Introduction
Maize has the greatest potential for increased production and productivity in the savannah belt of sub-Saharan Africa (SSA) due to high solar radiation, low night temperatures and low incidence of pests and diseases that characterize the region. Despite this potential, grain yield of maize in farmers' fields has been consistently low, with an average of 1.7 t/ha (FAO, 2016). This challenge has been ascribed to several factors including Striga hermonthica infestation, drought and low nitrogen (N).
The response of maize to drought and low-N has been found to be influenced by similar mechanisms (Badu-Apraku et al., Reference Badu-Apraku, Akinwale, Franco and Oyekunle2012) and the two stresses together cause significant yield reduction in SSA (Badu-Apraku et al., Reference Badu-Apraku, Fakorede, Oyekunle and Akinwale2011a). Drought at flowering and grain filling periods may cause losses of 40–90% (Menkir and Akintunde, Reference Menkir and Akintunde2001). Grain yield losses resulting from low-N, on the other hand, could vary between 10 and 50% (Wolfe et al., Reference Wolfe, Henderson, Hsiao and Alvio1988). Improving maize to tolerate drought and low-N conditions is crucial to the ongoing efforts to reduce the yield gap between optimal and stress conditions. Edmeades (Reference Edmeades2013) found that about 0.20–0.25 of the yield gap between potential yield and moisture-limited yield could be eliminated by genetic improvement for drought and low-N tolerance.
Vitamin A is essentially needed by human beings for improved eyesight and enhanced immune system. Unfortunately, the requirement for vitamin A in humans is met through external sources because the human body is unable to synthesize this important micronutrient. Provitamin A (PVA) maize has the potential to supply more than the daily dietary requirement of 15.0 µg/g dry weight (DW) PVA for human beings as compared to about 2.0 µg/g DW available in the commonly cultivated and consumed yellow maize cultivars (Pixley et al., Reference Pixley, Rojas, Babu, Mutale, Surles, Simpungwe and Tanumihardjo2013). Efforts at improving the PVA content in maize have resulted in the discovery of three genes [phytoene synthase1 (PSY1), lycopene epsilon cyclase (LcyE) and β-carotene hydroxylase1 (crtRB1)] that regulate the key steps involved in the synthesis and accruing of PVA carotenoids (Wurtzel et al., Reference Wurtzel, Cuttriss and Vallabhaneni2012). The allele crtRB1 (−5′TE and 3′TE) has been the most functional for increased β-carotene contents (Yan et al., Reference Yan, Kandianis, Harjes, Bai, Kim, Yang, Skinner, Fu, Mitchell, Li, Fernandez, Zaharieva, Babu, Fu, Palacios, Li, DellaPenna, Brutnell, Buckler, Warburton and Rocheford2010).
Conventional maize has inadequate levels of tryptophan and lysine and therefore its consumption without a balanced protein source, especially by infants, could result in initial growth failures such as ‘kwashiorkor’, reduced immune system and consequently, death (Sultana et al., Reference Sultana, Jamil, Aslam, Shahzad, Fatima, Maqbool and Iqbal2019). On the contrary, quality protein maize (QPM) has the potential to supply about 0.73 of human protein requirements compared to about 0.46 from conventional maize (Badu-Apraku and Fakorede, Reference Badu-Apraku, Fakorede, Badu-Apraku and Fakorede2017). Studies aimed at elevating the protein content of maize have resulted in identification of the recessive homozygous allele of the opaque-2 gene (o2o2) and its modifiers, which are capable of doubling the tryptophan and lysine contents of QPM relative to their conventional counterparts (Ma and Nelson, Reference Ma and Nelson1975; Crow and Kermicle, Reference Crow and Kermicle2002; Krivanek et al., Reference Krivanek, De Groote, Gunaratna, Diallo and Friesen2007; Badu-Apraku et al., Reference Badu-Apraku, Fakorede, Talabi, Oyekunle, Akaogu, Akinwale, Annor, Melaku, Fasanmade and Aderounmu2016).
Through the concerted efforts of the International Institute of Tropical Agriculture Maize Improvement Programme (IITA-MIP), PVA-QPM inbred lines with drought and low-N tolerance genetic backgrounds have been developed over the years to produce hybrids and synthetic varieties. Although several studies on the combining ability using different mating designs and heterotic grouping of maize inbred lines under contrasting environments have been conducted in SSA (Menkir et al., Reference Menkir, Badu-Apraku, Thé and Adepoju2003; Badu-Apraku et al., Reference Badu-Apraku, Oyekunle, Fakorede, Vroh, Akinwale and Aderounmu2013, Reference Badu-Apraku, Annor, Oyekunle, Akinwale, Fakorede, Talabi, Akaogu, Melaku and Fasanmade2015; Annor and Badu-Apraku, Reference Annor and Badu-Apraku2016), it is still very important to conduct such studies for newly developed inbred lines because there have been inconsistent reports on the type of gene action conditioning the inheritance of grain yield and other agronomic traits in maize under drought and low-N conditions. Furthermore, unlike lysine and tryptophan which have been widely reported to be influenced by maternal and additive genetic effects (Ngaboyisonga et al., Reference Ngaboyisonga, Njoroge, Kirubi and Githiri2009; Badu-Apraku and Fakorede, Reference Badu-Apraku, Fakorede, Badu-Apraku and Fakorede2017; Varadaraju and Joel, Reference Varadaraju and Joel2017), there have been inconsistent reports on the gene action controlling the inheritance of PVA accumulation. Several workers have reported the preponderance of additive over non-additive genetic effects in determining PVA concentrations in maize (Egesel et al., Reference Egesel, Wong, Lambert and Rocheford2003; Suwarno, Reference Suwarno2012; Menkir et al., Reference Menkir, Gedil, Tanumihardjo, Adepoju and Bossey2014; Owens et al., Reference Owens, Lipka, Magallanes-Lundback, Tiede, Diepenbrock, Kandianis, Kim, Cepela, Mateos-Hernandez, Buell, Buckler, DellaPenna, Gore and Rocheford2014; Suwarno et al., Reference Suwarno, Pixley, Palacios-Rojas, Kaeppler and Babu2015), suggesting that using recurrent selection methods for the improvement of PVA content would be successful (Coors, Reference Coors, Coors and Pandey1999). Furthermore, Menkir et al. (Reference Menkir, Liu, White, Maziya-Dixon and Rocheford2008) and Owens et al. (Reference Owens, Lipka, Magallanes-Lundback, Tiede, Diepenbrock, Kandianis, Kim, Cepela, Mateos-Hernandez, Buell, Buckler, DellaPenna, Gore and Rocheford2014) reported positive correlations among individual carotenoids while Menkir et al. (Reference Menkir, Gedil, Tanumihardjo, Adepoju and Bossey2014) as well as Suwarno et al. (Reference Suwarno, Pixley, Palacios-Rojas, Kaeppler and Babu2015) found positive relationships between PVA content and grain yield. These findings suggested that simultaneous increases in PVA carotenoids and grain yield may be effective (Bouis and Welch, Reference Bouis and Welch2010; Menkir et al., Reference Menkir, Gedil, Tanumihardjo, Adepoju and Bossey2014). On the contrary, Halilu et al. (Reference Halilu, Ado, Aba and Usman2016) reported non-additive gene action to be more important in controlling the PVA carotenoid concentrations. They also found no significant genotypic correlations between carotenoid content and grain yield, suggesting that the two traits could be improved independently (Halilu et al., Reference Halilu, Ado, Aba and Usman2016).
Assessing the combining ability of the panel of newly developed IITA early (90–95 days to physiological maturity) inbred lines is necessary to guide breeding strategies under low-N, drought and optimal growing environments. Classifying inbred lines into different heterotic groups will help to reduce the development and evaluation of less productive crosses, while exploiting maximum heterosis by crossing opposing inbred lines (Terron et al., Reference Terron, Preciado, Cordova, Mickelson and Lopez1997). The current study was designed to (i) examine the combining ability of selected early maturing PVA-QPM inbred lines for grain yield and other traits under low-N, drought and optimal conditions, and across environments, (ii) determine the gene action conditioning PVA accumulation under optimal growing environments, (iii) categorize the inbred lines into heterotic groups and identify the best testers across the contrasting environments and (iv) assess the grain yield and stability of the single crosses across test environments.
Materials and methods
Genetic materials and development of inbred lines and hybrids
The 24 early-maturing PVA-QPM inbred lines used in the study were developed by the IITA-MIP (Table 1). The inbred lines were selected based on their reactions to drought and low-N environments in previous studies (Badu-Apraku and Fakorede, Reference Badu-Apraku, Fakorede, Badu-Apraku and Fakorede2017). For the PVA and quality protein traits, kernel colour and endosperm modification, respectively, were used to select parental lines. The 24 parental inbreds were grouped into six sets with each set containing four inbred lines that were used to generate 96 single cross hybrids, employing the North Carolina Design II (NCD II) method proposed by Comstock and Robinson (Reference Comstock and Robinson1948) during the 2015/2016 dry season at IITA, Ibadan, Nigeria.
