Osteoporosis is a major cause of morbidity and mortality worldwide, principally due to its association with fractures(Reference Torgerson, Iglesias, Reid, Barlow, Francis and Miles1). Evidence that factors operating early in life may programme later bone mass and geometry and, perhaps, later osteoporosis risk has led to an increased focus on measures that may optimise the accretion of bone mass during fetal life, infancy and childhood(Reference Fewtrell, Lucas and Sampson2). Little research has focused specifically on the effect of infant diet, particularly in healthy term infants. In one prospective cohort study, higher bone mass was found at age 8 years in children previously breast-fed compared with those who had been formula-fed(Reference Jones, Riley and Dwyer3). More recently, we reported an association between neonatal intake of human milk in preterm infants and whole-body bone mass in early adult life(Reference Fewtrell, Williams and Singhal4).
Although the possibility of residual confounding remains in these observational studies, beneficial effects of breast milk on bone could result from one of the many growth factors or hormones present in human milk, or from differences in macro- or micronutrient content and bioavailability between human milk and infant formulas. Another plausible mechanism relates to structural differences in TAG in breast milk and infant formula. Palmitate constitutes about one-quarter of the fatty acids in human milk, with 70 % in the sn-2 (middle) position on the glycerol backbone. Most modern formulas contain a mixture of animal and vegetable oils, adjusted to mimic the pattern of fatty acid saturation and chain lengths found in breast milk. However, such fat mixtures have a reduced content of fatty acids esterified to glycerol in the sn-2 position and an increase in those esterified in the sn-1 and sn-3 positions. The latter undergo hydrolysis in the gut, releasing palmitic acid, which is poorly absorbed and tends to form Ca soaps; this may be partly responsible for the harder stools seen in formula-fed infants, and could influence Ca absorption.
To address this problem in formulas, two approaches have been used: reducing the palmitate content, or using a modified synthetic fat blend (‘Betapol’) with a high proportion of fatty acids esterified in the sn-2 position to mimic that found in human milk. A recent review identified nine publications in which the use of ‘standard’ preterm or term infant formulas was compared with either low-palmitate formulas or formulas containing a modified ‘high-sn-2’ TAG(Reference Koo, Hockman and Dow5). Standardised results from these studies were consistently positive in favour of the low/synthetic palmitate groups with respect to intestinal fractional absorption of fat, palmitic acid and Ca. Total body bone mass was also significantly higher in two studies(Reference Koo, Hammami and Margeson6, Reference Kennedy, Fewtrell and Morley7), including our own randomised trial using a formula containing a high-sn-2 fat blend, in which whole-body bone mass at 12 weeks of age was similar in the intervention group to that of the breast-fed reference group(Reference Kennedy, Fewtrell and Morley7). These results suggest that increased Ca absorption results in measurable biological effects in the short term. However, it is not known whether such benefits persist, resulting in long-term improvements in bone mass which could have implications for later osteoporosis risk.
To investigate whether the type of infant feeding influences later bone mass, we performed a 10-year follow-up of subjects born at term and either breast-fed or randomised to use a standard formula or an experimental high-sn-2 infant formula for the first 12 weeks of life.
Methods
Original randomised trial: subjects and trial design
Full details of the original trial have been given elsewhere(Reference Kennedy, Fewtrell and Morley7); a summary is provided here. A total of 203 formula-feeding infants were recruited from the Rosie Maternity Hospital in Cambridge, UK. Subjects were term infants ( ≥ 37 weeks of gestation) with birth weight >5th percentile. They were enrolled within the first 8 d, after the mother had decided on her chosen mode of feeding and had started either breast- or formula feeding, and randomised to receive one of two formulas using a double-blind random permuted block allocation with dietary assignments identified by a barcode. A reference group of 120 term breast-fed infants with birth weights >5th percentile were also recruited. The mothers of these infants were approached soon after birth and re-contacted at 10 weeks; if still being exclusively breast-fed, they were invited to join the study. Subjects completed the study at 12 weeks. All infants completed the study at 12 weeks. Ethics approval was obtained from the Cambridge Health Authority and local Medical Research Council ethics committees. Parents gave written informed consent.
