Introduction
Aversive social experiences are reported from a majority of patients with social anxiety disorder (SAD) (Erwin, Heimberg, Marx, & Franklin, Reference Erwin, Heimberg, Marx and Franklin2006; McCabe, Antony, Summerfeldt, Liss, & Swinson, Reference McCabe, Antony, Summerfeldt, Liss and Swinson2003; Norton & Abbott, Reference Norton and Abbott2017) and are identified as a crucial factor in the etiology of the disorder (Mineka & Zinbarg, Reference Mineka and Zinbarg2006; Norton & Abbott, Reference Norton and Abbott2017; Wong & Rapee, Reference Wong and Rapee2016). In response to these etiologically relevant aversive social events, some patients with SAD suffer from posttraumatic stress symptoms (PTSS), such as reexperiencing/intrusive memories (Bjornsson et al., Reference Bjornsson, Hardarson, Valdimarsdottir, Gudmundsdottir, Tryggvadottir, Thorarinsdottir and Thorisdottir2020; Erwin et al., Reference Erwin, Heimberg, Marx and Franklin2006), which are known in the context of post-traumatic stress disorder (PTSD) after traumatic events. Intrusion symptoms after an aversive social experience, for example in the form of negatively distorted self-images, are considered in explanatory models of SAD (Clark & Wells, Reference Clark, Wells, Heimberg, Liebowitz, Hope and Schneier1995; Rapee & Heimberg, Reference Rapee and Heimberg1997), leading to dysfunctional shifts of attention that contribute to the maintenance of the disorder. They also cause the affected person to re-experience features of the original aversive social situation, even in novel social situations (Hackmann, Clark, & McManus, Reference Hackmann, Clark and McManus2000). Obviously, there are some phenomenological similarities between SAD and PTSD like a triggering aversive event and subsequent PTSS, such as intrusions.
Intrusions can be understood as conditioned fear responses (Franke et al., Reference Franke, Rattel, Miedl, Danböck, Bürkner and Wilhelm2021). Altered fear conditioning processes are assumed to underlie the development and maintenance of PTSD and anxiety disorders (Duits et al., Reference Duits, Cath, Lissek, Hox, Hamm, Engelhard and Baas2015; Dvir, Horovitz, Aderka, & Shechner, Reference Dvir, Horovitz, Aderka and Shechner2019; Lissek et al., Reference Lissek, Powers, McClure, Phelps, Woldehawariat, Grillon and Pine2005; Lissek & Grillon, Reference Lissek, Grillon, Beck and Sloan2012; Mineka & Zinbarg, Reference Mineka and Zinbarg2006; Wong & Rapee, Reference Wong and Rapee2016). Laboratory fear conditioning studies in patients with SAD indicate increased fear responses toward the conditioned stimulus and the safety stimulus during fear acquisition, as well as deficient extinction learning, although this is not shown to be consistent across studies or across outcome measures within studies (Ahrens et al., Reference Ahrens, Pauli, Reif, Mühlberger, Langs, Aalderink and Wieser2016; Chauret et al., Reference Chauret, Suffren, Pine, Nassim, Saint-Amour and Maheu2019; Hermann et al., Reference Hermann, Ziegler and Birbaumer2002; Lissek et al., Reference Lissek, Levenson, Biggs, Johnson, Ameli, Pine and Grillon2008; Marin, Hammoud, Klumpp, Simon, & Milad, Reference Marin, Hammoud, Klumpp, Simon and Milad2020; Rabinak et al., Reference Rabinak, Mori, Lyons, Milad and Phan2017; Savage, Davey, Fullana, & Harrison, Reference Savage, Davey, Fullana and Harrison2020; Tinoco-González et al., Reference Tinoco-González, Fullana, Torrents-Rodas, Bonillo, Vervliet, Pailhez and Torrubia2015).
On the neural level, the following brain regions are in particular involved in the modulation of conditioned fear expression via the amygdala: hippocampus (especially context-dependent modulation), insula (cortical representation of fear), ventromedial prefrontal cortex (vmPFC; inhibitory function), and dorsal anterior cingulate cortex (dACC; excitatory function) (Bouton, Westbrook, Corcoran, & Maren, Reference Bouton, Westbrook, Corcoran and Maren2006; Hermann, Stark, Milad, & Merz, Reference Hermann, Stark, Milad and Merz2016; Kalisch et al., Reference Kalisch, Korenfeld, Stephan, Weiskopf, Seymour and Dolan2006; Maren, Phan, & Liberzon, Reference Maren, Phan and Liberzon2013; Phelps et al., Reference Phelps, O'Connor, Gatenby, Gore, Grillon and Davis2001; Sehlmeyer et al., Reference Sehlmeyer, Schöning, Zwitserlood, Pfleiderer, Kircher, Arolt and Konrad2009). These neural processes of fear conditioning are altered in PTSD (Garfinkel et al., Reference Garfinkel, Abelson, King, Sripada, Wang, Gaines and Liberzon2014; Milad et al., Reference Milad, Pitman, Ellis, Gold, Shin, Lasko and Rauch2009; Suarez-Jimenez et al., Reference Suarez-Jimenez, Albajes-Eizagirre, Lazarov, Zhu, Harrison, Radua and Fullana2020; VanElzakker, Staples-Bradley, & Shin, Reference VanElzakker, Staples-Bradley, Shin, Vermetten, Germain and Neylan2018) and anxiety disorders (Chauret et al., Reference Chauret, Suffren, Pine, Nassim, Saint-Amour and Maheu2019; Marin et al., Reference Marin, Zsido, Song, Lasko, Killgore, Rauch and Milad2017). In a previous study, social anxiety in healthy participants was related to greater differential conditioned activation of the amygdala and hippocampus during fear acquisition, but lower activation during extinction learning, as well as a tendency for reduced vmPFC responses during extinction recall (Pejic, Hermann, Vaitl, & Stark, Reference Pejic, Hermann, Vaitl and Stark2013).
In PTSD, return of fear even in safe contexts is of high clinical relevance, probably resulting from inadequate contextualization of the trauma memory (Ehlers & Clark, Reference Ehlers and Clark2000). In line with this, patients with PTSD compared with trauma-exposed controls showed a stronger return of fear and lower activation of the vmPFC and hippocampus, as well as hyperactivity of the dACC during extinction recall in the safe extinction context (Milad et al., Reference Milad, Pitman, Ellis, Gold, Shin, Lasko and Rauch2009). In a further study, reduced vmPFC activation and higher SCRs toward the conditioned stimulus (CS+), and stronger differential activation in the insula (CS+ v. CS−) during extinction recall, but lower activation in the amygdala and vmPFC and less SCRs toward the CS+ during renewal in the acquisition context were found in patients with PTSD compared to combat controls (Garfinkel et al., Reference Garfinkel, Abelson, King, Sripada, Wang, Gaines and Liberzon2014). Moreover, during fear renewal in a novel context, patients with PTSD had higher differential conditioned hippocampal and amygdala activity as well as higher conditioned SCRs compared to healthy controls (HC) (Wicking et al., Reference Wicking, Steiger, Nees, Diener, Grimm, Ruttorf and Flor2016). These results overall point to deficits in context-dependent fear conditioning in patients with PTSD, which may arise due to deficits in using contextual information for the modulation of fear.
