From birth onwards, there is rapid microbial colonisation of the gastrointestinal tract of infants( Reference Mackie, Sghir and Gaskins 1 ). The gut microbiota plays an important role in the priming and maturation of the immune system of infants( Reference Kelly, King and Aminov 2 , Reference Martin, Nauta and Ben Amor 3 ). It is influenced by many factors including the duration of gestation, mode of delivery, mode of infant feeding, hospitalisation, antibiotic treatment, geographical location( Reference Biasucci, Rubini and Riboni 4 – Reference Roger and McCartney 7 ) and, according to recent evidence, maternal pregnancy-associated weight gain and BMI( Reference Collado, Isolauri and Laitinen 8 ). Perturbations in the composition of the gut microbiota have been reported to be associated with inflammation and disease, including, but not limited to, allergies( Reference Penders, Stobberingh and van den Brandt 9 ), metabolic disorders( Reference Munukka, Wiklund and Pekkala 10 ), CVD( Reference Manco, Putignani and Bottazzo 11 ), obesity( Reference Manco, Putignani and Bottazzo 11 ) and Crohn's disease( Reference Sokol, Pigneur and Watterlot 12 ). The consumption of probiotics by women during pregnancy and/or early lactation has been reported to influence the microbiota of their infants( Reference Sanz 13 , Reference Rautava, Collado and Salminen 14 ), which in some cases has lasting effects beyond the duration of the intervention( Reference Sanz 13 , Reference Grzeskowiak, Gronlund and Beckmann 15 ). Some studies have suggested that PUFA have an effect on faecal microbiota in animals( Reference Andersen, Molbak and Thymann 16 – Reference Leonard, Sweeney and Bahar 19 ), in infants aged 9–18 months( Reference Andersen, Molbak and Michaelsen 20 , Reference Nielsen, Nielsen and Lauritzen 21 ) and in adult humans( Reference Fava, Gitau and Griffin 22 ). To the best of our knowledge, there are no studies that have investigated the faecal microbiota within a population of pregnant women with increased oily fish consumption. The Salmon in Pregnancy Study (SiPS) is a randomised, controlled intervention investigating the effects of the consumption of two portions of salmon per week, providing 3·45 g/week EPA and DHA, from 20 weeks of pregnancy to delivery( Reference Miles, Noakes and Kremmyda 23 ). We have demonstrated higher levels of EPA and DHA in maternal and cord blood plasma( Reference Miles, Noakes and Kremmyda 23 ) and in breast milk( Reference Urwin, Miles and Noakes 24 ) in the salmon group in the SiPS. The primary focus of the study is the effect of long-chain (LC) n-3 PUFA from the salmon, although, as a whole food, salmon is a good source of vitamin D and Se. In the present study, we tested the hypotheses that increased consumption of salmon by pregnant women would affect the composition of their faecal microbiota and that of the faecal microbiota of their infants.
Materials and methods
Study design and subject characteristics
The SiPS is a single-blind, randomised, controlled intervention with salmon during pregnancy; the full study design and baseline subject characteristics have been reported previously( Reference Miles, Noakes and Kremmyda 23 ). In brief, women (n 123) who rarely ate oily fish and were at a risk of having a child with atopy because of a family history were randomly assigned to either continue consuming their habitual diet (control group; n 61) or consume farmed salmon and requested to consume two 150 g portions of salmon per week from 20 weeks of pregnancy to delivery (salmon group; n 62). The complete details of the farmed salmon have been reported previously( Reference Miles, Noakes and Kremmyda 23 ); the two portions of salmon typically provided 3·45 g of EPA plus DHA per week, equivalent to approximately 0·48 g/d EPA+DHA. The two portions of salmon also provided 28 μg of vitamin D and 86 μg of Se; contaminant levels were below the provisional weekly tolerable intake levels( Reference Miles, Noakes and Kremmyda 23 ). The study was conducted according to the guidelines laid down in the Declaration of Helsinki. All procedures involving human subjects were approved by the Southampton and South West Hampshire Research Ethics Committee (07/Q1704/43), and written informed consent was obtained from all the women. The SiPS is registered at http://www.clinicaltrials.gov (clinical trials identifier NCT00801502).
