Introduction
Crustacean cuticle is an extracellular matrix secreted by epidermal and gut ectodermal cells. The structure and function of the cuticles has been studied extensively, and its structural and compositional features have been described in different body parts of adults and during development in different crustacean groups (Storch & Štrus, Reference Storch and Štrus1989; Strus & Storch, Reference Strus, Storch, Juchault and Mocquard1991; Wägele, Reference Wägele, Harrison and Humes1992; Strus et al., Reference Strus, Drobne, Licar and Alikhan1995; Ziegler, Reference Ziegler1997; Žnidaršič et al., Reference Žnidaršič, Tušek-Žnidarič, Strus, Matsko, Letofsky-Papst, Grogger and Hofer2010; Luquet, Reference Luquet2012; Dillaman et al., Reference Dillaman, Roer, Shafer, Modla, Watling and Thiel2013; Mrak et al., Reference Mrak, Žnidaršič, Tušek-Žnidarič, Klepal, Gruber and Strus2014; Roer et al., Reference Roer, Abehsera and Sagi2015). Cuticle is a complex mineralized chitinous-proteinaceous matrix with a multilayered structure. In crustaceans it is constantly being remodelled by exuviation during reproduction, development and growth. Several studies describe cuticle structure in terrestrial isopods during embryonic development and during growth in adults. They mostly focus on time-dependent events of cuticle secretion during development of embryos and larvae (Wolff, Reference Wolff2009; Milatovič et al., Reference Milatovič, Kostanjšek and Strus2010; Žnidaršič et al., Reference Žnidaršič, Mrak, Tušek-Žnidarič and Štrus2012; Mrak et al., Reference Mrak, Žnidaršič, Tušek-Žnidarič, Klepal, Gruber and Strus2014, Reference Mrak, Bogataj, Štrus and Žnidaršič2017) or on cuticle structure and biomineralization during moult cycle (Ziegler, Reference Ziegler1997; Hild et al., Reference Hild, Marti and Ziegler2008; Ziegler et al., Reference Ziegler, Neues, Janáček, Beckmann and Epple2017; Žnidaršič et al., Reference Žnidaršič, Mrak, Rajh, Žagar Soderžnik, Čeh and Štrus2018). There are also several reports about terrestrial isopod cuticle structure and function from the viewpoint of adaptation to lifestyles in different habitats, mostly with regard to epicuticle ornamentation, number and thickness of cuticular lamellae and intensity of mineralization (Schmalfuss, Reference Schmalfuss, Sutton and Holdich1984; Hild et al., Reference Hild, Neues, Žnidaršič, Štrus, Epple, Marti and Ziegler2009; Seidl et al., Reference Seidl, Huemer, Neues, Hild, Epple and Ziegler2011; Hornung, Reference Hornung2011; Luquet, Reference Luquet2012; Vittori & Štrus, Reference Vittori and Štrus2014; Wood et al., Reference Wood, Kostanjšek, Araujo and Štrus2017).
