Introduction
The period between c. ad 800 and 1200 saw dramatic changes in farming practices across large parts of Europe. These sustained an increase in cereal production so great that it has been described as an ‘agricultural revolution’ which fuelled population growth and underpinned the expansion of towns and markets as well as the rise of lordship (White, Reference White1940; Duby, Reference Duby1954; Dyer et al., Reference Dyer, Thoen, Williamson, Dyer, Thoen and Williamson2018). Three key innovations made this increase in yields possible: two- and three-field crop rotation, which enabled a larger proportion of arable land to be cultivated; widespread adoption of the mouldboard plough, allowing farmers to cultivate heavier, more fertile soils; and low-input cultivation regimes, in which fertility was maintained by regular, short fallow periods during which sheep grazed on stubble and weeds, rather than by intensive manuring. This enabled farmers to extend the area of land under cultivation by decreasing input, i.e. labour and manure, per unit area, a process referred to here as ‘extensification’. The result was much larger overall yields, despite a decline in yield per land unit. In many regions (including around one-third of England and much of Europe), these innovations culminated in the introduction of ‘open fields’, in which the holdings of individual farmers took the form of strips dispersed across several large, unenclosed areas of arable land, intermingled with those of their neighbours.
To operate this more productive system, farmers shared expensive resources such as teams of oxen and mouldboard ploughs, and the cultivation of the open fields had to be agreed and managed communally. The need to coordinate cultivation activities is widely thought to lie behind the formation of the nucleated villages that still characterize many parts of the countryside today. In this way, innovations in farming transformed not only large parts of England's landscape, but also its social geography. When, where, and how this unprecedented form of agriculture emerged, however, and whether it had a significant impact before the Norman Conquest, remain contentious issues, largely because scholars have been forced to rely on a limited range of indirect evidence, written and archaeological (Banham & Faith, Reference Banham and Faith2014; Hall, Reference Hall2014; Dyer et al., Reference Dyer, Thoen, Williamson, Dyer, Thoen and Williamson2018). The aim of the ‘Feeding Anglo-Saxon England’ project (hereafter FeedSax) is to break this impasse by generating the first direct evidence for the conditions in which medieval cereals were grown by subjecting plant and animal remains from archaeological contexts to a range of scientific analyses, allowing the associated farming regimes to be reconstructed.
FeedSax is deploying a range of analyses in conjunction to trace the development of farming regimes involving crop rotation, use of the mouldboard plough and low-input cultivation. Analysis of stable isotopes in preserved cereal grains is being used to establish the conditions in which crops were grown, whether in light, dry soils or heavy, wetter soils, the degree to which productivity was boosted by manuring, and whether crop rotation was practised. Analysis of the associated weed flora is providing further indications of soil fertility and disturbance, reflecting the extent to which fields were manured and tilled, as well as likely sowing times. The lower limb bones of cattle are being examined for the kinds of pathologies that can be caused by pulling a heavy plough, while other zooarchaeological data are providing evidence of changing patterns of animal husbandry. Pollen data are being analysed to gauge the impact of cereal farming on the medieval landscape more widely, for example, on the ratio of arable to pasture, and on the overall scale of agricultural land use. Patterns emerging from these bioarchaeological data are being compared with evidence from excavated settlements—buildings, enclosures, droveways, etc.—to explore the inter-relationship between arable production, stock management, and settlement forms (see Hamerow, Reference Hamerow2012). A suite of radiocarbon dates on charred cereals, bones, and pollen cores is being used to locate the spread of low-input farming practices in time as well as space.
A national database of charred plant remains from this period is being compiled as part of the project, while plant and faunal remains from a series of case studies are being examined in more detail. These case studies span several ecological zones, within and beyond the core area of open fields and nucleated villages, sometimes referred to as the ‘central province’ (Roberts & Wrathmell, Reference Roberts and Wrathmell2000). The purpose of this article is to present one of these case studies, that of Stafford, as a ‘worked example’ to illustrate how results from these various analyses can be integrated.
Anglo-Saxon and Medieval Stafford
Stafford provides a particularly useful case study: modern, well recorded excavations at several locations in the present-day town have produced plant remains spanning the late ninth to thirteenth centuries, and local pollen data are relatively abundant. The faunal remains, on the other hand, are poorly preserved; it has, therefore, not been possible to conduct statistically meaningful analysis of lower limb bones and other pathologies. Stafford's early history is comparatively well documented. According to the Anglo-Saxon Chronicle, a burh (fortified settlement) was established at Stafford in ad 913 by Aethelflaed of Mercia. Stafford's earlier history is not recorded and excavations in the 1980s at several sites within the medieval town revealed no conclusive evidence for Mid-Saxon (seventh- to ninth-century) occupation (Carver, Reference Carver2010: 31, 39). Evidence was similarly lacking for post-Conquest activity, and it was argued that Stafford must have been virtually abandoned until a revival of activity in the late twelfth century (Carver, Reference Carver2010: 107–08).
This view was revised following excavations at Tipping Street in the south-eastern part of the medieval town in 2009. These uncovered three pottery kilns that produced substantial quantities of charred plant remains, probably representing crop processing waste used as fuel (Dodd et al., Reference Dodd, Goodwin, Griffiths, Norton, Poole and Teague2014: 72). Six radiocarbon dates from these kilns indicate that they probably came into use during the ninth century, suggesting that Stafford was well established before Aethelflaed fortified the site in ad 913, although the chronology of this earliest phase of activity has had to be revised again in light of a series of new dates obtained by the FeedSax project (see below; Dodd et al., Reference Dodd, Goodwin, Griffiths, Norton, Poole and Teague2014: 79–85, 100–05). It is therefore possible that Stafford was already a centre, perhaps established by Mercian royal initiative, where agricultural surpluses were being collected and processed at the time of its fortification in 913 (Dodd et al., Reference Dodd, Goodwin, Griffiths, Norton, Poole and Teague2014; Blair, Reference Blair2018: 263–64).