Table 1. Description of the 24 early maturing PVA-QPM inbreds selected for the NCD II crosses in the 2015/16 dry season at IITA Ibadan, Nigeria
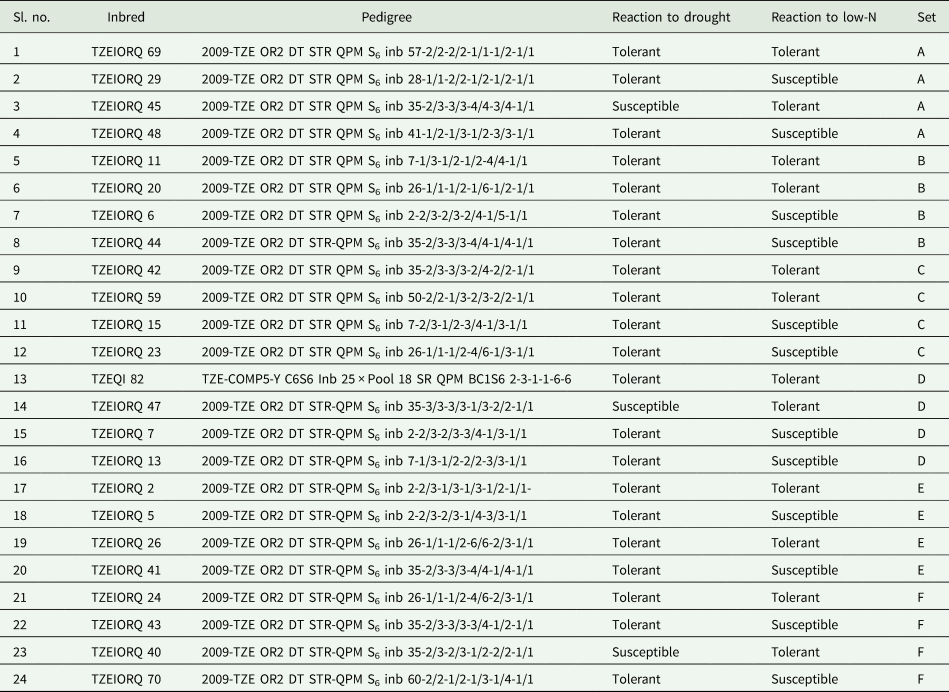
Field evaluations under the different environmental conditions
The 96 PVA-QPM single cross hybrids plus four controls were assessed under drought, low-N and optimal growing conditions in Nigeria, for 2 years each. The managed drought experiments were conducted in the dry seasons at Ikenne (forest–savannah transitional zone, 7°52′N, 30°44′E, 61 m a.s.l., 1200 mm mean annual precipitation) from November 2016 to February 2017 and at Kadawa (semi-arid Sudan savannah, 12°45′N, 9°45′E, 469 m a.s.l., 884 mm mean annual precipitation) from February to May 2018. The managed drought condition at Ikenne was attained by providing 17 mm of irrigation water every week from sowing until 28 days after planting (DAP), when the irrigation was terminated and the hybrids depended on stored soil moisture to reach physiological maturity. Nitrogen–phosphorus–potassium (NPK at 15 : 15 : 15) fertilizer was applied to the managed drought trials at the rate of 60 kg/ha each of N, P and K at planting. Additionally, 60 kg/ha of urea was applied 2 weeks after planting. The managed drought at Kadawa was achieved using a furrow irrigation system that also provided water once per week up to 28 DAP, when the irrigation was terminated. However, irrigation was resumed 2 weeks after flowering to avoid total loss of the trial due to the intense heat that characterizes Kadawa between February and March and the characteristic sandy and shallow top soil of the test environment.
The hybrids were also evaluated under low-N (30 kg/ha) environments at Ile-Ife (forest-savannah transition, 8°28′N, 5°34′E, and 245 m a.s.l., 1300 mm mean annual precipitation) and Mokwa (Southern Guinea savannah, 9°20′N, 5°5′E, 458 m a.s.l., 1100 mm mean annual precipitation) during the 2016 and 2017 growing seasons. The experimental fields at both locations were depleted of N by continuously sowing densely populated maize and removing all crop residues after harvest for three seasons. Before the low-N fields were prepared, soil samples from 0 to 15 cm depth were taken for analysis using the colorimetric and Kjeldahl digestion method (Bremner and Mulvaney, Reference Bremner, Mulvaney, Page, Miller and Keeney1982) to determine the levels of N, P and K. The soil from the low-N experimental field at Mokwa contained 0.85 g/kg of N, 0.006 g/kg of P and 0.20 g/kg of K, while that of Ile-Ife had 0.84 g/kg of N, 0.002 g/kg of P and 0.358 g/kg of K. Based on the soil tests, NPK fertilizer was formulated using urea, single-superphosphate and muriate of potash, respectively, which was applied at 14 DAP immediately after thinning to bring the levels of the total available basal N to 15 kg/ha. The levels of the single superphosphate and muriate of potash fertilizers applied were 60 kg/ha each of P and K. Additional 15 kg/ha of urea was applied at 28 DAP to bring the total available N to 30 kg/ha. Hybrid evaluations were carried out under optimal growing conditions at Ile-Ife, Mokwa and Ikenne during the 2016 and 2017 growing seasons. The soil at Ikenne and Ile-Ife is Alfisol, while that of Kadawa and Mokwa is Eutric Gambisol and Luvisol, respectively (Soil Survey Staff, 1999).
For the experiments planted under optimal conditions, the NPK (15 : 15 : 15) fertilizer was applied at 14 DAP to make available 60 kg/ha each of N, P and K. Additionally 30 kg N/ha was used for the top dressing at 28 DAP. A 10 × 10 alpha lattice design, replicated twice, was used for all hybrid experiments. Single-row plots each 4 m long, with a spacing of 0.75 m between rows and 0.40 m within rows, constituted an experimental unit. A plant population density of about 66 666 per ha was obtained by planting three seeds per hill and later thinning to two plants per stand. The inbred lines were also evaluated in adjacent blocks along with the hybrids in the different test environments. Each experimental unit of the inbred trials consisted of one-row plot each with a spacing of 0.75 m between rows and 0.40 m within rows. Weed control in the inbred and hybrid experiments was achieved by the application of pre- and post-emergence herbicides (atrazine and gramoxone) at 5 litres/ha followed by manual weeding.
Data collection
Data were collected for 50% days to anthesis (DA) and silking (DS), and plant and ear heights (PLHT and EHT). Plant aspect (PASP) was rated on a scale of 1–9, where 1 represented excellent overall phenotypic appeal and 9 extremely poor overall phenotypic appeal. Ear aspect (EASP) was also scored on a scale of 1–9, where 1 denoted excellent ears with well-filled grains without any damage resulting from insect and disease attack while 9 represented plots with only one or no ears. The difference between DA and DS was calculated as anthesis–silking interval, while the number of ears per plant was obtained as the ratio of the number of ears harvested in a plot to the number of plants. The low-N and drought trials were rated for stay-green characteristic (STGR) at 70 DAP [growth stage (GS)85 = soft dough development (Zadoks et al., Reference Zadoks, Chang and Konzak1974)] using a scale of 1–9 where 1 = 0–0.10 dead leaf area, and 9 = 0.81–1.00 dead leaf area. For the drought and low-N trials, grain weight per plot was measured. Grain moisture was determined and grain yield in kg/ha was subsequently calculated using the grain weight adjusted to 15% moisture content. For the optimal trials however, a shelling percentage of 80 was assumed per plot for the hybrids and grain yield (adjusted to 15% moisture content) based on ear weight was calculated and converted to kg/ha.
Generation of kernel samples of hybrids for carotenoids and tryptophan analyses
Fifty-four selected PVA-QPM hybrids (out of the 96 hybrids generated) plus a standard QPM variety control, ‘Obatanpa’, were planted under well-watered growing conditions in January 2018 at IITA Ibadan, to produce kernel samples for carotenoid and tryptophan analyses. These were the hybrids that yielded as much or more than the estimated mean grain yield across environments, and 54 were used on the basis of retaining three out of four inbred lines per set in order to maintain the NCD II arrangement for genetic analysis. This was due to cost implications as quantification of the carotenoids and tryptophan was very expensive. The hybrids were planted using 1 m single row plots with spacings of 0.75 and 0.20 m. Two seeds were sown per hill and were thinned to one plant to provide a minimum of five plants per hybrid. Plants in each plot were self-pollinated to produce seed samples for chemical analysis. Ears of the self-pollinated F1 hybrids were harvested and air-dried. Individual ears of each plot were shelled separately and an equal number of kernels from each ear were taken and bulked to represent a hybrid. Samples of 100 kernels for each hybrid were collected and analysed for carotenoid and tryptophan levels in the IITA nutritional laboratory.
Analysis of hybrids for carotenoids and tryptophan contents
The high-performance liquid chromatography method was used for the extraction and quantification of carotenoids based on the protocol described by Howe and Tanumihardjo (Reference Howe and Tanumihardjo2006). Beta-carotene (cis and trans isomers), α-carotene, β-cryptoxanthin, zeaxanthin and lutein were quantified using external standards. Total carotenoids were computed as the sum of concentrations of α-carotene, β-carotene, lutein, zeaxanthin and β-cryptoxanthin. PVA was computed as the sum of β-carotene, and half of each of β-cryptoxanthin and α-carotene contents, since β-cryptoxanthin and α-carotene contribute half of the value of β-carotene as PVA (US Institute of Medicine, 2001). Values of all carotenoids for each sample were obtained from two independent measurements.
Furthermore, the selected hybrids were analysed for tryptophan levels in whole grain flour using the colorimetric method proposed by Hernández and Bates (Reference Hernández and Bates1969). Tryptophan content was determined with the aid of a standard curve of a known control and values for each sample were obtained from two independent measurements. Percent tryptophan was expressed in terms of a given unit of samples in whole grain (Teklewold et al., Reference Teklewold, Wegary, Tadesse, Tadesse, Bantte, Friesen and Prasanna2015).