Assessment of maternal and infant diets
Maternal diet was assessed using an administered 100-item FFQ at 20 and 34 weeks of gestation and 3 months post-partum. Women reported their frequency of consumption of the listed foods during the preceding 3 months. This FFQ has been validated previously in a group of pregnant women and was used to assess the diets of women in the Southampton Women's Survey( Reference Crozier, Robinson and Borland 25 ). For the SiPS, a section on the intakes of functional foods was added to record any regular consumption of prebiotics and/or probiotics. A daily record of the mode of infant feeding, i.e. breast-, formula- or mixed-fed, was made for the first 6 months post-partum, including details on the type of infant formula, if used, and any illnesses that the infants may have suffered( Reference Vlachava 26 ). Infant formula was described as standard or supplemented depending on whether prebiotics (fructo-oligosaccharides/galacto-oligosaccharides) had been added. All infant formulae contained DHA (5·8–7·0 mg/100 ml).
Faecal sample collection and preparation
During the clinic visit at 38 weeks of gestation, freshly voided faecal samples were collected from the mothers. Faecal samples from infants were collected at their homes on days 7, 14, 28 and 84 post-partum from their nappies as soon as possible after defaecation, taking care to avoid collecting any faeces that had been mixed with urine. All the samples were immediately stored at − 20°C until processing. The samples were defrosted on ice, then diluted 1:10 (w/w) with PBS (0·1 m; pH 7·0) and mixed in a Stomacher400 (Seward) for 2 min at normal speed (460 paddle beats per min). Into a 50 ml sterile plastic centrifuge tube containing 2 g of glass beads (5 mm diameter), 5 ml of faecal slurry were transferred and vortexed for 30 s to further homogenise the samples. The samples collected from the mothers were subjected to centrifugation at 13 000 g for 2 min. The resulting suspensions from samples collected from infants and supernatants of the faecal samples collected from the mothers were immediately processed for the microbial analysis. For the determination of secretory IgA (sIgA) and calprotectin concentrations, two 1 ml aliquots of homogenates were frozen at − 20°C.
Culture-independent enumeration of faecal bacteria using 16S ribosomal RNA probes labelled with cyanine 3 and fluorescence in situ hybridisation
Fluorescence in situ hybridisation was carried out as described previously( Reference Daims, Stoecker, Wagner, Osborn and Smith 27 ) with some modifications. Briefly, faecal homogenate samples (375 μl) were fixed with ice-cold 4 % filtered paraformaldehyde (pH 7·2; Sigma-Aldrich) at a ratio of 1:3 (v/v) at 4°C overnight. They were then centrifuged at 13 000 g for 5 min and washed twice with filtered PBS (1 ml). The resultant pellet was then re-suspended in 300 μl of filter-sterilised PBS–ethanol (1:1, v/v) and stored at − 20°C until analysis. For the hybridisations, 20 μl of each sample were pipetted into a well (10 mm diameter) of Teflon and poly-l-lysine-coated six-well slides (Tekdon, Inc.). The samples were dried on a desktop plate incubator (Amersham International) at 46°C for 15 min. To permeabilise the cells for use with probes Bif164, Lab158 and Ato291, the samples were treated with 20 μl of lysozyme (1 mg/ml in 100 mm-HCl, pH 8·0) for 15 min at 37°C and then briefly washed with distilled water (2–3 s). All the samples were dehydrated in a series of ethanol solutions (50, 80 and 96 % ethanol in distilled water v/v) for 3 min each. The slides were dried for a further 2 min on a desktop plate incubator. The nucleic acid stain 4,6-diamidino-2-phenylindole (DAPI; Sigma Aldrich) was used for total cell counts. The group- or genus-specific probes used are listed out in Table 1: Bif164, specific for the Bifidobacterium genus; Lab158, for the Lactobacillus–Enterococcus group; Ato291, for the Atopobium cluster, including most Coriobacteriaceae species; Erec482, for the Eubacterium rectale–Clostridium coccoides group (Clostridium cluster XIVa); Bac303, for the Bacteroides and Prevotella group; CHis150, for the Clostridium histolyticum subgroup (Clostridium clusters I and II); EC1531, for Escherichia coli; FPra655, for the Faecalibacterium prausnitzii group (Clostridium cluster IV). All the probes were labelled at the 5′ end with the fluorescent dye cyanine 3 (Cy3) (Sigma Aldrich). The compositions of the hybridisation and wash buffers were as reported in probeBase( Reference Loy, Maixner and Wagner 28 ) depending on the ribosomal RNA-targeted oligonucleotide probe used. The probe (5 μl of a 5 ng/μl stock) in hybridisation buffer (45 μl) was applied to the surface of each well, and hybridisation was carried out for 4 h using an ISO20 oven (Grant Boekel). The slides were washed with wash buffer (50 ml) containing DAPI (20 μl, 50 ng/μl) for 15 min, then dipped briefly in ice-cold water (2–3 s) and dried with compressed air. Prolong Gold Antifade (Invitrogen) (5 μl) was added to each well, and the well was covered with a coverslip. The slides were stored in the dark at room temperature for 24 h and then at 4°C until cell count using a SP460F fluorescence microscope (Brunel Microscopes Limited). An average count from thirty random fields of view was taken for the enumeration of bacterial cells( Reference Blaut, Collins and Welling 29 ). Microbial counts are expressed as log10 bacterial cells per g faeces (wet weight) or as a percentage of total bacteria (DAPI). The limit of detection was about log10 5·06.
