Introduction
Gradually decreasing amphibian diversity is generally viewed as an early-warning signal of problems in the environment (Niemi and McDonald, Reference Niemi and McDonald2004). Nonetheless, the decrease is often likely due to a combination of a variety of factors, of which one of the most notable is the effect of parasitic organisms (Blaustein and Wake, Reference Blaustein and Wake1995; Semlitsch, Reference Semlitsch2003). Amphibians inhabit a multitude of habitats and exhibit a striking diversity of life history patterns, reproductive modes, body sizes and trophic relations (Wells, Reference Wells2007). Due to their physiological dependence on the aquatic environment, they represent optimal hosts for parasites, since the development and transmission of the infective stages of many parasites takes place in the same aquatic environment. This places amphibians into the role of an interesting model for studying parasite diversity, especially in regions considered as the world's biodiversity hotspots. Nonetheless, they are still one of the least explored groups in this regard (Aho, Reference Aho, Esch, Bush and Aho1990; Barton, Reference Barton1999; Poulin and Morand, Reference Poulin and Morand2000; Carlson et al., Reference Carlson, Dallas, Alexander, Phelan and Phillips2020).
Due to their wide occurrence and unique reproduction mode, some of the best-studied amphibians in Europe are water frogs of the genus Pelophylax. Currently, at least 14 species and 3 asexual hybrid forms live in the western Palearctic (Plötner, Reference Plötner2005; Frost, Reference Frost2021). The distribution of water frog species is unequal and putatively intimately linked with both historical, geological and climatic processes and geographic heterogeneity in the region (Beerli et al., Reference Beerli, Hotz and Uzzell1996; Lymberakis et al., Reference Lymberakis, Poulokakis, Manthalou, Tsigenopoulos, Magoulas and Mylonas2007; Akın et al., Reference Akın, Bilgin, Beerli, Westaway, Ohst, Litvinchuk, Uzzell, Bilgin, Hotz, Guex and Plötner2010; Plötner et al., Reference Plötner, Uzzell, Beerli, Akın, Bilgin, Haefeli, Ohst, Köhler, Schreiber, Guex, Litvinchuk, Westaway, Reyer, Hotz and Glaubrecht2010). Remarkable species diversity and high endemism are observed in the peri-Mediterranean area, especially in the Balkans and the Middle East (Speybroeck et al., Reference Speybroeck, Beukema, Bok and Voort2016; Dufresnes et al., Reference Dufresnes, Denoël, Di Santo and Dubey2017). To the north, species diversity is declining, with only 2 species living in central and northern Europe – specifically, P. ridibundus (Pallas, 1771) and P. lessonae (Camerano, 1882) – whose extensive hybridization gave rise to an asexual hybrid form, P. esculentus (Linnaeus, 1758) (Graf and Polls Pelaz, Reference Graf, Polls Pelaz, Dawley and Bogart1989; Plötner, Reference Plötner2005). According to molecular data, basal radiation among the major European Pelophylax lineages took place in the middle Miocene, with subsequent species diversification during the upper Miocene and the Pliocene (Beerli et al., Reference Beerli, Hotz and Uzzell1996; Lymberakis et al., Reference Lymberakis, Poulokakis, Manthalou, Tsigenopoulos, Magoulas and Mylonas2007; Akın et al., Reference Akın, Bilgin, Beerli, Westaway, Ohst, Litvinchuk, Uzzell, Bilgin, Hotz, Guex and Plötner2010). The distribution and current intraspecific diversity of water frogs were also influenced by Pleistocene climatic oscillations, which led to the retraction of populations to the climatically favourable southern refugia during the glacials, and later expansion to the north in interglacials (Snell et al., Reference Snell, Tetteh and Evans2005; Hoffmann et al., Reference Hoffmann, Plötner, Pruvost, Christiansen, Röthlisberger, Choleva, Mikulíček, Cogalniceanu, Sas-Kovacs, Shabanov, Morozov-Leonov and Reyer2015).
Although the current distribution, phylogeny and genetic diversity of Pelophylax species are relatively well known, this is no longer the case with their parasites. Water frogs are important hosts for many parasitic groups, from protists to arthropods (e.g., Günther, Reference Günther1990; Herczeg et al., Reference Herczeg, Vörös, Végvári, Kuzmin and Brooks2016; Chikhlyaev et al., Reference Chikhlyaev, Ruchin and Fayzulin2018; Iacob, Reference Iacob2021). One of the common parasites of amphibians are paramphistomoid trematodes of the families Diplodiscidae Cohn, Reference Cohn1904 and Cladorchiidae Fischoeder, 1901, which have been reported from frogs all around the world (e.g., Skrjabin, Reference Skrjabin1949; Yamaguti, Reference Yamaguti1971; Sey, Reference Sey1991; Vojtková and Roca, Reference Vojtková and Roca1994; Jones, Reference Jones, Jones, Bray and Gibson2005). Since the original descriptions of the families, taxonomic revisions have included several reorganizations of genera and formerly described subfamilies according to their morphological traits and/or geographical distribution (e.g., Cohn, Reference Cohn1904; Skrjabin, Reference Skrjabin1949; Odening, Reference Odening1959; Yamaguti, Reference Yamaguti1971; Grabda-Kazubska, Reference Grabda-Kazubska1980; Sey, Reference Sey1983). Diplodiscidae and Cladorchiidae share several morphological characters (e.g., the absence of an oral sucker; a sucker-like attachment organ at the posterior end; paired pharyngeal sacs; and an absent pharyngeal bulb) and can be differentiated primarily by the number of testes – representatives of Diplodiscidae have 1, while Cladorchiidae have 2 (Jones, Reference Jones, Jones, Bray and Gibson2005; Sey, Reference Sey2005). Moreover, these 2 families exhibit highly different host ranges; while Diplodiscidae include only a few genera, predominantly parasitizing in amphibians and reptiles, numerous highly diversified Cladorchiidae genera can be found in the guts of various marine and freshwater fishes, amphibians, reptiles and mammals (Jones, Reference Jones, Jones, Bray and Gibson2005).
