Introduction
The causes of global amphibian decline are diverse but the majority are anthropogenic (Carey & Alexander, Reference Carey and Alexander2003; Beebee & Griffiths, Reference Beebee and Griffiths2005, Brum et al., Reference Brum, Gonçalves, Cappelatti, Carlucci, Debastiani and Salengue2013). Given the complexity of ecological processes the causes of species decline are typically studied and managed in isolation but multiple threats, such as climate change and habitat loss (Mantyka-Pringle et al., Reference Mantyka-Pringle, Martin and Rhodes2012), can affect species and ecosystems concurrently (Rohr et al., Reference Rohr, Raffel, Romansic, McCallum and Hudson2008). Furthermore, changes in climate could modify dispersion and infection characteristics of the chytrid fungus, Batrachochytrium dendrobatidis (Pounds et al., Reference Pounds, Bustamante, Coloma, Consuegra, Fogden and Foster2006; Rödder et al., Reference Rödder, Kielgast and Lötters2010). Although a global estimate of areas at risk from the occurrence of these threats has been developed (Hof et al., Reference Hof, Araújo, Jetz and Rahbek2011), variation in local conditions and species necessitates greater precision in identifying priority areas for conservation and management (Mantyka-Pringle et al., Reference Mantyka-Pringle, Martin, Moffatt, Linke and Rhodes2014).
Peltophryne longinasus is a bufonid endemic to Cuba and is a uniquely polytypic species of the genus in the Caribbean basin (Henderson & Powell, Reference Henderson and Powell2009). There are four recognized subspecies, with disjunct distributions in mountainous areas (Díaz & Cádiz, Reference Díaz and Cádiz2008). Peltophryne longinasus longinasus and P. longinasus cajalbanensis inhabit the Alturas de Pizarras in the south of Pinar del Río province and Cajálbana upland, respectively. Peltophryne longinasus dunni is distributed in the Guamuhaya mountain range in the centre of the island and is threatened by the chytrid fungus (Díaz et al., Reference Díaz, Cádiz, Chong and Silva2007). Although only one sick and dying individual has been found, in October 2006, the presence of the disease in this population was confirmed. Since that date no more cases have been reported. Peltophryne longinasus ramsdeni is known only from the Guaso upland in Guantanamo province, in eastern Cuba, but it has not been found since 1976 (Valdes de la Osa & Ruiz García, Reference Valdés de la Osa and Ruiz García1980).
This diurnal species occupies a variety of vegetation types, mainly woodlands (pinewoods and mesic broadleaf forests) and secondary vegetation, at altitudes of 100–820 m (Valdes de la Osa & Ruiz García, Reference Valdés de la Osa and Ruiz García1980; Díaz & Cádiz, Reference Díaz and Cádiz2008), and reproduces in mountain streams (Valdés de la Osa & Ruiz García, Reference Valdés de la Osa and Ruiz García1980). Peltophryne longinasus is categorized as Endangered on the IUCN Red List because its Area of Occupancy is < 500 km2, its distribution is severely fragmented and the extent of its upland forest habitat is declining (Hedges & Díaz, Reference Hedges and Díaz2004). Its threat status, ecological and morphological features and disjunct distribution make it an ideal species to evaluate the additive threats of climate change and habitat loss.
Habitat fragmentation and climate change have been recognized as two principal threats to biodiversity in Cuba (CITMA, 2014) but their possible additive impacts on amphibian diversity have received little consideration. Here we (1) evaluate the potential impact of predicted climate change scenarios for 2050 and 2070 on habitats suitable for P. longinasus, (2) estimate habitat loss (measured as forest lost) during 2000–2012 in the areas suitable for this species, (3) identify suitable areas for P. longinasus affected by additive stressors (habitat loss, disease and climate change), and (4) assess the representation of suitable areas for the species (for 2000, 2050 and 2070 scenarios) and the threats it faces within Cuban protected areas.
Study area
The study area (the Cuban archipelago) was divided into four physical–geographical regions (Fig. 1), simplifying the regionalization of Mateo & Acevedo (Reference Mateo, Acevedo, Oliva, Lluís and Sánchez1989). Regions inhabited by P. longinasus (western, central and eastern Cuba) comprise the more densely forested areas of the archipelago (Mateo & Acevedo, Reference Mateo, Acevedo, Oliva, Lluís and Sánchez1989).