Table 1 16S ribosomal RNA probes used in the fluorescence in situ hybridisation analysis
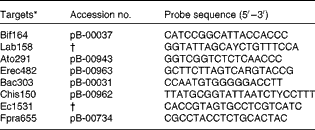
* Probe designation according to probeBase (http://www.microbial-ecology.net/probebase). All the probes were used at a concentration of 50 ng/ml.
† No information related to these probes has been deposited in probeBase.
Determination of secretory IgA concentrations in faeces
A commercially available ELISA kit was used for the determination of sIgA concentrations in faeces (Immundiagnostik AG). A faecal suspension (500 μl; 1:10, v/v faeces in 0·1 m-PBS) was centrifuged at 13 000 g for 5 min, and the resulting supernatant was used in the assay. The manufacturer's guidelines were followed. The plates were read at 450 nm with 620 nm as the reference wavelength on a GENios SpectraFLUOR plus (Tecan UK Limited). The limit of detection was 13·4 ng/ml, and the intra-and inter-assay CV were 3·0 and 14 %, respectively.
Determination of calprotectin concentrations in faeces
A commercially available ELISA kit (Nova Tec Immundiagnostica GmbH) was used for the determination of calprotectin concentrations in faeces. A faecal suspension (500 μl; 1:10, v/v faeces in 0·1 m-PBS) was mixed at a ratio of 1:5 with the extraction buffer. In a sealed Sterilin tube, the sample was shaken on a Vibrax-VXR (IKA-Werke GmbH & Co. KG) for 30 min at 1000 rpm, and then the sample (1 ml) was centrifuged at 10 000 g for 20 min at 4°C. The resulting supernatant was used in the assay. The manufacturer's guidelines were followed. The plates were read at 405 nm on a GENios SpectraFLUOR plus (Tecan UK Limited). The intra- and inter-assay CV were 3·5 and 13 %, respectively.
Statistical analysis
Data were checked for normality with histograms and the Kolmogorov–Smirnov test; data were log-transformed to establish normality as required. Continuous data are expressed as means and their standard errors or medians and 25th–75th percentiles as appropriate. In the analysis of the composition of the faecal microbiota using fluorescence in situ hybridisation, when a bacterial group was not detected, the minimum detection value (log10 5·06 cells/g wet faeces) was applied for the statistical analysis. Infant feeding was categorised as exclusively breast-fed (BF) or exclusively formula-fed (FF) from day 2 post-partum. Infants who were mixed-fed were excluded from the analysis. The generalised linear mixed model was used to test the main effects of intervention, time post-partum and infant feeding and any interaction effects. Categorical data are expressed as number and proportion with comparisons using the χ2 test or Fisher's exact test as appropriate. Correlations were carried out with Spearman's rank correlation or partial correlations on transformed data, adjusting for intervention, time post-partum or mode of infant feeding. The Statistical Package for Social Sciences version 20 (IBM Corporation) was used for all the statistical tests. In all cases, a P value < 0·05, after Bonferroni adjustment for multiple comparisons, was considered statistically significant.
Results
Subject characteristics
The characteristics of women who provided a faecal sample at 38 weeks of gestation are given in Table 2. Weights at the screening appointment (13 weeks of gestation) compared with those at the clinic appointment during the third trimester (34 weeks of gestation) were used to calculate weight gain during pregnancy. Normal weight gain was considered to be 11·4–15·9 kg for normal-weight women (BMI 18·5–24·9 kg/m2), 6·8–11·4 kg for overweight women (BMI 25·0–29·9 kg/m2) and 5·0–9·1 kg for obese women (BMI >30·0 kg/m2)( 30 ). Total weight gains above these values were considered excessive. Age, parity, self-reported atopy (report of asthma or allergy in a first-degree relative of the unborn child), weight, weight gain and BMI did not differ significantly between the two groups (Table 2). There were no differences in the intake of total energy, protein, fat, SFA, MUFA, carbohydrates, dietary fibre and functional foods between the groups (Table 2), but there was an increased intake of oily fish (salmon), EPA and DHA( Reference Miles, Noakes and Kremmyda 23 ) and two other oily fish-derived nutrients, vitamin D and Se in the salmon group (Table 2)( Reference Miles, Noakes and Kremmyda 23 ).