So far, only 1 Diplodiscidae species is reported from European water frogs. Diplodiscus subclavatus (Pallas, 1760) is a common species which parasitizes the rectum of amphibians all across Europe, with a distribution range from the Iberian Peninsula in the west up to the Ukraine and Turkey in the East (e.g., Bailenger and Chanseau, Reference Bailenger and Chanseau1954; Gomez, Reference Gomez1987; Vojtková and Roca, Reference Vojtková and Roca1994; Navarro and Lluch, Reference Navarro and Lluch2006; Bjelić-Čabrilo et al., Reference Bjelić-Čabrilo, Popović and Paunović2009; Popiołek et al., Reference Popiołek, Rozenblut-Kościsty, Kot, Nosal and Ogielska2011; Düşen and Öz, Reference Düşen and Öz2013; Karakaş, Reference Karakaş2015; Herczeg et al., Reference Herczeg, Vörös, Végvári, Kuzmin and Brooks2016; Kuzmin et al., Reference Kuzmin, Dmytrieva, Marushchak, Morozov-Leonov, Oskyrko and Nekrasova2020; Čeirāns et al., Reference Čeirāns, Gravele, Gavarane, Pupins, Mezaraupe, Rubenina, Kvach, Skute, Oskyrko, Nekrasova, Marushchak and Kirjushina2021). The family Cladorchiidae is represented by 2 species parasitizing water frogs in Europe – Opisthodiscus diplodiscoides Cohn, Reference Cohn1904 and O. nigrivasis Mehély, Reference Mehély1929. Species of this genus are less prevalent and are reported from France (Dollfus, Reference Dollfus1951; Bailenger and Chanseau, Reference Bailenger and Chanseau1954; Combes et al., Reference Combes, Leger and Vidal1973), Germany (Andreas, Reference Andreas2007), Slovakia (Kopřiva, Reference Kopřiva1957; Vojtková, Reference Vojtková1974), Poland (Grabda-Kazubska, Reference Grabda-Kazubska1980), Spain (Combes and Gerbaux, Reference Combes and Gerbaux1970; Combes and Sarrouy, Reference Combes and Sarrouy1971; Martínez-Fernández et al., Reference Martínez-Fernández, Simón-Vicente and Cordero del Campillo1988) and Hungary (Mehély, Reference Mehély1929; Sey, Reference Sey1991). The 2 Opisthodiscus species have often been considered a single taxon, since their morphological differentiation is not fully clear (Odening, Reference Odening1959), and, therefore, their hosts and expected distribution have to be viewed with caution.
The distribution and diversity of paramphistome parasites of frogs were investigated to some degree in the past; however, little is known about their genetic diversity in this group of hosts. Publicly available sequence data on diplodiscid parasites of frogs are limited and include only several partial sequences of genes coding ribosomal subunits for 2 Diplodiscus species (D. mehrai Pande, 1937 and D. japonicus (Yamaguti, 1936) from Besprozvannykh et al., Reference Besprozvannykh, Rozhkovan, Ermolenko and Izrailskaya2018) and 1 species of Catadiscus (C. marinholutzi Freitas & Lent, 1939 from Queiroz et al., Reference Queiroz, Alves, López- Hernández, Anjos and Pinto2021). To date, there is no molecular data for any species of Opisthodiscus, which could be used to assess phylogenetic relationships based on DNA sequences. Although numerous partial 18S and 28S rRNA sequences from Diplodiscus specimens, with unambiguous species designation, and from D. amphichrus (Tubangi, 1933) originating from Indian Euphlyctis cyanophlyctis (Schneider, 1799) are available, they have not been used in any previous publication and are, therefore, considered unreliable. In addition, only Besprozvannykh et al. (Reference Besprozvannykh, Rozhkovan, Ermolenko and Izrailskaya2018) and Queiroz et al. (Reference Queiroz, Alves, López- Hernández, Anjos and Pinto2021) investigated more thoroughly the phylogenetic relationships among diplodiscids using available genetic markers; however, neither study fully tackled the intraspecific variability in the respective species. Regarding mitochondrial DNA (mtDNA), the complete mitogenome of D. nigromaculati Wang, 1977 from the east Asian water frog species P. nigromaculatus (Hallowell, 1861) was recently added to public databases; however, these data have not yet been utilized in any published work.
The present study therefore aimed to reveal the distribution, prevalence and abundance of paramphistomes in European water frogs in 2 geographically distant regions (central Europe and the south-west Balkans), and to assess the intraspecific genetic variability among collected paramphistomes using highly variable genetic markers, i.e., mtDNA genes – a novel method in the study of Diplodiscidae. On the basis of knowledge about amphibian hosts, we can hypothesize that (1) endemic species will be parasitized by endemic paramphistome taxa with a limited distribution range, and (2) the genetic diversity of recent parasite lineages and the relationships among them will be intimately linked with the evolutionary history and historical dispersion patterns proposed for European water frogs. Furthermore, we also assume that (3) Pelophylax hybrid forms might share parasite lineages associated with parental species in the region of sympatric occurrence (e.g., in the Slovakia – P. esculentus × P. ridibundus).
Material and methods
Material collection, parasite fixation and identification
A total of 161 Pelophylax water frogs from 13 localities in the Balkans and Slovakia were examined for the presence of parasites (Fig. 1, Table 1) Trematodes were collected from 4 host species: P. esculentus (n = 50) and P. ridibundus (n = 72) in Slovakia, and P. epeiroticus (Schneider, Sofianidou & Kyriakopolou-Sklavounou, 1984) (n = 30) and P. kurtmuelleri (Gayda, 1940) (n = 9) in Greece and Albania, respectively. Although the latter 2 species have been considered as endemics of the Balkan Peninsula since description, haplotypes and alleles of P. kurtmuelleri have been recorded in several European countries in the last few decades (e.g., Dufresnes et al., Reference Dufresnes, Denoël, Di Santo and Dubey2017, Reference Dufresnes, Lauenberger, Amrhein, Bühler, Thiébaud, Bohnenstengel and Dubey2018; Herczeg et al., Reference Herczeg, Vörös, Christiansen, Benovics and Mikulíček2017; Kolenda et al., Reference Kolenda, Pietras-Lebioda, Hofman, Ogielska and Pabijan2017; Bellati et al., Reference Bellati, Bassu, Nulchis and Corti2019 and literature therein; Litvinchuk et al., Reference Litvinchuk, Ivanov, Lukonina and Ermakov2020). Water frog species were identified according to morphological traits (Günther, Reference Günther1990; Plötner, Reference Plötner2005) and molecular markers – specifically, sequences of the mitochondrial ND2 fragment and microsatellites. Details of ND2 and microsatellite laboratory analyses and the genetic identification of water frogs have been studied by Plötner et al. (Reference Plötner, Uzzell, Beerli, Spolsky, Ohst, Litvinchuk, Guex, Reyer, Pruvost and Hotz2008), Hoffmann et al. (Reference Hoffmann, Plötner, Pruvost, Christiansen, Röthlisberger, Choleva, Mikulíček, Cogalniceanu, Sas-Kovacs, Shabanov, Morozov-Leonov and Reyer2015) and Papežík et al. (Reference Papežík, Kubala, Jablonski, Doležálková-Kaštánková, Choleva, Benovics and Mikulíček2021).

Fig. 1. Map showing the collection sites in Slovakia and the Balkans. A, the collection sites in the Slovakia; B, the collection sites in the Balkans; C, map of the Europe. The same scale bar is applicable for parts A and B. The same colouration palette of the collection site points is used for the sites in the subsequent figures.
