğitbaş, Elmas & Yılmaz, Reference Yığıtbaş, Elmas and Yılmaz1999; Uğuz, Sevın & Duru, Reference Uğuz, Sevın and Duru2002), has recently been shown to be of Jurassic and Cretaceous age (Okay et al. Reference Okay, Tüysüz, Satır, Özkan-Altıner, Altıner, Sherlock and Eren2006, Reference Okay, Sunal, Sherlock, Altıner, Tüysüz, Kylander-Clark and Aygül2013).
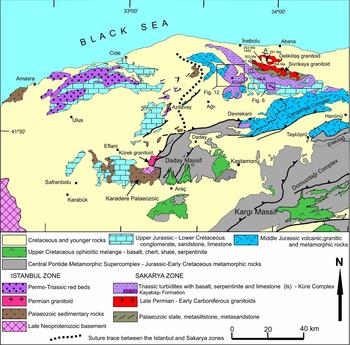
Figure 3. Geological map of the Central Pontides with outcrops of pre-Cretaceous units and Central Pontide Metamorphic Supercomplex (based on Aydın et al. Reference Aydın, Demir, Özçelık, Terzioğlu, Satır, Erler, Ercan, Bingöl and Örçen1995; Aksay et al. Reference Aksay, Pehlıvan, Gedik, Bilginer, Duru, Akbaş and Altun2002; Uğuz, Sevın & Duru, Reference Uğuz, Sevın and Duru2002; Okay et al. Reference Okay, Sunal, Sherlock, Altıner, Tüysüz, Kylander-Clark and Aygül2013).
The Permo-Carboniferous granitoids in the Central Pontides are overlain in the south by the Upper Triassic turbidites, which constitute part of an orogenic Triassic volcano-sedimentary unit called the Küre Complex (Ustaömer & Robertson, Reference Ustaömer and Robertson1994). The Upper Triassic turbidites also crop out in the southern Crimea as the Tauric series (Zonenshain, Kuzmin & Natapov, Reference Zonenshain, Kuzmin and Natapov1990). The Küre Complex and the Tauric series are intruded by Middle Jurassic shallow level intrusions and are unconformably overlain by Upper Jurassic continental clastic rocks and limestones (Fig. 2). For this study we worked in detail in the Central Pontides and made a geological field trip to Crimea.
3. The late Variscan basement
Palaeozoic low-grade metasedimentary rocks intruded by Permian and Carboniferous granitoids constitute the basement of the Central Pontides. The basement crops out poorly in the densely vegetated coastal region south of İnebolu and Abana (Fig. 3). The metasedimentary rocks consist of black to brown slates to phyllites interbedded with metasiltstone and fine-grained metasandstone (Boztuğ & Yılmaz, Reference Boztuğ and Yılmaz1983). A serpentinite lens, c. 100 m thick occurs within the slates close to the Late Carboniferous granitoid (Fig. 4, UTM coordinates 36T 0573450–4633030) and has undergone contact metamorphism, which indicates a pre-Permian age for the serpentinite. Boztuğ & Yılmaz (Reference Boztuğ and Yılmaz1983) also described from further east a serpentinite lens in the contact metamorphic aureole of the Late Carboniferous granitoid. The metaclastic rocks are lithologically similar to the Upper Triassic turbidites, and have previously been mapped as such (Boztuğ & Yılmaz, Reference Boztuğ and Yılmaz1983); however, they have undergone a low-greenschist-facies regional metamorphism, and a penetrative cleavage is well developed even in sandstones. The region is later deformed by thrusting and folding and is cut by normal faulting, all probably of Eocene and younger age (Fig. 4).

Figure 4. Geological cross-section from the northern part of the Central Pontides showing the relationship between the Variscan basement and the overlying units. For location of the section see Figure 3.
The metasedimentary rocks are intruded by granitoids leading to the generation of hornfels in the contact aureole. Previously, these granitoids were considered Middle Jurassic in age (Boztuğ & Yılmaz, Reference Boztuğ and Yılmaz1983; Boztuğ et al. Reference Boztuğ, Debon, Le Fort and Yılmaz1984, Reference Boztuğ, Debon, Le Fort and Yılmaz1995). However, isotopic dating by Nzegge et al. (Reference Nzegge, Satır, Sıebel and Taubald2006) produced latest Carboniferous and Early Permian ages (Table 1). The Variscan granitoids comprise the Deliklitaş granitoid in the north and the Sivrikaya Granitoid in the south; they may be connected under the Cretaceous cover (Figs 3, 4). Close to the northern margin of the Sivrikaya Granitoid, there are numerous of 1–10 m thick enclaves of gneissic micaschists with the mineral assemblage of quartz + K-feldspar + muscovite + biotite + sillimanite.
Table 1. Selected isotopic ages from the Central Pontides

a – Nzegge et al. Reference Nzegge, Satır, Sıebel and Taubald2006; O. M. Nzegge, unpub. Ph.D. thesis, Univ. Tübingen, 2008; b – this study; c – Okay et al. Reference Okay, Sunal, Tüysüz, Sherlock, Keskin and Kylander-Clark2014; d – Okay et al. Reference Okay, Sunal, Sherlock, Altıner, Tüysüz, Kylander-Clark and Aygül2013.
The UTM coordinates are from the European 1979 grid, which is closely compatible with the 1: 25 000 scale topographic maps of Turkey.