Table 2 Characteristics of the women who provided a faecal sample at 38 weeks of pregnancy (Mean values with their standard errors; median values with their 25th and 75th percentiles; number of participants and percentages)

* Weight measurement and parity data missing for one woman in the salmon group.
† Values were compared with Student's t test.
‡ Values were compared with the Mann–Whitney U-test.
§ Weight gain between week 13 and week 34 of pregnancy.
∥ Values were compared with the χ2 test.
Effect of salmon intervention and habitual diet on maternal microbiota composition
There were no significant effects of increased oily fish consumption on any of the bacteria enumerated in maternal faecal samples, sampled at 38 weeks, expressed as either log10 cells/g of wet faeces (Table 3) or as a percentage of total bacteria (data not shown).
Table 3 Effect of salmon consumption on bacterial numbers (log10 cells/g wet faeces) and secretory IgA (sIgA) and calprotectin concentrations (mg/kg wet faeces) in maternal faecal samples at 38 weeks of pregnancy (Median values with their 25th and 75th percentiles)
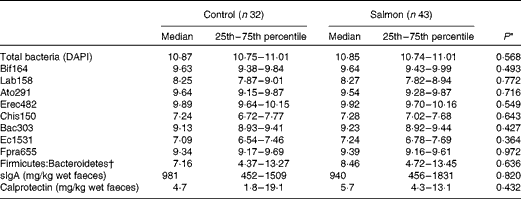
DAPI, 4,6-diamidino-2-phenylindole.
* Mann–Whitney U-test.
† Firmicutes = Lab158+Erec482+Chis150+Fpra655; Bacteroidetes = Bac303.
Influence of weight gain on maternal microbiota composition
Women who had a normal weight gain had a median weight gain of 9·3 kg (7·3–11·2 kg; 25th–75th percentiles), which was lower (P< 0·001) than that observed in women who had an excessive weight gain (median 13·8 kg (12·3–15·9 kg; 25th–75th percentiles)). The BMI values of women with normal and excessive weight gains were significantly different at both 13 weeks of gestation (median 22·8 v. 27·7 kg/m2; P< 0·001) and 34 weeks of gestation (median 26·6 v. 33·1 kg/m2; P< 0·001). The numbers of total bacteria (P= 0·045; ratio 1·23) and bacteria of the F. prausnitzii group (P= 0·039; ratio 1·91) in samples collected from women with a normal weight gain were higher than those present in samples collected from women with an excessive weight gain (Table 4). There were also trends towards higher numbers (log10 cells/g wet faeces) of bacteria of the Bifidobacterium genus (P= 0·094) and Atopobium cluster (P= 0·057) in women with a normal weight gain (Table 4). There was a difference in the faecal microbiota of women with a normal weight gain compared with that of women with an excessive weight gain.
Table 4 Effect of weight gain during pregnancy on bacterial numbers (log10 cells/g wet faeces) and secretory IgA (sIgA) and calprotectin concentrations (mg/g wet faeces) in maternal faecal samples at 38 weeks of pregnancy (Median values with their 25th and 75th percentiles)
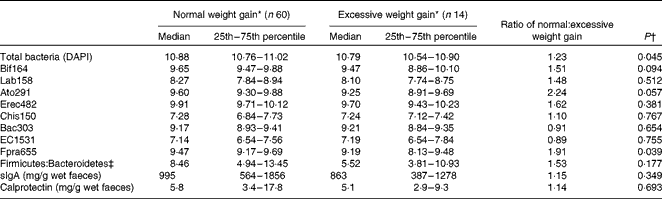
DAPI, 4,6-diamidino-2-phenylindole.
* Normal weight gains over pregnancy according to the Institute of Medicine( 30 ): 11·4–15·9 kg (BMI 18·5–24·9 kg/m2); 6·8–11·4 kg (BMI 25·0–29·9 kg/m2); 5·0–9·1 kg (BMI >30·0 kg/m2).
† Mann–Whitney U-test.
‡ Firmicutes = Lab158+Erec482+Chis150+Fpra655; Bacteroidetes = Bac303.
Effect of salmon consumption on maternal faecal calprotectin and secretory IgA concentrations
There were no effects of salmon intervention on maternal faecal calprotectin or sIgA concentrations (Table 3). There was also no influence of weight gain (normal v. excessive) on either calprotectin or sIgA concentrations (Table 4), and there was no correlation between breast milk sIgA concentrations( Reference Urwin, Miles and Noakes 24 ) and maternal faecal sIgA concentrations (P= 0·630).