Statistical analysis
Analysis of variance
The combination of location and year represented an environment while the low-N, drought and optimal conditions were regarded as research conditions. The NCD II analysis of variance (ANOVA) for each and across research conditions were performed on plot means for all data collected using the general linear model procedure (PROC GLM) implemented in Statistical Analyses System, version 9.4 (SAS Institute, 2012). In the model, environments, replicates within environments and incomplete blocks within replicates × environment interaction were considered as random factors whereas the set of hybrids was regarded as a fixed factor. Block effects on hybrid means were adjusted using the lattice design proposed by Cochran and Cox (Reference Cochran and Cox1960) and standard error of difference (s.e.d.) was used for the separation of means.
The variation due to hybrids was separated into male sets, female sets and male × female interaction sets. The mean squares of male sets, female sets and female × male sets were subjected to F-test using their respective interactions with the environment. The male × female × environment within sets mean squares were tested using the pooled error mean squares. The main effects of male sets plus female sets constituted the general combining ability (GCA) effects and that of male × female sets interaction was the specific combining ability (SCA) effects (Hallauer and Miranda, Reference Hallauer and Miranda1988). Similarly, ANOVA of PVA was performed for the 54 selected hybrids plus one control only for the data collected under the optimal environment at Ibadan, Nigeria.
Proportionate contribution of general combining ability and specific combining ability effects of traits
The proportionate contribution of each agronomic trait was computed as percentage of the sum of squares for the crosses ascribed to GCA and SCA (Singh and Chaudhary, Reference Singh and Chaudhary1985; Annor and Badu-Apraku, Reference Annor and Badu-Apraku2016) and standard errors for GCA and SCA effects were estimated by the method proposed by Cox and Frey (Reference Cox and Frey1984). The significance of the GCA-female, GCA-male, and SCA effects of the individual inbred lines were determined using the respective standard errors. In addition, variance ratio of the mean squares of the GCA-male and female were compared (Kearsey and Pooni, Reference Kearsey and Pooni1996) to determine the relative significance of cytoplasmic effects. Similarly, the proportion of GCA and SCA effects and the significance of maternal and paternal contributions were estimated for PVA content under optimal growing conditions.
Heterotic grouping of inbred lines
The heterotic grouping based on GCA of multiple traits also referred to as the HGCAMT method (Badu-Apraku et al., Reference Badu-Apraku, Oyekunle, Fakorede, Vroh, Akinwale and Aderounmu2013) was employed to group the inbred lines under each and across environments. This was done by identifying the traits with significant mean squares across the test environments (low-N, drought and optimal) and standardizing their GCA effects to reduce the effects of the different rating scales used to measure the traits. Importance was, however, attached to the six traits employed in the low-N and drought base index, which included stay-green characteristic, anthesis–silking interval, plant and ear aspects, ears per plant and grain yield. Thereafter, the standardized GCA values were used for cluster analysis based on Ward's minimum variance method implemented in SAS (SAS Institute, 2012) to group the inbreds under the different environments.
Identification of inbred testers and selection of low-nitrogen and drought tolerant single crosses
The criteria proposed by Pswarayi and Vivek (Reference Pswarayi and Vivek2008) were adopted to identify an inbred tester. According to the criteria, a tester must (i) belong to a known heterotic group, (ii) have a high significant positive GCA across the test environments and (iii) have high yield per se. In addition to these criteria, the inbred tester was expected to have significant positive GCA-male and female effects for PVA and its carotenoids (Halilu et al., Reference Halilu, Ado, Aba and Usman2016). Identification of low-N and drought tolerant inbreds and hybrids was aided by the multiple trait base index (MI) under each of the stress conditions as proposed by Badu-Apraku et al. (Reference Badu-Apraku, Fakorede, Oyekunle and Akinwale2011a). A positive MI value indicated low-N or drought tolerance while a negative index denoted susceptibility of a genotype to the stressful conditions (Badu-Apraku et al., Reference Badu-Apraku, Fakorede, Oyekunle and Akinwale2011a). Furthermore, the MI was applied to data across drought and low-N environments to assess the reactions of the inbred lines to the two stresses.
Identification of high-yielding and stable hybrids across environments
Using the MI across drought and low-N environments, a set of 29 hybrids, including the best 15 drought and low-N tolerant, and 10 most drought and low-N susceptible hybrids, as well as the four controls were selected for G × E analysis using the GGE-biplot package (Yan, Reference Yan2001). The most promising hybrids across low-N, drought and optimal environments were identified and their stability across environments was examined.
Results
Analysis of variance of agronomic traits under the contrasting environments
Across the two drought environments, the ANOVA showed significant (P < 0.05 or P < 0.01) differences among environment (E), genotype (G) and genotype × environment interaction (G × E) mean squares for grain yield and all traits except for the E mean squares for anthesis–silking interval (Table 1). Significant (P < 0.05 or P < 0.01) variations were also observed for GCA-male and female, SCA and their interactions with E for all traits except SCA × E effects for ear and plant aspects.
Across the four low-N environments, significant (P < 0.05 or P < 0.01) mean squares of E, G and G × E interaction were revealed for grain yield and other measured traits except G × E interaction for anthesis–silking interval (Table 2). The GCA-male, GCA-female, SCA, GCA-male × E, GCA-female × E, and SCA × E revealed significant (P < 0.05 or P < 0.01) differences for all traits under low-N except for SCA effect for anthesis–silking interval. Under optimal environments, significant differences were observed among E, G and G × E interaction mean squares for all traits but not for anthesis–silking interval (Table 3). Similarly, GCA-male, GCA-female and SCA effects were significant (P < 0.05 or P < 0.01) for all traits except for anthesis–silking interval. Significant (P < 0.05 or P < 0.01) GCA-male × E, GCA-female × E and SCA × E were obtained for all traits except for anthesis–silking interval. Across environments, significant (P < 0.05 or P < 0.01) differences were observed among E, G and G × E interaction mean squares for all measured traits except for anthesis–silking interval for G × E interaction (Table 3). Furthermore, effects of GCA-male, GCA-female, SCA, GCA-male × E, GCA-female × E and SCA × E were significant (P < 0.05 or P < 0.01) for all traits except for the GCA-male, GCA-female and their interactions with E for anthesis–silking interval as well as the SCA × E for anthesis–silking interval.
Table 2. Mean squares of grain yield and other agronomic traits of early maturing PVA-QPM hybrids evaluated under drought at Ikenne during the 2016/17 and 2017/18 dry seasons and under low-N conditions at Ile-Ife and Mokwa during the 2016 and 2017 growing seasons in Nigeria

Env, environment; GY, grain yield (kg/ha); DS, days to 50% silking; ASI, anthesis–silking interval; PLHT, plant height (cm); PASP, plant aspect (1–9); EASP, ear aspect (1–9); EPP, ears per plant; STGR, stay-green characteristic (1–9).
Table 3. Mean squares of grain yield and other agronomic traits of early maturing PVA-QPM hybrids under optimal environments at Ikenne, Ile-Ife and Mokwa during the 2016 and 2017 growing seasons and across all the test environments in Nigeria

Env, environment; GY, grain yield (kg/ha); DS, days to 50% silking; ASI, anthesis–silking interval; PLHT, plant height (cm); PASP, plant aspect (1–9); EASP, ear aspect (1–9); EPP, ears per plant; STGR, stay-green characteristic (1–9).
Analysis of variance of carotenoids and tryptophan and proportionate contributions of combining ability effects
Significant (P < 0.05 or P < 0.01) variations were detected among the hybrids for all PVA carotenoids and tryptophan (Table 4). Also, significant (P < 0.05 or P < 0.01) differences were observed among GCA-male, GCA-female and SCA effects for all PVA carotenoids, and tryptophan except for the SCA effects of β-cryptoxanthin, β-carotene and PVA.
Table 4. Mean squares and narrow sense heritability estimates of carotenoids and tryptophan of selected early maturing PVA-QPM hybrids

Carotenoids are abbreviated as Lut, lutein; Zeax, zeaxanthin; β-cryp, β-cryptoxanthin; α-caro, alpha-carotene; β-caro, β-carotene; PVA, provitamin A; Tcaro, total carotenoid; Tryp, tryptophan.
The contributions of GCA (male + female) sum of squares were greater than SCA effect for all traits under drought, low-N and optimal conditions as well as across environments. GCA accounted for 67.2, 58.4, 60.3 and 59.3% of the sum of squares for grain yield under drought, low-N, optimal, and across research conditions, respectively. GCA effect was 75.9% for stay-green characteristic under drought and 57.6% under low-N (Table 5). The variation among proportions of GCA-female and GCA-male effects was not significant for all traits under drought, low-N and optimal conditions with the exception of stay-green characteristic, which showed significantly greater (P < 0.05) GCA-female effect relative to GCA-male effect under drought, and ears per plant, which recorded significantly (P < 0.05) larger GCA-male effect over that of female effect under optimal conditions. The proportionate contributions of GCA-male and female effects did not significantly vary among the hybrids for all traits across environments apart from anthesis–silking interval and stay-green characteristic, which recorded significantly (P < 0.05) greater GCA-female effects than GCA-male effects.