In terms of mineral assemblage, the Deliklitaş and Sivrikaya intrusions range from hornblende-biotite granodiorite to two-mica granite (Boztuğ & Yılmaz, Reference Boztuğ and Yılmaz1983; Nzegge et al. Reference Nzegge, Satır, Sıebel and Taubald2006). They are peraluminous, calc-alkaline and high-K in composition (Nzegge et al. Reference Nzegge, Satır, Sıebel and Taubald2006). Geochemical features of the granitoids, including their εNd(t), δ18O values and Sr(i) ratios, suggest derivation by dehydration melting of metapelitic and mafic crust (Nzegge et al. Reference Nzegge, Satır, Sıebel and Taubald2006). This is also supported by the presence of primary muscovite and enclaves of high-temperature metamorphic rocks in the Deliklitaş granitoid. On the tectonic discrimination diagrams most samples plot in the field of volcanic arc granitoids (Nzegge et al. Reference Nzegge, Satır, Sıebel and Taubald2006). Their peraluminous nature, high K and Sr contents, high Rb/Sr values and initial Sr ratios point to crustal melting and suggest an episode of crustal thickening.
U–Pb magmatic zircon ages from two samples of the Sivrikaya Granitoid are 303 ± 2 Ma and 301 ± 2 Ma (latest Carboniferous) (Nzegge et al. Reference Nzegge, Satır, Sıebel and Taubald2006; O. M. Nzegge, unpub. Ph.D. thesis, Univ. Tübingen, 2008; Table 1). During this study, coarse muscovites from a sample of the Sivrikaya Granitoid were dated using the Ar–Ar laser probe at the Open University in the UK. The location of sample 1444 is shown in Figure 3. The mineral separation and analytical methods are explained in the online Supplementary Material available at http://journals.cambridge.org/geo; the analytical data are given in Table S1 in the online Supplementary Material available at http://journals.cambridge.org/geo. Twelve grains of muscovite produced a mean age of 298 ± 2 Ma (Fig. 5; Table 1), which is similar to the zircon U–Pb ages. The muscovites in the sample are 4–10 mm long, and the blocking temperature for such coarse-grained muscovites may reach 600–650 °C (Cliff, Reference Cliff1985). To constrain the cooling history of the Sivrikaya Granitoid, we used the Ar–Ar method to date small muscovites (c. 0.4 mm long) from a micaschist enclave (sample 1442 in Fig. 3; Table 2). The micaschist consists of quartz + muscovite + biotite + sillimanite. Eleven muscovite grains gave ages ranging from 279 Ma to 262 Ma with an average of 267 ± 6 Ma (Fig. 5; Table 1; Table S1 in the online Supplementary Material available at http://journals.cambridge.org/geo), suggesting a long period of cooling. O. M. Nzegge (unpub. Ph.D. thesis, Univ. Tübingen, 2008) also obtained a biotite whole-rock Rb–Sr age of 275 ± 10 Ma from the Sivrikaya Granitoid. The zircon U–Pb, muscovite Ar–Ar and biotite Rb–Sr ages indicate that the Sivrikaya Granitoid crystallized in latest Carboniferous time (c. 302 Ma) and underwent a slow cooling during Permian time.
Table 2. Ar–Ar data from the Middle Jurassic porphyry intruding into serpentinite


Figure 5. Isotopic age data from the Permo-Carboniferous granitoids in the Pontides (Yılmaz, Reference Yılmaz1975; Nzegge et al. Reference Nzegge, Satır, Sıebel and Taubald2006; O. M. Nzegge, unpub. Ph.D. thesis, Univ. Tübingen, 2008; Okay et al. Reference Okay, Satır, Tüysüz, Akyüz and Chen2001, Reference Okay, Sunal, Sherlock, Altıner, Tüysüz, Kylander-Clark and Aygül2013; Şahin et al. Reference Şahin, Güngör, Aysal and Öngen2009; Sunal et al. Reference Sunal, Natal’ın, Satır and Toraman2006; Topuz et al. Reference Topuz, Altherr, Siebel, Schwartz, Zack, Hasözbek, Barth, Satır and Şen2010; Ustaömer, Ustaömer & Robertson, Reference Ustaömer, Ustaömer and Robertson2012; this study).
The Deliklitaş Granitoid is slightly younger than the Sivrikaya Granitoid; two samples from the granitoid produced Early Permian zircon U–Pb ages of 294 ± 1 Ma and 291 ± 5 Ma (Nzegge et al. Reference Nzegge, Satır, Sıebel and Taubald2006; O. M. Nzegge, unpub. Ph.D. thesis, Univ. Tübingen, 2008; Table 1). The Sivrikaya and Deliklitaş granitoids crystallized at around the Permian–Carboniferous boundary, and this was followed by a prolonged cooling. The magmatism appears to have changed from slightly metaluminous in latest Carboniferous time to peraluminous S-type in Early Permian time (Nzegge et al. Reference Nzegge, Satır, Sıebel and Taubald2006). Granitoids of Early Carboniferous to latest Permian age occur over a wide area in the Pontides (Figs. 1, 5; Table 1) and are also described from the core of the Greater Caucasus (Hanel, Gurbanov, & Lippolt, Reference Hanel, Gurbanov and Lippolt1992; Somin, Reference Somin2011). They constitute a link between the Variscan belt of central Europe and the Urals.
The Palaeozoic metasedimentary rocks are overlain in the south by the Upper Triassic turbidites (Figs. 3, 4). No clear contact can be observed in the field; however, the presence of debris flows with metamorphic rock clasts in the Upper Triassic turbidites just above the contact suggests the presence of an unconformity (Fig. 4).
4. The Küre Complex
The Küre Complex consists of a thick siliciclastic Upper Triassic turbidite sequence, called the Akgöl Formation, associated with basalt, gabbro and serpentinite (Ustaömer & Robertson, Reference Ustaömer and Robertson1994). Economic massive sulphide deposits occur along the shale–basalt contacts. In the flysch there is also a large outcrop of Triassic limestone, which was studied in detail.