Trial diets
Both formulas were supplied by Nutricia and had similar concentrations of palmitate; however, the experimental formula with synthetic TAG (‘Betapol’, high-sn-2 formula; Loders Croklaan b.v) contained 50 % of palmitate in the sn-2 position compared with 12 % in the control formula. The Betapol fat blend was prepared by enzymatic interesterification of fractionated palm oil with fatty acids derived from high-oleic sunflower oil. Formula packaging was identical except for differences in the barcodes; investigators and parents were blinded to the dietary allocation.
Main outcomes in the initial trial were bone mineral content (BMC) at 12 weeks of age measured by single-photon absorptiometry and dual-energy X-ray absorptiometry, and stool characteristics.
Follow-up at age 10 years
All subjects from the original cohort who could be traced were invited to participate in the follow-up study. Those willing to do so attended a research clinic in Cambridge (Wellcome Trust Clinical Research Facility; n 57) or London (Institute of Child Health; n 31). Written informed consent was obtained from the parent or guardian and assent from the child. The study was conducted according to the guidelines laid down by the Declaration of Helsinki, and all procedures involving human subjects were approved by the Eastern Multi-Centre Research Ethics Committee.
Weight was measured using digital scales and height using a wall-mounted stadiometer. Weight and height standard deviation scores (SDS) were calculated using UK population reference data. A FFQ quantified current Ca intake (Calquest(Reference Nelson, Hague and Cooper8)); a simple questionnaire determined hours of weight-bearing activity/week; and parents rated the child's activity level compared with his peers (rated 1–5: 1 = much less active; 5 = much more active). A general medical and fracture history was taken. Maternal and paternal height was reported by the mother.
Bone densitometry
Dual-energy X-ray absorptiometry (software version 8.1; Lunar Prodigy) was used to measure BMC, bone area (BA) and bone mineral density (BMD) at the lumbar spine (LS, L2–L4) and whole body. Subjects wore light indoor clothing after removing metal objects. Total radiation exposure was below daily background levels (approximately 7 μSv/d in the UK). As recommended by the International Society for Clinical Densitometry(Reference Bishop, Braillon and Burnham9), we used ‘total body less head’ values for whole-body scans. The same make and model of the dual-energy X-ray absorptiometry scanner was used in both study sites and machines were cross-calibrated using the European Spine Phantom.
Statistics
In view of the significant cohort attrition, anthropometric and bone densitometry data were compared between the breast-fed reference group and the formula-fed groups using ANOVA or χ2 as appropriate with variables transformed where necessary to ensure a normal distribution. Where a significant between-group effect was identified using ANOVA, post hoc pairwise comparisons were performed using the Bonferroni test. We adopted this approach rather than comparing previously randomised groups using the t test as our primary analysis, in view of the significant cohort attrition experienced (follow-up rate ≤ 30 %). Bone mass was adjusted for size in three ways: (1) bone mineral apparent density (BMAD) of the LS, calculated as BMC/BA1·5. BMAD SDS were calculated for age, sex and ethnic group using UK machine-specific reference data(Reference Crabtree, Oldroyal and Truscott10); (2) for whole-body bone mass, a two-stage procedure was used. The indices lean/height1·5 and BMC/lean1·2 were calculated using the power relationships required to remove any residual association with height determined using log–log regression; (3) multiple regression was used first to examine the effect of infant diet on later bone mass at skeletal sites after adjusting for age, sex, pubertal stage and body size (weight and height), and, second, to adjust for potential confounding factors, including current physical activity and Ca intake.