Amphibious sea slaters Ligia pallasii Brandt, 1833 are very convenient for studies of cuticle structure and formation due to frequent biphasic moulting and poorly mineralized exocuticle in contrast to crab cuticle. Cuticle of sea slaters is an important surface barrier, which prevents ion and water loss and enables mobility and communication in the terrestrial environment. Its structural and functional adaptations show features related to transition from marine to land habitats (Carefoot & Taylor, Reference Carefoot, Taylor and Alikhan1995; Strus & Compere, Reference Strus and Compere1996; Strus & Blejec, Reference Strus, Blejec, Kensley and Brusca2001). Cuticle of strictly terrestrial woodlice is well described and its structure reflects specific adaptations to different land lifestyles (Hornung, Reference Hornung2011; Seidl & Ziegler, Reference Seidl and Ziegler2012; Vittori et al., Reference Vittori, Kostanjšek, Žnidaršič and Strus2012, Reference Vittori, Tušek-Žnidarič and Štrus2017). Imaging of cuticle is a demanding procedure, due to its compositional and structural instability during the moult cycle. Adequate preparation of tissues that secrete cuticle is of utmost importance for preservation of structural and chemical components. Microscopic structure of crustacean cuticles at different resolution levels is presented in various papers mostly focused on studies of presence and distribution of biominerals in the extensive chitinous-proteinaceous matrix (Dillaman et al., Reference Dillaman, Hequembourg and Gay2005; Ziegler et al., Reference Ziegler, Hagedorn, Ahearn and Carefoot2006; Luquet, Reference Luquet2012). Histological methods, fluorescence microscopy with acridine orange and electron microscopy combined with various analytical techniques are the most frequent approaches in studies of cuticle structure and composition (Marlowe et al., Reference Marlowe, Dillaman and Roer1994). A new approach of mineralized cuticle visualization in moulting terrestrial isopod Porcellio scaber with SR micro CT shows that different parts of exoskeletal elements are involved at varying extents in mineral recycling during the moult cycle (Ziegler et al., Reference Ziegler, Neues, Janáček, Beckmann and Epple2017).
In this paper we introduce X-ray microtomography (micro CT) and transmission electron microscopy of wheat germ agglutinin (WGA) lectin gold labelled Tokuyasu thawed cryosections for investigation of chemical composition of different cuticular layers. The aim of this contribution is visualization of surface epithelia and cuticle which they secrete during the moult cycle, using a non-invasive method of micro CT and electron microscopy of cryosections. Structural and compositional dynamics of cuticular layers are presented during three different phases of the moult cycle in Ligia pallasii and discussed based on known data about cuticle secretion and mineralization in terrestrial isopods.
Materials and methods
Experimental animals
Specimens of Ligia pallasii Brandt, 1833 (Crustacea: Isopoda: Oniscidea) from the US North Pacific coast were collected at Reuben Tart, Eagle Cove and Lime Kiln on San Juan Island, Washington State, USA in 2016. They were kept individually in glass containers, fed with fish food and local weeds and inspected twice daily to determine the phase of the moult cycle. The different phases of the moult cycle, namely premoult, intramoult and postmoult, were determined according to the described features for the moulting in terrestrial isopods (Zidar et al., Reference Zidar, Drobne and Štrus1998). The size, sex and feeding status of specimens was determined prior to fixation.
Sample preparation for computer tomography (CT) scanning
Animals were fixed in 96% ethanol or Dent's fixative (Metscher, Reference Metscher2009) and transferred to 1% lugol iodine. They were wrapped in alcohol-saturated cheesecloth, installed in plastic cylinders and scanned at 60 KV and 100 µA and a voxel resolution of 35.5 µm with a Brucker Skyscan 1173 at the Karl F. Liem Bioimaging Center at Friday Harbor Labs. The images were reconstructed and visualized with Amira (Thermo Fisher Scientific).
Sample preparation for electron microscopy
Animals were dissected and dorsal parts of the anterior exoskeleton (tergites 3, 4) were fixed in 2% glutaraldehyde and 2% paraformaldehyde in 0.1 M HEPES buffer (Fixative 1) for a structural analysis using a Hitachi S-4800 Field Emission Scanning Electron Microscope. Samples were prepared as described before (Vittori & Štrus, Reference Vittori and Štrus2014). Alternatively, samples were fixed in 4% paraformaldehyde in 0.1 M HEPES buffer (fixative 2) for chitin and protein localization with TEM using Tokuyasu technique for sample preparation. For this, tergites were embedded in 12% bovine gelatine and infiltrated with 2.3 M sucrose before they were cut into smaller pieces, which were additionally infiltrated with sucrose for 24 h. Samples were mounted on metal pins and snap frozen in liquid nitrogen for cryo-sectioning in a Leica EM UC7 ultramicrotome. Sections (80–100 nm) were thawed and transferred to carbon- and formvar-coated copper grids. For immunogold labelling Wheat Germ Agglutinin (WGA)-biotin (Vector Laboratories) was used at 1:300 dilution, followed by rabbit anti-biotin (Abcam) at 1:300 and protein A gold-10 nm (CMC UMC Utrecht) at 1:50, for 30 min. Sections were then embedded in 1.8% methyl cellulose with 0.2% uranyl acetate and examined with a JEM1400 transmission electron microscope (JEOL). Images were taken with a TemCam-F216 using EM-MENU software (Tvips).