4.a. Middle–Upper Triassic limestone
In the Central Pontides the Permo-Carboniferous granitoids are unconformably overlain by the Upper Triassic turbidites indicating a period of uplift and erosion before Late Triassic time. The earlier Triassic sequences are found as sedimentary blocks in the Upper Triassic flysch. The largest block, known since Blumenthal documented it in Reference Blumenthal1948, crops out northeast of Devrekani (Fig. 3). Önder (Reference Önder1988) named the limestones the Kayabaşı Formation, and ascribed a ‘topmost Middle Triassic – Late Triassic’ age based on conodonts. The Kayabaşı Formation is of palaeogeographic and stratigraphic importance as it constitutes the only coherent marine limestone Triassic section in the Pontides with the exception of the Triassic in the western part of the Istanbul Zone. The Kayabaşı Formation was mapped in detail, several stratigraphic sections were measured and over 80 samples were collected for foraminifera and conodonts (Fig. 6).

Figure 6. Geological map and cross-section of the Triassic limestones northeast of Devrekani. For location see Figure 3.
The Kayabaşı Formation forms a c. 550 m thick and 4 km long block in the turbidites of the Akgöl Formation, cropping out along a NW–SE-trending ridge (Fig. 7a). The bedding in the limestone is difficult to recognize; when it is recognized it dips steeply (60–70°) south to southwest forming an inverted sequence (Fig. 6). The limestone is bounded in the northeast by a normal fault, and is stratigraphically overlain in the south by the turbidites of the Akgöl Formation. The Akgöl Formation above the Kayabaşı Formation includes small blocks of Triassic limestone (Fig. 7b).

Figure 7. Field photographs from the Triassic limestone in the Central Pontides. (a) The Triassic limestone ridge looking towards the west. The low-lying ground on the right (north) consists of Upper Triassic turbidites. (b) Triassic limestone clast in the Upper Triassic sandstone (locality 1877). (c) Limestone breccia – micritic limestone clasts in a red sandy-carbonate matrix (locality 1870). (d) Limestone breccia. The limestone beds are boudinaged but the continuity of the bedding suggests in situ brecciation with minimum transport (locality 1872). For locations see Figure 6.
The Kayabaşı Formation is subdivided into three members (Fig. 8):

Figure 8. Composite stratigraphic section of the Kayabaşı Formation and the overlying turbidites of the Akgöl Formation. Approximate positions of some important biostratigraphic samples are indicated.
(a) The limestone breccia member (Anisian). It constitutes the basal 200 m of the Kayabaşı Formation and is best observed on the ridge east of Çal village (Fig. 6). It consists of limestone breccia, with 1 to 10 cm sized light grey carbonate clasts set in a red micritic or sandy matrix (Fig. 7c). The limestone breccias are locally separated by thinly bedded micritic limestone beds. Textures showing a transition from bedded limestone to breccia, and the preservation of ghost bedding in some of the breccias (Fig. 7d) suggest that brecciation occurred largely in situ without significant transport.
A sample from the thinly bedded micritic limestones from the lower part of the succession (sample 1874) contains foraminifera characteristic of the Anisian: Meandrospira pusilla, Meandrospira dinarica, Endoteba controversa, Nodasoria elabugae and Trochammina sp. (Fig. 9). Samples from further up in the sequence contain Anisian conodonts: Neogondolella regalis (sample 1866), Gladigondolella timorensis budurovi (juvenile) and Neogondolella regalis (sample 1868) (Fig. 10). These beds also contain Anisian foraminifera: Arenovidalina amylovoluta, Arenovidalina chialingchiangensis, Opthalmidium? ubeyliense (sample 1867) and Arenovidalina amylovoluta (sample 1868; Fig. 9). The palaeontological data indicate an Anisian age for the limestone breccia member.

Figure 9. Photomicrographs of foraminifera from the Kayabaşı Formation. For location of the specimens see Figure 6. (a) Meandrospira pusilla (Ho), sample 3115E. (b, c) Meandrospira dinarica Kochansky-Devidé & Pantic: (b) sample 1874, (c) sample 3115E. (d, e) Planiinvoluta? mesotriasica Baud, Zaninetti & Bronnimann, sample 3115E. (f–i) Arenovidalina chialingchiangensis Ho: (f, g, i) sample 1886, (h) sample 3011A. (j, k) Arenovidalina chialingchiangensis rhombea Ho, sample 1886. (l, m) Arenovidalina amylovoluta Ho, sample 1867; (n–p) Arenovidalina spp.: (n, o) sample 1886, (p) sample 3011A. (q) Arenovidalina sp. or Eoophthalmidium sp., sample 1886. (r) Ophthalmidium? ubeyliense Dağer, sample 3011A. (s, t) Eoophthalmidium tricki Langer: (s) sample 3011A, (t) sample 1886. (u) Decapoalina schaeferae (Zaninetti, Altıner, Dağer & Ducret), sample 3113-ST-2. (v) Ophthalmidium sp., sample 1886. (w) Hoyenella sp., sample 1886. (x) Turriglomina mesotriasica (Koehn-Zaninetti), sample 1890. (y) Turriglomina mesotriasica (Koehn-Zaninetti) form B?, sample 1889. (z) Turriglomina scandonei Zaninetti, Ciarapica, Martini, Salvini-Bonnard & Rettori, sample 1889. (aa, bb) Trochammina almtalensis Koehn-Zaninetti: (aa) sample 1869, (bb) sample 3115E. (cc) Gsolbergella spiriloculiformis (Oraveczne Scheffer), sample 1890. (dd) Cucurbita sp., sample 1890. (ee) Endoteba controversa Vachard & Razgallah, sample 3115E. (ff, gg) Endoteba kuepperi (Oberhauser), sample 1890. (hh) ‘Nodosaria’ elabugae Cherdyntsev, sample 1874. (ii) ‘Nodosaria’ skyphica Efimova, sample 1886. (jj) Tubiphytes obscurus Maslov, sample 1890. (kk) Baccanella floriformis Pantic, sample 1890. (ll) Auloconus? sp., sample 1829B. (mm) Aulotortus communis (Kristan), sample 1829B. (nn) Planiinvoluta carinata Leischner, sample 1829A. (oo) Semiinvoluta sp., sample 1829B. Scale bars equal 100 μm.