Fig. 1 Distribution of records of Peltophryne longinasus in Cuba, which is divided into physical–geographical regions, based on Mateo & Acevedo (Reference Mateo, Acevedo, Oliva, Lluís and Sánchez1989).
Methods
We used ecological niche models to identify areas suitable for P. longinasus and predict future changes in these areas. We evaluated recent forest loss using the results of Hansen et al. (Reference Hansen, Potapov, Moore, Hancher, Turubanova and Tyukavina2013), to identify patterns and levels of habitat degradation. We also assessed climate change in areas where suitable conditions will decline in the future, using the bioclimatic variables of the WorldClim database (Hijmans et al., Reference Hijmans, Cameron, Parra, Jones and Jarvis2005). With the resultant information we identified suitable areas potentially affected by additive threats from climate change, habitat loss and chytridiomycosis. We also evaluated the representation of the suitable areas and threats in protected areas.
Climatic data
The modelling dataset included 13 bioclimatic variables (Table 2) obtained from the WorldClim database version 1.4 (S4 data group) at a resolution of 30 arc-seconds (Hijmans et al., Reference Hijmans, Cameron, Parra, Jones and Jarvis2005). We selected these variables on the assumption that they are most strongly related to the natural history of the studied species. We did not check for collinearity among our selected variables. We followed the criteria of Braunisch et al. (Reference Braunisch, Coppes, Arlettaz, Suchant, Schmid and Bollmann2013), who stated that the inclusion of several correlated, potentially relevant variables in the same model may be preferable when the intention is to predict rather than infer, as it may lead to a ‘less wrong’ result than when operating with only a single, irrelevant predictor arbitrarily.
To evaluate the effect of climate change by 2050 and 2070 on the potential distribution of P. longinasus we used two Representative Concentration Pathway (RCP) scenarios (2.6 and 8.5) of the general circulation model of the Beijing Climate Center. These scenarios describe two climatic configurations based on different concentrations of greenhouse gases (Moss et al., Reference Moss, Babiker, Brinkman, Calvo, Carter and Edmonds2008). The RCP 2.6 scenario assumes that concentrations of greenhouse gases will increase in the future, reaching a maximum by 2040, and the RCP 8.5 assumes that concentrations will continue to increase even up to 2100 (IPCC, Reference Stocker, Qin, Plattner, Tignor, Allen and Boschung2013). Future bioclimatic variables were obtained from the WorldClim database.
Habitat suitability models and projections
We used distribution records (Alonso, Reference Alonso2011) and the aforementioned bioclimatic variables as presence and environmental data. To prevent possible errors duplicate records were removed. With the remaining 20 samples and selected variables we created habitat suitability models in MaxEnt 3.3.3k (Phillips et al., Reference Phillips, Anderson and Schapire2006), using its default configuration and 50 replicates for bootstrapping. To obtain presence–absence data from the mean logistic model we used the 10th percentile threshold (Peterson et al., Reference Peterson, Papes and Eaton2007), which should not be sensitive to extreme environmental values (Radosavljevic & Anderson, Reference Radosavljevic and Anderson2014). We consider it to be appropriate because a small sample size could increase the weight of extreme records in the model (Araujo & Peterson, Reference Araújo and Peterson2012) and consequently the estimated area of suitable habitat for the species. To evaluate model performance we used the area under the receiver operating characteristic curve (AUC; Phillips et al., Reference Phillips, Anderson and Schapire2006), difference of AUC (AUCDIFF; Warren & Seifert, Reference Warren and Seifert2011) and 10% training omission rate (Peterson et al., Reference Peterson, Soberón, Pearson, Anderson, Martínez-Meyer, Nakamura and Araújo2011).
Habitat suitability models created for the year 2000 were projected to 2050 and 2070 using MaxEnt. Results of each projection were compared with the initial model, considering the change in suitable areas (presence areas). For the future projections we identified areas where suitable climatic conditions for P. longinasus could be lost, suitable areas consistent with compared scenarios, and potentially new suitable areas. The conserved range is the area of overlap between each of the projected ranges and the 2000 range.