Results
Moult cycle and cuticle morphology in Li gia pallasii
Frequent moults in adult non-breeding animals enable growth and regeneration of injured body parts. Appearance of milky white sternal calcium deposits in the ecdysial gap between old and newly secreted cuticles of the four anterior sternites is the first obvious sign of premoult. When white deposits fully cover the sternal part of the animal, extending to the bases of pereopods, the exuviation begins. Biphasic moult in terrestrial isopods starts by shedding first the posterior half of the exoskeleton, followed by ecdysis of the anterior half. Intramoult animals with cast posterior exoskeleton (Figure 1A) can be recognized by a soft greyish new cuticle of the posterior part and a detached old yellowish cuticle of the anterior part. After exuviation of the anterior half of the exoskeleton a thin greyish new cuticle can be observed in postmoult animals (Figure 1B).
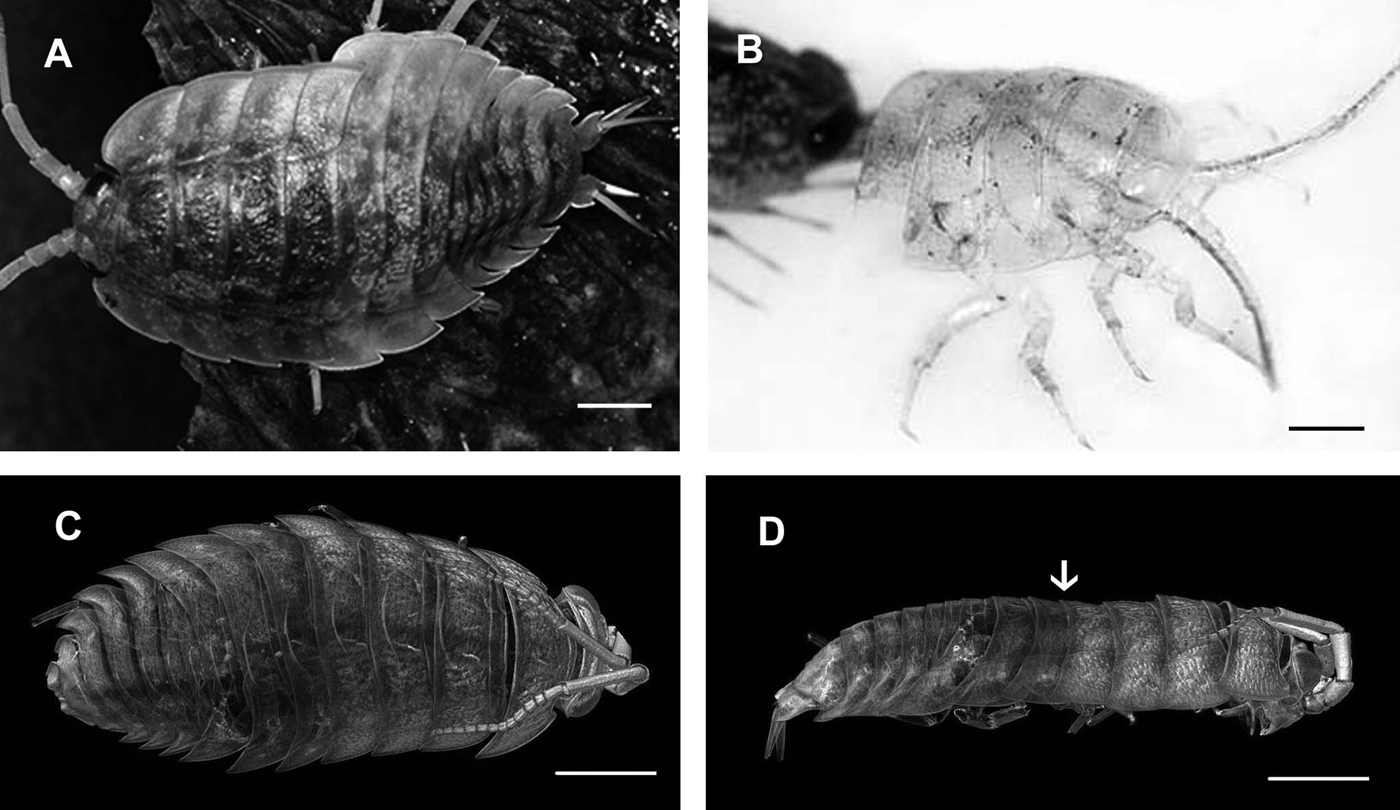
Fig. 1. Light micrographs and micro CT of moulting sea slater Ligia pallasii.(A) Intramoult animal with shed posterior half of the exoskeleton. (B) Front part of the postmoult animal with shed anterior half of the exoskeleton. (C) Dorsal view of an intramoult animal with shed posterior half of the exoskeleton, showing progressive resorption of mineral from the anterior half of the old cuticle in posterior-anterior direction. (D) Lateral view of the intramoult animal with mineral prevailing in the head with antennae and mouthparts. The separation line between anterior and posterior half of the body is marked by an arrow. Scale bars: 5 mm.
Micro CT of animals in different stages of the moult cycle gives useful information on cuticle surface structure and intensity of exoskeleton mineralization in different body regions during the moult cycle. The method is non-destructive and enables virtual sectioning without time-consuming preparation of histological or ultrathin sections. In combination with SEM it can offer a thorough morphological description of cuticular surface during different phases of the moult cycle. Premoult and