There is only one fMRI study investigating context-dependent fear conditioning in patients with SAD (Marin et al., Reference Marin, Hammoud, Klumpp, Simon and Milad2020). They found hypoactivation of the vmPFC during extinction recall in the safe extinction context in SAD compared with HC, but no significant differences in SCRs. However, there are no studies up to date investigating the neural correlates of conditioned fear renewal in a novel or the acquisition context, nor the association between PTSS, such as intrusions and altered fear conditioning processes in SAD.
Therefore, we conducted a two-day context-dependent fear conditioning paradigm with fear acquisition (context A) and extinction learning (context B) on the first day, and extinction recall (context B) and fear renewal in a novel (context C) as well as the acquisition context (context A) approximately 24 h later during functional magnetic resonance imaging (fMRI) in 54 patients with SAD and 54 HC. We expected reduced extinction recall in the safe context (reduced differential conditioned activation in hippocampus and vmPFC, stronger differential conditioned activation in dACC, insula and amygdala and stronger differential conditioned SCRs) and lower fear renewal in the novel and the acquisition context (reduced differential conditioned activation in amygdala, dACC, insula and hippocampus, stronger activation in vmPFC and reduced differential SCRs) in SAD compared to HC. These changes should be particularly evident in patients with intrusion symptoms compared to patients without intrusion symptoms. Moreover, these group differences should not only be reflected in altered activation but also in altered connectivity of fear- and extinction-relevant brain areas.
Methods and materials
Subjects
Recruitment was conducted via the mailing list of the local university, the associated outpatient clinic, posters, flyers, and announcements in newspapers and on websites. In patients, SAD had to be the primary mental disorder; several disorders (e.g. borderline personality disorder, psychosis, PTSD), current substance abuse or use of psychotropic drugs and current psychotherapy were exclusion criteria. HC were required not to have a current or past mental disorder (for details regarding exclusion criteria see online Supplementary Table S1). In the course of the study, there was a dropout of 18 patients with SAD and 12 HC (cancellation without reason [6 SAD/2 HC], scheduling reasons [3 SAD/3 HC], discomfort in scanner [4 SAD/2 HC], technical difficulties [3 SAD/4 HC], strong head movements during scanning [2 SAD/1 HC]). This resulted in a final sample of 54 patients and 54 HC who were matched for age, sex, and number of years of education. Table 1 shows relevant demographic as well as diagnostic information for both groups. An a priori power analysis for the comparison of patients with HC using G*Power (version 3.1.9.2; Faul, Erdfelder, Lang, and Buchner, Reference Faul, Erdfelder, Lang and Buchner2007), determined a group size of at least n = 51 within each group, in order to be able to identify a group difference (t-test) with a mean effect size of d = 0.5 (power (1−β) = 0.8, α = 0.05). The study protocol was approved by the local ethics committee of the Department of Psychology and Sport Science at the Justus Liebig University Giessen and was conducted in accordance with the Declaration of Helsinki. All participants gave written informed consent.
Table 1. Demographic and clinical variables for patients with social anxiety disorder and healthy controls
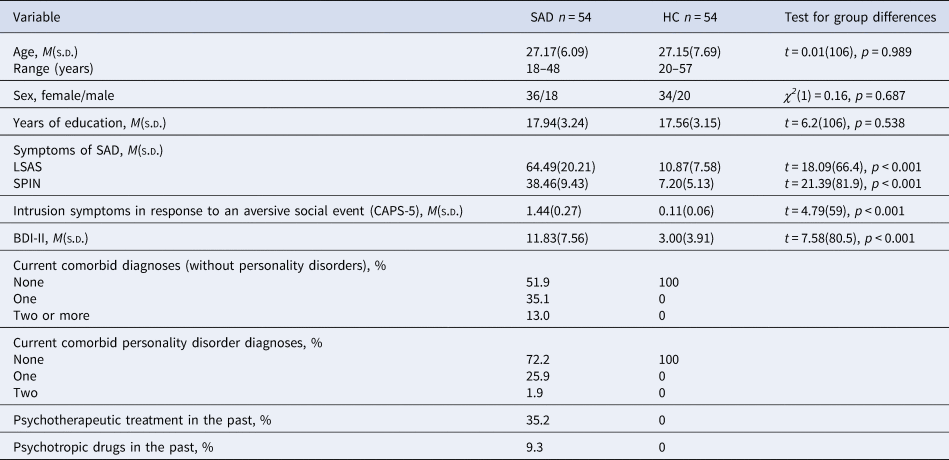
Note. Table 1 shows descriptive variables separated for groups (SAD, social anxiety disorder; HC, healthy controls) and test statistics for group differences. T- test, degrees of freedom (df), χ2-statistics, significance level (p), LSAS, Liebowitz Social Anxiety Scale; SPIN, Social Phobia Inventory; CAPS-5, Clinician-administered PTSD scale; BDI-II, Beck Depression Inventory II, diagnoses were assessed by using the DIPS (Diagnostic Interview for Mental Disorders) and the SKID-II (Structured clinical interview for DSM-IV Axis II: Personality Disorders), CAPS-5 = Clinician-administered PTSD scale, sample size varied for HC: CAPS-5 n = 53, BDI n = 51 and for SAD: LSAS n = 53.
To investigate the relevance of intrusion symptoms for context-dependent fear conditioning processes in SAD, we divided the patients into two groups by using the raw score of criterion B (intrusion symptoms) of the Clinician-administered PTSD scale (CAPS-5; Cwik and Woud, Reference Cwik and Woud2015). As an intrusion score >1 is defined as clinically relevant, patients with clinically relevant intrusions (INT; raw score > 1, n = 19) and patients without intrusions (NO-INT; raw score = 0, n = 29) were grouped together, respectively. We excluded patients with a raw score of 1 (n = 7) for these group comparisons. Table 2 shows relevant demographic as well as diagnostic information for both groups separately as well as group differences between groups.
Table 2. Sociodemographic and clinical variables separated for groups of patients with clinically relevant intrusions in response to the aversive social event and patients without intrusions
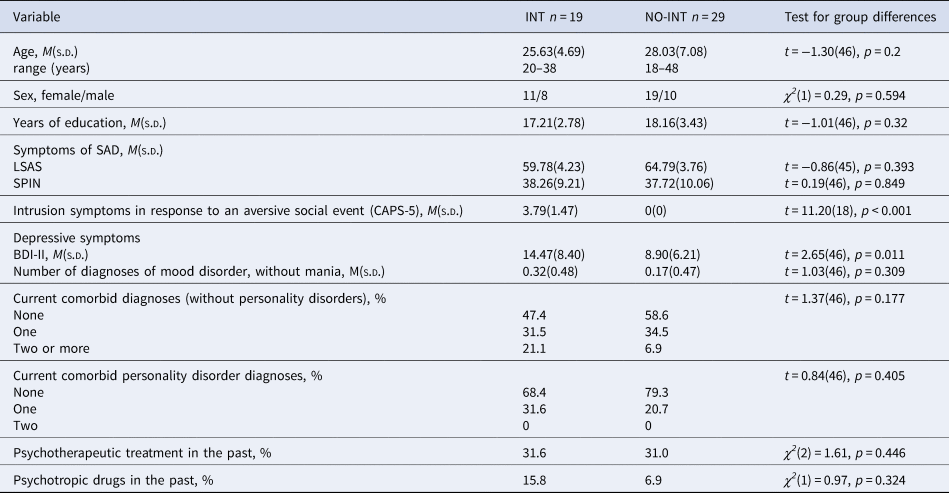
Note. INT, patients with clinically relevant intrusions; NO-INT, patients without intrusions, t test, degrees of freedom (df), χ2-statistics, significance level (p), LSAS, Liebowitz Social Anxiety Scale; SPIN, Social Phobia Inventory; BDI-II, Beck Depression Inventory II, diagnoses were assessed by using the DIPS (Diagnostic Interview for Mental Disorders) and the SKID-II (Structured clinical interview for DSM-IV Axis II: Personality Disorders), CAPS-5 = Clinician-administered PTSD scale, sample size varied for INT: LSAS n = 18.