Table 5. Proportion of total genotypic sum of squares of grain yield and other agronomic traits of early maturing PVA-QPM inbreds attributable to GCA and SCA effects under drought, low-N, optimal and across environments
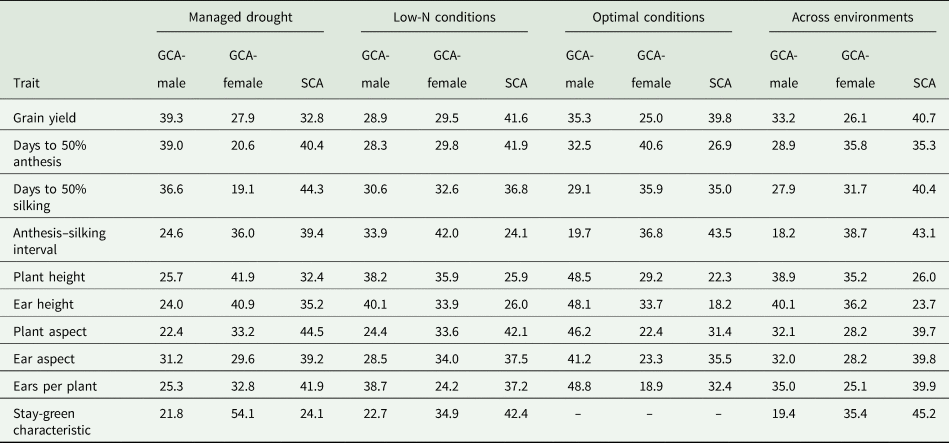
GCA-male, general combining ability effects of inbreds as male parents; GCA-female, general combining ability effects of inbreds as female parents; SCA, specific combining ability effects of inbreds
The GCA effects for PVA and all carotenoids compared to their respective total genotypic sum of squares were greater than SCA effects (Fig. 1). The GCA effects accounted for 87% of the PVA, while the three PVA carotenoids (α-carotene, β-carotene and β-cryptoxanthin) had 83, 81 and 90%, respectively. The variation among GCA-male and female effects was not significant for PVA and all the carotenoids.

Fig. 1. Proportion of total genotypic sum of squares for carotenoids of early maturing PVA-QPM inbreds attributable to GCA of male and female (GCA-m and GCA-f) and SCA effects. Carotenoids are abbreviated as Lut, lutein; Zeax, zeaxanthin; β-cryp, β-cryptoxanthin; α-caro, alpha-carotene; β-caro, β-carotene; PVA, provitamin A; Tcaro, total carotenoids.
General combining ability effects of major agronomic traits and carotenoids of selected inbred lines
Under drought conditions, TZEIORQ 29 was the only inbred which had significant (P < 0.05 and P < 0.01) and positive GCA-male and female effects for grain yield (Table 6). Also, two inbred lines, TZEIORQ 13 and TZEIORQ 24 had significant (P < 0.05 and P < 0.01) and positive GCA-male effects for grain yield, while TZEIORQ 6 recorded significant (P < 0.05) and positive GCA-female effects for grain yield. In addition, TZEIORQ 13 had significant (P < 0.05) negative GCA-male effect for anthesis–silking interval.
Table 6. GCA effects of grain yield of early PVA-QPM inbreds evaluated under drought, low-N, optimal and across environments as well as ASI and stay-green characteristic under stress for one years in Nigeria

ASI, anthesis–silking interval; STGR, stay-green characteristic (1–9); GCA-m, general combining ability effects of inbred as a male parent; GCA-f, general combining ability effects of inbred as a female parent.
Under the low-N environment, the inbreds TZEIORQ 11, TZEIORQ 59, TZEQI 82 and TZEIORQ 2 had significant (P < 0.05 or P < 0.01) and positive GCA-male and female effects for grain yield. In contrast, TZEIORQ 13 and TZEIORQ 24 had significant (P < 0.01) and positive GCA-male effects for grain yield, while TZEIORQ 29, TZEIORQ 48 and TZEIORQ 43 recorded significant (P < 0.05 or P < 0.01) and positive GCA-female effects. For stay green characteristic, TZEIORQ 29 and TZEQI 82 had significant (P < 0.05 or P < 0.01) and negative GCA-male and female effects, TZEIORQ 44 and TZEIORQ 24 had significant (P < 0.05) and negative GCA-male effects, while TZEIORQ 70 recorded significant (P < 0.05) and negative GCA-female effects (Table 6). Out of the 24 parental lines, only TZEIORQ 59 and TZEQI 82 had significant (P < 0.05 or P < 0.01) and positive GCA-male and female effects for grain yield under optimal environments. Other inbreds that exhibited significant (P < 0.05 or P < 0.01) and positive GCA-male effects for grain yield were TZEIORQ 11, TZEIORQ 13 and TZEIORQ 2, and TZEIORQ 29 for GCA-female effects.
Across the 12 test environments, five inbred lines were good combiners for grain yield both as males and females as evident in the significant (P < 0.05 or P < 0.01) and positive GCA-male and female effects recorded for the trait. Inbred lines TZEIORQ 13 and TZEIORQ 24 were good combiners for grain yield as male parents whereas TZEIORQ 48, TZEIORQ 43 and TZEIORQ 70 were good combiners for grain yield when used as female parents (Table 6).
Assessment of the combining ability effects on carotenoids under optimal growing conditions revealed TZEIORQ 29 as the only inbred with highly significant (P < 0.01) positive GCA-male and GCA-female effects for PVA, α-carotene, β-carotene and β-cryptoxanthin (Table 7). Additionally, TZEIORQ 29 had significant (P < 0.05 and P < 0.01) and negative GCA-male and female effects for zeaxanthin which is a non-PVA carotenoid. Also, TZEIORQ 13 had significant (P < 0.05) and positive GCA-female effects for PVA and β-carotene. However, significant (P < 0.05) and negative GCA-male and female effects for total carotenoids were detected for TZEIORQ 13 (Table 7).
Table 7. GCA effects of carotenoids (μg/g DW) for 24 selected early PVA-QPM inbred lines under optimal conditions in 2018, Nigeria

GCA-m, GCA effects of inbred as a male parent; GCA-f, GCA effects of inbred as a female parent.
Heterotic groups and inbred testers identified across test environments
The dendrogram constructed for data across the 12 test environments using the HGCAMT method placed the parental lines into four heterotic groups when 50% of the variation among inbreds was explained (Fig. 2). Eight inbred lines TZEIORQ 69, TZEIORQ 6, TZEIORQ 15, TZEIORQ 43, TZEIORQ 47, TZEIORQ 42, TZEIORQ 40 and TZEIORQ 5 constituted heterotic group I while two inbred lines TZEIORQ 7 and TZEIORQ 41 were classified into heterotic group II. It is striking that the five inbred lines, TZEIORQ 29, TZEIORQ 11, TZEIORQ 59, TZEQI 82 and TZEIORQ 2 identified as good combiners for grain yield when used either as male or female parents were placed in the same heterotic group (group IV) while inbred lines TZEIORQ 13 and TZEIORQ 24 with highly significant (P < 0.01) positive GCA-male effects for grain yield were placed in heterotic group III across environments.
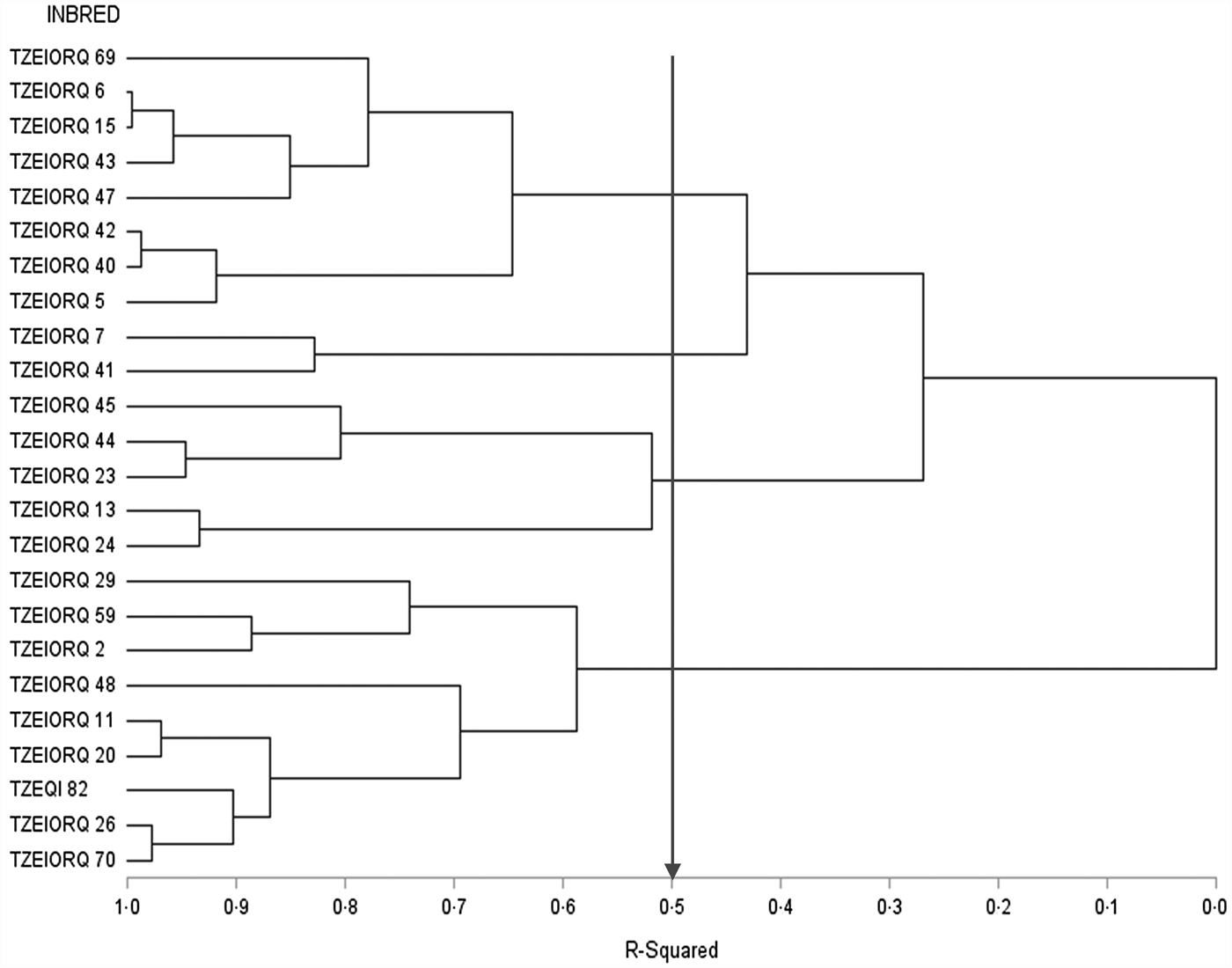
Fig. 2. Dendrogram of 24 early maturing PVA-QPM parental lines constructed from GCA effects of grain yield and other traits (HGCAMT) using Ward's minimum variance cluster analysis method across drought, low-N and optimal environments in Nigeria, 2016–17.