Where a loss of suitable habitat was predicted we used QGIS 2.8 (QGIS Development Team, 2015) to calculate differences in variables between 2000 and projected values for 2050 and 2070 (using all pixel values) for the two scenarios (RCP 2.6 and 8.5).
We used data from the Global Forest Change database (Hansen et al., Reference Hansen, Potapov, Moore, Hancher, Turubanova and Tyukavina2013) to evaluate loss of forest cover, which we considered to be an indicator of fragmentation and habitat loss. We estimated annual forest loss (resolution 30 m) in the quadrants that include the Cuban archipelago, and extracted the areas of interest in the layer composition of forest cover loss (2000–2012), using the suitable areas identified by habitat suitability modelling. In QGIS we calculated the surface area of deteriorated areas by year for each region and for all areas of suitable habitat, attempting to discriminate between vegetation types according to the vegetation map of Estrada et al. (Reference Estrada, Martín, Martínez, Vioel, Capote and Reyes2011).
To detect areas affected by additive stressors we created a map of known distribution records of P. longinasus subspecies, projected climate change, trends in temperature and precipitation, habitat loss (density of forest cover loss), and reported presence of Batrachochytrium dendrobatidis. Potential impacts of climate change were categorized as High, Medium or Low (based on projected habitat loss), and were compared among regions. We also identified areas predicted to become more suitable for P. longinasus as a result of climate change.
We assessed the importance of protected areas for conservation of P. longinasus and its habitats using the map of Cuba's national system of protected areas (CNAP, 2013). We calculated the area of current and future suitable habitat for the species within protected areas. We also estimated the area of suitable habitat that will potentially be lost under future climate scenarios. Finally, we compared the areas of forest loss in suitable habitat areas inside and outside protected areas in each region of the study area.
Results
Habitat suitability models