The place-name Stafford, meaning ‘landing-place ford’, refers to the town's location at the intersection of land-based and riverine transport routes, on what is effectively a peninsula surrounded by marshy land (Dodd et al., Reference Dodd, Goodwin, Griffiths, Norton, Poole and Teague2014). It also lies at the interface of light soils associated with the river terraces and heavier clays. Stafford's immediate hinterland is thus well suited for crop husbandry and there is good evidence for its history as an arable landscape. Several sites of (undated) ridge-and-furrow earthworks formed by mouldboard plough cultivation and marking the location of medieval fields have been identified in aerial photographs (Cotswold Archaeology, 2010) (Figure 1). As one would expect, given the likely role of ridge-and-furrow in improving drainage, nearly all are on heavier soils. A concentration of ridge-and-furrow lay less than 2 km north of the burh, near the deserted medieval village of Marston (the place-name suggesting a settlement near wet ground; Cotswold Archaeology, 2010). Indeed, Speed's 1610 map of Stafford depicts a farmer and plough team at work in fields apparently to the north of the town (Figure 2). The ready availability of chaff, seemingly used as fuel, suggests furthermore that crops were threshed and winnowed in the immediate vicinity of the town; these activities are usually undertaken close to arable fields to reduce the bulk and weight of the crop prior to transport. Most of the charred plant remains analysed here are therefore likely to have been grown close to Stafford (Moffett, Reference Moffett2010; Dodd et al., Reference Dodd, Goodwin, Griffiths, Norton, Poole and Teague2014: 73).

Figure 1. Location map of Stafford and the surrounding region showing key sites, soil types, and preserved ridge-and-furrow.
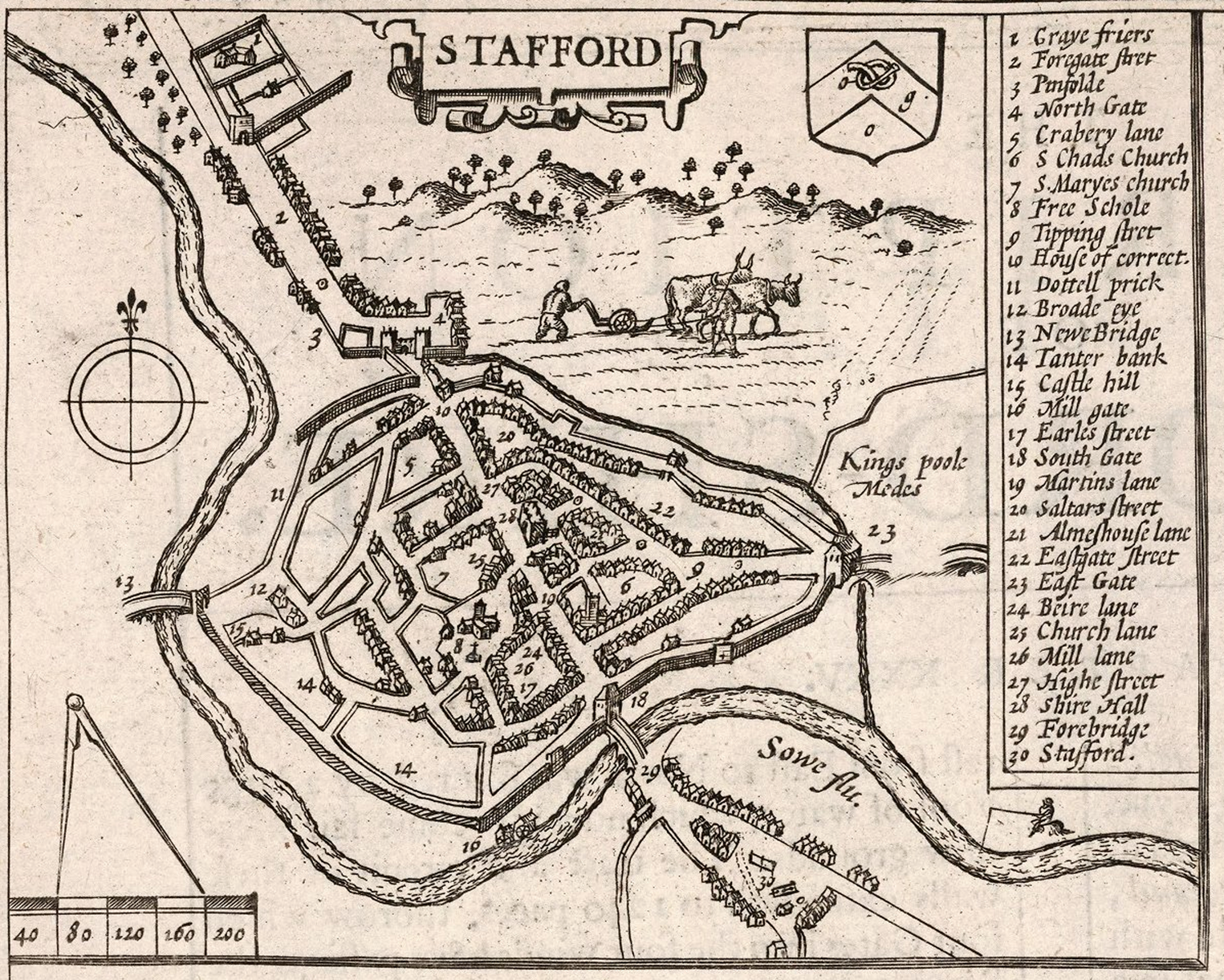
Figure 2. John Speed's town plan of Stafford, 1610 (published in 1676 by Bassett and Chiswell)
Image copyright © 2000 by Cartography Associates (https://www.davidrumsey.com/luna/servlet/detail/RUMSEY~8~1~285263~90057936:-Staffordshire---Stafford-Countie-#, accessed 31 January 2020). Licensed under Creative Commons Licence (https://creativecommons.org/licenses/by-nc-sa/3.0/, accessed 31 January 2020).