Table 1. Quantitative descriptors of the populations of collected paramphistomes calculated for each metapopulation
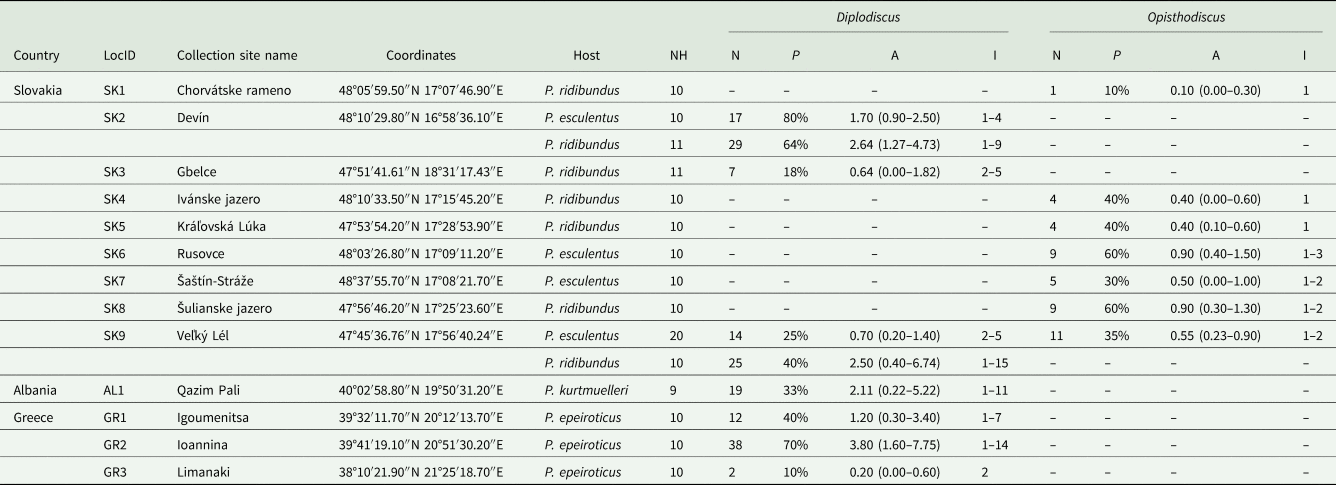
NH, number of processed host individuals; N, number of collected parasite individuals; P, prevalence; A, mean abundance (a 95% confidence interval); I, range of intensity of infection; absence of data is marked by dashes (–).
A total of 206 paramphistomoid trematodes were collected from frog rectums and urinary bladders. First, all collected specimens were doused in heated physiological solution to ensure their flexibility. Then, preliminary morphological identification was carried out using an Olympus SZX7 stereomicroscope (Olympus, Tokyo, Japan). The specimens of each preliminarily identified parasite taxon were divided into 2 groups: 1–3 whole specimens were preserved in 96% ethanol for later DNA extraction, while the rest were used for morphological analyses. Prior to mounting in Canada balsam, these latter specimens were stained with iron-acetocarmine, following the protocol of Georgiev et al. (Reference Georgiev, Biserkov and Genov1986). Determination followed Jones (Reference Jones, Jones, Bray and Gibson2005) and Vojtková (Reference Vojtková1974). Basic quantitative descriptors of the parasite populations, i.e., prevalence, mean abundance and minimum and maximum intensities of infection, were calculated for each parasite species according to Bush et al. (Reference Bush, Lafferty, Lotzs and Shostakll1997). Prevalence, as the percentage of frogs infected by a given parasite species, and mean abundance, as the mean number of parasite specimens per individual host taking into account both infected and uninfected hosts, were calculated. Following the suggestion of Rózsa et al. (Reference Rózsa, Reiczigel and Majoros2000) for interpreting quantitative data, a 95% confidence interval was calculated for mean abundance. Due to the relatively low host sample size per population, the bias-corrected and accelerated bootstrap (BCa) method QPweb (Reiczigel et al., Reference Reiczigel, Marozzi, Fábián and Rózsa2019) was used to calculate a confidence interval for mean abundances.
DNA extraction, amplification and sequencing
Genomic DNA was extracted following the protocol described by Georgieva et al. (Reference Georgieva, Soldánová, Pérez-del-Olmo, Dangel, Sitko, Sures and Kostadinova2013). The partial region of 28S rRNA (hereinafter abbreviated as 28S) was amplified using the primers digl2 (5-AAG CAT ATC ACT AAG CGG-3) (Tkach et al., Reference Tkach, Pawlowski, Mariaux, Swiderski, Littlewood and Bray2001) and 1500R (5-GCT ATC CTG AGG GAA ACT TCG-3) (Tkach et al., Reference Tkach, Littlewood, Olson, Kinsella and Swiderski2003); the additional internal primers ECD2 (5ʹ-CCT TGG TCC GTG TTT CAA GAC GGG-3ʹ) (Littlewood et al., Reference Littlewood, Rohde and Clough1997) and 300F (5ʹ-CAA GTA CCG TGA GGG AAA GTT G-3ʹ) (Littlewood et al., Reference Littlewood, Curini-Galletti and Herniou2000) were used for Sanger sequencing. The partial mtDNA gene coding cytochrome c oxidase subunit I (hereinafter abbreviated as cox1) was amplified using the primers JB3 (forward; 5′-TTT TTT GGG CAT CCT GAG GTT TAT-3′) (Bowles et al., Reference Bowles, Blair and McManus1995) and CO1-R trema (reverse; 5′-CAA CAA ATC ATG ATG CAA AAG G-3′) (Miura et al., Reference Miura, Kuris, Torchin, Hechinger, Dunham and Chiba2005). PCR reactions were performed in a total volume of 25 μL and included 12.5 μL of MyFi™ Mix, 1.25 μL of each primer (10 mm), 8 μL of ddH2O and 1.5 μL of genomic DNA. The cycling parameters of PCR amplification followed those of Cutmore et al. (Reference Cutmore, Miller, Curran, Bennett and Cribb2013) for 28S and Koehler et al. (Reference Koehler, Springer, Keeney and Poulin2011) for cox1. Amplified products were purified with Exo-SAP-IT Kit™ Express Reagent (Thermo Fisher Scientific Baltics UAB, Vilnius, Lithuania) following the manufacturer's instructions, and sequenced with the same primers as were used in PCR, or alternatively with internal primers. The ABI PRISM BigDye Terminator Cycle Sequencing Ready Reaction Kit (Applied Biosystems-Perkin Elmer, Waltham, Massachusetts) and the MegaBACE sequencer (GE Healthcare Life Sciences) were used for sequencing. Contiguous sequences were assembled using Geneious v. 11 (Biomatters, Auckland, New Zealand) and deposited in GenBank.