Habitat suitability models yielded high mean values of AUC (0.98 ± SD 0.03), low AUCDIFF (0.02 ± SD 0.05) and a mean 10% training omission rate of 0.053 ± SD 0.02. The AUC results indicate good predictive power, and AUCDIFF and the 10% training omission rate indicate that overfitting could be avoided in these models. The major contributing bioclimatic variables were annual mean temperature (BIO1), maximum temperature of warmest month (BIO5), and temperature seasonality (BIO4). The logistic output for 2000 revealed a high probability of presence of P. longinasus in mountain zones in all three regions inhabited by the species. In the initial binary results (Fig. 2a,b) derived from reclassification of the mean logistic model (probability of presence > 0.24 = mean 10th percentile threshold) we observed a fragmented pattern with suitable areas in all regions except Camagüey-Maniabón.
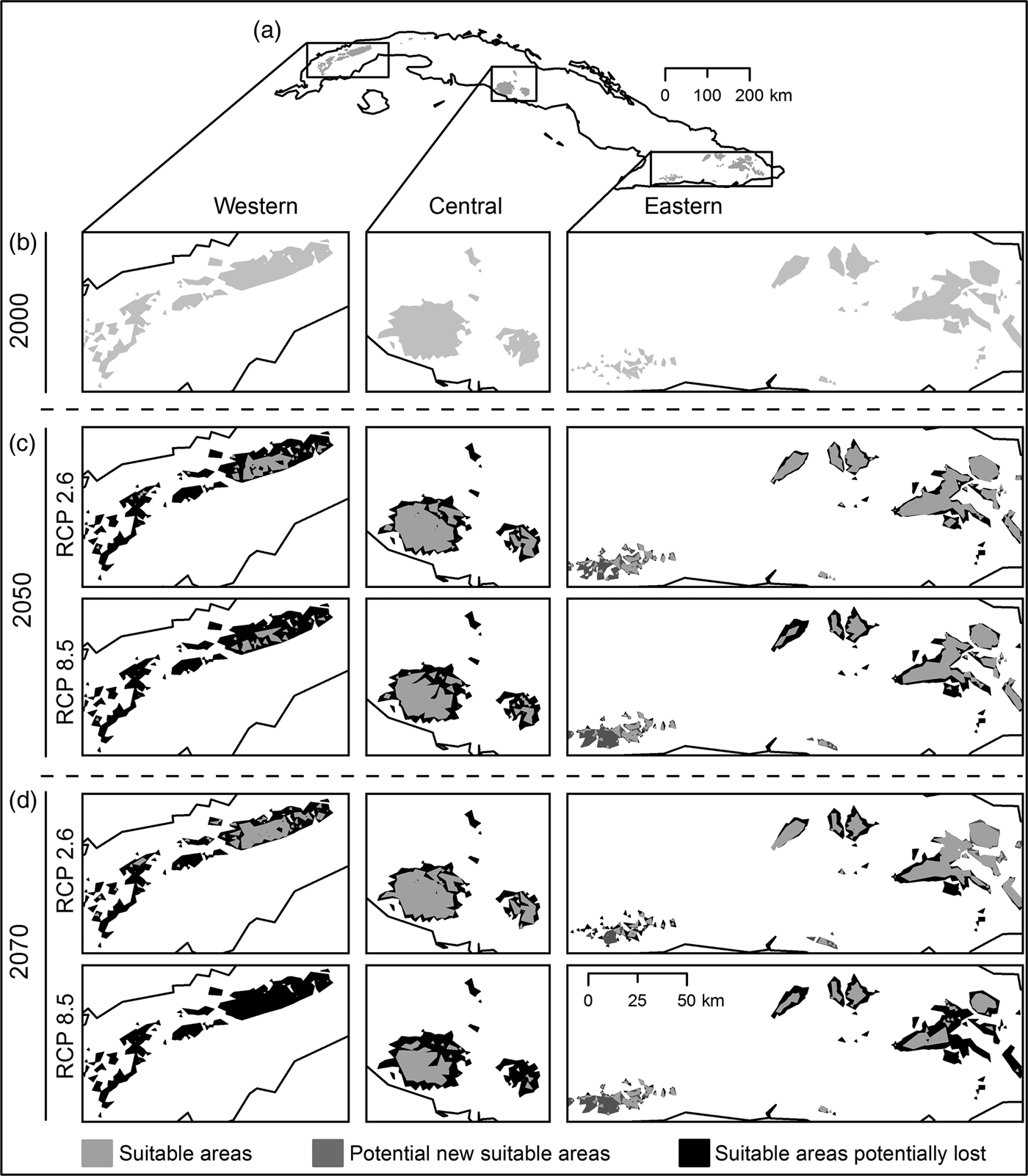
Fig. 2 Result of habitat suitability models for Peltophryne longinasus: Potential distribution range of Peltophryne longinasus for 2000 (a & b). Predicted change in species potential distribution ranges by (c) 2050 and (d) 2070.
Potential modifications of suitable areas
Suitable areas for P. longinasus (Fig. 2a,b) in 2000 represented 2.98% of all Cuban territory, with substantial decreases predicted by future projections (Fig. 2c,d). By 2050 losses of 51.03% (RCP 2.6) and 62.64% (RCP 8.5) of suitable habitat are expected, and the western region will be most affected, with reductions of 84.5 and 93.46% in the respective scenarios (Table 1). Projections for 2070 are similar, with decreases of 48.98% (RCP 2.6) and 78.23% (RCP 8.5). However, in the Sierra Maestra mountain range (eastern region) the area of suitable habitat is predicted to increase significantly in scenario RCP 8.5 (Fig. 2c,d), but P. longinasus has never been found there. In scenario RCP 2.6, even though suitable habitat is predicted to diminish by 2050, an improvement relative to 2050 is expected by 2070, especially in the central and western regions. The conserved range (suitable areas consistent with compared scenarios) will be proportionally higher in the central and eastern regions in comparison with the western region (Table 1). Suitable areas are expected to be found at higher altitudes in the future (relative to 2000). By 2050 mean altitudinal increases of 197.22 m (RCP 2.6) and 279.38 m (RCP 8.5) are projected. By 2070 the altitude of suitable habitat could increase by 148 m (RCP 2.6) and 349.50 m (RCP 8.5) relative to 2000.
Table 1 The potential distribution range of the Cuban toad Peltophryne longinasus in western, central and eastern Cuba in 2000, and projected values for 2050 and 2070 under two climate change scenarios (RCP 2.6 and RCP 8.5). The conserved range is the area of overlap between each of the projected ranges with the 2000 range.