The Charred Plant Remains from Anglo-Saxon and Medieval Stafford
The archaeobotanical analysis presented here is based on forty-six soil samples excavated at Stafford whose archaeobotanical contents are dominated by charred cereal remains (Supplementary Material 1). These samples derive from three excavations in central Stafford: the St Mary's Grove and Bath Street sites, excavated as part of the campaign led by Carver, Cane and Cane between 1975 and 1985 (Carver, Reference Carver2010); and the Tipping Street South site which was excavated by Oxford Archaeology in 2009–2010 (Dodd et al., Reference Dodd, Goodwin, Griffiths, Norton, Poole and Teague2014). The quantitative work presented here is based on the original archaeobotanical data produced by Lisa Moffett (Reference Moffett1987) and Denise Druce (Reference Druce2014).
Selection and dating of samples for biochemical analysis
Of the forty-six samples included in this study, seventeen were selected for stable isotope analysis of charred cereal grains (Supplementary Material 1). These samples, all containing large quantities of well-preserved grains, were chosen to ensure that both Late Saxon and post-Conquest contexts were represented, and to allow the inclusion of grains from all four of the cereals found at Stafford, i.e. hulled barley, free-threshing wheat, oats and rye. Additionally, grains from each of the same seventeen samples were selected for radiocarbon dating. These new radiocarbon dates, in combination with those obtained during previous excavations, were used to construct a new chronological model (summary and further detail in Supplementary Material 2).
Six samples belong to Phase 1, a period of activity at Tipping Street South dated to between the late ninth and early tenth centuries, centred on three pottery kilns; substantial quantities of charred plant remains associated with the kilns and nearby pits suggest that cereals were also processed nearby (Dodd et al., Reference Dodd, Goodwin, Griffiths, Norton, Poole and Teague2014: 10–31, 73). While the chronometric study undertaken by Oxford Archaeology postulated that Late Saxon activity at Tipping Street began before ad 850 (Dodd et al., Reference Dodd, Goodwin, Griffiths, Norton, Poole and Teague2014: 92–93), this suggestion is not supported by the model produced here. The discrepancy is due partly to the inclusion of new radiocarbon dates, and partly to the application of an outlier model to allow for the possibility of an old wood effect in the dates derived from charcoal (Supplementary Material 2). Nonetheless, these adjustments do not contradict Oxford Archaeology's essential conclusion that significant activity, including pottery production and crop processing, took place at Stafford before the historically attested establishment of the burh in ad 913.
Fifteen samples belong to Phase 2, which encompasses the mid-tenth to mid-eleventh centuries, broadly spanning the early decades of the burh to the Norman Conquest. The most substantial deposits of charred cereal remains from this phase were excavated from a group of ovens at St Mary's Grove variously interpreted as crop-driers and baking ovens (Moffett, Reference Moffett and Rackham1994).
Twenty samples belong to Phase 3, which spans the mid-eleventh to early thirteenth centuries. Most derive from St Mary's Grove, chiefly characterized in this period by a sunken-featured structure containing rich deposits of charred cereal remains (Carver, Reference Carver2010: 103). Other rich samples derive from an extensive spread of charred material in the backfill of nearby large quarry pits (Moffett, Reference Moffett1987: 14).
Four samples belong to Phase 4, spanning the thirteenth to sixteenth centuries, when Stafford became established as a market town. These samples come from pits, ditches, and a kiln at St Mary's Grove, the site of a medieval residence (Carver, Reference Carver2010: 122). The one remaining sample studied here belongs to the post-medieval Phase 5 and derives from a pit near a putative brewery (Carver, Reference Carver2010: 35).
Patterns Among the Cereal Crops
The crop contents of all forty-six samples are dominated by four cereals: hulled barley (Hordeum vulgare L.), wheat (Triticum L. free-threshing type), oat (Avena L.), and rye (Secale cereale L.). The predominance of these cereals at Stafford in the Anglo-Saxon and medieval periods is consistent with national patterns (Moffett, Reference Moffett, Hamerow, Hinton and Crawford2011). The fact that not only grains but also charred chaff items from all four cereals occur in the assemblage implies that all were most probably grown locally. A broad picture of changes in crop choices over time can be obtained by calculating the relative proportions of grains in each sample belonging to each cereal genus (Figure 3; Supplementary Material 1).

Figure 3. Percentage of cereal grains in samples grouped by phase.
Hulled barley occurs in negligible proportions at Stafford before Sub-Phase 2.2. This suggests that barley only made a notable contribution to the local cereal economy from the latter part of the tenth century onwards (Figure 3). Most of the barley remains are likely to represent a six-row variety, but Moffett also identified two-row barley amongst the rachis segments (Reference Moffett1987: 5–6). Remains of two-row barley are found only from the latter part of the tenth century onwards, when proportions of barley increase more generally; together these trends suggest that barley cultivation in Stafford's hinterland became more productive and more diverse during that period (Moffett, Reference Moffett1987: 5–6).