Interviews and questionnaires
To confirm the inclusion or exclusion criteria of SAD and other diagnoses, two trained psychologists administered the Diagnostic Interview for Mental Disorders (DIPS; Margraf, Cwik, Suppiger, and Schneider, Reference Margraf, Cwik, Suppiger and Schneider2017). The Liebowitz Social Anxiety Scale (LSAS; Consbruch, Stangier, and Heidenreich, Reference Consbruch, Stangier and Heidenreich2016; Fresco et al., Reference Fresco, Coles, Heimberg, Liebowitz, Hami, Stein and Goetz2001; Liebowitz, Reference Liebowitz1987) was used as an interview to assess symptom severity and as an additional criterion for inclusion in the SAD group or exclusion in the HC group (total score ⩾ 30). We additionally used the Social Phobia Inventory (SPIN; Connor et al., Reference Connor, Davidson, Churchill, Sherwood, Foa and Weisler2000; Sosic, Gieler, and Stangier, Reference Sosic, Gieler and Stangier2008) to validate inclusion/exclusion decisions. The total score had to be ⩾25 (SAD) or <25 (HC).
To select an autobiographical event, we used a list of aversive social events, which was adapted from Erwin, Heimberg, Marx & Franklin (Erwin et al., Reference Erwin, Heimberg, Marx and Franklin2006). The subsequent exploration of PTSS was referred to an aversive social event most likely to be associated with the onset of the disorder, or the worst social event participants have ever experienced. Two psychologists assessed associated PTSS (intrusion symptoms, avoidance, alterations in cognitions and mood and alterations in arousal and reactivity) in the past month via the CAPS-5 (Cwik & Woud, Reference Cwik and Woud2015; Spies et al., Reference Spies, Woud, Kessler, Rau, Willmund, Köhler and Cwik2020).
Procedure
This study refers to data acquired within a larger project with six appointments (see online Supplement A). Data reported here were collected on three appointments: first appointment with diagnostic and other interviews and the third and fourth appointment with the fear conditioning paradigm. The fMRI measurements took place within 14 days of the diagnostic interviews and followed each other for approximately 24 h. Participation was compensated with ten euros/hour or course credit.
Context-dependent fear conditioning paradigm
The two-day context-dependent fear conditioning paradigm was conducted in the MRI Scanner. The electrical stimulation, which served as UCS, was delivered via electrodes (Coulbourn Transcuaneous Aversive Finger Stimulator, E13-22, Holliston Massachussets) attached to the fingertips of the index and middle fingers of the dominant hand. The strength of the electrical stimulation was individually adjusted at the beginning of the first MRI session so that it was very uncomfortable for the individual, but not yet painful. The groups did not differ in the intensity of adjusted electrical stimulation (SAD: M = 1.80 mA, s.d. = 0.68; HC: M = 1.77 mA, s.d. = 0.69; t(105) = 0.19, p = 0.79; range in both groups = 0.6–4 mA; INT: M = 1.90 mA, s.d. = 0.60, range = 1.1–4 mA; NO-INT: M = 1.76 mA, s.d. = 0.77; t(45) = 0.66, p = 0.51; range = 0.6–4 mA).
The paradigm is adapted from previous studies (Hermann et al., Reference Hermann, Stark, Milad and Merz2016; Reference Hermann, Stark, Müller, Kruse, Wolf and Merz2020; Milad et al., Reference Milad, Wright, Orr, Pitman, Quirk and Rauch2007). Pictures of different rooms (e.g. conference room) served as contexts. A lamp was placed in each of them and its light colors (yellow, blue) were used as reinforced conditioned (CS+) and non-reinforced (CS−) stimuli. During the first fMRI measurement, fear acquisition took place in context A (8 trials per CS type; 5 trials with UCS presentation), followed by extinction training in context B (16 trials per CS type). Approximately 24 h later, retrieval testing was performed in the safe extinction context B, in a novel context C, and in the acquisition context A (each 8 trials per CS type). Each trial began with the picture of a context with the lamp turned off (3s), which then lighted yellow or blue (6s), followed by electrical stimulation (UCS) in reinforced CS+ trials during fear acquisition. The whole paradigm and trial structure is described in detail in online Supplement B (see also online Supplementary Figure S1).
After the second scanning session, participants filled in a post hoc rating (detailed information in online Supplement C) to assess their knowledge about the different contexts (context recognition), as well as the expected probability for the occurrence of the electrical stimulation (UCS expectancy) in the three different contexts. On 9-point Likert scales, participants were asked to indicate valence, arousal, and fear in each context (turned-off lamp), respectively.
Functional magnetic resonance imaging
We used a 3 Tesla whole body scanner with a 64 channel standard head coil (Siemens Prisma, Erlangen, Germany). A T2*-weighted gradient echo-planar imaging sequence (EPI) with 42 slices (slice thickness = 3 mm; 0.75 mm gap; descending slice order; TE = 30 ms; TR = 2.5 s; flip angle = 81°; Field of View = 220 × 220 mm2; matrix-size = 74 × 74 pixel, parallel imaging: GRAPPA acceleration 2; oblique slices 30 degrees tilt from the AC-PC line) covering the entire brain was used to assess BOLD responses. Three persons (two patients with SAD, one HC) were excluded from fMRI analyses due to a framewise displacement of more than 0.5 mm in at least 20% of the volumes of one scanning session. We used Statistical Parametric Mapping (SPM12, r7219, Wellcome Department of Cognitive Neurology, London, UK; 2009), implemented in Matlab R2019a (Mathworks Inc., Sherborn, MA, USA) for data preprocessing and analysis. After preprocessing and first-level analyses (for details see online Supplement D), the following contrasts were calculated on an individual level: differential conditioned responses (CS+ minus CS−) for acquisition, extinction learning (first 8 compared with/and last 8 trials) and early recall/renewal (first 4 trials), respectively. In order two analyze differences between SAD and HC (both groups n = 54) we conducted two-sample t tests for the experimental phases of the conditioning paradigm (acquisition, early extinction, late extinction, extinction learning, early extinction recall, early fear renewal in the novel context and early fear renewal in the acquisition context) in second-level analyses. The same analyses were done for comparing the two groups of patients with clinically relevant intrusions (INT, n = 19) and without intrusions (NO-INT, n = 29). For the analyses of main effects, one sample t tests for each experimental phase were performed with all participants in one group (n = 108). Region of interest (ROI) analyses were planned a priori for amygdala, insula, hippocampus, dACC and vmPFC. We used the small volume correction option of SPM12 and a significance threshold of α ⩽ 0.05 on voxel level with family-wise error (FWE) correction.The reported coordinates (x, y, z) refer to the MNI space.