* RCP 2.6 assumes that concentrations of greenhouse gases will increase in the future, reaching a maximum by 2040, and RCP 8.5 assumes that concentrations will continue to increase even up to 2100.
Expected climate change in areas predicted to become unsuitable for P. longinasus
In areas where suitable conditions for the species will be lost, values of all temperature variables (except mean diurnal range, BIO2) are expected to increase in both climate scenarios (Table 2). Significant increases of temperature and decreases of three of six precipitation variables by 2070 are predicted (RCP 8.5). Precipitation in the driest month and driest quarter (BIO14 and BIO 17) is predicted to decrease significantly by 2070 (RCP 2.6). Similarly, a reduction in precipitation seasonality (coefficient of variation) by 2050 is expected (RCP 2.6). We also recorded increments in precipitation in the wettest month (BIO13) and precipitation seasonality (BIO15) by 2050 (RCP 8.5) and 2070 (RCP 2.6) (Table 2).
Table 2 Mean values (min., max.) of bioclimatic variables for the initial (2000) distribution range of P. longinasus, and differences between projected values for 2050 and 2070 under two climate scenarios (RCP 2.6 and RCP 8.5) and 2000 values.

Habitat loss in areas that were suitable in 2000
Total initial forest cover (2000) in areas suitable for P. longinasus comprised 3,043.75 km2, with the majority of the vegetation distributed in the eastern region (Fig. 3). The most significant forest loss during 2000–2012 took place in the western region (Figs 3 & 4), where major losses were recorded in 2008. Significant reductions in forest cover occurred in the central and eastern regions in 2005 and 2002, respectively (Fig. 3b). Peltophryne longinasus has been recorded in at least eight distinct vegetation formations (Supplementary Table S1). These formations occupy 37.27% of the species’ total suitable area. We identified that secondary vegetation, Pinus caribaea and P. tropicalis pinewoods, were most affected in the western region, submontane mesophyllous evergreen forests and secondary vegetation in the central, and submontane mesophyllous evergreen forests in the eastern region.

Fig. 3 (a) Forest cover in the potential distribution range of Peltophryne longinasus in 2000, and total forest loss during 2000–2012, in the western, central and eastern regions of Cuba (Fig. 1). (b) Forest loss per year during 2001–2012 in each region.

Fig. 4 Density of forest cover loss during 2000–2012 in areas of initially suitable habitat for Peltophryne longinasus in the western, central and eastern regions of Cuba.
Areas affected by additive stressors
Predicted future trends include an increase in temperature and a decrease in precipitation throughout the entire area of suitable habitat for P. longinasus. Three types of additive threats have also been identified: climate change and habitat loss, climate change and chytridiomycosis, and habitat loss and chytridiomycosis. The first combination was detected throughout the species’ range (Fig. 5) but the level of impact varied among regions, and therefore the subspecies will be affected differentially. The greatest impacts of climate change with habitat loss were observed in the western region, intermediate impacts were detected in the eastern region, and the lowest impact was observed in the central region. The second and third types of additive threats appear to occur only in the central region, as a result of the reported presence of chytridiomycosis in this zone and the absence of connectivity with other regions.

Fig. 5 Additive stressors of climate change and habitat loss on the distribution range of Peltophryne longinasus in Cuba.
Importance of protected areas
The current percentage of suitable habitat area for P. longinasus (Fig. 2b) within protected areas is 36.6% but this is expected to decrease under all future scenarios (Supplementary Table S2). The eastern region had the highest initial and future representations of suitable habitat within protected areas in all evaluated scenarios. We predict that by 2050 (RCP 2.6) suitable areas within protected areas will be reduced by 30.15%, and by 2070 24.99%. In the RCP 8.5 scenario we predict reductions of 29.26 and 56.23% by 2050 and 2070, respectively. Nevertheless, the majority of the reduction in suitable areas in the future (Fig. 2c,d) will occur outside protected areas, with only 26.34–32.77% of losses occurring within protected areas (Supplementary Table S3). We detected considerable differences in forest loss during 2000–2012 inside and outside protected areas (Supplementary Table S4), with only 8.6% of the forest loss occurring inside protected areas.