Figure 10. Electron microscope images of conodonts from the Kayabaşı Formation. For location of the specimens see Figure 6. (a, b) Neogondolella constricta, Mosher & Clark; middle–upper Illyrian – lower Fassanian (upper Anisian – lower Ladinian): (a) upper view; adult specimen shows constriction near posterior end and probable slight bifid basal cavity, sample 1897; (b) lower view; younger specimen, small loop-like pit, sample 1897. (c) Gladigondolella malayensis, Nogami; Julian (Carnian); sample 1895. (d) Metapolygnathus tadpole (Hayashi); Cordevolian – lower Tuvalian (Carnian); angular view, sample 1895. (e) Neogondolella regalis (Mosher); middle Aegean – Bithynian (Anisian); sample 1868. (f) Metapolygnathus inclinatus (Kovarcs); Julian (Carnian); sample 1895. (g–i) Metapolygnathus cf. fuelopi; Ladinian; sample 1889. (j) M. Gr. inclinatus; Julian (Carnian); sample 1894. (k, l) Metapolygnathus Gr. excelsus-inclinatus; Late Ladinian – Early Carnian; sample 1889. (m–u) Gladigondolella tethydis; Middle Triassic: (m–s) P element, (t) S1, (u) M element; (m, n) sample 1892, (o–u) sample 1889. Scale bar equals 500 μm
(b) The Hallstatt-type micritic limestone member (Anisian to Carnian). This includes pinkish, ammonoid-bearing pelagic limestones, which form the middle part of the Kayabaşı Formation and is c. 300 m thick. It is best observed on the Kayabaşı ridge east of Mermerli Stream (Fig. 6). The basal part of the Hallstatt member consists of dark grey micritic limestones. These pass upwards into Hallstatt-type pinkish, slightly nodular, micritic limestones locally with intercalations of grey medium-bedded calc-arenite. Ammonites of the Arcestidae group, characteristic of the Middle–Upper Triassic, occur in the pink limestones.
A 220 m thick section was measured in the Hallstatt member. Anisian conodonts (Paragondolella bulgarica and Neogondolella regalis, samples 1883 and 1884), the Anisian–Ladinian conodont Neogondolella constricta and Anisian–Ladinian foraminifera Arenovidalina chialingchiangensis, Arenovidalina chialingchiangensis rhombea, Arenovidalina amylovoluta, Arenovidalina spp., Hoyanella sp., Eoophthalmidium tricki, Ophthalmidium sp. and ‘Nodasaria’ skyphica (sample 1886) occur in the basal massive grey micrites. A sample taken 20 m above sample 1886 contains Ladinian conodonts: Gladigondolella tethydis and Metapolygnathus excelsa (sample 1888). Ladinian to Carnian conodonts (Gladigondolella tethydis, Metapolygnathus Gr. excelsus-inclinatus, M. inclinatus, M. cf. fueloepi and M. carpathicus, sample 1889) and foraminifera (Turriglomina mesotriasica and Turriglomina scandonei, sample 1889) and Carnian foraminifera (Gsolbergella spiriloculiformis, Ophthalmidium sp., Cucurbita sp., Endoteba kuepperi, Endoteba obturata, Endotebanella sp. (sample 1890) associated with Tubiphytes obscurus, and Baccanella floriformis) were determined in the succeeding samples. A sample taken from the top part of the Hallstatt limestone member comprises Carnian conodonts: Gladigondolella tethydis and Metapolygnathus polygnathiformis (sample 1892), and Gladigondolella malayensis and M. tadpole (sample 1895). The palaeontological data indicate an Anisian to Carnian age for the Hallstatt member.
(c) The black limestone–shale member. Black limestone and interbedded shale occur in the northernmost part of the limestone ridge and constitute stratigraphically the upper member of the Kayabaşı Formation. Its contact with the Hallstatt member is not exposed in the field. The member consists of medium-bedded black limestone with abundant thin-shelled bivalve fragments intercalated with thin black shale beds. Limestone samples from this member contain Lagenid-type foraminifera and thin-shelled bivalves but no age diagnostic fossils.
The palaeontological data indicate an Anisian to Carnian age for the Kayabaşı Formation. In terms of facies and age, it can be compared with the Triassic sequence in Dobrugea (Seghedi, Reference Seghedı, Ziegler, Cavazza, Robertson and Crasquin-Soleau2001) and to a lesser degree with the Triassic in the western part of the Istanbul Zone (Yurttaş-Özdemir, Reference Yurttaş-Özdemir1971; Assereto, Reference Assereto1972); however, the conodont fauna in the Istanbul–Gebze region is quite different (Gedik, Reference Gedık1975; A. M. Kılıç, unpub. Ph.D. thesis, Cumhuriyet Univ., 2004).
Sandstone beds overlying the Kayabaşı Formation contain debris flow horizons with clasts of Triassic limestone (Fig. 7b). The following lower–middle Anisian foraminifera were determined in one of the limestone clasts: Meandrospira dinarica, Meandrospira pusilla, Pilammina sp., Endoteba controversa, Planiinvoluta? mesotriasica and Trochammina almtalensis (sample 3115E in Fig. 6). Similar Anisian limestone clasts are described by Kozur et al. (Reference Kozur, Aydın, Demir, Yakar, Göncüoğlu and Kuru2000) from a debris flow in the Akgöl Formation between İnebolu and Küre.