Results
At follow-up, thirty-four breast-fed subjects and fifty-seven formula-fed subjects were seen (thirty previously randomised to the high-sn-2 formula and twenty-seven to the control formula). The overall follow-up rate was 28, 30 and 26 % of subjects enrolled for the breast-fed, high-sn-2 and control groups, respectively, with corresponding rates of 31, 35 and 31 % for infants seen at 12 weeks of age (Fig. 1). Reasons for non-participation for the remaining subjects were as follows: declined to take part (n 18); did not reply to two invitation letters (n 139); agreed to take part but did not attend appointments (n 24); moved abroad (n 10); general practitioner advised contact would not be appropriate (n 6); not traceable (n 35). There were no significant differences in baseline characteristics or outcome measures at 12-week follow-up between the formula-fed or breast-fed subjects seen or not seen at the 10-year follow-up (Table 1). At follow-up, one child from the breast-fed group, four from the high-sn-2 group and two from the control group were reported to have asthma treated with inhaled bronchodilators; four of these children (one breast-fed, two high sn-2 and one control) also used inhaled corticosteroids. Also, eight previously breast-fed children and three formula-fed children (one high-sn-2 and two controls) were reported to be taking a multivitamin supplement including vitamin D. Estimated daily Ca intake and time spent in vigorous physical activity were not significantly different between the groups. A significantly greater proportion of the breast-fed group came from higher socio-economic backgrounds.
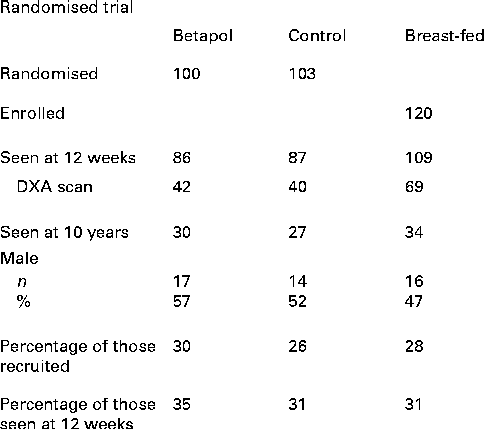
Fig. 1 Study flow chart. DXA, dual-energy X-ray absorptiometry.
Table 1 Baseline characteristics for the formula-fed and breast-fed subjects seen v. not seen at 10 years (Mean values and standard deviations)
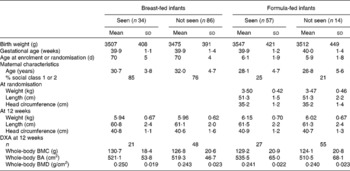
DXA, dual-energy X-ray absorptiometry; BMC, bone mineral content; BA, bone area; BMD, bone mineral density.
The breast-fed group was significantly older when seen for follow-up compared with both formula-fed groups (post hoc P= 0·005 for breast-feeding (BF) v. high sn-2; P ≤0·001 for BF v. control) and a lower proportion were pre-pubertal (32 v. 60 % for the high sn-2 group and 67 % for the control group; P= 0·02). However, the control formula subjects had significantly higher weight SDS than the breast-fed subjects (post hoc P= 0·041), with intermediate values for the high-sn-2 group (post hoc P= 0·6 v. BF group). Although there was a trend towards higher LS BMD SDS in the high-sn-2 group compared with the breast-fed reference group (post hoc P= 0·06), the BMAD SDS did not differ significantly between the breast-fed and formula-fed groups (Table 2). Furthermore, in models with adjustment for body size (weight and height), sex, pubertal status (pre-pubertal v. pubertal) and BA, there were no significant differences in bone mass at any skeletal site between the dietary groups, nor any significant interaction between sex and milk group or pubertal status and milk group. Significant predictors in these models were BA for LS BMC, and BA, height and sex for total body BMC. There were no significant differences between the groups in the number of children who reported a previous fracture, nor in estimated current daily Ca intake.
Table 2 Anthropometry and bone densitometry data at baseline, during infancy and at 10-year follow-up according to infant diet group (Mean values and standard deviations)
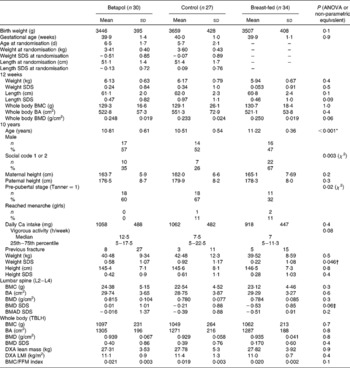
SDS, standard deviation score; BMC, bone mineral content; BA, bone area; BMD, bone mineral density; BMAD, bone mineral apparent density; TBLH, total body less head; DXA, dual-energy X-ray absorptiometry; LMI, lean mass index; FFM, fat-free mass.