To investigate the connectivity between the resulting (significant) voxels and the other fear-relevant brain areas (amygdala, hippocampus, vmPFC, dACC, insula) in the corresponding experimental conditions, psychophysiological interaction (PPI) analyses were performed. For this purpose, the corresponding voxels were used as seed region (volume of interest; 6 mm sphere around the peak voxel), respectively.
Skin conductance responses
We placed Ag/AgCl electrodes filled with isotonic (0.05 M NaCl) electrolyte medium on the hypothenar surface of the non-dominant hand to measure SCRs. Data were preprocessed as well as analyzed using Ledalab 3.4.4 (available under www.ledalab.de), a toolbox integrated in MATLAB R2018B (The MathWorks, Natick, MA, USA). We used the ‘trough-to-peak’ analyses (Pineles, Orr, & Orr, Reference Pineles, Orr and Orr2009). Detailed information is given in online Supplement E. To correct for violation of normal distribution, we log(μS + 1) transformed all responses.
Due to classification as non-responders (less than two SCRs >0.02 μS in reaction to the UCS after CS+ during fear acquisition, n = 6) or technical problems during measurement (n = 13), we had to exclude 19 persons, resulting in a sample of 48 SAD (INT n = 17, NO-INT n = 27) and 44 HC subjects for the SCR analyses (which also were included in fMRI data analyses). Statistical analyses were performed using IBM SPSS 28 (IBM Corporation, Armonk, USA). We conducted nine mixed ANOVAs for each of the two group comparisons, each with the within-subject factor ‘stimulus’ (CS + /CS−) and the between-subject factor ‘group’ (HC and SAD or INT and NO-INT). Analogous to the fMRI analyses, we conducted five ANOVAs for acquisition, ‘late’ extinction, and for the ‘early’ phases of extinction recall in the extinction context, renewal in the novel context, and renewal in the acquisition context, with these two factors. To investigate the decrease during extinction training, and especially the return of the conditioned SCRs between phases, we additionally performed four more ANOVAs. For these ANOVAs on extinction training and return of fear, each involving two phases, we added the within-subjects factor ‘phase’ (first/second half of extinction training; last two trials of the previous phase [subsequently referred to as ‘late’] and the first four trials of the following phase [subsequently referred to as ‘early’]: late extinction training/early extinction recall in the extinction context, late extinction recall/early renewal in the novel context, late renewal in the novel context/early renewal in the acquisition context).
Results
Comparison of patients with SAD and HC
Patients did not differ from HC in age, sex, and years of education, but, as expected, in severity of social anxiety and intrusion symptoms (CAPS-5) regarding their worst aversive social experience (see Table 1).
Analyses of main effects for context-dependent fear conditioning across both groups (SAD and HC) indicated successful fear conditioning and extinction learning, but also spontaneous recovery in the extinction context on the next day. In the novel, as well as acquisition context, fear renewal was shown (see online Supplement F).
Mixed ANOVAs regarding SCRs during all phases of fear conditioning (see Fig. 1), revealed a significant interaction between group and phase for early extinction recall in the extinction context compared with late extinction training (Greenhouse-Geisser F (1,90) = 4.09, p = 0.046). In Fig. 1, patients with SAD appear to have a greater increase in activation in early extinction recall compared to late extinction training, although separate ANOVAs for each phase showed no significant main effects of group (all p ⩾ 0.121). Regarding the other experimental phases, all other ANOVAs revealed no significant main effects of (all p ⩾ 0.121) or interactions with (all p ⩾ 0.108) group.
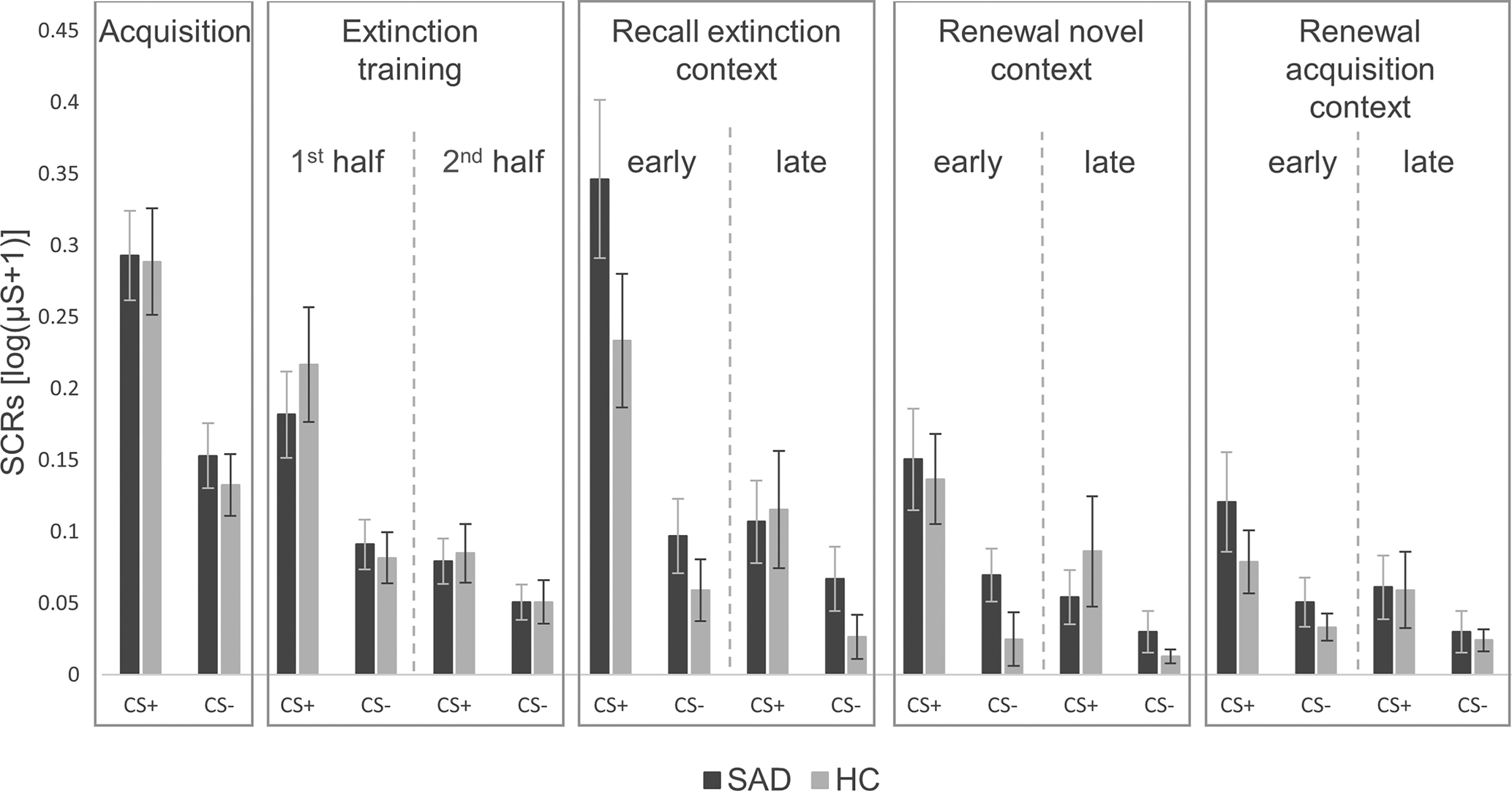
Figure 1. Skin conductance responses for CS+ and CS− during all phases of fear conditioning in patients with social anxiety disorder and healthy controls.