The large limestone outcrop of the Kayabaşı Formation is surrounded by the sandstones and shales of the Akgöl Formation. This observation, the irregular contacts between these two units (Fig. 6) and the presence of Triassic limestone clasts in the surrounding sandstones (Fig. 7b) indicate that the Triassic limestone forms a large slide block in the turbidites. The in situ brecciation observed in the basal parts of the Kayabaşı Formation (Fig. 7d) most likely occurred during sliding of the block into the basin.
A different type of limestone block occurs in the Upper Triassic turbidites a few hundred metres north of the Kayabaşı Formation close to the village of Softa (Fig. 6; Yılmaz & Boztuğ, Reference Yılmaz and Boztuğ1987). It consists of c. 20 m thick, thickly bedded to massive, bluish grey, bioclastic limestone with abundant brachiopods, lamellibranchs, coral, algae, sponge spicules, bryozoa and echinoid spine fragments, deposited probably in a fore-reef environment. The foraminiferal fauna include Decapoalina schaeferae, Lenticulina sp. and Opthalmidium sp. (Fig. 9, sample 3113-ST-2) and indicates a Norian–Rhaetian age, although some of the agglutinating forms recognized in the upper part of the block are suggestive of the lowermost Jurassic. A 20 cm large reddish limestone clast east of Çal village also contains Norian–Rhaetian foraminifera of Aulotortus communis, Aulotortus sp., Auloconus? sp., Semiinvoluta sp., Planiinvoluta carinata, Caronipora sp. and Lenticulina sp. (Fig. 9, sample 1829). These blocks can be compared with the uppermost Triassic limestone block described from the Karakaya Complex in NW Turkey (Okay & Altıner, Reference Okay and Altıner2004).
4.b. Upper Triassic – ?Lower Jurassic turbidites: the Akgöl Formation
The Akgöl Formation, which includes the Middle–Upper Triassic limestone blocks, consists of black shale intercalated with thin beds of dark siltstone and sandstone (Fig. 11a); the average shale to siltstone and sandstone ratio is 65:35, although their distribution is highly uneven with black shale making up over 90 % in some outcrops. The sandstones are generally thinly to medium-bedded, generally fine grained and of greywacke type. Graded bedding is observed in some outcrops; sole marks are rare. The sandstones are poorly sorted, and consist mainly of angular quartz, feldspar and rock clasts; the latter are dominated by andesitic and more acidic volcanic rocks. The sedimentological features indicate a distal turbidite fan. The geochemistry of the shale and sandstone suggests deposition in an active continental margin (Ustaömer & Robertson, Reference Ustaömer and Robertson1994). The thickness of the flysch is difficult to determine because of strong deformation but is in excess of 2000 m. In most outcrops the Akgöl Formation shows intense deformation by shearing and folding, and has been transformed into a broken formation (Fig. 11b). The wavelength of the folding is at the metre to decimetre scale. This intense deformation is not observed in the stratigraphically overlying Upper Jurassic sequences or in the Middle Jurassic intrusive rocks and hence is constrained to latest Triassic or Early Jurassic time. The Akgöl Formation is not metamorphosed; however, the illite crystallinity indicates high diagenetic conditions (Ustaömer & Robertson, Reference Ustaömer and Robertson1994).

Figure 11. Field photographs from the Triassic Küre Complex. (a) Distal turbidites of the Upper Triassic Akgöl Formation. (b) Sheared and folded turbidites of the Akgöl Formation; the light coloured block on the right is a sandstone boudin. (c) The Akgöl Formation intruded by the Middle Jurassic Karaman Granitoid. (d) Black shales of the Akgöl Formation overlain unconformably by the fluviatile red sandstone and conglomerate and by the shallow marine limestones of the Upper Jurassic (Kimmeridgian) İnaltı Formation. (e, f) The Late Triassic (Norian) bivalve Monotis salinaria in the siltstones of the Akgöl Formation in the Central Pontides (sample 1833) (e) and in the Tauric flysch in Crimea (location 54, Table S2 in the online Supplementary Material available at http://journals.cambridge.org/geo) (f). (g) Basaltic pillow lavas overlain stratigraphically by the black shales of the Akgöl Formation. (h) Black shale and basalt in the Küre mine; the chalcopyrite mineralization occurs in the shales along the contact. The height of the mine face is approximately 70 m.
The Akgöl Formation is intruded by Middle Jurassic (Bathonian–Callovian) dacite-porphyries and granitoids (Yılmaz & Boztuğ, Reference Yılmaz and Boztuğ1986; Okay et al. Reference Okay, Sunal, Tüysüz, Sherlock, Keskin and Kylander-Clark2014) and is unconformably overlain by the Upper Jurassic (Kimmeridgian) – Lower Cretaceous limestones (Fig. 11c, d), which provide an upper age limit. The lower age limit is set by the Carnian and Norian limestone blocks (Kozur et al. Reference Kozur, Aydın, Demir, Yakar, Göncüoğlu and Kuru2000; this study). Clastic zircons from a sandstone sample from the Akgöl Formation are dominated by Permian and Triassic zircons (Karslıoğlu et al. Reference Karslıoğlu, Ustaömer, Robertson and Peytcheva2012).
The only precise palaeontological data on the age of the Akgöl Formation, with photographs and information on location, is the trace fossil Torlessia sp., which indicates a Late Triassic (Carnian–Norian) age (Kozur et al. Reference Kozur, Aydın, Demir, Yakar, Göncüoğlu and Kuru2000). During our study we found a siltstone bed in the Akgöl Formation (location 1833 in Fig. 6, UTM coordinates 36T 0578661–4625270) containing thin-shelled bivalves identified as Monotis salinaria (Fig. 11e, identification by Leopold Krystyn), characteristic of the Norian (e.g. McRoberts, Reference McRoberts and Lucas2010). This constitutes the first precise fossil identification from the Akgöl Formation. The same bivalve species also occurs in the Tauric series in Crimea (Fig. 11f). In summary, the palaeontological data indicate a Late Triassic (Norian) age for the Akgöl Formation; its age may go into the Early Jurassic, as generally accepted for the Tauric flysch in Crimea.