* Post hoc Bonferroni: breast-fed v. Betapol, P= 0·005; breast-fed v. control, P< 0·001; Betapol v. control, P= 0·09.
† Post hoc Bonferroni: breast-fed v. Betapol, P= 0·6; breast-fed v. control, P= 0·04; Betapol v. control, P= 0·7.
‡ Post hoc Bonferroni: breast-fed v. Betapol, P= 0·06; breast-fed v. control, P= 0·5; Betapol v. control, P= 1·0.
Comparing data for the pooled formula-fed groups and the breast-fed group (Table 3), breast-fed children were significantly older with a significantly higher proportion in pubertal stage 2 or above (68 v. 37 %), but they were also significantly lighter (by 0·5 SDS, P= 0·03) with significantly lower LS BMD SDS (by 0·44, P= 0·03). However, size-adjusted LS bone mass (BMAD SDS) was not significantly different between the groups, and after adjusting for bone and body size and sex, there were no differences in whole-body or LS bone mass between the groups.
Table 3 Anthropometry and bone measurements for combined formula-fed subjects v. breast-fed subjects (Mean values and standard deviations)
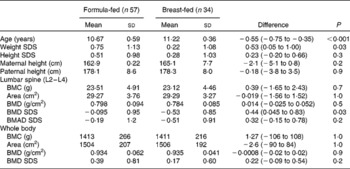
SDS, standard deviation score; BMC, bone mineral content; BMD, bone mineral density; BMAD, bone mineral apparent density.
Discussion
We previously reported that healthy breast-fed infants had bone mass similar to that of infants who received a high-sn-2 fat blend infant formula, and higher than that of infants fed a standard term formula(Reference Kennedy, Fewtrell and Morley7). At follow-up, the breast-fed reference group was significantly older and lighter, with a trend towards lower LS BMD z-scores. However, there were no significant differences in bone mass between the formula-fed and breast-fed subjects after adjusting for size. Only two previous studies have reported on the long-term effects of BF on the offspring's bone health. Jones et al. (Reference Jones, Riley and Dwyer3) studied 330 8-year-old children from a Tasmanian cohort and reported that those who were born at term and who had been breast-fed had significantly higher BMD at the femoral neck (sd +0·26), LS (sd +0·34) and whole body (sd +0·41) compared with those not breast-fed. This effect remained after adjusting for size, lifestyle and socio-economic factors, but was only seen in those breast-fed for more than 3 months. There was also some evidence for a ‘dose–response’ effect in that BMD was highest in subjects reported to breast-feed more frequently at night at age 3 months. Interestingly, the effect was not observed in preterm subjects from the same cohort. In contrast, we recently reported higher whole-body BA and BMC in young adults who were born preterm and received human milk, with an apparent ‘dose–response’ effect relating the proportion of human milk in the diet during the neonatal period to later bone outcomes(Reference Fewtrell, Williams and Singhal4). This effect was seen despite the inadequate nutrient content of human milk for preterm infants – in particular, the low Ca and phosphate content and the lack of nutrient or mineral supplementation of these infants. This effect was not observed at the 10-year follow-up of the same cohort. The reason for the discrepancy between the previous findings of a positive longer-term effect of BF on bone mass in term infants and those from the present study is not clear but could relate to the duration or ‘dose’ of BF. We do not have reliable data on the duration or exclusivity of BF for the present subjects. To be enrolled in the breast-fed reference group, infants had to be exclusively breast-fed at 10 weeks of age; however, given BF practices in the UK at the time, it is unlikely that exclusive or predominant BF was continued much beyond 3 months in most of the subjects. Another possibility is that the lack of an observed effect of BF in the present cohort reflects the small sample size; this seems less plausible as an explanation given that, if anything, the bone mass was lower in the breast-fed group.