Discussion
Our results indicate that, in the future, areas of habitat suitable for P. longinasus will be reduced. Similar studies have reported spatial changes in suitable areas (decrease and displacement of ranges) for many herpetofaunal species in Europe and South America (Araújo et al., Reference Araújo, Thuiller and Pearson2006; Zank et al., Reference Zank, Becker, Abadie, Baldo, Maneyro and Borges-Martins2014; Courtois et al., Reference Courtois, Michel, Martinez, Pineau, Dewynter, Ficetola and Fouquet2016). Suitable areas for P. longinasus will be found at high altitudes; this concurs with the general evidence of changes in species’ distributions in response to global warming (Walther et al., Reference Walther, Post, Convey, Menzel, Parmesan and Beebee2002; Raxworthy et al., Reference Raxworthy, Pearson, Rabibisoa, Rakotondrazafy, Ramanamanjato and Raselimanana2008). However, the archipelagic nature of Cuba, the lack of connectivity among ranges and the presence of barriers will impede future movement of the subspecies of P. longinasus to places with suitable conditions at other latitudes (i.e. the Sierra Maestra mountain range). Displacement to adjacent locations at higher altitudes within the same region is therefore the most likely alternative.
Not all subspecies have the same opportunity and ability to move to higher altitudes in the same region. Our results suggest that P. longinasus dunni has more opportunities for displacement because suitable areas adjacent to its current range will be less affected compared to other subspecies in other regions. Further studies on movement patterns are needed to investigate the capability of each subspecies to move to higher altitudes, and to obtain a better understanding of the impact of climate change on this species sensu lato.
Projected decreases in precipitation could also affect the typical reproductive phenology of P. longinasus. Such decreases could reduce the spatial and temporal accessibility of sites for calling and breeding, and also affect nesting success and optimum larval development. In the microhabitat of P. longinasus longinasus air temperature is 26–31°C (Díaz & Cádiz, Reference Díaz and Cádiz2006) and forecasted changes in bioclimatic variables include temperature changes. At the biological level these could generate variations in physiology, vocal behaviour and the availability and accessibility of prey, and could potentially increase the risk of predation (Blaustein et al., Reference Blaustein, Han, Relyea, Johnson, Buck, Gervasi and Kats2011; Li et al., Reference Li, Cohen and Rohr2013).
Amphibian species with small ranges tend to have more habitat specificity, which makes them vulnerable to habitat alterations (Williams & Hero, Reference Williams and Hero1998). The deterioration in forest cover is resulting in loss and fragmentation of habitats of P. longinasus in some of its most frequented vegetation types (e.g. Pinus forests). Although we have taken into account a period of only 12 years prior to our future projections, there was no apparent decrease in deforestation within this period, and deforestation is likely to continue, especially outside protected areas. In fragmented landscapes dispersion is often insufficient to maintain population viability (Cushman, Reference Cushman2006), and processes such as habitat split are common in fragmented landscapes with complex topography (Becker et al., Reference Becker, Fonseca, Haddad and Prado2010). Forest cover loss is therefore a significant concern, as it has been shown that habitat split, loss and fragmentation affect principally species with aquatic larvae (Fonseca et al., Reference Fonseca, Becker, Haddad and Prado2008).
Other studies have suggested potential synergistic effects of climate change and other stressors when they co-occur (Pyke, Reference Pyke2004; Brook et al., Reference Brook, Sodhi and Bradshaw2008; Mantyka-Pringle et al., Reference Mantyka-Pringle, Martin and Rhodes2012). We have identified areas where additive effects of global warming and habitat loss could be occurring, alerting us to potential synergies between these threats (Fig. 5).