The lowest proportions of free-threshing wheat grain are found in the samples dating from between the late tenth and mid-twelfth centuries (Sub-Phases 2.3 to 3.1). Rye exhibits a converse pattern, with notably high proportions of rye grain principally in the samples belonging to Sub-Phase 3.1 (Figure 3). The situation is reversed in Sub-Phase 3.2, when wheat grains tend to be present in higher proportions than rye grains.
It is during this same period (Sub-Phase 3.1), when wheat was apparently less important than rye, that some diversification appears amongst the charred wheat remains. Moffett's analysis reveals that two species of free-threshing wheat were present at Stafford from around the mid-eleventh century onwards: bread-type wheat (Triticum aestivum L.) and rivet-type wheat (Triticum turgidum L.). Whereas bread-type wheat appears to be ubiquitous in the Stafford assemblages, rivet wheat chaff has been identified in only six of the forty-six samples, all dating from Phases 3 and 4. Its relative scarcity at Stafford is consistent with the wider national picture for this period (Moffett, Reference Moffett, Woolgar, Serjeantson and Waldron2006: 49; Druce, Reference Druce2014: 72). In addition, Moffett has observed that some of the wheat grains dating from between the mid-eleventh and mid-twelfth centuries were of a distinctive but archaeobotanically nondescript ‘bullet-shaped’ form (Moffett, Reference Moffett1987: 5). It may be that these grains represent not a different species or subspecies of free-threshing wheat, but a wheat landrace: a variety specifically adapted to thrive in a particular local environment. More work is needed to establish whether this is indeed the case.
The relative proportions of oat grains suggest that oats were a significant crop from the late ninth century onwards (Figure 3). Moffett's analysis (Reference Moffett1987: 6) of the preserved chaff indicates that two domesticated species of oat were present: common oat (Avena sativa L.) and bristle oat (Avena strigosa Schreb.). Bristle oat has been identified only in samples dating from between the late tenth and mid-twelfth centuries, whereas common oat is present from the late ninth century onwards. Moffett notes that bristle oat is usually only grown in environments too harsh for other crops to thrive, and therefore suggests that its occurrence at Stafford—in a lowland region of relatively hospitable farmland—is most probably unintentional (Moffett, Reference Moffett and Rackham1994: 63).
In summary, the relative proportions of charred cereal grain in the archaeobotanical samples from Stafford suggest that, between the mid-tenth and mid-twelfth centuries, the cultivation regimes feeding the town underwent some diversification in crop choices, while rye increased in importance relative to wheat. Such patterns provide an essential framework within which to consider the pollen, weed ecological, and biomolecular evidence for key developments in crop husbandry strategies, notably land use, extensification, and crop rotation.
Landscape and Land Use
There are many pollen records from the Stafford region, but relatively few dated sequences covering the period from ad 450 to 1450. New, high resolution data from Stafford West and Cranberry Bed in South Yorkshire are presented here, together with data from the European Pollen Database (IMBE, 2007), British Pollen Database (BPOL, M. Grant, pers. comm. 2019) and published diagrams (Hicks, Reference Hicks1971; Tallis & Switsur, Reference Tallis and Switsur1973; Beales, Reference Beales1980; Brown, Reference Brown1988; Bartley & Morgan, Reference Bartley and Morgan1990). Alluvial and enclosed valley sites are expected to have small, localized catchments, while lakes and upland bogs should represent broader, regional trends (Figure 4; see Jacobson & Bradshaw, Reference Jacobson and Bradshaw1981). The published records referred to here are some of the most detailed for the West Midlands and Southern Pennines yet few have more than one sample per century for the early medieval period, making it difficult to establish exactly when changes in land use occurred. For FeedSax, we are sampling cores at higher resolution for pollen and radiocarbon dating, in order to understand changes over shorter timescales. ‘Best fit’ chronologies have been applied to allow comparison between sites, but, as the number of radiocarbon dates for each site and especially those falling within the medieval period is variable, the error margins within the age-depth models are equally variable. For example, four of the seven dates for Featherbed Moss (Derbyshire) are Anglo-Saxon to medieval, while the Hipper Sick (also in Derbyshire) chronology is extrapolated from a single Bronze Age date (Supplementary Material 3). Radiocarbon dates rejected as too early or late by the original researchers have been excluded from the models.

Figure 4. Pollen site locations. Map produced in ArcGIS Pro (ESRI) and ASTER GDEM (elevation).
Establishing the changing proportions of broad vegetation types over time allows us to identify large-scale changes in landcover, e.g. from woodland to open grassland. However, recognizing changes in land use within open landscapes, such as a shift from pastoral to arable farming, requires closer examination of the herbaceous taxa present. Turner's (Reference Turner1964) Arable/Pastoral (AP) index, while problematic in some ways, provides a rough indication of land use trends. AP indices are used here together with a thorough assessment of the diversity and relative proportions of pollen types associated with grazing and cultivation. Cereal pollen identification requires measurement, and designations beyond ‘Cereal type/Cerealia’ were not available for any of the published datasets. For Stafford West and Cranberry Bed (South Yorkshire), large grasses were categorized as: Hordeum type, which represents barley but also large wetland grasses, Avena-Triticum type (oats and wheats), or Secale cereale (rye) (after Andersen, Reference Andersen1979; Tweddle et al., Reference Tweddle, Edwards and Fieller2005). Methodologies for sample preparation and critical identifications are provided online, together with further information about the pollen sites (Supplementary Material 3).