4.c. Upper Triassic dismembered ophiolite
Associated with the turbidites of the Akgöl Formation there are thick sequences of basaltic pillow lavas and pillow breccias (Fig. 12; Bailey, Barnes & Hupfer, Reference Baıley, Barnes and Hupfer1967; Ustaömer & Robertson, Reference Ustaömer and Robertson1994). The basalts are tholeiitic and mostly of mid-ocean ridge (MORB) type with some analyses falling in the island-arc tholeiite field (Ustaömer & Robertson, Reference Ustaömer and Robertson1994). Most of the contacts between the basalt and turbidite are represented by faults; however, at a few localities basaltic pillow lavas and pillow breccias are overlain stratigraphically by black shales of the Akgöl Formation (Fig. 11g), as also observed by Bailey, Barnes & Hupfer (Reference Baıley, Barnes and Hupfer1967) and Ustaömer & Robertson (Reference Ustaömer and Robertson1994). The ancient Küre copper mine with chalcopyrite and pyrite as the main ore minerals is located along the contact between the basalt and black shale (Fig. 11h); the mineralization has developed mainly in the basalt (Bailey, Barnes & Hupfer, Reference Baıley, Barnes and Hupfer1967). Apart from the basalt, there are a few hundred-metre-sized bodies of gabbro and plagioclase-lherzolite within the basalts. The plagioclase-lherzolites consist mainly of olivine, plagioclase and clinopyroxene (Çakır, Genç & Paktunç, Reference Çakır, Genç and Paktunç2006) and are probably cumulate bodies.

Figure 12. Geological map and cross-section of the Küre region based on Bailey, Barnes & Hupfer (Reference Baıley, Barnes and Hupfer1967), O. Tüysüz et al. (unpub. report, 2000), Okay et al. (Reference Okay, Sunal, Tüysüz, Sherlock, Keskin and Kylander-Clark2014) and this study. For location see Figure 3.
Serpentinite occurs as large slices in the turbidites of the Akgöl Formation. Because of its significance as a possible mantle rock, the serpentinite was mapped in detail (Fig. 12). It occurs as up to 4 km long tectonic slices surrounded by shale and sandstone of the Akgöl Formation, and consists of completely serpentinized massive harzburgite. The lack of serpentinite clasts or debris flows in the surrounding sandstone–shale sequence indicates that the serpentinite was emplaced tectonically.
The age of the serpentinite is critical for the tectonic interpretation of the Akgöl Formation. Cretaceous ophiolitic melange with serpentinite slivers occurs 40 km south of the serpentinite outcrop (Fig. 3), and the serpentinite could have been emplaced any time after the Late Triassic and possibly during Cretaceous time. The serpentinite and the turbidites of the Akgöl Formation are intruded by the Karaman granitoid, which forms an intrusive body measuring 5 km by 5 km (Fig. 12). It is part of a series of Middle Jurassic intrusions emplaced along a major magmatic arc (Yılmaz & Boztuğ, Reference Yılmaz and Boztuğ1986; Okay et al. Reference Okay, Sunal, Tüysüz, Sherlock, Keskin and Kylander-Clark2014). The Karaman intrusion has a zonal structure with a core of medium-grained microgranodiorite to microdiorite surrounded by dacite-porphyry. The microgranodiorite, which makes up the bulk of the intrusion, has the mineral assemblage of plagioclase + biotite + quartz ± hornblende ± cordierite (Okay et al. Reference Okay, Sunal, Tüysüz, Sherlock, Keskin and Kylander-Clark2014). The marginal dacite-porphyry consists of plagioclase, biotite and quartz and locally hornblende phenocrysts in a fine-grained matrix of the same minerals. To constrain the age of the serpentinite, we dated ten biotite grains from a dacite-porphyry sample (1723), which gave a coherent age of 162 ± 4 Ma (Table 2; Okay et al. Reference Okay, Sunal, Tüysüz, Sherlock, Keskin and Kylander-Clark2014). This shows that the serpentinite was emplaced prior to Middle Jurassic time. Considering the association of serpentinite with the Upper Triassic turbidites and pillow lavas, a Triassic age is likely for the serpentinite. The serpentinite, gabbro and basalt most probably constitute a dismembered ophiolite on which the Upper Triassic to ?Lower Jurassic turbidites of the Akgöl Formation were deposited (Ustaömer & Robertson, Reference Ustaömer and Robertson1994).
5. Comparison with the Tauric Flysch in Crimea
Upper Triassic turbidites in the Central Pontides and those in Crimea share several common features. (1) In both regions the Upper Triassic sequence consists predominantly of distal siliciclastic turbidites, with a dominance of black shales. (2) At least part of the turbidite sequence is of Late Triassic (Norian) age based on the macrofauna. Monotis salinaria, a bivalve characteristic for the Norian, is found both in the Crimea and Central Pontides (Fig. 11e, f). The turbidite sequence in Crimea is thought to extend into the Lower Jurassic. (3) Clastic zircon populations from both regions are similar (Karslıoğlu et al. Reference Karslıoğlu, Ustaömer, Robertson and Peytcheva2012; A. Nikishin pers. comm.) with a dominance of Triassic and Permo-Carboniferous zircons. (4) The turbidites in the Crimea and Central Pontides have undergone a strong contractional deformation during latest Triassic – Early Jurassic time. (5) They are intruded or overlain by Middle Jurassic magmatic rocks. Upper Jurassic shallow marine carbonates lie also unconformably over the Triassic flysch. These features indicate that prior to the Late Cretaceous opening of the Black Sea as a back-arc basin (e.g. Okay, Şengör & Görür, Reference Okay, Şengör and Görür1994), the Upper Triassic turbidites in Crimea and in the Central Pontides were contiguous and were deposited in the same basin.