Phylogenetic analyses
Two alignments (for 28S and cox1) including novel sequences of paramphistomoids and orthologue sequences retrieved from GenBank were built using the fast Fourier transform algorithm implemented in MAFFT software (Katoh et al., Reference Katoh, Misawa, Kuma and Miyata2002). The initial alignment built from orthologue sequences of 28S was used to assess the taxonomical designation and phylogenetic position of the collected paramphistomoid trematodes. The dataset included 8 sequences of Diplodiscus spp. available in GenBank and 1 of Catadiscus marinholutzi (Diplodiscidae). To assess the phylogenetic relationships of the investigated trematodes to cladorchiids, homologue sequences of 15 cladorchiid species belonging to 10 genera were also included in the analyses. Following the phylogeny proposed by Alves et al. (Reference Alves, Assis, López-Hernández, Pulido-Murillo, Melo, Locke and Pinto2020), an outgroup comprising Carmyerius spatiotus (Brandes, 1898) (JX518958), Fischoederius elongatus (Poirier, 1883) Stiles & Goldberger, 1910 (JX518966), Gastrothylax crumenifer (Creplin, 1847) (JX518971) (Gastrothylacidae) and Paramphistomum cervi (Zeder, 1790) Fischoeder, 1901 (KJ459936) (Paramphistomatidae) was used for the rooting of the phylogram. The final alignment was manually trimmed to the shortest available sequence to unify the length. The general time-reversible evolutionary model (GTR) was applied for the subsequent phylogenetic analyses. The gamma shape and proportion of invariable sites were calculated with jModelTest 2.1.2 (Darriba et al., Reference Darriba, Taboada, Doallo and Posada2012). Phylogenetic trees were constructed using the Bayesian inference (BI) and maximum likelihood (ML) approaches in MrBayes 3.2 (Ronquist et al., Reference Ronquist, Teslenko, van der Mark, Ayres, Darling, Höhna, Larget, Liu, Suchard and Huelsenbeck2012) and RaxML 8.1.12 (Stamatakis, Reference Stamatakis2006, Reference Stamatakis2014), respectively. BI analysis used the Metropolis-coupled Markov chain Monte Carlo algorithm with 2 parallel runs of 1 cold and 3 hot chains, which was run for 107 generations, sampling trees every 100 generations. The initial 30% of all saved trees were discarded as ‘burn-in’ after checking that the standard deviation split frequency fell below 0.01. Whether the runs and parameters of individual runs converged was checked using Tracer 1.7.1 (Rambaut et al., Reference Rambaut, Drummond, Xie, Baele and Suchard2018). Posterior probabilities (PP) for each tree node were calculated as the frequency of samples recovering a given clade. The clade bootstrap support (BS) for ML trees was assessed by simulating 103 pseudoreplicates.
The alignment built of cox1 sequences was used to assess the genetic diversity among the collected paramphistomoids and included 60 newly generated sequences and an orthologue sequence of D. nigromaculati extracted from its complete mitochondrial genome data (accession number MW698822). All sequences were trimmed to equalize their length and translated into amino acids to avoid any signal misreads. The sequence data were treated as codon partitioned, and a GTR model was selected independently for each position within the codon, including both a gamma distribution and the proportion of invariable sites. ML and BI analyses were conducted with the same number of iterations as for 28S. The cox1 sequence of Zygocotyle lunata (Diesing, 1836) (MT511682) (Paramphistomatidae) was used as the outgroup for rooting the phylogram. The level of DNA polymorphism in cox1 sequences, i.e., haplotype diversity (Hd), nucleotide diversity (π), number of unique haplotypes and number of variable sites, was assessed using DnaSP 5 (Librado and Rozas, Reference Librado and Rozas2009). The median-joining haplotype networks, constructed in PopART (Leigh and Bryant, Reference Leigh and Bryant2015) for each species individually, were used to assess population-genetic structure based on cox1 haplotypes. All new sequences generated in the present study are presented in Table 2, and those downloaded from GenBank are presented in Figs 2 and 3.

Fig. 2. Phylogenetic tree of 25 28S sequences of 9 Paramphistomoidea species reconstructed by Bayesian inference. The tree is based on 810 bp-long sequences and rooted using Carmyerius spatiosus, Gastrothylax crumenifer, Fischoederius elongatus and Paramphistomum cervi as the outgroup. Values at the nodes indicate posterior probabilities (>70) from the Bayesian inference, and bootstrap values (>50) from the maximum likelihood analysis. Lower values are shown as dashes (–). The length of branches represents the number of substitutions per site. The silhouettes at the taxa with coloured background represent common hosts of the respective trematode species – frogs. The new sequences generated from this study are in red.

Fig. 3. Phylogenetic tree of 61 cox1 sequences of 3 Paramphistomoidea species reconstructed by Bayesian inference. The tree is based on 683 bp-long sequences and rooted using Zygocotyle lunata as the outgroup. Values at the nodes indicate posterior probabilities (>70) from the Bayesian inference, and bootstrap values (>50) from the maximum likelihood analysis. Lower values are shown as dashes (–). The length of branches represents the number of substitutions per site. The numbers and letters in the squares represent clades and lineages further discussed in the Results section. The new sequences generated from this study are shown in colour: red – Opisthodiscus diplodiscoides; blue – Diplodiscus subclavatus.
Table 2. A list of sequenced Diplodiscus subclavatus and Opisthodiscus diplodiscoides individuals with respective hosts and collection sites

Results
Taxonomic diversity and the occurrence of water frog paramphistomes in the Balkans and Slovakia
According to morphology, 2 paramphistome species were identified. The first was the diplodiscid Diplodiscus subclavatus, which was collected from P. epeiroticus and P. kurtmuelleri at all 3 Balkan sites, and from P. esculentus and P. ridibundus at Devín, Gbelce, and Veľký Lél in Slovakia. Our specimens correspond to the morphology of Diplodiscus subclavatus originally described by Pallas (1760), mainly by (i) a pyriform body shape with an acetabulum on the posterior end, (ii) a bifurcated caecum reaching the anterior margin of the acetabulum, (iii) a genital pore near the oral opening, (iv) lateral vitelline follicles, (v) a uterus dorsal to testis and (vi) a singular equatorial and median testis at the level of the ovary. The highest prevalence of D. subclavatus per host population was reported from P. esculentus in Devín (P = 80%); however, the highest mean abundance was from P. epeiroticus in Ioannina (a = 3.80). In localities where P. ridibundus and P. esculentus occur sympatrically (i.e., Devín and Veľký Lél), D. subclavatus individuals were collected from both host species. The second trematode species was the cladorchiid Opisthodiscus diplodiscoides, which was present, contrastingly to D. subclavatus, in Slovakia but not in the Balkans. The species-specific morphological characteristics of the collected specimens were in accordance with the original description by Cohn (Reference Cohn1904), i.e., they had (i) a cylindrical body shape with an acetabulum on the posterior end, (ii) a large acetabulum with an accessory sucker, (iii) a bifurcated caecum terminating at the base of the acetabulum, (iv) a genital opening in front of the bifurcation of the caecum, (v) a uterus occupying the intercaecal field and (vi) 2 testes, preovarian, at the same zone. The overall mean abundance of, and intensity of infection by O. diplodiscoides was lower in comparison to D. subclavatus, and the highest mean abundance was observed in water frogs from Rusovce and Šulianske jazero (a = 0.90). In Veľký Lél, Opisthodiscus was collected only from P. esculentus but not from sympatrically living P. ridibundus. Surprisingly, no mixed infections of both paramphistome species were observed among the investigated host specimens. The quantitative descriptors of parasite populations for each host species per collection site are presented in Table 1.