Low-lying areas around Stafford appear to have been remarkably open throughout the Anglo-Saxon and medieval periods, and in most cases by the later Roman period (Figure 5); whereas, at the lowland lake site of Crose Mere in Shropshire, tree cover is higher, suggesting a more wooded landscape. Nevertheless, considering overrepresentation of tree pollen and site-catchment biases, the latter landscape was still relatively open (see Tauber, Reference Tauber1965). AP indices for the lowland sites are all strongly arable by the mid-Roman period, if not earlier: all three cereal types are present at Stafford West, and cereals, Cannabis/Humulus (hemp/hops), and a wide range of arable indicators are present at Crose Mere at this time (Figure 6 and Supplementary Material 3).

Figure 5. Cumulative pollen percentage diagrams for the Stafford region showing changes in proportions of key vegetation over time.

Figure 6. Relative proportions of arable and pastoral land use over time in the Stafford region. Inferred land use is based on the number and variety of indicator taxa present and AP (arable pastoral) indices (after Turner, Reference Turner1964).
Interestingly, by c. ad 550–650 there is a small increase in the range and quantity of arable indicators and/or cereals at most of the lowland sites (Figure 5). At Crose Mere in this period, a slight increase in pasture is suggested by the AP index, but this is accompanied by an increase in Cannabis/Humulus and the first occurrence of Centaurea cyanus (cornflower), a weed associated with Anglo-Saxon farming. Although there are error margins in the age-depth models, the fact that Stafford West, King's Pool, and Wilden Marsh (Worcestershire) show continuation or even expansion of arable farming (Figure 6) suggests it is a real pattern, in contrast to evidence for an arable decline, or a major shift towards pastoral farming, in eastern England at this time (e.g. Banham & Faith, Reference Banham and Faith2014). This adds weight to the conclusion that not all regions experienced a post-Roman decline (e.g. Rippon et al., Reference Rippon, Smart and Pears2015). It is worth noting that both Stafford sites and Wilden Marsh are likely to have had small catchments, and would strongly reflect local cultivation, even if there was a decline in the wider landscape. In contrast, Crose Mere, a lake site, should represent a larger catchment; there is a slight increase in pasture here, but no arable decline.
In the Mid-Saxon period (c. ad 650–850), a dramatic increase in the variety and percentages of cereals and arable indicators occurs in Stafford, with Cannabis/Humulus present at both Stafford West and King's Pool by the mid to late eighth century. These developments are consistent with an increase in the scale of arable farming, together with a change in methods of cultivation and the crops being grown. Significantly, this activity predates Phase 1 at Stafford and the foundation of the burh. None of the Wilden Marsh (a floodplain site in Worcestershire) samples date to this period, but by c. ad 900 a range of arable indicators, including Centaurea cyanus, is present. All the lowland sites reflect broadly arable landscapes throughout the Late Saxon period.
At Crose Mere in Shropshire, arable farming remains dominant until the late eleventh century, when pastoral land use increases; this may be linked to the construction of a nearby Norman motte of Stockett at Cockshutt (Pastscape, 2019: Monument 68643). By c. ad 1200, there is also a decline in arable farming around Wilden Marsh, although the wide spacing of samples makes it difficult to establish when this happens (Figures 5 and 6). Cultivation remains important at Stafford West and King's Pool until the thirteenth to fourteenth centuries, when arable indicators decline and the AP index for the latter becomes more pastoral (Figure 6). These changes might result from agricultural land shifting further away from the sampling sites as the town increased in size.
The upland sites reflect much more varied landcover and a greater emphasis on pastoral land use, as might be expected given the topography and prevalence of peat/acid soils. The upland valley bog of Cranberry Bed (South Yorkshire) shows a relatively open landscape with Hordeum type, Secale cereale and a wide range of arable indicators present in the early Roman period, giving way to heathland expansion by c. ad 250, perhaps owing to farming-induced soil deterioration and cooler/wetter conditions (see Hicks, Reference Hicks1971: 666). There is little or no evidence of farming at Cranberry Bed or Featherbed Moss (Derbyshire) for the fifth to ninth centuries (Figure 6), but at Hipper Sick (also in Derbyshire) there is an increase in pasture and a slight rise in arable indicators in the seventh to eighth centuries. Hipper Sick is on Beeley Moor, which, alongside copious Bronze Age remains, has vestiges of medieval occupation (Pastscape, 2019; e.g. Monuments 31104, 311113, 311119, 1342500, 311053). It is probable that the nearby lowlands were farmed and that the changes in the pollen record reflect the same Mid-Saxon changes seen around Stafford, though with more emphasis on pasture.
The ninth to tenth centuries see a marked decline in tree cover at Cranberry Bed and Featherbed Moss, followed by an increase in arable and pastoral indicators. All three cereal types and Cannabis/Humulus are present at Cranberry Bed. This indicates a Late Saxon woodland clearance, and an expansion and change in methods of cultivation similar to that seen in the Mid-Saxon period elsewhere. The spread of arable farming into, or near to, upland areas suggests larger scale cultivation, while the marked increase in pastoral land use could be the result of rough grazing of livestock on heathland.
A short-lived twelfth-century birch regeneration at Cranberry Bed ends with a substantial clearance event in c. ad 1160, with evidence for ongoing arable and pastoral farming. Grazing and cultivation continue into the central Middle Ages despite a fourteenth-century expansion of heathland (Figures 5 and 6). A post-medieval farmstead and pasture existed nearby (Bevan, Reference Bevan2003: 280), but the pollen data show a much longer record of local farming. The landscape remains broadly pastoral in later periods at Hipper Sick and Featherbed Moss, though evidence for cultivation persists (Figure 6).