Five inbred lines, TZEIORQ 29, TZEIORQ 11, TZEIORQ 59, TZEQI 82 and TZEIORQ 2 had significant (P < 0.05 or P < 0.01) and positive GCA-male and female effects for grain yield across environments. In addition, the five inbred lines had moderately high to high grain yield across environments. The HGCAMT method classified all the five inbred lines into heterotic group IV (Fig. 2). Based on the combining ability effects of the five inbred lines for carotenoids accumulation, only TZEIORQ 29 obtained highly significant (P < 0.01) and positive GCA-male and GCA-female effects for PVA, β-carotene, α-carotene and β-cryptoxanthin. Therefore, TZEIORQ 29 was identified as the best male and female early maturing PVA-QPM inbred tester for heterotic group IV. No other inbred met the set criteria for the remaining three heterotic groups across the test environments.
Grain yield in contrasting environments, and levels of carotenoids and tryptophan under optimal conditions
Under drought environments, grain yield of the highest yielding PVA-QPM hybrid, TZEIORQ 29 × TZEIORQ 24 significantly (P < 0.01) exceeded the highest yielding drought tolerant commercial hybrid control, TZEI 124 × TZEI 25, by 31% (Table 8). Comparison of the grain yield of hybrids under drought to that of optimal environments revealed a wide yield reduction (5–84%) with a mean of 37%. Under low-N conditions, the highest-ranking hybrid, TZEIORQ 29 × TZEIORQ 43 significantly (P < 0.05) out-yielded the best PVA hybrid control, TZEIOR 127 × TZEIOR 57, by 19%. Comparison of the yield under low-N and that under optimal environments revealed yield reduction varying from 7% for TZEIORQ 29 × TZEIORQ 43 to 80% for TZEIORQ 45 × TZEIORQ 43 with a mean of 33%. The low-N tolerant hybrids recorded lower grain yield reductions than the susceptible hybrids. The performance of the hybrids under optimal conditions revealed the commercial normal yellow endosperm hybrid control, TZEI 124 × TZEI 25 as comparable in yield to the top performing PVA-QPM hybrids including TZEIORQ 40 × TZEIORQ 26, TZEIORQ 23 × TZEIORQ 44, TZEIORQ 26 × TZEIORQ 47 and TZEIORQ 29 × TZEIORQ 44. Across drought, low-N and optimal environments, grain yield of the best performing hybrid (TZEIORQ 40 × TZEIORQ 26) according to the multiple trait base index for combined drought and low-N tolerance was not significantly different from that of the best commercial control (TZEI 124 × TZEI 25). Higher yield reductions were observed among susceptible hybrids across drought, low-N and optimal environments (Table 8).
Table 8. Mean grain yield performance of selected hybrids based on the multiple trait base index across environments, and levels of PVA carotenoids and tryptophan under optimal conditions in Nigeria

Carotenoids: β-cryp, β-cryptoxanthin; α-caro, alpha-carotene; β-caro, β-carotene; PVA, provitamin A; Across, across environments; MI, multiple trait base index across environments.
Generally, the α-carotene contents of the hybrids were very low compared with the levels of the other carotenoids. PVA levels of the hybrids varied from 3.66 µg/g for TZEIORQ 20 × TZEIORQ 48 to 9.82 µg/g for TZEIORQ 29 × TZEIORQ 40 with a mean of 6.19 µg/g. The best-performing hybrid across test environments, TZEIORQ 40 × TZEIORQ 26, had PVA content of 6.90 µg/g. Tryptophan contents varied from 0.08% for TZEIORQ 20 × TZEIORQ 45 to 0.20% for ‘Obatanpa’ with a mean of 0.12%. Obatanpa was 29% higher in tryptophan content than TZEIORQ 40 × TZEIORQ 26, which was second to 'Obatanpa' in terms of tryptophan levels. The threshold of tryptophan content for a QPM genotype is 0.075% in sample in whole grain. All the hybrids had >0.075% tryptophan in sample in whole grain, suggesting that the hybrids met the quality standards of QPM genotypes. Grain yield correlated significantly and positively with PVA (r = 0.40, P < 0.01), α-carotene (r = 0.31, P < 0.05), β-cryptoxanthin (r = 0.38, P < 0.01) and β-carotene (r = 0.33, P < 0.05) but not with total carotenoids, lutein, zeaxanthin and tryptophan (Table 9).
Table 9. Phenotypic correlation among traits of selected PVA-QPM hybrids under optimal environments in Nigeria, 2018

PVA, provitamin A; β-cryp, β-cryptoxanthin; α-caro, alpha-carotene; β-caro, β-carotene; Tcaro, total carotenoid; Lut, lutein; Zeax, zeaxanthin; Tryp, tryptophan; GY, grain yield across drought, low-N and optimal environments.
Grain yield stability of hybrids across test environments
Due to the significant differences observed for G × E interactions for grain yield under each and across environments, the GGE biplot procedure was employed to investigate the G × E interactions and to examine yield stability across environments. The first and the second principal component axes (PC1 and PC2) showed 77.7% of the variation in grain yield (Figs 3 and 4).

Fig. 3. (Colour online) A ‘which-won-where’ genotype plus G × E interaction biplot of grain yield of 25 (best 15 and worst 10 based on the combined drought and low-N base index) selected early maturing PVA-QPM hybrids plus four controls evaluated across drought, low-N and optimal environments from 2016 to 2018 in Nigeria.
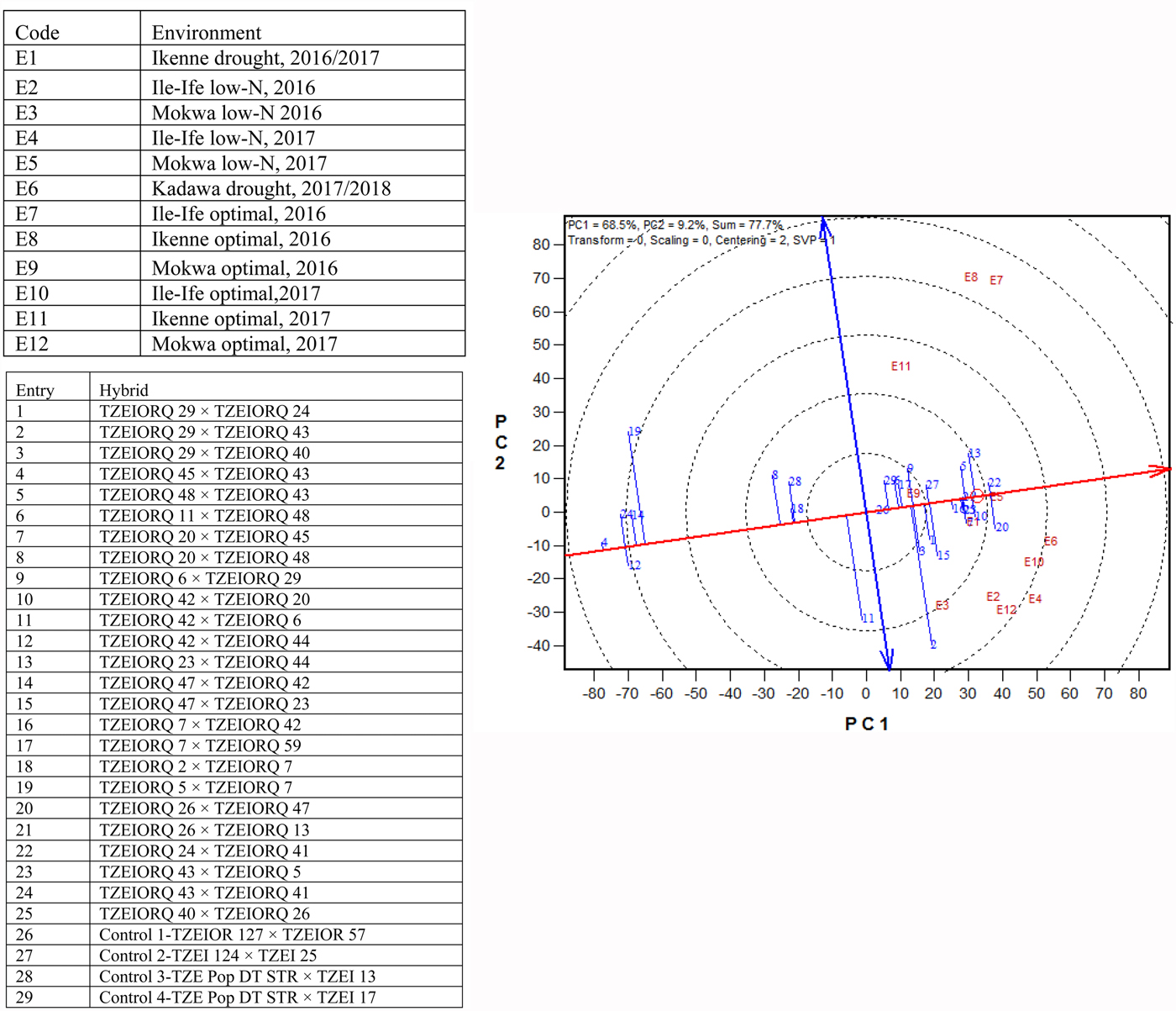
Fig. 4. (Colour online) An entry/tester genotype main effect plus G × E biplot of grain yield for 25 (best 15 and worst 10 based on the combined drought and low-N base index) selected early maturing PVA-QPM hybrids plus four controls evaluated across drought, low-N and optimal environments from 2016 to 2018 in Nigeria.