Molecular characterization, genetic diversity and phylogenetic relationships of paramphistomes
Sequences of 28S and cox1 from 60 isolates were used to corroborate the taxonomic status of the sampled parasites. Ten and 60 novel sequences of 28S and cox1, respectively, were generated.
The final alignment for assessing the phylogenetic positions of the collected paramphistomes built from 28S sequences spanned 810 unambiguously aligned nucleotide positions. The ML and BI analyses generated trees with congruent topologies and, therefore, only the BI tree is presented in Fig. 2. The paramphistomes were divided into 2 well-supported phylogenetic lineages, with Catadiscus marinholutzi occupying a weakly supported basal position. The first phylogenetic lineage included 5 individuals of O. diplodiscoides, preliminary identified according to the morphology – all from water frogs from Slovakia. Weak intraspecific genetic divergence in 28S was observed among 5 analysed individuals (p-distance ⩽0.2%). The second lineage included sequences of all Diplodiscus spp. retrieved from GenBank and the novel sequences of 5 individuals of D. subclavatus. In comparison to O. diplodiscoides, no intraspecific genetic variability in 28S was observed among D. subclavatus individuals. Five isolates of D. subclavatus from our study differed from the D. subclavatus sequence obtained from GenBank by 6 nucleotide substitutions (p-distance = 0.8%), the GenBank sequence, AY222212, being a specimen collected from P. ridibundus in Bulgaria.
The second alignment was 683 bp long and included 60 novel isolates of cox1. Zygocotyle lunata (MT511682) was used as an outgroup for rooting the phylogenetic tree. The ML and BI analyses generated trees with congruent topologies and, therefore, only the BI tree is presented in Fig. 3. Congruently with 28S, the phylogenetic analyses divided the sequences of all investigated individuals into 2 well-supported clades. Clade 1 included the sequences of all individuals recognized as O. diplodiscoides, and 2 phylogenetic lineages were revealed within it. Lineage 1A (PP = 1, BS = 99) encompassed sequences of 19 specimens from all Slovakian collection sites where O. diplodiscoides was collected from P. ridibundus and P. esculentus (p-distance ⩽0.3%). In contrast, lineage 1B (PP = 1, BS = 95) included sequences of 5 parasites collected from P. esculentus from the site Veľký Lél (SK9). Slightly higher genetic variability was observed among haplotypes from lineage 1B in comparison to haplotypes within lineage 1A (p-distance ⩽0.7%). Clade 2 included the sequences of all individuals recognized as D. subclavatus, which showed a well-supported sister position to D. nigromaculati (PP = 1, BS = 75). Three phylogenetic lineages, with not-fully resolved relationships between them, were recognized within clade 2. Three observed lineages expressed a strong geographic pattern. The first lineage (2A; PP = 0.97, BS = 76) included sequences of 22 D. subclavatus individuals collected from Pelophylax frogs in Slovakia (p-distance ⩽0.6%). The second lineage (2B; PP = 1, BS = 98) encompassed 12 sequences of D. subclavatus individuals collected from P. epeiroticus in Greece, and P. kurtmuelleri in Albania. A notable geographical structure was observed among individuals in lineage 2B, as D. subclavatus individuals from P. kurtmuelleri in Albania were in the basal position to all Greek individuals, and individuals from Greek sites were divided into 2 lineages according to the collection sites (GR1 and GR2). The last lineage (2C; PP = 1, BS = 98) included the sequences of 2 D. subclavatus individuals collected from 1 P. epeiroticus individual in southern Greece (Peloponnese Peninsula): collection site GR3 (p-distance = 0.3%).
Population-genetic structure in 2 paramphistome taxa
According to the phylogenetic reconstruction, cox1 sequences were divided into 2 lineages: the first one included 24 sequences of O. diplodiscoides, and the second one included 36 sequences of D. subclavatus. A total of 6 unique cox1 haplotypes were recognized in O. diplodiscoides, and from 683 nucleotide positions, 71 sites were identified as polymorphic. The overall haplotype diversity (Hd) was 0.500, and nucleotide diversity (π) reached a value of 3.4%. The majority of the infrapopulations (i.e., parasite individuals from a single host specimen) shared the haplotype O_H1 (Fig. 4). The highest haplotype diversity was observed in individuals from Veľký Lél (site SK9), where, besides 1 individual carrying haplotype O_H1, 5 individuals carried a genetically divergent group of similar haplotypes O_H4-O_H6.

Fig. 4. Population-genetic structure of Opisthodiscus diplodiscoides found in Slovak populations of water frogs, based on cox1 haplotypes presented as a median-joining haplotype network. The sizes of the circles in the network are proportional to the relative frequencies of the haplotypes; small black circles represent missing haplotypes. The vertical lines represent the number of substitutions between individual haplotypes. Different colours represent sample sites according to the legend.
A total of 16 unique cox1 haplotypes were recognized in D. subclavatus, and from 683 nucleotide positions, 96 sites were identified as polymorphic. The overall haplotype diversity (Hd) was 0.778, and nucleotide diversity (π) reached a value of 4.5%. Infrapopulations from Slovakia carried 4 similar haplotypes (D_H1-D_H4), and 17 sequences out of 22 collapsed into haplotype D_H1, i.e., this haplotype was identified in individuals from all 3 localities in Slovakia where Diplodiscus was present. Sequences from south-western Balkan Diplodiscus formed 2 divergent haplogroups. Two similar haplotypes from southern-Greek Diplodiscus (Fig. 5, yellow) diverged from all other haplotype clusters. Interestingly, both Diplodiscus individuals were collected from a single frog. The other divergent group encompassed haplotypes from northern Greece and Albania, and most of them were represented by singletons. In general, Diplodiscus individuals from a single collection site carried genetically similar haplotypes (i.e., only 1 haplotype was recognized among individuals from the site AL1 and 2 similar haplotypes among individuals from the site GR1). The divergent haplotype D_H10 was recognized in 1 Diplodiscus individual from Ioannina (ex. 3299); however, it was genetically close to other haplotypes from this collection site (D_H11-D_H16). Similarly to the southern-Greek haplogroup, 2 divergent haplotypes were also recognized in an infrapopulations from 2 frogs (ex. 3609a – D_H11 and ex. 3609b – D_H14 and ex. 3614a – D_H13 and ex. 3614b – D_H12), suggesting repetitive infections of a single host by Diplodiscus.