Influence of mode of infant feeding on faecal microbiota composition
The numbers of bacteria of the Clostridium cluster XIVa, Atopobium cluster, C. histolyticum subgroup and Bacteroides–Prevotella group in the faeces of the exclusively BF infants were lower than those in the faeces of the exclusively FF infants (P= 0·005, 0·050, 0·016 and 0·037, respectively). After stratification of the data for intervention, the numbers of bacteria of the Atopobium cluster in the faeces of the BF infants were significantly lower than those in the faeces of the FF infants in the control group (P= 0·012, P< 0·05 after adjustment for multiple testing), but this was not the case for those in the salmon group (P= 0·671). The numbers of bacteria of the C. histolyticum subgroup in the faeces of the BF infants were lower than those in the faeces of the FF infants in the salmon group (P= 0·008, P< 0·05 after adjusting for multiple testing), but this was not significant in the control group (P= 0·647). The numbers of bacteria of the Clostridium cluster XIVa in the faeces of the BF infants were lower than those in the faeces of the FF infants in the control group (P= 0·006, P< 0·05 after adjusting for multiple testing) but not in the faeces of those in the salmon group (P= 0·580).
Effect of salmon intervention on infant microbiota composition
There were no effects of maternal salmon consumption on the duration of gestation, birth weight or mode of delivery (Table 5), and there was no statistically significant difference in mode of infant feeding and/or formula type used between the control and intervention groups (Table 5). There was an increase over time in the number of total bacteria and of the individual bacterial groups enumerated, with the exception of E. coli, the bacterial counts of which decreased, and the Atopobium cluster, the bacterial counts of which did not change significantly during the sampling period (Table 6). In a primary analysis of infants who were exclusively BF or exclusively FF during the first 84 d post-partum, a trend towards lower numbers of bacteria of the Atopobium cluster (P= 0·097) in the faeces of infants in the salmon group was observed. In a post hoc analysis, maternal salmon consumption during pregnancy was found to be associated with significantly lower numbers of bacteria of the Atopobium cluster in the faeces of the exclusively FF infants (P< 0·05), but not with those in the exclusively BF infants (Fig. 1).
Table 5 Characteristics of the infants from whom faecal samples were collected at days 7, 14, 28 and 84 post-partum (Mean values with their standard errors; median values with their 25th and 75th percentiles; number of participants and percentages)
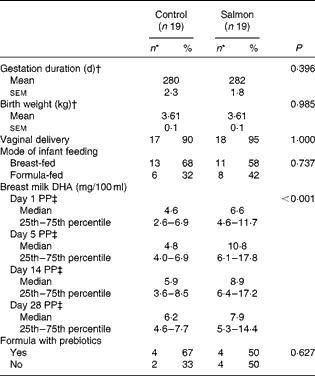
PP, post partum.
* Values were compared with the χ2 test.
† Values were compared with Student's t test.
‡ Values were compared with the generalised linear mixed model.
Table 6 Effect of maternal salmon consumption on infant faecal bacterial numbers (log10 cells/g wet faeces) for those infants who were exclusively breast-fed (BF) or exclusively formula-fed (FF)* (Median values with their 25th and 75th percentiles)

a,bValues within a row with unlike superscript letters were significantly different (P< 0·05).
* Control (n 19) and salmon (n 19); samples were not available at all time points from all the infants. There were no significant group × time interactions. Although there were significant effects of time and mode of infant feeding, there were a limited number of significant post hoc tests.
† Generalised linear mixed model.
‡ Significant difference between modes of infant feeding (P< 0·05).

Fig. 1 Influence of maternal salmon consumption during pregnancy and mode of infant feeding on the numbers of bacteria of the Atopobium cluster (Ato291) in the faeces of infants. Statistical significance of intervention, time and mode of infant feeding was P= 0·097, 0·297 and 0·050, respectively. In the post hoc analysis, the numbers of Ato291 in the (a) breast-fed infants were found to be not significantly affected by salmon consumption, but they were significantly affected in the (b) formula-fed infants (intervention: P< 0·05). □, Control group; , salmon group. Circles represent outliers.
Effect of salmon intervention on infant faecal secretory IgA and calprotectin concentrations
Infant faecal sIgA concentrations were not significantly affected by maternal salmon consumption during pregnancy (data not shown). However, there was a main effect of mode of infant feeding and a mode of infant feeding × time interaction (P< 0·001, for both) (Fig. 2); compared with those in the faeces of the FF infants, sIgA concentrations were higher in the faeces of the BF infants at all post-partum sampling times (P< 0·05, for all), and with time, faecal sIgA concentrations decreased in the BF infants but increased in the FF infants (Fig. 2). However, in the exclusively BF infants, there was no correlation between breast milk sIgA concentrations( Reference Urwin, Miles and Noakes 24 ) and infant faecal sIgA concentrations (P= 0·579; data not shown). There were no significant effects of salmon intervention or mode of infant feeding on faecal calprotectin concentrations (Fig. 2).