The polygon view (which-won-where) of the biplot identified location-specific hybrids, with the vertex entries in a sector representing the highest-yielding genotypes in the environments that fell within the sector (Fig. 3). Thus, the vertex genotypes in each sector were more responsive to their locations than those within the polygon and located close to the biplot origin. The polygon view displayed seven sectors with entries 2, 20, 22, 13, 19, 4 and 12 representing the vertex hybrids. Three environments, E2, E3 and E12 fell within the sector which had entry 2 (TZEIORQ 29 × TZEIORQ 43) as the vertex hybrid, implying that it was the ideal hybrid in terms of yield for those environments. Similarly, five environments E1, E4, E5, E6 and E10 were in the sector where entry 20 (TZEIORQ 26 × TZEIORQ 47) was the vertex hybrid and therefore was the highest yielding hybrid in those environments. Two vertex hybrids, entry 22 (TZEIORQ 24 × TZEIORQ 41) and entry 13 (TZEIORQ 23 × TZEIORQ 44) were placed in environment E9 and were therefore the highest-yielding hybrids in that environment. Environments E7, E8 and E11 did not have any vertex hybrids and therefore the highest yielding hybrids could not be identified in those environments. Entries 4, 12 and 19 were vertex hybrids but they were not identified with any of the environments used. The control, entry 26 (TZEIOR 127 × TZEIOR 57), was the least responsive genotype to environmental variability.
The ‘mean performance v. stability’ GGE biplot view was used to identify the highest yielding and most stable hybrids across the 12 test environments. In the biplot (Fig. 4), the average tester coordinate separated hybrids that had means above the grand mean of all hybrids (to the right side of the line) from those with means below the grand mean (to the left side of the line). The yield performance of the hybrids was measured by the imaginary projections from the positions of the hybrids onto the vertical axis, while stability of the hybrids was determined by their projections onto the horizontal axis. Hybrids positioned far from the vertical axis in the right direction were the higher-yielding genotypes, while those with shorter projections on to the horizontal axis were the most stable genotypes. Based on this interpretation, the entry/tester GGE biplot identified entries 22 (TZEIORQ 24 × TZEIORQ 41) and 20 (TZEIORQ 26 × TZEIORQ 47) as the highest-yielding hybrids with TZEIORQ 24 × TZEIORQ 41 as the most stable across research environments. These two hybrids were apparently among the top six high-yielding hybrids identified by the multiple trait base index for combined drought and low-N tolerance. The highest yielding and most stable hybrid, TZEIORQ 24 × TZEIORQ 41, out-yielded the best commercial hybrid control, entry 27 (TZEI 124 × TZEI 25), by 4% across test environments.
Discussion
The significant mean squares of hybrid and environment observed for grain yield and most of the measured traits under low-N, drought, optimal and across environments indicated the presence of genetic variation among the hybrids (Badu-Apraku and Oyekunle, Reference Badu-Apraku and Oyekunle2012) and that the test environments were unique and could detect genetic differences among the hybrids. The significant mean squares of G × E interaction observed for the important agronomic traits including grain yield under each and across test environments suggested that environmental differences influenced the expression of the measured traits. This result substantiated the need to conduct genotype evaluations across multiple environments (Najafian et al., Reference Najafian, Kaffashi and Jafar-Nezhad2010; Zali et al., Reference Zali, Farshadfar and Sabaghpour2011; Badu-Apraku et al., Reference Badu-Apraku, Fakorede, Oyekunle and Akinwale2011a, Reference Badu-Apraku, Fontem, Akinwale and Oyekunle2011b) and to employ one of the available statistical tools for assessing yield performance and stability of genotypes (Yan et al., Reference Yan, Hunt, Sheng and Szlavnics2000). The hybrid × research condition interaction mean squares were not significant for the measured traits including grain yield across environments, implying that there was consistency in the ranking of the hybrids across research conditions and that hybrid performance in the varying environments was influenced significantly by their genetic potential. This result also implied that selection for a hybrid that performed well across drought or low-N environments will not carry a yield penalty under optimal conditions, as reported by Bolaños and Edmeades (Reference Bolaños and Edmeades1993).
The significant mean squares observed for all carotenoids and tryptophan indicated high genetic variation among the hybrids to allow genetic gains from selection. This agreed with earlier reports that there was adequate genetic variability in carotenoids within yellow maize germplasm in the tropics (Menkir et al., Reference Menkir, Liu, White, Maziya-Dixon and Rocheford2008; Suwarno et al., Reference Suwarno, Pixley, Palacios-Rojas, Kaeppler and Babu2015). The preponderance of GCA (male + female) effects over SCA for grain yield and most measured agronomic traits under the contrasting environments indicated that additive gene effect was greater than the non-additive and that GCA largely controlled the inheritance of the traits measured for the 96 early PVA-QPM hybrids. This result suggested that superior hybrids could be produced from crosses between parents with significant and positive GCA effects (Badu-Apraku et al., Reference Badu-Apraku, Oyekunle, Fakorede, Vroh, Akinwale and Aderounmu2013). Several authors have reported similar results under drought (Betrán et al., Reference Betrán, Beck, Bänziger and Edmeades2003; Badu-Apraku et al., Reference Badu-Apraku, Fakorede, Menkir, Kamara and Adam2004; Hallauer et al., Reference Hallauer, Carena and Miranda-Filho2010; Adebayo et al., Reference Adebayo, Menkir, Blay, Gracen, Danquah and Hearne2014; Oyekunle and Badu-Apraku, Reference Oyekunle and Badu-Apraku2014; Annor and Badu-Apraku, Reference Annor and Badu-Apraku2016) and under low-N (Lafitte and Edmeades, Reference Lafitte and Edmeades1995; Kling et al., Reference Kling, Oikeh, Akintoye, Heuberger, Horst, Edmeades, Bänzinger, Mickelson and Peña-Valdivia1997; Ifie et al., Reference Ifie, Badu-Apraku, Gracen and Danquah2015). Additionally, the relative importance of variances of GCA effects over the variances of SCA effects may be due to the initial subjection of the inbred lines used in the study to low-N and drought conditions, as reported by Makumbi et al. (Reference Makumbi, Betrán, Bänziger and Ribaut2011). However, this result is not consistent with the findings of other studies where the inheritance of grain yield and most agronomic traits of maize hybrids were influenced by non-additive genetic factors under drought (Meseka et al., Reference Meseka, Menkir, Ibrahim and Ajala2013), under low-N (Betrán et al., Reference Betrán, Beck, Bänziger and Edmeades2003; Meseka et al., Reference Meseka, Menkir, Ibrahim and Ajala2006; Makumbi et al., Reference Makumbi, Betrán, Bänziger and Ribaut2011) and under optimal conditions (Fan et al., Reference Fan, Zhang, Yao, Bi, Liu, Chen and Kang2014). The inconsistencies in the type of gene action identified by the different authors may be due to the variations in the inbred lines studied, the level of inbreeding and the intensity of drought or low-N imposed. Another implication of the superiority of GCA over SCA is that recurrent selection in a population formed by inter-crossing the set of inbred lines that form the hybrids could lead to increase in the frequency of the beneficial alleles (Sprague and Tatum, Reference Sprague and Tatum1942; Iglesias, Reference Iglesias1989; Topal et al., Reference Topal, Aydin, Akgün and Babaoglu2004; Chigeza et al., Reference Chigeza, Mashingaidze and Shanahan2014).
The significant GCA (male + female) effects for PVA and other carotenoids, as well as SCA effects for most carotenoids suggested that additive and non-additive genetic factors were equally important in the inheritance of these traits. However, the preponderance of GCA effects over SCA for the PVA and the other carotenoids indicated that GCA was the major contributor to the heritable variation in PVA carotenoids of the hybrids. This finding agreed with reports of other studies (Egesel et al., Reference Egesel, Wong, Lambert and Rocheford2003; Suwarno, Reference Suwarno2012; Menkir et al., Reference Menkir, Gedil, Tanumihardjo, Adepoju and Bossey2014; Owens et al., Reference Owens, Lipka, Magallanes-Lundback, Tiede, Diepenbrock, Kandianis, Kim, Cepela, Mateos-Hernandez, Buell, Buckler, DellaPenna, Gore and Rocheford2014; Suwarno et al., Reference Suwarno, Pixley, Palacios-Rojas, Kaeppler and Babu2015), which revealed that accumulation of PVA carotenoids in maize endosperm is largely influenced by additive genetic effects. This observation suggested that favourable alleles of PVA could be pyramided in the inbreds to produce outstanding hybrids and synthetics (Menkir et al., Reference Menkir, Maziya-Dixon, Mengesha, Rocheford and Alamu2017). The preponderance of GCA effects compared to SCA effects indicated that early generation testing for high PVA using the set of 24 inbred lines would be effective (Fasahat et al., Reference Fasahat, Rajabi, Rad and Derera2016). The result also implied that the adoption of recurrent selection methods for the improvement of PVA content would be successful (Sprague and Tatum, Reference Sprague and Tatum1942; Coors, Reference Coors, Coors and Pandey1999). This finding, however, contradicted the report by Halilu et al. (Reference Halilu, Ado, Aba and Usman2016), who found non-additive genetic variance to be higher than the additive for all the carotenoids measured, including PVA.