Fig. 5. Population-genetic structure of Diplodiscus subclavatus based on cox1 haplotypes presented as a median-joining haplotype network. The sizes of the circles in the network are proportional to the relative frequencies of the haplotypes; small black circles represent missing haplotypes. Different colours represent sample sites according to the legend. The vertical lines represent the number of substitutions between individual haplotypes. The abbreviations in the legend represent countries where the collection site was situated: AL, Albania; GR, Greece; SK, Slovakia.
Discussion
Helminth communities in Pelophylax water frogs have been studied relatively extensively but most studies have employed only a basic morphological approach for species determination (e.g., Vojtková and Roca, Reference Vojtková and Roca1994, Reference Vojtková and Roca1996; Bjelić-Čabrilo, et al., Reference Bjelić-Čabrilo, Popović and Paunović2009; Popiołek et al., Reference Popiołek, Rozenblut-Kościsty, Kot, Nosal and Ogielska2011; Okulewicz et al., Reference Okulewicz, Hildebrand, Łysowski, Buńkowska and Perec-Matysiak2014; Herczeg et al., Reference Herczeg, Vörös, Végvári, Kuzmin and Brooks2016; Kuzmin et al., Reference Kuzmin, Dmytrieva, Marushchak, Morozov-Leonov, Oskyrko and Nekrasova2020). They were not focused on genetic diversity, and therefore might have underestimated the real species diversity of frog parasites. According to Carlson et al. (Reference Carlson, Dallas, Alexander, Phelan and Phillips2020), the helminth fauna of amphibians appears to be strongly underexplored in comparison to other vertebrate taxa, and even in Europe, we can find some regions, such as the Balkan Peninsula, which are still only superficially investigated in this regard. In the present study, we therefore focused on these neglected regions. By applying molecular methods, we revealed unexpected genetic variability in the mitochondrial cox1 fragment for diplodiscids, exhibiting also a geographic structure associated with the distribution of the Pelophylax frog hosts.
Our study revealed the presence of 2 paramphistome taxa in Slovak water frogs. The first was identified as Opisthodiscus diplodiscoides, with average to low prevalence per host population and low intensity of infection per examined host individual, and the second was Diplodiscus subclavatus, with comparatively higher prevalence and infection intensity. Previously, representatives of the genus Opisthodiscus have been found relatively rarely in European amphibians, in comparison to those of the genus Diplodiscus (Bailenger and Chanseau, Reference Bailenger and Chanseau1954; Vojtková et al., Reference Vojtková, Moravec and Nabelkova1963; Combes and Gerbaux, Reference Combes and Gerbaux1970; Combes and Sarrouy, Reference Combes and Sarrouy1971; Grabda-Kazubska, Reference Grabda-Kazubska1980; Martínez-Fernández et al., Reference Martínez-Fernández, Simón-Vicente and Cordero del Campillo1988; Vojtková and Roca, Reference Vojtková and Roca1994; Andreas, Reference Andreas2007; Navarro and Lluch, Reference Navarro and Lluch2006). In Western Europe, the genus Opisthodiscus is represented by O. nigrivasis parasitizing endemic Iberian P. perezi (López-Seoane, 1885) and widely distributed P. esculentus (Combes and Gerbaux, Reference Combes and Gerbaux1970; Combes and Sarrouy, Reference Combes and Sarrouy1971; Martínez-Fernández et al., Reference Martínez-Fernández, Simón-Vicente and Cordero del Campillo1988). In addition, Rodrigues (Reference Rodrigues2014) documented O. nigrivasis also from non-native Xenopus laevis (Daudin, 1802) in Portugal, indicating its strong host switch potential. In central and eastern Europe, the more common species is O. diplodiscoides, which was almost exclusively reported from P. esculentus or P. ridibundus (Vojtková, Reference Vojtková1974; Grabda-Kazubska, Reference Grabda-Kazubska1980; Andreas, Reference Andreas2007). Nevertheless, the identification of the 2 Opisthodiscus species is often unreliable in older literature, and, therefore, the real distribution ranges are uncertain and might overlap (Odening, Reference Odening1959). Vojtková et al. (Reference Vojtková, Moravec and Nabelkova1963) reported rare infections by O. diplodiscoides in P. esculentus in southern Slovakia (around city Komárno), which is congruent with our observations. We detected this parasite in P. ridibundus and P. esculentus frogs from all investigated sites in the Pannonian Basin. Most of them were geographically close to the sites from Vojtková et al. (Reference Vojtková, Moravec and Nabelkova1963) (SK1, SK4-SK6, SK8 and SK9), with only 1 site (SK7) situated further, in the north-western corner of the Pannonian Basin. Surprisingly, only in Veľký Lél (SK9) was O. diplodiscoides present in the host population also with D. subclavatus. However, in 20 examined P. esculentus individuals, we did not observe any instances of coinfection by both species, which might indicate that D. subclavatus and O. diplodiscoides exhibit antagonistic relationships. Interspecific competition between parasite taxa has been widely studied among adult helminths in their definitive vertebrate hosts (see Poulin, Reference Poulin2007 and references therein) and was also documented among anuran parasites sharing microhabitats within the host, i.e., between Rhabdias bufonis (Schrank, 1788) Stiles and Hassall, 1905 (Nematoda), Haematoloechus variegatus (Rudolphi, 1819) and Haplometra cylindracea (Zeder, 1800) (Trematoda) parasitizing the lungs of frogs (Mazurmovič, Reference Mazurmovič1957; Okulewicz et al., Reference Okulewicz, Hildebrand, Łysowski, Buńkowska and Perec-Matysiak2014), and between Aplectana acuminata (Schrank, 1788) (Nematoda) and Opisthioglyphe ranae (Fröhlich, 1791) (Trematoda), which are intestinal parasites (Mazurmovič, Reference Mazurmovič1957; Bjelić-Čabrilo et al., Reference Bjelić-Čabrilo, Popović and Paunović2009). The presence of 1 parasite species in the host might preclude other parasites from becoming established (Plasota, Reference Plasota1969; Bjelić-Čabrilo et al., Reference Bjelić-Čabrilo, Popović and Paunović2009). A similar situation might be hypothesized in the case of Diplodiscus and Opisthodiscus where both share the same microhabitat within the frog, i.e., the rectum and the terminal end of the intestine. An antagonistic relationship was suggested for these species also by Navarro and Lluch (Reference Navarro and Lluch2006), who similarly reported no mixed infection by D. subclavatus and O. nigrivasis in Moroccan P. saharicus (Boulenger, 1913). Unfortunately, interspecies relationships were not thoroughly investigated in the present study, and the limited host sample size per collection site, in combination with the relatively low prevalence of the 2 potentially antagonistic species, impede the drawing of complex conclusions. Thus, further research is necessary to reveal whether the antagonistic relationship is due to competitive resource exploitation (Mouritsen and Andersen, Reference Mouritsen and Andersen2017), the active elimination of a coexisting competitor (e.g., predation, Polis et al., Reference Polis, Myers and Holt1989), or the passive release of metabolites negatively impacting the competitor (i.e., interference competition, see review by Mideo, Reference Mideo2009).