In our original publication from this randomised trial, we also reported that use of a term infant formula containing a modified fat blend with a higher proportion of palmitate in the sn-2 position as found in breast milk, designed to improve Ca absorption, resulted in higher whole-body bone mass at the time of the intervention(Reference Kennedy, Fewtrell and Morley7). Our hypothesis that this beneficial effect would persist beyond the intervention period was not confirmed by the present follow-up study; we found no evidence of a persisting effect on bone mass in mid-childhood. Children previously assigned to the high-sn-2 formula had measures of bone mass that were slightly higher than children from the control group, with a difference of 0·22 SDS for LS BMD and 0·37 SDS for LS BMAD. Children from this group were also slightly smaller than controls, both in infancy and at follow-up; nevertheless, bone mass adjusted for size using three different methods was not significantly different between the groups. It is important to note that, although the present study was a follow-up of a randomised trial, and the primary analysis would therefore normally be a comparison of randomised groups, in view of the significant attrition experienced (less than 30 % follow-up overall) which may to some extent invalidate some of the benefits of random assignment(Reference Fewtrell, Kennedy and Singhal11), we adopted a conservative approach and examined the associations between early diet and later bone outcomes in a three-way ANOVA with subsequent post hoc pairwise comparisons performed only if a significant main effect was identified.
Although possibly a reflection of our relatively small sample size, given the results of trials of Ca or mineral supplementation during infancy and childhood, the absence of a sizeable long-term effect of the high-sn-2 formula on bone mass is perhaps not surprising. In a randomised trial comparing infant formulas with varying Ca and P contents in healthy term infants, together with a breast-fed reference group, Specker et al. (Reference Specker, Beck and Kalkwarf12) reported transient effects during the first 6 months, with higher bone mineralisation in infants consuming a higher mineral formula; however, by 12 months of age, there were no differences in bone mass among the different feeding groups. It is not clear whether a transient increase in bone mineralisation, such as that reported by Specker, and during the intervention phase of the present trial, would have any short-term benefit for a healthy term infant with low fracture risk. Winsenberg et al. (13) performed a meta-analysis of nineteen studies of Ca supplementation in children aged 3 years and above, with bone outcomes measured after at least 6 months of follow-up. Ca supplementation had no effect on LS or hip BMD. A small and transient effect was observed for whole-body BMC, and this has been postulated to result from a temporary reduction in bone remodelling. A small persisting effect was seen at the upper limb; however, this was considered to be unlikely to reduce the risk of fracture either in childhood or later life, and therefore of little public health significance.
Conclusion
In the present cohort of healthy term-born subjects, we found no difference in childhood bone mass between subjects who had been breast-fed or formula-fed, and no persisting beneficial effect of an infant formula with a high-sn-2-modified fat blend, designed to increase Ca absorption. The present finding is consistent with trials of Ca or mineral supplementation during infancy and childhood, and suggests that the major beneficial effect of the addition of this modified fat blend to infant formulas may be the observed alteration in stool characteristics seen during infancy. However, both findings must be interpreted in the context of the relatively small sample size, which means that we cannot exclude a difference of < 0·7 sd between the groups. A larger study would be required to exclude smaller, but potentially biologically relevant effects.
Acknowledgements
We thank the children and parents who participated in the study; Penny Lucas, Elaine Marriott and Emma Sutton for help with tracing and data collection. The project was funded by a grant from the Medical Research Council (MRC) UK and from the European Union (EU) FP-6 Early Nutrition Programming Project (Food-CT-2005-007036). Funding for the original randomised trial was provided by a research grant from Nutricia UK. M. S. F. designed and was principal investigator (PI) for the present follow-up study, analysed the data and wrote the manuscript; K. K. assisted with the design of the study and was responsible for the database management; P. R. M. was chief investigator for the Cambridge part of the follow-up study; J. E. W. and S. C. assisted with the design of the study and was responsible for the conduct of the study in London; A. L. was PI for the original randomised trial and contributed to the concepts for the follow-up study. All authors contributed intellectually to the writing and revision of the manuscript. The authors have no conflicts of interest to declare with respect to this manuscript. This submission represents original work that has not been published previously. It is not currently being considered by another journal, and if accepted for the British Journal of Nutrition it will not be published elsewhere in the same form, in English or in any other language, without the written consent of the Nutrition Society. Each author has seen and approved the contents of the submitted manuscript.