The non-significant variations observed among the contributions of GCA-male and GCA-female for the traits measured including grain yield under the contrasting environments implied that maternal and paternal effects were equally important in the inheritance of the traits measured for the hybrids. This result supported the findings of Annor and Badu-Apraku (Reference Annor and Badu-Apraku2016), who found equal contributions of the GCA-male and GCA-female sum of squares for grain yield and other measured traits under drought. Similar result was reported by Derera et al. (Reference Derera, Tongoona, Vivek and Laing2008) and Khehra and Bhalla (Reference Khehra and Bhalla1976) under optimal conditions. These results, however, are inconsistent with those of Derera et al. (Reference Derera, Tongoona, Vivek and Laing2008) who reported that cytoplasmic effect influenced grain yield, prolificacy, anthesis–silking interval and ear aspect under drought, as well as anthesis–silking interval, ears per plant and ear aspect under both drought and optimal conditions. Similar inconsistent results were reported by Adebayo et al. (Reference Adebayo, Menkir, Blay, Gracen, Danquah and Hearne2014), who found that paternal effects modified the inheritance of ear aspect under drought. The significantly greater GCA-female effects relative to GCA-male effects recorded for stay-green characteristic under drought and across environments implied that inbred lines with significant negative GCA-female effects for stay-green characteristic should be used as females in their crosses to take maximum advantage of the effects of cytoplasmic inheritance on leaf senescence in their progenies under drought. Also, the significantly large GCA-female effects of anthesis–silking interval relative to that of GCA-male across research environments suggested that maternal effects controlled the inheritance of anthesis–silking interval in this set of inbred lines and that inbred lines with significant negative GCA-female effects for anthesis–silking interval should be used as females in their crosses to contribute to reduced anthesis and silking days in their offspring across the three contrasting environments. It was, however, surprising to find non-significant contributions of GCA-male and GCA-female effects for PVA and its component carotenoids, implying that cytoplasmic genes did not influence the inheritance of these traits in the PVA-QPM inbred lines used.
GCA effect or additive gene effect of inbred lines for a trait is useful in determining the contributions of the parental lines to their hybrids. For example, inbred lines which have highly significant positive GCA effects for grain yield under drought or low-N have high probability of contributing favourable alleles for grain yield to the progenies in a recurrent selection programme to develop drought and/or low-N tolerant populations (Iglesias, Reference Iglesias1989; Topal et al., Reference Topal, Aydin, Akgün and Babaoglu2004; Chigeza et al., Reference Chigeza, Mashingaidze and Shanahan2014). Additionally, such inbreds could be used to improve existing populations and to develop drought and/or low-N hybrid and synthetic varieties for commercialization. The observed significant positive GCA-male and GCA-female effects for grain yield for inbred lines TZEIORQ 29, TZEIORQ 11, TZEIORQ 59, TZEQI 82 and TZEIORQ 2 across drought, low-N and optimal environments suggested that these inbred lines would contribute favourable alleles for improved grain yield to their progenies when used as parental males or females. Similarly, the significant and positive GCA-female effects of grain yield observed for inbreds TZEIORQ 48, TZEIORQ 43 and TZEIORQ 70 across test environments indicated their high potentials to contribute favourable alleles for grain yield to their offspring as female parents. A similar inference could also be drawn for TZEIORQ 13 and TZEIORQ 24 as male parents since they displayed significant and positive GCA-male effects for grain yield across environments. In addition, the significant and negative GCA-male and female effects of stay green characteristic displayed by inbreds TZEIORQ 29, TZEIORQ 59 and TZEQI 82 across the test environments was an indication that these inbreds, when used either as males or females, would contribute to the offspring, desirable alleles for delayed senescence, increased photosynthesis and hence increased assimilate production.
The significant and positive GCA-male and female effects observed for the inbred TZEIORQ 29 for PVA and its component carotenoids suggested that it contributed to increased levels of these carotenoids in its crosses either as a male or a female. The inbred could therefore be exploited for PVA favourable alleles in the development of superior hybrids and synthetics and for the improvement of the early maturing PVA-QPM inbred lines. The significant positive GCA-female effect recorded for TZEIORQ 13 for PVA and β-carotene indicated that it could be used as a female parent to make gains in PVA and β-carotene levels of its hybrids. However, the significant negative GCA-male and GCA-female effects for total carotenoids detected for TZEIORQ 13 implied that it could contribute to reduced total carotenoids in its hybrids when used as a female parent. This result could be a disadvantage for TZEIORQ 13 because high levels of total carotenoids could be an advantage if the influx of total carotenoids favours the accumulation of PVA carotenoids in the carotenoid biosynthetic pathway.
Classification of the newly developed early maturing PVA-QPM inbreds into appropriate heterotic groups is important for the exploitation of maximum heterosis through crossing of inbreds from opposing heterotic groups (Terron et al., Reference Terron, Preciado, Cordova, Mickelson and Lopez1997). The four heterotic groups identified by the HGCAMT method across environments would increase the chances of developing novel and superior early maturing PVA-QPM hybrids and synthetics with combined drought and low-N tolerance for commercialization in SSA. Also, heterotic populations could be constituted by recombining inbred lines from the same heterotic group and improve the population through recurrent selection. The identification of TZEIORQ 29 as the best male and female inbred tester for heterotic group IV implied that the inbred could be used either as a male or a female parent to classify the other lines into heterotic groups and to develop high-yielding PVA-QPM hybrids and synthetics. It was, therefore, not surprising to find TZEIORQ 29 × TZEIORQ 24 as the best hybrid under drought, and among the top performing hybrids across drought, low-N and optimal environments in Nigeria.
Assessment of the 96 early PVA-QPM single cross hybrids plus four controls under drought, low-N and optimal conditions was necessary to identify high-yielding hybrids under each and more importantly, across the three research environments. The average grain yield reduction (37%) recorded under drought at flowering was within the reported range of 40–90% (Grant et al., Reference Grant, Jackson, Kiniry and Arkin1989; Menkir and Akintunde, Reference Menkir and Akintunde2001; Badu-Apraku et al., Reference Badu-Apraku, Fakorede, Menkir, Kamara and Dapaah2005; Derera et al., Reference Derera, Tongoona, Vivek and Laing2008; Badu-Apraku et al., Reference Badu-Apraku, Fontem, Akinwale and Oyekunle2011b). This result indicated that the induced drought at flowering was severe enough to effectively discriminate between the drought-tolerant and susceptible hybrids, thus facilitating the identification and selection of outstanding hybrids. Applying a selection intensity of 10%, the drought base index which involves increased grain yield and prolificacy with short anthesis–silking interval and delayed leaf senescence, as well as excellent plant and ear aspects under drought (Badu-Apraku et al., Reference Badu-Apraku, Fakorede, Oyekunle and Akinwale2011a) identified the top performers of which TZEIORQ 29 × TZEIORQ 24 was the highest yielding hybrid. TZEIORQ 29 × TZEIORQ 24 yielded better than the best PVA commercial hybrid control (TZEI 124 × TZEI 25) by 31%. TZEI 124 × TZEI 25 is an orange endosperm single-cross hybrid that has been released in Ghana as ‘CSIR-Denbea’, in Mali as ‘Tamalaka’ and in Nigeria as ‘Sammaz 41’. This result indicated that TZEIORQ 29 × TZEIORQ 24 would not only be useful in enhancing maize production and productivity in drought prone areas of SSA, but also consumption of this hybrid would help address the health disorders emanating from vitamin A deficiency and protein energy malnutrition (Bressani, Reference Bressani and Mertz1992; Badu-Apraku and Fontem Lum, Reference Badu-Apraku and Fontem Lum2010; Pixley et al., Reference Pixley, Rojas, Babu, Mutale, Surles, Simpungwe and Tanumihardjo2013).
Under low-N conditions, the 33% average grain yield reduction recorded was within the ranges of 10–50% and 20–50% reported by Wolfe et al. (Reference Wolfe, Henderson, Hsiao and Alvio1988) and Bänziger et al. (Reference Bänziger, Edmeades and Lafitte1999), respectively. This result indicated that the low-N condition imposed was adequate to effectively differentiate between low-N tolerant and susceptible hybrids. With a selection intensity of 10%, the low-N base index (Badu-Apraku et al., Reference Badu-Apraku, Fakorede, Oyekunle and Akinwale2011a) was effectively used to identify TZEIORQ 29 × TZEIORQ 43 as the best hybrid which out-yielded the best control, TZEIOR 127 × TZEIOR 57, by 25% under low-N conditions. The best control under low-N is a PVA non-QPM single cross hybrid identified as promising in previous studies and promoted to the regional trials of the IITA-MIP (Konaté et al., Reference Konaté, Badu-Apraku and Traoré2017). This result, therefore, implied that the top-performing early PVA-QPM hybrid would be crucial in enhancing maize production under low-N environments in the region. The result has also highlighted the enormous progress made in the development of the early maturing PVA-QPM hybrids for low-N tolerance.