The phylogenetic analyses suggested that the investigated Opisthodiscus individuals were divided into 2 phylogenetic lineages. The genetic divergence was supported by both molecular markers, and while the first phylogenetic group encompassed individuals from all collection sites where the presence of Opisthodiscus was documented, the second group included only 5 individuals collected from P. esculentus at the Veľký Lél site (SK9). Genetic variability was even more prominent in the mitochondrial cox1 gene, as 3 divergent haplotypes (O_H4-O_H6) were recognized in these 5 Opisthodiscus individuals. Such observations might indicate either the presence of 2 Opisthodiscus taxa in Southern Slovakia (e.g., O. diplodiscoides and O. nigrivasis, or O. diplodiscoides and another taxon) or substantial intraspecific differentiation within O. diplodiscoides. Unfortunately, a morphological evaluation that might have shed light on the taxonomic status of genetically diverged O. diplodiscoides individuals was not possible, as all 4 specimens collected from infrapopulations carrying haplotypes O_H1-O_H3 were either highly damaged (2 individuals), and therefore immeasurable, or did not show any significant differences in comparison to O. diplodiscoides individuals from infrapopulations carrying the divergent haplotypes O_H1-O_H3 (2 individuals). Such strong genetic differentiation might be due to the geographically distant origin of some Opisthodiscus individuals, potentially introduced with intermediate hosts (i.e., aquatic snails) or definitive hosts (i.e., amphibians). Information about the life cycle of Opisthodiscus is scarce; however, Simón-Vicente et al. (Reference Simón-Vicente, Martínez-Fernandez and Cordero Del Campillo1974) reported Ancylus fluviatilis (O. F. Müller, 1774) as the intermediate host for O. nigrivasis, a gastropod with a distribution range covering almost the entirety of Europe, including Slovakia (Cordellier and Pfenninger, Reference Cordellier and Pfenninger2008; Horsák et al., Reference Horsák, Čejka, Juřičková, Beran, Horáčková, Hlaváč, Dvořák, Hájek, Divíšek, Maňas and Ložek2021). Freshwater molluscs exhibit various upstream and downstream dispersal capabilities which can be even further increased by anthropogenic vectors (Kappes and Haase, Reference Kappes and Haase2012). Since the water bodies in Veľký Lél are river arms and gravel pits with direct communication with the Danube River, the Danube might represent an optimal pathway for the transmission of gastropods (including A. fluviatilis), thus introducing divergent genetic variants of larval stages of trematodes into geographically distant regions (e.g., O. diplodiscoides).
Diplodiscus subclavatus was collected from all 4 investigated Pelophylax species across both geographical regions, and exhibited a higher host range, and also genetic diversity, in comparison with the O. diplodiscoides. Diplodiscus species appear to be common parasites of Pelophylax frogs across the distribution range of the parasite genus, i.e., from east Asia (Men et al., Reference Men, Han, Zhao, Xia, Dong, Zhu, Wang, Li, Zhu and Huang2016) up to the western Mediterranean region (Navarro and Lluch, Reference Navarro and Lluch2006). In Europe, the only species of the genus is D. subclavatus (e.g., Bailenger and Chanseau, Reference Bailenger and Chanseau1954; Vojtková and Roca, Reference Vojtková and Roca1994; Andreas, Reference Andreas2007; Okulewicz et al., Reference Okulewicz, Hildebrand, Łysowski, Buńkowska and Perec-Matysiak2014; Herczeg et al., Reference Herczeg, Vörös, Végvári, Kuzmin and Brooks2016; Kuzmin et al., Reference Kuzmin, Dmytrieva, Marushchak, Morozov-Leonov, Oskyrko and Nekrasova2020), which was also documented in our study. The wide host range of this parasite species, exhibiting a life cycle with the planorbid snail as an intermediate host (e.g., Planorbis planorbis (Linnaeus, 1758), P. umbilicalis Benson, 1836, Anisus vortex (Linnaeus, 1758) or Gyraulus albus (O. F. Müller, 1774), Aho (Reference Aho, Esch, Bush and Aho1990); Cichy et al. (Reference Cichy, Faltýnková and Žbikowska2011)) and frogs as definitive hosts, suggests its low degree of host specificity, and therefore might explain its wide distribution range. Nevertheless, the genetic variability within the species was not previously studied.
The herein used mitochondrial genetic markers revealed exceptionally high genetic variability in cox1, although the more conservative 28S genetic marker did not show any variability among the investigated individuals. Both, the phylogenetic tree and the haplotype network, divided cox1 sequences into 3 divergent haplogroups. The relationships between haplogroups were not fully resolved in the phylogenetic tree; however, the network suggested that 2 Balkan haplogroups had a closer relationship to the haplogroup from Slovak D. subclavatus than to each other. Nonetheless, this might be an artefact of the genetic distances between the 3 haplogroups being too high, resulting in, at first sight, an illogical pairing in the network. In contrast to Slovak D. subclavatus, where no genetic structure was observed regarding the geographical distribution (i.e., the majority of infrapopulations carried the same haplotype), a spatial genetic structure was apparent in the Balkans - individuals from the same collection site carried similar haplotypes. Furthermore, in comparison to infrapopulations from Slovakia, the observed haplotype diversity was much higher in the Balkans. High intraspecific genetic diversity in southern Europe compared to the more northerly areas has been observed in many organisms and is associated with a long-term allopatric divergence in refugial populations during the Pleistocene glacials (e.g., Taberlet et al., Reference Taberlet, Fumagalli, Wust-Saucy and Cosson1998; Hewitt, Reference Hewitt2000, Reference Hewitt2004). Alternatively, high genetic diversity might also be a result of the current gene flow between adjacent populations that are not fully geographically isolated. The first assumption would be in congruence with the proposed phylogeographical scenario for Pelophylax frogs and their assumed historical dispersion. Molecular clocks indicate that the basal radiation within western Palearctic water frogs took place in the middle Miocene, with subsequent speciation events during the upper Miocene and the Pliocene (Lymberakis et al., Reference Lymberakis, Poulokakis, Manthalou, Tsigenopoulos, Magoulas and Mylonas2007; Wiens et al., Reference Wiens, Sukumaran, Pyron and Brown2009; Akın et al., Reference Akın, Bilgin, Beerli, Westaway, Ohst, Litvinchuk, Uzzell, Bilgin, Hotz, Guex and Plötner2010). Later, during the Pleistocene, it is supposed that water frogs (and also other organisms) expanded to the north during the interglacial periods and retracted back to the south in the glacials (Snell et al., Reference Snell, Tetteh and Evans2005; Hoffmann et al., Reference Hoffmann, Plötner, Pruvost, Christiansen, Röthlisberger, Choleva, Mikulíček, Cogalniceanu, Sas-Kovacs, Shabanov, Morozov-Leonov and Reyer2015). During the ice ages, these southern regions served as refugia which were often divided into several microrefugia (Schmitt, Reference Schmitt2007; Schmitt and Varga, Reference Schmitt and Varga2012). As the distribution and dispersion of parasites are strongly limited and dependent on the dispersal capabilities of their hosts (Blouin et al., Reference Blouin, Yowell, Courtney and Dame1995), it is tempting to assume that a similar distribution pattern will be observed also for the parasites of Pelophylax frogs.