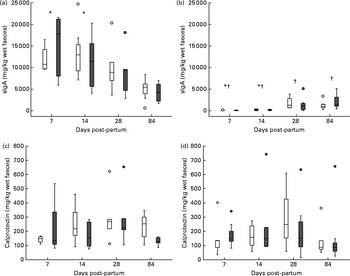
Fig. 2 Influence of mode of infant feeding on (a, b) infant faecal secretory IgA (sIgA) concentrations (mg/kg faeces) and (c, d) infant faecal calprotectin concentrations (mg/kg faeces). (a) Breast-fed infants (time: P< 0·001); (b) formula-fed infants (time: P< 0·001); (c) breast-fed infants (time: P= 0·167); (d) formula-fed infants (time: P= 0·068). Statistical significance for the main effects of mode of infant feeding and time for sIgA was P< 0·001 and 0·012, respectively. * Values were significantly different from day 84 post-partum (P< 0·05). † Values were significantly different from breast-fed (P< 0·05). Statistical significance of mode of infant feeding and time for calprotectin was P= 0·474 and 0·010, respectively. □, Control group; , salmon group. Circles represent outliers.
Discussion
Herein, we report that increased maternal oily fish consumption during pregnancy is associated with a trend towards lower numbers of bacteria of the Atopobium cluster in the faeces of infants during the first 84 d post-partum. Exclusive breast-feeding was associated with lower numbers of bacteria of the Clostridium cluster XIVa, Atopobium cluster, C. histolyticum subgroup and Bacteroides–Prevotella group compared with exclusive formula feeding, in agreement with the findings of other studies( Reference Roger and McCartney 7 ). However, in the salmon group, the numbers of bacteria of the Atopobium cluster in the faeces of the BF infants compared with those in the faeces of the FF infants were not significantly different, in contrast to previous findings( Reference Roger and McCartney 7 ). It appears from the present study that the mode of infant feeding also had an impact on the effect of intervention, since the faeces of the FF infants in the salmon group had significantly lower numbers of bacteria of the Atopobium cluster than those of the FF infants in the control group, but this was not significant for the BF infants. In a study( Reference Andersen, Molbak and Michaelsen 20 ) where infants aged 9–18 months were supplemented with fish oil, the magnitude of the effect of intervention on the composition of the microbiota was also found to be affected by the mode of infant feeding. In that study, the composition of the faecal microbiota was determined using terminal restriction fragment length polymorphism. Terminal restriction fragments Bp100 and Bp102, both presumed to be related to Bacteroidetes, exhibited larger and smaller increases, respectively, during the course of intervention, in the faeces of the fish oil-supplemented group compared with those in the faeces of the sunflower oil-supplemented group, but this was only significant for those infants who had ceased breast-feeding before intervention( Reference Andersen, Molbak and Michaelsen 20 ). In the present study, we did not find a significant difference in numbers of bacteria of the Bacteroides–Prevotella group between the groups. Furthermore, in a recent study in mice, n-3 PUFA supplementation has been found to decrease the number of Bacteroidetes, but not to the same extent as SFA did, which had a greater overall influence on the shift in the composition of the faecal microbiota than PUFA did( Reference Liu, Hougen and Vollmer 17 ). The effect of PUFA supplementation has also been investigated in piglet caeca( Reference Andersen, Molbak and Thymann 16 ), where fish oil was found to be associated with higher numbers of bacterial types assumed to be Actinobacteria and Proteobacteria, while sunflower oil was found to be associated with a higher abundance of bacterial types assumed to be Bacteroidetes. It is important to acknowledge that in the previous studies, the provision of n-3 PUFA was postnatal (i.e. directly to the infant), whereas in the present study, that of salmon, a source of n-3 PUFA, was prenatal (i.e. indirectly to the fetus), although breast milk content of n-3 PUFA was higher in the salmon group in the present study( Reference Urwin, Miles and Noakes 24 ). It is also important to note that although n-3 PUFA might be mediating the effect of salmon feeding, the salmon used was also a good source of some other nutrients including vitamin D and Se, and these nutrients may have a role in any effect of the salmon.
The influence of the difference in the numbers of bacteria of the Atopobium group, as has been observed in the present study, and any effect that they may have on the maturation of the immune system and the longer-term health of infants are unknown, although higher bacterial counts of the Atopobium group have been found in children with active coeliac disease( Reference Collado, Calabuig and Sanz 31 ). Further development and research on the bacterial communities and their effect on the host, such as the Microbiome Project( Reference Proctor 32 ), will provide valuable information in the future.