Peltophryne longinasus longinasus and P. longinasus cajalbanensis will face major risks from the additive effects of global warming and habitat degradation. Peltophryne longinasus dunni (which may represent a distinctive form and is probably a ninth species of Cuban Peltophryne; Alonso et al., Reference Alonso, Crawford and Bermingham2012) is the subspecies with the lowest level of risk linked to these threats; however, it may also be threatened by the presence of the chytrid fungus. This pathogen disperses rapidly (Lips et al., Reference Lips, Diffendorfer, Mendelson and Sears2008) and its spread may be facilitated by anthropogenic activities (Weldon et al., Reference Weldon, Du Preez, Hyatt, Muller and Spears2004; Fisher & Garner, Reference Fisher and Garner2007). Establishment and intensity of infection are highest in elevated places with lower temperatures and higher precipitation (Drew et al., Reference Drew, Allen and Allen2006). Nevertheless, there is evidence that warmer years favour the development of the fungus at high elevations (Pounds et al., Reference Pounds, Bustamante, Coloma, Consuegra, Fogden and Foster2006; Harvell et al., Reference Harvell, Altizer, Cattadori, Harrington and Weil2009). Peltophryne longinasus ramsdeni will suffer intermediate effects in comparison with the other three subspecies. The almost non-existent possibility of migration to new, potentially suitable areas and the absence of records of this subspecies since 1976 justify its highly threatened status. However, it cannot yet be categorized as Extinct, given the few observations in the field and the lack of information on its biology, population size and habitat use. Based on our precautionary criteria we therefore categorize this subspecies as Critically Endangered, Possibly Extinct (CR A2ac; IUCN, 2015), until further surveys confirm, or not, that no individuals remain.
Our results show that all subspecies of P. longinasus are threatened; however, each one requires different research and management priorities according to the identified impacts. Habitat protection and restoration efforts could be a priority, especially in western areas where the major risks are potentially the additive effects of climate change and habitat loss. Further efforts are required to clarify the existence, prevalence, dissemination and pathogenicity of the chytrid fungus in relation to other natural and anthropogenic stressors in the central region. Some ex situ conservation initiatives began 10 years ago with a preliminary captive breeding protocol for P. longinasus longinasus (Díaz & Cádiz, Reference Díaz and Cádiz2006). However, the differences in ecology and behaviour of subspecies (Valdés de la Osa & Ruiz García, Reference Valdés de la Osa and Ruiz García1980) necessitate the development of variants of this protocol.
Amphibians are the group with the most species whose ranges fall completely outside protected areas (Nori et al., Reference Nori, Lemes, Urbina-Cardona, Baldo, Lescano and Loyola2015). Although the original design of Cuba's national system of protected areas does not provide adequate protection for amphibians (Fong et al., Reference Fong, Dávila and López-Iborra2015) and fails in the representation of suitable areas for P. longinasus (Supplementary Table S3), it appears to have contributed to avoiding excessive forest loss (Supplementary Table S4).
Integration of multiple approaches and perspectives is needed to obtain more accurate information about how species and habitats may respond to climate change (Dawson et al., Reference Dawson, Jackson, House, Prentice and Mace2011). The synergies between ecological/life history traits and environmental conditions demonstrate how a management strategy focused on multiple, diverse threats is a necessary precursor to any successful conservation action (Sodhi et al., Reference Sodhi, Bickford, Diesmos, Lee, Koh and Brook2008). This, together with adequate monitoring (e.g. Courtois et al., Reference Courtois, Michel, Martinez, Pineau, Dewynter, Ficetola and Fouquet2016), constitutes a basis for appropriate management decisions to conserve P. longinasus.
Acknowledgements
Many people helped RAB during the field expeditions to locate and verify the presence of the Cuban toad: Ariel Rodríguez, L. Yusnaviel García, Arturo Hernández, Luis Alvarez Lajonchere, and Rafael García-Morera. RAB thanks the National Center of Cuban Protected Areas and the Center for Inspection and Environmental Control for support and permission to access the study areas. Rafael Marquez and Chrystal S. Mantyka-Pringle provided comments on the text. Boris Fabres and Sergio J. Pastrana helped to improve the English. Prof. Tim Halliday offered valuable suggestions and corrections to the language, and helped enhance the quality of the article. Two anonymous reviewers provided constructive criticism. MEC is grateful for the support received during his postgraduate studies from the Secretaria Nacional de Educación Superior, Ciencia y Tecnología and the Instituto de Fomento del Talento Humano.
Author contributions
MEC and RAB conceived the study and wrote the article. RAB conducted all fieldwork and compiled the original database of geographical localities. MEC analysed the data and performed the modelling.
Biographical sketches
Marlon E. Cobos’ research interests are focused on the biogeography, ecology and conservation of tropical fauna, particularly amphibians. Roberto Alonso Bosch’s research interests are focused on the natural history, behaviour, evolution and conservation of Caribbean amphibians and reptiles, with special emphasis on the endemic toads of Cuba.