SAD, social anxiety disorder; HC, healthy controls; μS, Microsiemens. ‘1st half’ refers to the first eight trials, ‘2nd half’ to the last eight trials of both CSs. The last two trials of each CS of the extinction training, which were used for the analyses on spontaneous recovery in the extinction context, are not illustrated here. ‘Early’ refers to the first four trials, ‘late’ to the last two trials of both CSs. Error bars represent standard errors of the mean.
On the neural level, HC compared with patients showed increased differential conditioned activation (CS + >CS−) in the right amygdala during early extinction recall in the extinction context (T max = 3.52, p FWEcorr = 0.024, MNI: x = 30, y = −2, z = −22) and during early renewal in the acquisition context (T max = 3.31, p FWEcorr = 0.039, MNI: x = 32, y = −2, z = −22) (see Fig. 2). PPI analyses with these right amygdala activation foci as seed regions revealed no significant group differences in connectivity with activation in any ROIs. There were no differences at the neural level in the other phases.
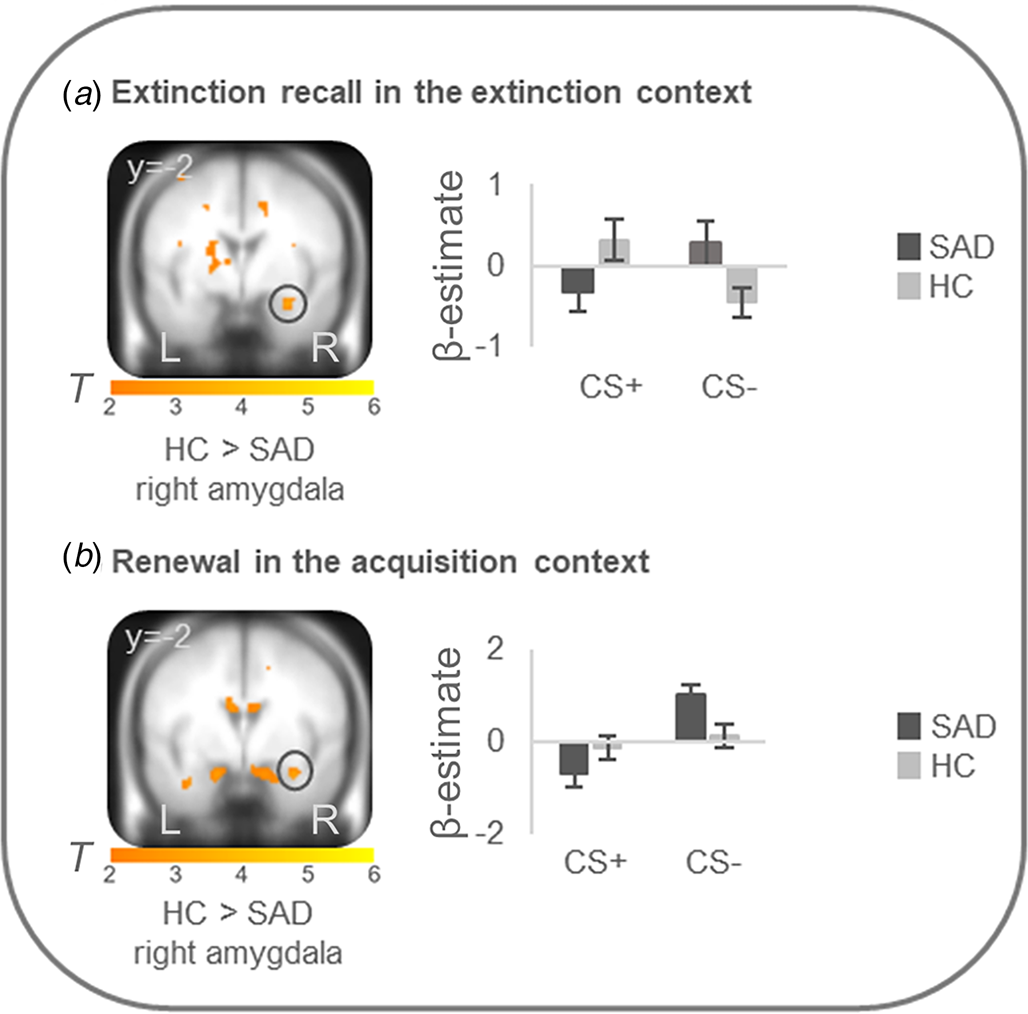
Figure 2. Stronger neural activation for differential conditioned responding (CS+minus CS−) in the right amygdala in healthy controls in comparison to patients with social anxiety disorder during (a) extinction recall in the extinction context and (b) renewal in the acquisition context.
Social anxiety disorder, SAD; healthy controls, HC. Figure shows stronger differential conditioned activation in the right amygdala in HC than in SAD for a recall in the extinction context (T max = 3.52, p FWE = 0.024, MNI: x = 30, y = −2, z = −22) and for b renewal in the acquisition context (T max = 3.31, p FWE = 0.039, MNI: x = 32, y = −2, z = −22). Coordinates are given in MNI space; color bars depict T-values; activations were superimposed on the MNI305 T1 template; bar graphs depict contrast values in peak voxels for CS+ and CS− separately; error bars depict standard errors of the mean.
Analyses of post hoc context ratings did not reveal a significant difference in the overall context recognition score (t (107) = 0.91, p = 0.364), indicating that both groups were equally accurate in telling which contexts they saw and when, and whether they were electrically stimulated in that context. A significant main effect of group was found for fear ratings (F (1, 99) = 9.19, p = 0.003), with the SAD group reporting more fear in all three contexts (acquisition context: t (95.3) = 0.286, p = 0.005; extinction context: t (100) = 2.65, p = 0.009; novel context: t (99) = 2.54, p = 0.013; see online Supplementary Figure S2). There were no significant main effects of or interaction effects with group regarding arousal, valence and UCS expectancy ratings (all p ⩾ 0.073).
Comparisons of patients with clinically relevant and without intrusion symptoms
INT did not differ from NO-INT in age, sex, and years of education, nor in severity of social anxiety symptoms (see Table 2). The INT group reported more intrusion symptoms regarding their aversive social experience and a higher depression score (BDI-II), but they did not meet a depression diagnosis more often than the NO-INT group.