The BF infants in the salmon group consumed milk enriched with LC n-3 PUFA and lower in MUFA during the first month post-partum compared with controls( Reference Urwin, Miles and Noakes 24 ). The exclusively FF infants consumed milk supplemented with DHA, but at a concentration lower than that in the breast milk of the salmon group. Since the exclusively FF infants were not exposed to breast milk, any possible effect of intervention in this group must have originated in utero. The mothers of infants in the salmon group had a higher intake of LC n-3 PUFA, vitamin D and Se during pregnancy, and infants in the salmon group were born with a higher LC n-3 PUFA status( Reference Miles, Noakes and Kremmyda 23 ) and with some differences in cord blood immune cell phenotypes and cord blood immune response to endotoxins( Reference Noakes, Vlachava and Kremmyda 33 ). The increased LC n-3 PUFA status( Reference Miles, Noakes and Kremmyda 23 ) and altered immune response( Reference Noakes, Vlachava and Kremmyda 33 ) may have had an impact on the success and viability of the initial colonisers of the gut, having made the environment more favourable for some species of bacteria. It has been found that when PUFA are added to intestinal epithelial cells, they are incorporated into the membrane phospholipid fraction and improve barrier integrity( Reference Willemsen, Koetsier and Balvers 34 ), and higher concentrations of PUFA have been reported to alter bacterial adhesion sites( Reference Kankaanpaa, Salminen and Isolauri 35 ). There is recent evidence to suggest that microbiological exposure can take place in utero ( Reference Rautava, Collado and Salminen 14 , Reference Satokari, Gronroos and Laitinen 36 , Reference Jimenez, Marin and Martin 37 ). Since the effect of intervention is significant in the exclusively FF infants, this may, in part, be because breast-feeding per se and the associated predominant bifidus microbiota are influenced to a lesser degree by salmon intervention and to a greater degree by formula feeding. There is also the possibility that the differences between the FF infants in the control and salmon groups were due to differences in the types of infant formulae consumed, since formulae with and without supplementation of prebiotics (galacto-oligosaccharide and fructo-oligosaccharide) can influence the microbiota( Reference Fanaro, Boehm and Garssen 38 ). However, the records of infant feeding practices during the first 6 months post-partum show that there was no significant difference in their usage between the control and intervention groups( Reference Vlachava 26 ).
Faecal sIgA has a role in mucosal immunity, protecting the gastrointestinal tract from pathogenic invasion. The production of sIgA in infants is limited during the first month post-partum( Reference Brandtzaeg, Nilssen and Rognum 39 ), but the BF infants benefit from the supply in their mothers' milk( Reference Hanson 40 ). Breast milk also contains prebiotic oligosaccharides that pass undigested into the lower gastrointestinal tract( Reference Chaturvedi, Warren and Buescher 41 ) and may also provide protection against potential pathogens( Reference Newburg, Ruiz-Palacios and Morrow 42 ). Infants fed a formula supplemented with a blend of galacto-oligosaccharide and fructo-oligosaccharide have been found to have higher concentrations of sIgA in their faeces than those consuming an unsupplemented formula( Reference Scholtens, Alliet and Raes 43 , Reference Bakker-Zierikzee, Tol and Kroes 44 ). In the present study, sIgA concentrations in the faeces of the exclusively BF infants were higher than those in the faeces of the exclusively FF infants throughout the study period. However, the concentrations declined over time in the BF infants, perhaps reflecting the reduction in breast milk sIgA concentrations( Reference Urwin, Miles and Noakes 24 ). In contrast, in FF infants, faecal sIgA concentrations increased with time, perhaps reflecting the initiation of the infants' own production. Faecal calprotectin is a non-specific biomarker indicating the presence of intestinal inflammation( Reference Costa, Mumolo and Bellini 45 ). Concentrations in healthy adults and school-aged children are typically < 50 mg/kg faeces, whereas those in infants are much higher( Reference Olafsdottir, Aksnes and Fluge 46 ). The median faecal calprotectin concentration of the infants in the present study during the first 84 d post-partum was 146 mg/kg, range 13–743 mg/kg (data not shown), which is in agreement with previous findings( Reference Olafsdottir, Aksnes and Fluge 46 ). We found no significant effect of mode of infant feeding on faecal calprotectin concentrations, which is in agreement with the findings of some studies( Reference Rosti, Braga and Fulcieri 47 ), but not with those of others, where elevated concentrations have been reported in exclusively BF infants( Reference Savino, Castagno and Calabrese 48 ). The faecal calprotectin concentrations of the BF infants were higher than those of the FF infants, but large inter-individual variation and limited sample size possibly limited our ability to detect a significant difference. There was also no effect of salmon intervention on faecal calprotectin. A pilot study of patients with inflammatory bowel disease supplemented with seal oil, a source of n-3 PUFA, found a decrease in disease activity, but faecal calprotectin concentrations were found to be unchanged( Reference Arslan, Brunborg and Froyland 49 ).