Extensification
A functional ecological study of weed flora developed under traditional agricultural regimes in Asturias in Spain and Haute Provence in France successfully differentiated between high- and low-input farming methods (Bogaard et al., Reference Bogaard, Hodgson, Nitsch, Jones, Styring and Diffey2016). The aim of the study was to use discriminant analysis to develop a model for differentiating fields managed with high inputs per unit area (intensive manuring and weeding) from those, like medieval open fields, receiving low inputs (low/no manuring and weeding). This was achieved on the basis of five functional traits that predict the response of weed species to soil fertility and/or disturbance due to tillage and weeding: specific leaf area (leaf area/leaf dry weight), canopy height and diameter, the ratio of leaf area per node to fresh leaf thickness, and flowering duration (Figure 7a). This model is suitable for archaeobotanical studies since it is based on the presence or absence of weed species in different farming regimes, rather than their ubiquity within individual fields.

Figure 7. a) The relationship of fields in Haute Provence (open circles) and fields in Asturias (filled circles) to the discriminant function extracted to distinguish these two groups (larger symbols indicate group centroids). b–e) The relationship of archaeobotanical samples from Stafford to the discriminant function (larger symbols indicate centroids for the modern groups). f) Correlations between the functional attribute scores used as discriminating variables and the discriminant function.
Samples from Stafford containing at least ten seeds of weed taxa identified to species level were entered into the classification phase of the discriminant analysis as unknown cases, in order to assess their similarity to the modern high- versus low-input regimes. The results (Figure 7) show that late ninth- to early tenth-century samples (Phase 1) exhibit variable scores on the discriminant function, albeit mostly within the low-input spectrum. From the mid-tenth century onwards, however, there is a clear tendency for the Stafford weed data to conform to ‘low-input’ growing conditions, resembling present-day extensive farming of cereals in Haute Provence. It should be acknowledged that within each phase there is some variation, as observed among present-day fields (Figure 7a), but the results clearly point to extensification. This indicates that, from the mid-tenth century onwards, the great majority of cereals appear to have been grown with little or no manuring and hand-weeding. This evidence, combined with palynological evidence for largely open, arable landscapes around Stafford in this period (see above), implies that cereal cultivation featured low-input management within an extensive, large-scale system in which land (rather than labour inputs per unit area) was the limiting factor of production (see Bogaard et al., Reference Bogaard, Fochesato and Bowlesin press).
A study of the present-day arable and hay meadow flora at Laxton, Nottinghamshire, known for its extant open field landscape, offers a complementary perspective focused on levels of mechanical soil disturbance and what this might reveal about ploughing regimes (Orwin & Orwin, Reference Orwin and Orwin1938). At Laxton we conducted a botanical survey of twenty sites, ranging from unploughed but periodically grazed and annually cut ‘sykes’ (hay meadow areas, unsprayed by herbicides, between or on the edges of the open fields) to weed flora in arable fields, either along cereal field edges without herbicide spraying or in fallow fields (i.e. in the third, fallow year of the rotation scheme) that had not recently been sprayed. Figure 8a shows the results of a discriminant analysis that separates (with 100 per cent success) the grassland flora of the ‘sykes’ from the weed flora of the arable fields, using two attributes relating to soil disturbance as discriminating variables: flowering duration and (for perennials only) vegetative propagation (i.e. the ability to regenerate from fragments of root/stolon/rhizome). Figure 8b–e shows, in comparison, the discriminant scores of the Stafford samples, by phase. There is an increasing tendency through time for the Stafford weed data to conform to the more disturbed conditions of arable fields; none of the samples dating to the twelfth century or later resembles the poorly disturbed grassland of the ‘sykes’. This contrasts with earlier phases where samples resembling those from the sykes could represent the intermittent cultivation of land normally used for pasture (see Hooke, Reference Hooke1981: 207) or the interface of arable and grassland. The implication is that, within the extensification process, arable fields at Stafford were more consistently and comprehensively disturbed from the twelfth century onwards than in earlier periods. This observation is consistent with a more pervasive use of the mouldboard plough in this final phase, when it appears that effectively all cereal farming took place in heavily disturbed conditions.

Figure 8. a) The relationship of Laxton arable fields (grey and black squares) and hay meadow ‘sykes’ (open squares) to the discriminant function extracted to distinguish these two groups (larger symbols indicate group centroids). b–e) The relationship of archaeobotanical samples from Stafford to the discriminant function (larger symbols indicate centroids for the modern groups). f) Correlations between the functional attribute scores used as discriminating variables and the discriminant function.
Crop Rotation
A functional ecological study of field surveys in modern Germany successfully differentiated the weed flora of autumn- and spring-sown crops, using flowering onset and duration of arable weeds as a functional trait in discriminant analyses (Bogaard et al., Reference Bogaard, Jones, Charles and Hodgson2001). A recent study of Anglo-Saxon charred plant remains found that the application of correspondence analysis to quantified weed seed data, using the same functional attributes as the German study, was effective at detecting subtle patterns in sowing seasonality (McKerracher, Reference McKerracher2019: 96–124). The same methods and criteria detailed in that study have been applied to the Stafford dataset. Correspondence analysis was performed using Canoco for Windows 4.51 and CanoDraw for Windows 4.1 programs (after Braak & Smilauer, Reference ter Braak and Smilauer2002).