There were no significant differences between the INT and the NO-INT group regarding SCRs during all conditioning phases (all p ⩾ 0.053; see online Supplementary Figure S3).
On the neural level, differential conditioned responses did not differ between groups during fear acquisition. During extinction learning (first minus second half of extinction learning), right amygdala activation (CS + >CS−) more strongly decreased in the INT group compared with the NO-INT group (T max = 4.00, p FWEcorr = 0.011, MNI: x = 26, y = −4, z = −22). Connectivity analyses with this right amygdala activation peak as seed region showed a stronger connectivity with the right insula (T max = 4.73, p FWEcorr = 0.005, MNI: x = 38, y = 14, z = −14) in the INT v. NO-INT group. Stronger left vmPFC activation (CS + >CS−) was found for the NO-INT compared with the INT group, during the late phase of extinction learning (T max = 3.89, p FWEcorr = 0.049, MNI: x = −10, y = 48, z = −18), as well as during early extinction recall in context B (T max = 4.23, p FWEcorr = 0.021, MNI: x = −8, y = 22, z = −24) and early renewal in the novel context C (T max = 4.42, p FWEcorr = 0.014, MNI: x = −8, y = 38, z = −10). The latter two activation differences are located in the posterior part of the vmPFC. In the novel context, PPI analyses revealed stronger connectivity of this left vmPFC activation locus with other subregions of bilateral vmPFC (T max = 4.01, p FWEcorr = 0.041, MNI: x = −2, y = 26, z = −14; T max = 4.29, p FWEcorr = 0.02, MNI: x = 0, y = 26, z = −12) for the NO-INT v. INT group. PPI analyses for vmPFC activation loci during late extinction learning and early extinction recall did not reveal any significant group differences. Since the sphere of the seed region (6 mm radius) for the left vmPFC voxel regarding extinction recall in the extinction context was not fully located in the brain in all subjects, the sphere was reduced to a radius of 4 mm for this single analysis, resulting in n = 18 individuals in the INT and n = 27 individuals in the NO-INT group. During renewal in the acquisition context A, the right amygdala (T max = 3.42, p FWEcorr = 0.041, MNI: x = 16, y = −8, z = −14) was activated more strongly in the INT compared with the NO-INT group (see Fig. 3), but no significant group differences appeared for PPI analyses with this right amygdala activation locus. Because the INT and NO-INT groups differed in their BDI-II scores, we performed the analyses on the significant activation differences described above again and added BDI-II scores as a covariate of no interest. The group differences remain predominantly the same (see online Supplement G).
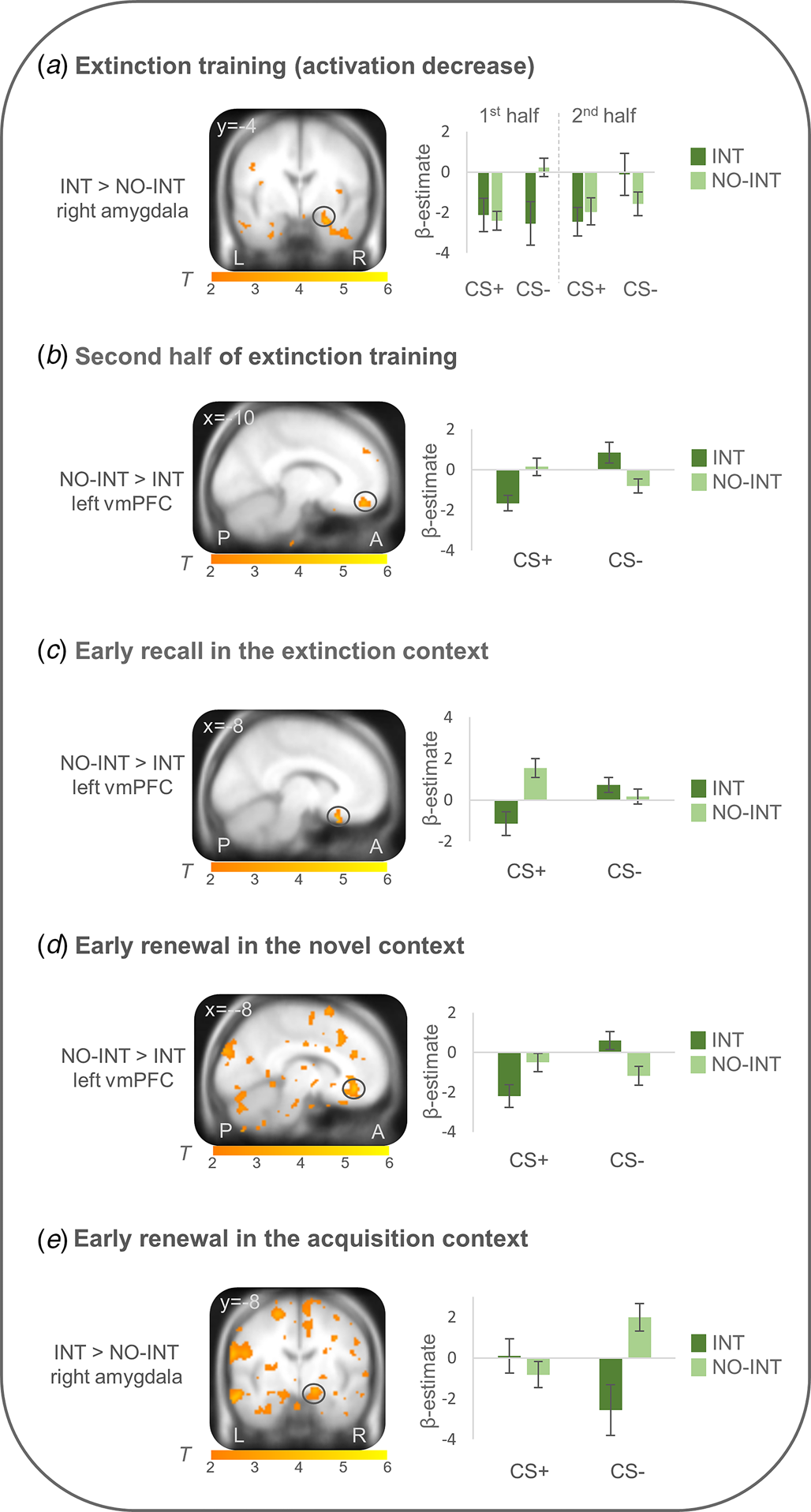
Figure 3. Neural activation of differential conditioned responding (CS+ minus CS−) during extinction training and extinction retrieval (~24h later) in patients with clinically relevant intrusions in comparison to patients without intrusions.