Combined assessment of grain yield performance using the multiple trait base index across drought, low-N and optimal environments revealed a set of ten top performing hybrids of which TZEIORQ 40 × TZEIORQ 26 was the most outstanding. The top performers identified across environments were essentially among the best performers under each environment including optimal conditions. This implied that the best hybrids selected across the three research conditions would consistently display superior grain yield performance across seasons and in varying production environments in SSA without yield penalties. Although the grain yield performance of the top ten PVA-QPM hybrids was statistically the same as the best commercial orange endosperm hybrid control, TZEI 124 × TZEI 25 under the contrasting environments, the identified hybrids have the extra advantage of elevated levels of PVA carotenoids as well as lysine and tryptophan.
The range of PVA concentrations (6.50–9.82 µg/g DW) recorded for the top 15 hybrids in the current study was moderate compared with the 15 µg/g DW target set by the Harvest-Plus challenge programme (Harjes et al., Reference Harjes, Rocheford, Bai, Brutnell, Kandianis, Sowinski, Stapleton, Vallabhaneni, Williams, Wurtzel, Yan and Buckler2008; Simpungwe et al., Reference Simpungwe, Dhliwayo, Palenberg, Taleon, Birol, Oparinde, Saltzman and Diressie2017). The PVA concentrations observed for the hybrids in the current study corroborated with the report by Andersson et al. (Reference Andersson, Saltzman, Virk and Pfeiffer2017), who indicated that although some progress has been made with respect to the improvement of PVA carotenoids in developed maize varieties in SSA, with over 40 varieties released, the PVA levels of these varieties range between 6 and 10 µg/g. This result suggested that there is the need to introgress favourable PVA alleles from other sources to improve the tropically adapted early PVA-QPM inbred lines. Fortunately, apart from the early PVA-QPM inbred line TZEIORQ 55 (15.1 µg/g) identified in the current study, extra-early PVA inbred lines TZEEIOR 202 (23.98 µg/g) and TZEEIOR 205 (22.58 µg/g), with PVA levels exceeding the 15 µg/g target by 50.4 and 59.9%, respectively, have been identified in the IITA-MIP. The extra-early maturing PVA inbreds have resulted in the development of PVA hybrids TZEEIOR 197 × TZEEIOR 205 (20.1 µg/g) and TZEEIOR 202 × TZEEIOR 205 (22.7 µg/g), containing about double the amount of PVA of the commercial PVA hybrid control, TZEE-Y Pop STR C5 × TZEEI 58 (11.4 µg/g), which are in the pipeline for release in SSA (Badu-Apraku et al., Reference Badu-Apraku, Talabi, Garcia-Oliveira and Gedil2018). These new sources of PVA beneficial alleles should help to facilitate the accumulation of PVA carotenoid concentrations in the existing early PVA-QPM hybrids and to even exceed the set target (15 µg/g DW). Exceeding the PVA set target is a practically feasible approach to maximize the benefits of consumers of PVA maize because studies have revealed that the amount of PVA lost during storage, milling and preparation of different local food items could be about 70% (Mugode et al., Reference Mugode, Ha, Kaunda, Sikombe, Phiri, Mutale, Davis, Tanumihardjo and De Moura2014; Pillay et al., Reference Pillay, Siwela, Derera and Veldman2014; De Moura et al., Reference De Moura, Miloff and Boy2015) and the degree of loss widely varies among maize genotypes. The range of values of tryptophan content (>0.075 in whole grain sample) recorded for the hybrids indicated that all the hybrids met the quality standards of a QPM genotype (Vivek et al., Reference Vivek, Krivanek, Palacios-Rojas, Twumasi-Afriyie and Diallo2008; Teklewold et al., Reference Teklewold, Wegary, Tadesse, Tadesse, Bantte, Friesen and Prasanna2015). The significant and positive correlation observed between PVA and grain yield indicated that improving the synthesis and accumulation of PVA and other carotenoids simultaneously may be effectively accomplished without reducing the grain yield potential and related important agronomic traits (Bouis and Welch, Reference Bouis and Welch2010; Menkir et al., Reference Menkir, Gedil, Tanumihardjo, Adepoju and Bossey2014). This finding contrasts with the results of Halilu et al. (Reference Halilu, Ado, Aba and Usman2016), who found non-significant correlations among grain yield and measured carotenoid concentrations, and indicated that the traits can be improved independently.
An important objective in the current study was to identify high and stable yielding hybrids across drought, low-N and optimal environments for further testing and commercialization. Significant G × E interactions are advantageous when the objective is to develop location specific varieties characterized by narrow adaptation. However, it is a disadvantage when developing varieties for broad adaptation (Badu-Apraku and Fakorede, Reference Badu-Apraku, Fakorede, Badu-Apraku and Fakorede2017). The observed significant genotype × environment interaction mean squares for grain yield and most other measured traits under drought, low-N, optimal, and across environments indicated that the expression of the traits varied with environments. This observation, therefore, warranted the use of the ‘which-won-where’ and the ‘mean performance v. stability’ GGE biplot views to identify hybrids with location-specific and broad adaptations, respectively. From the ‘which-won-where’ view of the GGE biplot, the first and the second principal component axes (PC1 and PC2) explained 77.7% of the variation in grain yield of the hybrids indicating that PC1 and PC2 sufficiently approximated the environment-centred data. Hybrids with broad adaptation would be most preferred by farmers, especially in SSA because of the significant variation in seasons and production environments. However, in a few instances, hybrids with location-specific adaptation may be necessary. Hybrid, TZEIORQ 29 × TZEIORQ 43 (entry 2) was the highest yielding in environments E2 (2016 low-N, Ile-Ife), E3 (2016 low-N, Mokwa) and E12 (2017 optimal, Mokwa). This result suggested that TZEIORQ 29 × TZEIORQ 43 would display superior performance in nitrogen deficient environments at Ile-Ife and Mokwa without compromising yield under optimal environments at Mokwa. Also, E2, E3 and E12 could constitute a mega-environment because they shared the same winning hybrid. However, several years of testing in these three locations would be needed to ascertain whether they are indeed mega-environments or not. Yan et al. (Reference Yan, Hunt, Sheng and Szlavnics2000, Reference Yan, Kang, Ma, Woods and Cornelius2007, Reference Yan, Kandianis, Harjes, Bai, Kim, Yang, Skinner, Fu, Mitchell, Li, Fernandez, Zaharieva, Babu, Fu, Palacios, Li, DellaPenna, Brutnell, Buckler, Warburton and Rocheford2010) found that selection of a mega-environment identified from location-groups depends on the consistency of the location groupings as well as the winning genotypes across years. Hybrid TZEIORQ 26 × TZEIORQ 47 (entry 20) was the highest yielding in environments E1 (2016 drought, Ikenne), E4 (2017 low-N, Ile-Ife), E5 (2017 low-N, Mokwa), E6 (2018 combined heat and drought, Kadawa) and E10 (2017 optimal, Ile-Ife). This result indicated that TZEIORQ 26 × TZEIORQ 47 would be high-yielding across drought and low-N environments at Ikenne, Kadawa, Ile-Ife and Mokwa with an acceptable performance under optimal conditions at Ile-Ife. What is also interesting with this result is that TZEIORQ 26 × TZEIORQ 47 would be the preferred hybrid under combined heat and drought stress at Kadawa located in the semi-arid/Sudan Savanna agro-ecology of Nigeria. Additionally, TZEIORQ 24 × TZEIORQ 41 (entry 22) and TZEIORQ 23 × TZEIORQ 44 (entry 13) were the highest-yielding hybrids only in environment E9 (2017 optimal, Mokwa), suggesting that these two hybrids were the most promising for the non-stressful environment at Mokwa. Moreover, the ‘mean performance v. stability’ GGE biplot view identified TZEIORQ 24 × TZEIORQ 41 (entry 22) and TZEIORQ 26 × TZEIORQ 47 (entry 20) as the best yielding single cross hybrids across the 12 research environments. Of the two hybrids, TZEIORQ 24 × TZEIORQ 41 was the most stable across the test environments and should be extensively tested on-farm for consistent performance and commercialization in SSA.
In conclusion, additive genetic factors were more significant than non-additive for grain yield, most of the measured agronomic traits under the contrasting environments and for PVA carotenoids under optimal conditions. The HGCAMT method revealed four heterotic groups for the inbreds based primarily on pedigree, with TZEIORQ 29 emerging as the best male and female tester for heterotic group IV. The hybrids TZEIORQ 29 × TZEIORQ 43, TZEIORQ 29 × TZEIORQ 40, TZEIORQ 29 × TZEIORQ 24 and TZEIORQ 6 × TZEIORQ 29 combined high-yielding ability with moderately high PVA levels and should be tested extensively for release and commercialization in SSA to reduce vitamin-A and protein deficiencies in the sub-region. The best-yielding and most adapted hybrid TZEIORQ 24 × TZEIORQ 41 should be further tested on-farm to confirm the consistency of performance under the contrasting environments for commercialization in SSA.
Acknowledgements
The authors are grateful to the staff of the IITA Maize Improvement Programme and the Food and Nutrition laboratory of IITA in Ibadan, Nigeria, for technical assistance. Finally, the authors are grateful to Abidemi Olutayo Talabi, Mustapha Abu Dadzie and Noudifoulè Tchala for their diverse contributions to this research.
Financial support
This research received financial support from the USAID through the West Africa Centre for Crop Improvement (WACCI), and the Bill and Melinda Gates Foundation through the DTMA/STMA Projects.
Conflict of interest
The authors hereby declare that the study was carried out without any financial and/or commercial relationship that could result in a potential conflict of interest.
Ethical standards
Not applicable.