Two complementary trends emerge from this analysis (Figure 9). On the x-axis, weed species associated with spring-sowing tend to occur at the positive end, along with barley grain and rachis. By contrast, weed species associated with autumn-sowing tend towards the negative end of the x-axis, as do wheat and, especially, rye; oat lies roughly between the two groups. The patterns detected are, thus, consistent with the idea, familiar from later medieval history, that the wheat and rye consumed at Stafford were typically autumn-sown crops, whereas barley tended to be sown in the spring (Banham & Faith, Reference Banham and Faith2014: 58). For Stafford, this is most clearly the case between the mid-tenth and early thirteenth centuries; the evidence for earlier and later phases is less compelling because of the relatively small number of samples. However, these results cannot exclude the possibility that the cereals were simply sown at different times in different fields, rather than grown in rotation in the same fields. To establish with greater certainty whether rotation was practised, the evidence of crop stable isotopes was considered.

Figure 9. Distribution of cereal items and weed seeds in correspondence analysis output, coded by cereal type and seasonality association.
Ratios of stable carbon isotopes in cereals are influenced by soil moisture during growth (Wallace et al., Reference Wallace, Jones, Charles, Fraser, Halstead, Heaton and Bogaard2013): more positive δ13C values generally reflect drier conditions, and negative values wetter conditions. Other environmental factors affecting crop δ13C values include slope, canopy cover, light intensity, and temperature (Heaton, Reference Heaton1999; Bogaard et al., Reference Bogaard, Hodgson, Nitsch, Jones, Styring and Diffey2016). Beyond environmental factors, interpretation of crop δ13C values must also take account of physiological differences among species. The δ13C values of mixed cereals grown under the same conditions show that oat is statistically significantly lower than rye, while rye appears to be comparable to free-threshing wheat (see Supplementary Materials 4 and 5 for details). Other research shows that barley tends to be ~1–2‰ lower than wheat due to physiological differences (Wallace et al., Reference Wallace, Jones, Charles, Fraser, Halstead, Heaton and Bogaard2013; Styring et al., Reference Styring, Charles, Fantone, Hald, McMahon and Meadow2017). If crops were grown systematically in rotation, it is expected that their δ13C values would reflect similar growing conditions, and hence that the expected physiological offsets between crops would be expressed.
The δ13C results from Stafford reflect the overall physiological differences expected between the four crops—rye, oat, barley, and free-threshing wheat—suggesting that they grew in similar conditions, i.e. in a similar landscape with a similar amount of rainfall (Figure 10a). The δ13C values of each species remain relatively stable over time, with the exception of free-threshing wheat (see below). There is no significant difference between oat and barley in any of the periods, and oat and barley are always ~1‰ or more offset from rye.

Figure 10. a) Cereal grain δ13C values shown by the whole assemblage and b) by phase; c) cereal grain δ15N values shown by the whole assemblage and d) by phase.
There is, however, a significant difference between wheat and rye during Phase 3 (broadly, the mid-eleventh to early thirteenth centuries), a departure from the pattern of similar values for the earlier periods (Table 1; Figure 10b). In Phase 2, there is no statistical difference between wheat and rye; whereas, in Phase 3, wheat becomes less enriched in 13C, and is statistically significantly different from rye, although it should be noted that there are only four wheat samples from Phase 3. Several causes for this change are possible. The samples analysed may represent wetter years, or wetter soils; the low number of samples analysed could also have produced a misleading picture. Alternatively, the isotopic difference could represent a change in the type of wheat cultivated; as mentioned above, the archaeobotanical remains from Phase 3 include the chaff of rivet wheat and ‘bullet-shaped’ grains of an otherwise unidentified variety, possibly a local landrace. It is therefore possible that the change in wheat isotopic values between Phases 2 and 3 is the consequence of a switch to a variety with lower δ13C, either for physiological reasons or because it was cultivated in wetter soils. It is of course also possible that wheat was no longer grown in rotation with the other species in Phase 3, although this seems unlikely given that the δ15N values for wheat remain consistent with rotation throughout all periods (see below).
Table 1. Results of the statistical tests conducted on stable isotope data from Stafford. Significant Tukey post hoc P-values shown in bold.

* T-test conduct instead of ANOVA
Ratios of the stable nitrogen isotopes in cereals reflect soil N composition. Multiple factors can affect this ratio, including environmental conditions, such as de-nitrification in waterlogged or periodically wet conditions, as well as anthropogenic causes, notably manuring (Handley et al., Reference Handley, Austin, Robinson, Scrimgeour, Raven and Heaton1999; Bogaard et al., Reference Bogaard, Heaton, Poulton and Merbach2007; Fraser et al., Reference Fraser, Bogaard, Heaton, Charles, Jones and Christensen2011). Overall mean δ15N values indicate similar soil 15N for oat, rye, and wheat (Figure 10c), while barley is consistently elevated in 15N compared to the other crops in Phases 2 and 3 (no barley samples were available for Phase 1) (Figure 10d). However, there is no statistically significant difference between the species’ δ15N values within Phases 2 and 3, potentially because of the uneven distribution of samples between species (Table 1). If Sub-Phase 3.1, with the highest number of samples per species, is examined, barley is statistically significantly different from the other three crop species (Table 1). Though the limited number of samples per species per phase must be borne in mind, it appears that barley was cultivated in soil more enriched in 15N than soil where the other three crops were cultivated, and therefore was not grown in rotation with them. The limited differences between rye, oat, and free-threshing wheat, on the other hand, suggest that these were grown in rotation.
A general trend of increasing 15N enrichment over time can be seen (Figure 11). Rye δ15N values change significantly between Phase 1 (5.3‰) and Phase 3 (8‰), while barley shows a significant difference between Phase 2 (8.7‰) and Phase 3 (10.6‰) (Table 1). There is no statistical difference among the means of oat or wheat from any phase but the mean values of oat and wheat do increase over time (for oat, Phase 1: 5.4‰, Phase 3: 8.0‰; for wheat, Phase 1: 5.9‰, Phase 3: 7.1‰). When all sample values are averaged irrespective of species, an increase in δ15N values and ranges for the three phases is evident with Phases 1 and 2 statistically different from Phase 3 (Table 1). Phases 1 and 2 remain statistically significantly different from Phase 3 even when barley is removed.