6. Discussion
6.a. Late Triassic fore-arc basin on the southern active margin of Laurasia
The pre-Permian basement in the Central Pontides consists of a low-grade metasedimentary sequence of interbedded sandstone and shale. The flyschoid character of the sequence and the presence of pre-Permian serpentinite slivers suggest deposition on a Palaeozoic active margin. The Palaeozoic metasedimentary rocks are intruded by the Late Carboniferous and Early Permian granitoids. A Variscan granitoidic basement in the Central Pontides is also reflected in the high percentage of Permian and Carboniferous zircons in the Triassic (Karslıoğlu et al. Reference Karslıoğlu, Ustaömer, Robertson and Peytcheva2012) and Lower Cretaceous turbidites (Okay et al. Reference Okay, Sunal, Sherlock, Altıner, Tüysüz, Kylander-Clark and Aygül2013). This crystalline basement was probably overlain by Triassic carbonates, represented by the Kayabaşı Formation in the Central Pontides. However, absence of Triassic carbonates along the contacts between the late Variscan basement and the Upper Triassic turbidites (Fig. 3) suggests a period of uplift and deformation during Carnian time. The buried Late Triassic volcanic arc in the Scythian Platform in the north (Fig. 1; Tikhomirov, Chalot-Prat & Nazarevich, Reference Tıkhomırov, Chalot-Prat and Nazarevıch2004) and Upper Triassic eclogites and blueschists in the southwest (Okay & Monié, Reference Okay and Monıé1997; Okay, Monod & Monié, Reference Okay, Monod and Monıé2002) suggest that the Upper Triassic turbidites were deposited in a fore-arc basin on the southern margin of Laurasia (Fig. 13a, b). In the north the fore-arc basin rested on continental crust and in the south on oceanic crust, similar to the Great Valley Group in California (e.g. Ingersoll, Reference Ingersoll1979).

Figure 13. Palaeogeographic map and cross-sections for the Late Triassic to Early Jurassic showing the palaeogeographic location and evolution of the tectonic units discussed in the text (based on Hamilton, Reference Hamilton1988; Barrier & Vrielynck, Reference Barrier and Vrielynck2008; Nikishin et al. Reference Nıkıshın, Zıegler, Bolotov and Fokın2012).
Disrupted Upper Triassic clastic sequences are widespread in the Sakarya Zone in NW Turkey, where they are known as the Upper Karakaya Complex (Fig. 1; Okay & Göncüoğlu, Reference Okay and Altıner2004). They contain olistoliths of Middle Triassic, as well as Permian and Carboniferous limestones in an Upper Triassic clastic matrix (Kaya, Reference Kaya1991; Wiedmann, Kozur, & Kaya, Reference Wiedmann, Kozur and Kaya1992; Leven & Okay, Reference Leven and Okay1996; Altıner, Özkan-Altıner & Koçyığıt, Reference Altıner, Özkan-Altıner, Koçyığıt, Bozkurt, Winchester and Piper2000). Within the Upper Triassic clastic sequences there are also rare blocks of Permian, Carboniferous and Devonian radiolarian cherts (Okay & Mostler, Reference Okay and Mostler1994; Kozur & Kaya, Reference Kozur and Kaya1994; Göncüoğlu et al. Reference Göncüoğlu, Kuwahara, Tekın and Turhan2004; Okay, Noble & Tekin, Reference Okay, Noble and Tekin2011). The Upper Karakaya Complex shares several common features with the Akgöl Formation of the Central Pontides, including tectonic setting, lithology and age, and was most probably deposited in same fore-arc on the southern margin of Laurasia. The Tauric flysch in Crimea and the Dizi Series in the Caucasus likely formed part of the same basinal deposits.
The Küre basin is commonly described as a back-arc basin (e.g. Ustaömer & Robertson, Reference Ustaömer and Robertson1993, Reference Ustaömer and Robertson1994; Barrier & Vrielynck, Reference Barrier and Vrielynck2008; Nikishin et al. Reference Nıkıshın, Zıegler, Bolotov and Fokın2012); this was based on the presence of ‘Permo-Triassic’ subduction–accretion complexes (Domuzdağı and Elekdağ complexes) and a Permo-Triassic magmatic arc (Çangaldağ Complex) south of the Küre Complex (Fig. 3). However, recent work has shown that the Domuzdağı and Elekdağ complexes are Early Cretaceous and the Çangaldağ Complex Middle Jurassic in age (Okay et al. Reference Okay, Tüysüz, Satır, Özkan-Altıner, Altıner, Sherlock and Eren2006, Reference Okay, Sunal, Sherlock, Altıner, Tüysüz, Kylander-Clark and Aygül2013, Reference Okay, Sunal, Tüysüz, Sherlock, Keskin and Kylander-Clark2014), and there is no Triassic or older unit between the Küre Complex and the İzmir–Ankara suture. Thus, during Triassic time the Küre basin was directly facing the Tethyan ocean in the south (Fig. 13).