INT, patients with clinically relevant intrusions; NO-INT, patients without intrusions, ‘2nd half’ refers to the last eight trials of both CSs; ‘early’ refers to the first four trials of both CSs. Figure shows a) significantly stronger decrease in right amygdala activation during extinction training (first minus second half, T max = 4.00, p FWEcorr = 0.01, MNI: x = 26, y = −4, z = −22), b) reduced vmPFC activation during late extinction (T max = 3.89, p FWEcorr<0.05, MNI: x = −10, y = 48, z = −18), c) reduced vmPFC activation during early recall in the extinction context (T max = 4.23, p FWEcorr = 0.02, MNI: x = −8, y = 22, z = −24) and d) early renewal in the novel context (T max = 4.42, p FWEcorr = 0.01, MNI: x = −8, y = 38, z = −10), as well as e) stronger right amygdala activation during early renewal in the acquisition context (T max = 3.42, p FWEcorr = 0.04, MNI: x = 16, y = −8, z = −14) in patients with clinically relevant intrusions compared to patients without intrusions. Coordinates are given in MNI space; color bars depict T-values; activations were superimposed on the MNI305 T1 template; bar graphs depict contrast values in peak voxels for CS+ and CS− separately; error bars depict standard errors of the mean.
Moreover, there were no significant differences between the INT and NO-INT group regarding the context recognition score (t (41) = 1.04, p = 0.303), nor UCS expectancy, fear, arousal, and valence ratings of the different contexts (all p ⩾ 0.082).
Discussion
The purpose of this study was to investigate neural correlates of context-dependent fear conditioning processes in SAD and their association with intrusion symptoms in response to an autobiographical aversive social event. In general, SAD patients showed reduced amygdala activation (CS+ v. CS−) during extinction recall in the safe extinction context and during fear renewal in the acquisition context as well as stronger fear ratings for all three contexts. Moreover, a subgroup of patients with clinically relevant intrusions in response to the aversive social event (INT group) showed reduced activation of the vmPFC (CS+ v. CS−) during extinction learning, extinction recall in the safe extinction context, and fear renewal in a novel context compared to a subgroup of patients without intrusions (NO-INT group), while there were no significant differences for self-report and SCRs.
Patients with SAD reported significantly more intrusions in response to aversive social events compared with HC. This finding is in line with previous results (Bjornsson et al., Reference Bjornsson, Hardarson, Valdimarsdottir, Gudmundsdottir, Tryggvadottir, Thorarinsdottir and Thorisdottir2020; Erwin et al., Reference Erwin, Heimberg, Marx and Franklin2006) and underpins the relevance of aversive social experiences and related PTSS in SAD. Regarding the context-dependent fear conditioning paradigm, reduced activation of the right amygdala in SAD compared to HC extends previous results showing diminished vmPFC activation during (context-dependent) extinction recall in social anxiety (disorder) (Marin et al., Reference Marin, Hammoud, Klumpp, Simon and Milad2020; Pejic et al., Reference Pejic, Hermann, Vaitl and Stark2013). In part, our findings correspond to results of a previous study in PTSD, showing reduced SCRs and amygdala activation toward the extinguished CS+ during fear renewal in the acquisition context, which might indicate an inability to use contextual information to regulate conditioned fear (Garfinkel et al., Reference Garfinkel, Abelson, King, Sripada, Wang, Gaines and Liberzon2014). However, visual inspection of the results suggests that the activation differences observed in our study are not due to a stronger recall of fear in HC, but more likely result from a stronger activation toward the CS− in SAD. In line with this, patients with SAD compared with HC reported more fear in all three contexts, including the safe extinction context. Thus, these findings might indicate poorer safety learning in SAD, which is consistent with previous literature (Chauret et al., Reference Chauret, Suffren, Pine, Nassim, Saint-Amour and Maheu2019; Duits et al., Reference Duits, Cath, Lissek, Hox, Hamm, Engelhard and Baas2015; Savage et al., Reference Savage, Davey, Fullana and Harrison2020) and has also been shown in PTSD (Garfinkel et al., Reference Garfinkel, Abelson, King, Sripada, Wang, Gaines and Liberzon2014; Jovanovic, Kazama, Bachevalier, & Davis, Reference Jovanovic, Kazama, Bachevalier and Davis2012). However, other studies found a positive association between symptoms of SAD and safety reversal learning (Savage et al., Reference Savage, Davey, Fullana and Harrison2020). Nevertheless, the meaning of activation/deactivation of the amygdala has not been fully elucidated yet. Previous studies showed inconsistent results regarding the role of the amygdala in extinction learning (Fullana et al., Reference Fullana, Albajes-Eizagirre, Soriano-Mas, Vervliet, Cardoner, Benet and Harrison2018), with different subnuclei of the amygdala and cell types within the amygdala probably subserving different functions in associative fear and extinction processes (Likhtik, Popa, Apergis-Schoute, Fidacaro, & Paré, Reference Likhtik, Popa, Apergis-Schoute, Fidacaro and Paré2008; Repa et al., Reference Repa, Muller, Apergis, Desrochers, Zhou and LeDoux2001). Moreover, amygdala deactivation may also be related to other processes as e.g. emotion regulation (Frank et al., Reference Frank, Dewitt, Hudgens-Haney, Schaeffer, Ball, Schwarz and Sabatinelli2014) or coping (Petrovic, Carlsson, Petersson, Hansson, & Ingvar, Reference Petrovic, Carlsson, Petersson, Hansson and Ingvar2004).
Differences between our and previous findings regarding fear conditioning processes in SAD might be due to methodological issues as e.g. the use of different stimuli (e.g. disorder specific v. unspecific stimuli) (Chauret et al., Reference Chauret, Suffren, Pine, Nassim, Saint-Amour and Maheu2019; Lissek et al., Reference Lissek, Levenson, Biggs, Johnson, Ameli, Pine and Grillon2008; Pejic et al., Reference Pejic, Hermann, Vaitl and Stark2013), different outcome measures or differences in sample size. All in all, our results do not show a clear correspondence between abnormalities in patients with SAD and PTSD regarding context-dependent fear conditioning.