Salmon consumption during pregnancy did not have a significant effect on maternal faecal microbiota composition. There was no baseline sample before intervention, and therefore, it was not possible to determine any longitudinal effects due to pregnancy itself, but only possible to compare the control and intervention groups at 38 weeks of gestation. There have been recent reports on maternal faecal microbiota during pregnancy and, in particular, the effects of maternal BMI and weight gain( Reference Santacruz, Collado and Garcia-Valdes 50 , Reference Collado, Isolauri and Laitinen 51 ). It is, therefore, possible that the effects of pregnancy combined with the large inter-individual variation outweighed any effect of increased salmon consumption. When the women were divided according to pregnancy weight gain, there were higher numbers of total bacteria and bacteria of the F. prausnitzii group in those with a normal weight gain than in those with an excessive weight gain, irrespective of the intervention group. There was also a trend towards higher numbers of bacteria of the Bifidobacterium genus and the Atopobium cluster in women showing a normal weight gain. These findings concur with those of previous studies, which also reported trends towards higher numbers of Bifidobacterium in the faeces of women with a normal weight gain in pregnancy( Reference Santacruz, Collado and Garcia-Valdes 50 ). Salmon also had no effect on maternal faecal sIgA or calprotectin concentrations. The faecal calprotectin concentrations of all the mothers were below the upper limit for healthy adults ( < 50 mg/kg faeces).
In conclusion, salmon consumption during pregnancy had no significant effect on the maternal microbiota, but it is possible that the effect of dietary intervention was obscured by changes associated with pregnancy per se, including the effect of maternal weight gain. Salmon consumption was associated with a trend towards lower bacterial counts of the Atopobium cluster in the faeces of infants during the first 84 d post-partum, especially those who were not exclusively BF. Although this group of bacteria comprises a small proportion of the total microbiota during the early stages post-partum, this is a critical time for the development and maturation of the immune system of infants, and the study demonstrates the potential of maternal diet during both pregnancy and lactation to influence the infant gut microbiota. This should be explored further.
Acknowledgements
The authors thank the subjects and their families and the staff of the Medical Research Council Lifecourse Epidemiology Unit and the Princess Anne Hospital for their assistance in carrying out the SiPS. They also thank Dr Lesley Hoyles for training in the analysis of faecal samples with the use of fluorescence in situ hybridisation and Professor Sue Todd for statistical advice. P. C. C. and K. M. G. are supported by the National Institute for Health Research through the NIHR Southampton Biomedical Research Centre.
The present study was supported by the European Commission under Framework 6: sustainable aquafeeds to maximise the health benefits of farmed fish for consumers (Aquamax: FOOD-CT-2006-16 249).
The funding body had no role in the design and analysis of the study or in the writing of this article.
The authors' contributions were as follows: E. A. M., K. M. G. and P. C. C. were responsible for designing the study and P. C. C. had overall responsibility for all aspects of the study; P. S. N., M. V., L.-S. K. and N. D. D. recruited and screened the volunteers, carried out the intervention and collected all the samples; H. J. U. carried out the laboratory analyses, supervised by P. Y. and J. V.; H. J. U. conducted the statistical analyses and drafted the manuscript; all authors contributed to and approved the final version of the manuscript.
P. C. C. serves on the Danone Scientific Advisory Board on Immunity and Allergy and on the Syral Scientific Advisory Board; acts as a consultant to the Danone Research Centre for Specialised Nutrition, Mead Johnson Nutritionals, Christian Hansen and Beneo-Orafti; has received speaking honoraria from Abbott Nutrition and Nestlé; and is currently receiving research funding from Abbott Nutrition and Beghin-Meiji. K. M. G. has acted as a consultant to Abbott Nutrition and Nestlé Nutrition and has received reimbursement for speaking at an Abbott Nutrition Conference on Pregnancy Nutrition and Later Health Outcomes and at a Nestlé Nutrition Institute Workshop. He is part of an academic consortium that has received research funding from Abbott Nutrition, Nestlé and Danone. J. V. is an employee of Clasado Research Services. P. Y. has received research funding from Cereal Partners UK. None of the other authors has any potential conflicts of interest.