6.b. Latest Triassic deformation and the Cimmeride orogeny
A transition from carbonate to clastic deposition is observed in Late Triassic time throughout the circum-Black Sea region, including in the western part of the Istanbul Zone (Yurttaş-Özdemir, Reference Yurttaş-Özdemir1971; Gedik, Reference Gedık1975), in Dobrugea (Seghedi, Reference Seghedı, Ziegler, Cavazza, Robertson and Crasquin-Soleau2001) and in the Balkans (Tari et al. Reference Tari, Dicea, Faulkerson, Georgiev, Popov, Stefanescu, Weir and Robinson1997). In latest Triassic and/or earliest Jurassic time, this clastic basin was inverted, and the circum-Black Sea region, including the Greater Caucasus and the Scythian Platform, was uplifted and eroded (e.g. Gaetani et al. Reference Gaetani, Garzanti, Polino, Kiricko, Korsakhov, Cirilli, Nicora, Rettori, Larghi and Palliani2005; Nikishin et al. Reference Nıkıshın, Zıegler, Bolotov and Fokın2012). In the Central Pontides this was associated with the emplacement of serpentinites, and in NW Turkey that of the uppermost Triassic eclogites and blueschists. The Cimmeride deformation was short lived; the unconformable sedimentary cover over the Cimmeride units is Early Jurassic (Sinemurian) in age in NW Turkey (Altıner et al. Reference Altıner, Koçyiğit, Farinacci, Nicosia and Conti1991). In the Central Pontides, the deformed Upper Triassic turbidites and the serpentinite are cut by undeformed Middle Jurassic (Bathonian–Callovian) acidic intrusions.
The Late Triassic deformation in the circum-Black Sea region was ascribed to the collision of a Cimmeride continent or continental blocks (e.g. Şengör, Reference Şengör1984). However, Triassic, Jurassic and Cretaceous accretionary complexes in the Pontides are directly juxtaposed without any intervening continental crustal material (Okay, Reference Okay, Bozkurt, Winchester and Piper2000; Çelik et al. Reference Çelik, Marzoli, Marschik, Chiaradia, Neubauer and Öz2011; Topuz et al. Reference Topuz, Göçmengıl, Rolland, Çelık, Zack and Schmıtt2013, in press). Furthermore, the brief period of deformation, constrained to c. 10 Ma in the latest Triassic – earliest Jurassic (Rhaetian–Hettangian), makes a continent–continent collision an unlikely cause for the Cimmeride orogeny.
During Late Triassic time, large thicknesses of mafic crustal material were accreted to the southern margin of Laurasia. These are known in the Sakarya Zone as the Lower Karakaya Complex or the Nilüfer Unit (Okay, Reference Okay, Bozkurt, Winchester and Piper2000; Okay & Göncüoğlu, Reference Okay and Göncüoğlu2004; Pickett & Robertson, Reference Pickett and Robertson2004; Robertson & Ustaömer, Reference Robertson and Ustaömer2012). The Lower Karakaya Complex shows metamorphism mainly to greenschist facies but also includes uppermost Triassic eclogites and blueschists (Okay & Monié, Reference Okay and Monıé1997; Okay, Monod & Monié, Reference Okay, Monod and Monıé2002). The geochemistry of the metabasites in the Lower Karakaya Complex shows within-plate characteristics; this is interpreted as formation in oceanic islands or in an oceanic plateau in Permo-Triassic time (Okay, Reference Okay, Bozkurt, Winchester and Piper2000; Genç, Reference Genç2004; Pickett & Roberson, Reference Pickett and Robertson2004; Sayit & Göncüoğlu, Reference Sayıt and Göncüoglu2013; Catlos, Hubert & Shin, Reference Catlos, Hubert and Shin2013). We suggest that the incipient collision of this oceanic edifice in Late Triassic time caused uplift along the Laurasian margin resulting in clastic sedimentation in the fore-arc region (Fig. 13c). This was followed by collision and accretion, which resulted in the inversion and deformation of the fore-arc basin.
7. Conclusions
(1) In the northern Central Pontides there is a pre-Permian basement of low-grade metasedimentary rocks with pre-Permian serpentinite lenses and intrusive Late Permian and Early Carboniferous granitoids (Figs 3, 4). The basement is overlain by the Upper Triassic turbidites. Similar basement rocks should be present under Crimea and under the Scythian Platform.
-
(2) In the Central Pontides a 550 m thick sequence of hemi-pelagic to pelagic Hallstatt-type limestone occurs as a large olistolith in the Upper Triassic turbidites. Conodonts and foraminifera indicate that the limestone sequence is Anisian to Carnian in age. The surrounding turbidites contain the bivalve Monotis salinaria, which indicates a Late Triassic (Norian) age for the clastic sequence. The same fossil is also described from the Tauric flysch in Crimea.
-
(3) The Upper Triassic turbidites are locally underlain by basaltic pillow lavas and contain kilometre-sized tectonic slices of serpentinite. The turbidites and the serpentinite are intruded by Middle Jurassic age (162 Ma) granitoids (Fig. 12), showing that the serpentinite is pre-Middle Jurassic in age. The Upper Triassic turbidites are deposited in a fore-arc basin above a northward-dipping subduction zone (Fig. 13). The Triassic magmatic arc is located subsurface in the Scythian Platform north of the Black Sea (Fig. 1).
-
(4) The Upper Triassic turbidites are strongly deformed by folding, faulting and shearing. This deformation is not observed in the Middle Jurassic magmatic rocks or in the overlying Upper Jurassic conglomerates and limestones, indicating that the deformation is of latest Triassic and/or Early Jurassic age.
-
(5) Data from the Central Pontides and regional geological constraints indicate that the latest Triassic to Early Jurassic Cimmeride deformation is related to accretion of large oceanic edifices to the southern margin of Laurasia rather than to a collision with a Cimmerian continent.
Acknowledgements
This study was supported by TÜBİTAK grant 109Y049 and partly by TÜBA. We thank Sarah Sherlock for the Ar–Ar analyses, Leopold Krystyn for discussion and palaeontological determination, Gürsel Sunal for assisting in mineral separation and Anatoly Nikishin for leading the field trip in Crimea. Andrea Zanchi and an anonymous reviewer are thanked for their pertinent and constructive comments.
Supplementary material
To view supplementary material for this article, please visit http://dx.doi.org/10.1017/S0016756814000429.