Figure 11. Cereal grain δ15N results from Stafford shown by phase, regardless of species.
The increasing enrichment of 15N over time could be due to several factors. The lack of wild fauna to create a ‘herbivore baseline’ (e.g. Styring et al., Reference Styring, Ater, Hmimsa, Fraser, Miller and Neef2016) means that understanding whether this enrichment is due to arable practices (e.g. manuring) or to wider environmental factors relies on other strands of evidence, particularly functional weed ecology. The weed results, as discussed above, indicate that fertility did not increase over time, which means that the increasing 15N enrichment is unlikely to be a consequence of manuring. Other possibilities include seasonal wetting and drying and/or waterlogging of heavier, poorly draining clays and seasonally wet soils surrounding Stafford (Figure 1). This is not, however, supported by the δ13C values which, apart from wheat, show only limited change during the later phases. It is possible that changes due to the Medieval Climate Anomaly enabled farmers, by the middle of the eleventh century, to cultivate previously saturated soils, as lower rainfall and/or higher temperatures dried out previously saturated land (Xoplaki et al., Reference Xoplaki, Fleitmann and Diaz2011). It is also likely that use of the mouldboard plough to create ridge-and-furrow improved drainage in wetter soils.
Conclusion
By bringing together the results of the various analyses presented above, it has been possible to draw certain conclusions regarding the farming regimes that fed the population of Stafford from the late ninth to thirteenth centuries.
The strongly arable signal in the pollen data from Stafford and the immediately surrounding region points to an emphasis on cereal farming beginning well before the foundation of the burh in ad 913. In the Mid-Saxon period, a broader range of crops and associated weeds appears, indicating an increase in the scale of arable farming and possibly changes in farming practices. This trend mirrors changes evident more widely across England, not only in the archaeobotanical record but also in the structure and character of Mid-Saxon settlements, with growing evidence of enclosures such as paddocks and pens as well as droveways pointing to new ways of managing the movement of livestock, while the appearance of barns, corn driers, and watermills suggests that cereal surpluses were being mobilized in new ways (see Hamerow, Reference Hamerow2012; McKerracher, Reference McKerracher2018). The arable signal remains dominant in the pollen record until the thirteenth century; the decline at this time could reflect the growth of Stafford pushing agricultural activity further away from the town.
An expansion or shift onto heavier, less well-drained soils is suggested by the increasing enrichment of soil N values from around the mid-eleventh century. This enrichment was not the result of increased manuring, as the weed data clearly show. Such a shift or expansion is supported by the distribution of ridge-and-furrow earthworks in the vicinity of Stafford. The carbon (δ13C) values also indicate that, from the mid-eleventh century onwards, a different variety of free-threshing wheat, possibly rivet wheat, and potentially a new landrace of wheat, may have been consumed in Stafford.
A shift to extensive, low-input farming regimes between the mid-tenth and mid-eleventh centuries is clearly indicated by a functional ecological analysis of the weed flora. The wide spectrum of regimes apparent during the later ninth to early tenth century, i.e. from relatively intensive to highly extensive, was replaced in this later period by a greater uniformity of practice and the widespread adoption of highly extensive regimes, presumably representing some form of open field farming. Comparison with modern weed data from Laxton in Nottinghamshire, furthermore, suggests a growing tendency over time for the Stafford crops to be grown in highly disturbed conditions, consistent with increasing use of the heavy plough. The ninth and tenth centuries also see woodland clearance at upland sites, together with an increase in arable indicators and cereal types, supporting the conclusion that a larger proportion of the landscape came under cultivation at this time. A corresponding increase in pastoral indicators suggests growing use of less cultivable heathland areas for grazing.
Crop rotation is indicated from at least the mid-tenth century onwards, by the weed flora indicating the presence of autumn- and spring-sown cereals, and by comparable δ13C and δ15N values for different cereals. The δ15N values for barley, however, were different across all phases, implying that this cereal was grown in different conditions. The fact that barley was also underrepresented in the archaeobotanical record supports the conclusion that the barley consumed in Stafford was not grown in rotation with the other cereals.
It should be emphasized that no single analytical method on its own would have made it possible to draw such a detailed picture. Only by comparing the results from crop stable isotope analysis, pollen analysis, functional weed ecology, and quantitative archaeobotanical analysis, has such a holistic and dynamic picture of farming practices during this critically important period emerged. The case study of Stafford thus illustrates the potential of integrated approaches to resolve long-standing debates about the spread of low-input cultivation, specifically open field farming, in medieval Europe.
Supplementary Material
To view supplementary material for this article, please visit https://doi.org/10.1017/eaa.2020.6.
Acknowledgements
The FeedSax project is supported by the European Research Council (ERC) under the European Union's Horizon 2020 research and innovation programme under grant agreement no. 741751. The authors would like to thank the following for their advice and assistance, notably in providing access to and permission to analyse archaeobotanical material from Stafford: Lisa Moffett (Historic England), Peter Ditchfield (School of Archaeology, University of Oxford), Mike Kimber (Headland Archaeology), Scott Timpany (University of the Highlands and Islands), Michael Grant (University of Southampton), Alison Nicholls (Gladstone Pottery Museum, Stoke-on-Trent), and Joseph Perry (Potteries Museum, Stoke-on-Trent).