Regarding the association of intrusion symptoms and context-dependent fear conditioning, the INT compared with the NO-INT group showed reduced vmPFC activation during late extinction training, early extinction recall, and early renewal in the novel context. During renewal in the novel context, the INT group also exhibited less connectivity within the vmPFC. These findings fit previous literature on vmPFC hypoactivation during extinction learning as well as extinction recall in PTSD (Diekhof, Geier, Falkai, & Gruber, Reference Diekhof, Geier, Falkai and Gruber2011; Garfinkel et al., Reference Garfinkel, Abelson, King, Sripada, Wang, Gaines and Liberzon2014; Milad et al., Reference Milad, Pitman, Ellis, Gold, Shin, Lasko and Rauch2009; Rougemont-Bücking et al., Reference Rougemont-Bücking, Linnman, Zeffiro, Zeidan, Lebron-Milad, Rodriguez-Romaguera and Milad2011; Shvil, Rusch, Sullivan, & Neria, Reference Shvil, Rusch, Sullivan and Neria2013; Suarez-Jimenez et al., Reference Suarez-Jimenez, Albajes-Eizagirre, Lazarov, Zhu, Harrison, Radua and Fullana2020; VanElzakker et al., Reference VanElzakker, Staples-Bradley, Shin, Vermetten, Germain and Neylan2018) and also extinction recall in SAD (Marin et al., Reference Marin, Hammoud, Klumpp, Simon and Milad2020). Since activation of the vmPFC is associated with safety or extinction learning (Fullana et al., Reference Fullana, Harrison, Soriano-Mas, Vervliet, Cardoner, Àvila-Parcet and Radua2016; Milad et al., Reference Milad, Wright, Orr, Pitman, Quirk and Rauch2007; Phelps, Delgado, Nearing, & LeDoux, Reference Phelps, Delgado, Nearing and LeDoux2004), these results support our hypothesis of a link between dysfunctional regulation of fear and intrusion symptoms. Another study (Garfinkel et al., Reference Garfinkel, Abelson, King, Sripada, Wang, Gaines and Liberzon2014) also found lower activation of the vmPFC in PTSD during renewal (in response to the extinguished CS + ), but in the acquisition context, and at the same time lower SCRs. These results suggest that vmPFC activation might not have a fear-inhibiting effect per se, but rather a fear-modulating effect. Missing differences in conditioned SCRs and subjective responses as well as vmPFC activation differences in the novel but not the acquisition context in our study could, however, not fully support this interpretation. While it is well known that the vmPFC is involved in the inhibition of fear expression via connections to the amygdala (Motzkin, Philippi, Wolf, Baskaya, & Koenigs, Reference Motzkin, Philippi, Wolf, Baskaya and Koenigs2015; Quirk & Mueller, Reference Quirk and Mueller2008), the abovementioned findings suggest that the role of the vmPFC in fear conditioning processes is more complex. Moreover, it is involved in the acquisition of new learning content (Battaglia, Garofalo, Di Pellegrino, & Starita, Reference Battaglia, Garofalo, Di Pellegrino and Starita2020), or the integration of information (Nieuwenhuis & Takashima, Reference Nieuwenhuis and Takashima2011), e.g. by using contextual information to regulate fear (Gonzalez & Fanselow, Reference Gonzalez and Fanselow2020; Hermann et al., Reference Hermann, Stark, Milad and Merz2016; Kalisch et al., Reference Kalisch, Korenfeld, Stephan, Weiskopf, Seymour and Dolan2006; Pennington, Anderson, & Fanselow, Reference Pennington, Anderson and Fanselow2017). Furthermore, previous studies have identified subregions of the vmPFC associated with different states (anticipated threat or processing of safety signals) (Battaglia, Harrison, & Fullana, Reference Battaglia, Harrison and Fullana2022; Harrison et al., Reference Harrison, Fullana, Via, Soriano-Mas, Vervliet, Martínez-Zalacaín and Cardoner2017; Tashjian, Zbozinek, & Mobbs, Reference Tashjian, Zbozinek and Mobbs2021). Considering different activation loci within the vmPFC as found in our study, a more detailed analyses of vmPFC subregions could help to more clearly understand its role in (context-dependent) fear conditioning.
Moreover, the INT compared to the NO-INT group exhibited altered activation of the right amygdala: during extinction training they showed a greater decrease of conditioned amygdala activation which could be interpreted as an indicator for both, a stronger reduction of conditioned fear or deficient extinction learning regarding the abovementioned different functions of amygdala subregions during extinction learning (Likhtik et al., Reference Likhtik, Popa, Apergis-Schoute, Fidacaro and Paré2008; Repa et al., Reference Repa, Muller, Apergis, Desrochers, Zhou and LeDoux2001). In addition, this result is contrary to previous findings in PTSD showing reduced decrease of amygdala activation during extinction learning (Milad et al., Reference Milad, Pitman, Ellis, Gold, Shin, Lasko and Rauch2009; Suarez-Jimenez et al., Reference Suarez-Jimenez, Albajes-Eizagirre, Lazarov, Zhu, Harrison, Radua and Fullana2020). In a study with healthy participants, social anxiety was negatively correlated with amygdala activity during extinction learning, which may indicate reduced extinction learning in people with stronger social anxiety (Pejic et al., Reference Pejic, Hermann, Vaitl and Stark2013). Moreover, hyperactivation of the right amygdala during renewal in the acquisition context in the INT compared with the NO-INT group was not in line with a previous study, where patients with PTSD demonstrated attenuated fear renewal in SCRs and decreased amygdala activity, however only in response to the CS+ alone (Garfinkel et al., Reference Garfinkel, Abelson, King, Sripada, Wang, Gaines and Liberzon2014). Visual inspection of the data also indicates that primarily activation differences in response to the CS− contributed to the abovementioned amygdala findings. All in all, our findings regarding altered amygdala activation in INT v. NO-INT patients are difficult to interpret and need further investigation.
A limitation of our study is that main effects analyses in both groups (SAD and HC) revealed a significant return of fear during extinction recall in the extinction context on the second day, which might be due to immediate extinction training after fear acquisition without a consolidation phase (Merz, Hamacher-Dang, & Wolf, Reference Merz, Hamacher-Dang and Wolf2016). Moreover, the specific instruction that contingencies will not change during the fear conditioning paradigm might have contributed to this effect. In addition, the INT group exhibited more severe depressive symptoms compared with the NO-INT group. Besides intrusion symptoms, this factor might also have contributed to deficient context-dependent modulation of conditioned fear.
Our study also raises a methodological discussion point. Multiple outcome measures ideally allow for a broad and clear interpretation of results. However, it also raises the question of the relevance of various biological measures, such as neural activation and SCR, if these are not reflected in subjective outcome measures (e.g. fear rating), or vice versa (see e.g. Ahrens et al., Reference Ahrens, Pauli, Reif, Mühlberger, Langs, Aalderink and Wieser2016).
Our results provide evidence for the transdiagnostic relevance of intrusion symptoms in association with context-dependent fear conditioning processes in SAD. Regarding implications for the treatment of SAD, it should be addressed how exposure therapy in SAD can be designed to facilitate recall of extinction memories in different contexts. Imagery rescripting seems to be a promising intervention in order to overcome aversive social memories in SAD (Norton & Abbott, Reference Norton and Abbott2017; Reimer & Moscovitch, Reference Reimer and Moscovitch2015) and its effects on extinction recall and fear renewal in real-life social situations should be investigated in futures studies.
Supplementary material
The supplementary material for this article can be found at https://doi.org/10.1017/S0033291723002179.
Acknowledgements
We thank our student assistants A. Küss, G. Schneider, S. Koch, D. Meschke, J. Theis, S. Streckmann, K. Ulrich, L. Geißert, J. Rechmann, L. Rosenplänter, W. Pitz, J. Gessner, and I. Birlenbach for help in participant recruitment and data collection. In addition, we thank Dr C. R. Blecker for technical support and Dr O. Kruse for support in SCR data analysis. MR-imaging for this study was performed at the Bender Institute of Neuroimaging (BION) at the Justus Liebig University Giessen, Germany.
Financial support
The study was supported by grants from the German Research Foundation (DFG) to A. Hermann (HE 7013/2-1), and R. Stark (STA 475/20-1). It was further supported by ‘The Adaptive Mind’, funded by the Excellence Program of the Hessian Ministry of Higher Education, Science, Research and Art (HMWK). The DFG and HMWK had no further role in study design, collection, analysis and interpretation of data, in the writing of the manuscript or in the decision to submit the paper for publication. Rosa Seinsche was supported by a PhD Scholarship from Justus Liebig University Giessen.
Ethical standards
The authors assert that all procedures contributing to this work comply with the ethical standards of the relevant national and institutional committees on human experimentation and with the Helsinki Declaration of 1975, as revised in 2008.
Competing interest
None.