The existence of microrefugia in the Balkans might also explain the existence of 2 diverse haplogroups among the Balkan Diplodiscus. Recent studies indicate that the Peloponnese Peninsula might have represented such a microrefugium for several taxa (e.g., Mesobuthus scorpions (Parmakelis et al., Reference Parmakelis, Stathi, Chatzaki, Simaiakis, Spanos, Louis and Mylonas2006); Parnassius butterflies (Gratton et al., Reference Gratton, Konopinski and Sbordoni2008); Anguis legless lizards (Jablonski et al., Reference Jablonski, Jandzik, Mikulíček, Džukić, Ljubisavljević, Tzankov, Jelić, Thanou, Moravec and Gvoždík2016); or Pelobates frogs (Dufresnes et al., Reference Dufresnes, Strachinis, Tzoras, Litvinchuk and Denoël2019). Moreover, Radojičić et al. (Reference Radojičić, Krizmanić, Kasapidis and Zouros2015) observed genetic divergence between P. epeiroticus populations in Northern and Southern Greece, further supporting our assumption of the divergence of Pelophylax frogs with Diplodiscus.
Congruently with our observations, from the genus Diplodiscus only D. subclavatus was previously reported from Slovakia (Vojtková et al., Reference Vojtková, Moravec and Nabelkova1963; Vojtková, Reference Vojtková1974) and the Balkans (Vojtková and Roca, Reference Vojtková and Roca1994). Surprisingly, the phylogenetic reconstruction based on 28S and the computation of pair-wise genetic distances revealed quite substantial genetic differences between D. subclavatus in our study and the sequence used as a representative of the species which was employed in phylogeny by Olson et al. (Reference Olson, Cribb, Tkach, Bray and Littlewood2003) (AY222212).
Although the observed high genetic variability might suggest the existence of 3 divergent Diplodiscus taxa in the studied regions – 2 in the Balkans and 1 in Slovakia – the morphological data (i.e., morphometrics) did not reveal any statistically significant differences between individuals from the respective parasite metapopulations. However, due to the insufficient quality of the material, and its scarcity, we couldn't obtain a comparable dataset from each suggested group, and, therefore, further collection and morphological re-evaluation are necessary to confirm the taxonomical status of the 3 genetic haplogroups.
The phylogenetic relationships within the family Diplodiscidae were first tackled by Sey (Reference Sey1983), who, according to the morphological apomorphies and geographical distribution, split diplodiscids into 2 lineages – Opisthodiscinae, encompassing Opisthodiscus and Megalodiscus, and Diplodiscinae, encompassing all other Diplodiscidae genera. Later, Sey (Reference Sey1988, Reference Sey1991) re-classified Opisthodiscus and Megalodiscus into Megalodiscinae within Cladorchiidae; however, this taxonomical revision was not generally accepted. Our reconstruction based on the conservative 28S gene did not support the monophyly of the family Cladorchiidae (sensu Alves et al., Reference Alves, Assis, López-Hernández, Pulido-Murillo, Melo, Locke and Pinto2020), although it was previously suggested (nonetheless, only on the basis of weak support). Even though Opisthodiscus and Megalodiscus share similar morphological traits (e.g., 2 testes, and an oesophagus that is shorter than the pharynx, Sey, Reference Sey1991), recent phylogenetic analyses based on molecular data (Alves et al., Reference Alves, Assis, López-Hernández, Pulido-Murillo, Melo, Locke and Pinto2020; Queiroz et al., Reference Queiroz, Alves, López- Hernández, Anjos and Pinto2021; and this study) suggest that these 2 genera are in paraphyly, and while the position of Opisthodiscus is nested within Diplodiscidae (i.e., a sister position to species of the genus Diplodiscus), Megalodiscus is positioned within Cladorchiidae. Thus, we can only assume that convergent adaptation to amphibian hosts evolved among paramphistomoids in different geographical regions; however, to fully resolve the classification of cladorchiids, more comprehensive morphological evaluation is required. The sister position (albeit weakly supported) of South American Catadiscus to Eurasian Opisthodiscus and Diplodiscus with a deep nodal split might indicate historical allopatric divergence during the ancient division of the continents (as proposed by Sey, Reference Sey1983). Although, the monophyly of Diplodiscidae was not fully supported by Queiroz et al. (Reference Queiroz, Alves, López- Hernández, Anjos and Pinto2021), the current addition of Opisthodiscus into phylogeny helps to shed more light on the relationships within this family. Moreover, with further addition of (still missing) molecular data from other genera from the family Diplodiscidae (i.e., Progonimodiscus Vercammen-Grandjean, 1960 (in frogs from Africa), Dermatemytrema Price, 1937 (in turtles from North America), Pseudodiplodiscus Manter, 1962 (in fish from South America) and Australodiscus Sey, Reference Sey1983 (in frogs from Oceania – Australia) (Sey, Reference Sey1991; Jones, Reference Jones, Jones, Bray and Gibson2005)) might help to finally resolve relationships of the diplodiscids to the cladorchiids, and further elucidate the historical dispersion routes and diversification within diplodiscids.
Acknowledgements
We are grateful to Adam Javorčík, Simona Papežíková and Lucie Seidlová for assistance in the field. Scientific permits were provided by the Directorate of Forest Management, the Ministry for Environment and Energy of the Hellenic Republic (181012/807/28-3-2019); the National Agency of Protected Areas, the Ministry of Tourism and Environment of the Albanian Republic (No. 480/2019) and the Ministry of the Environment of the Slovak Republic (No. 2963/2013-2.2). We kindly thank Matthew Nicholls for English revision of the final draft.
Author contributions
MB conceived and designed the study. MB, PM, PP and ZŽ collected parasitological material, and MB and ZŽ performed microscopical observations and determination of the species. MB and CP performed laboratory procedures and phylogenetic and statistical analyses. MB wrote the draft of the manuscript and all other authors critically reviewed it. All authors read and approved the final version of the manuscript.
Financial support
The study was supported by the Scientific Grant Agency of the Slovak Republic VEGA 1/0286/19, and the Slovak Research and Development Agency under contract APVV-19-0076.
Conflict of interest
The authors declare that there are no conflicts of interest.
Ethical standards
All applicable institutional, national and international guidelines for the care and use of animals were followed. This study was approved by the Animal Care and Use Committee of the Faculty of Science, Masaryk University in Brno (Czech Republic).