intramoult animals can be easily distinguished from postmoult animals based on the mineral distribution in different parts of the exoskeleton. Dorsal and side views of the sea slater in intramoult phase with the shed posterior half of the cuticle, show differences in the distribution of minerals in the anterior and posterior half of the exoskeleton (Figure 1C, D). In the anterior half of the exoskeleton a progressive resorption of mineral from the fourth to the anteriormost pereonite can be seen with the highest concentration of the mineral in the head capsule with antennae and mouthparts.
Scanning electron microscopy of the dorsal surface of the third pereon tergite in intramoult animals shows numerous cuticular scales arranged in a shingle-like pattern and regularly arranged artichoke-like structures (Figure 2A). Cuticle of postmoult animals is composed of different layers, namely epicuticle with three sublayers of horizontally and vertically arranged components, exocuticle with a distinct plywood arrangement of proteic-chitinous fibres and innermost endocuticle with a lamellar structure (Figure 2B). Artichoke-like structures are tricorn sensilla encircled by lifted wedge-shaped scales with curved tips (Figure 2C, D). Rounded scales (Figure 2E) and wedge-shaped scales (Figure 2F) of premoult and intramoult animals are surrounded by a dense nanotubular network mostly at their bases. After exuviation of the anterior cuticle, animals enter the postmoult period and the new cuticle is fully elaborated and mineralized in intermoult animals. The duration of the intermoult stage is difficult to define morphologically, as the apolysis, separation of the old cuticle and formation of the new cuticle starts during early premoult, just several days after exuviation of the anterior exoskeleton.

Fig. 2. SEM micrographs of epicuticular surface in intramoult Ligia pallasii Brandt, 1833. (A) Epicuticular surface of the third pereon tergite with a lateral border to the right; scale bar: 250 µm. (B) Vertical section through a layered new cuticle of the third pereon tergite in postmoult animal; epicuticle (Ep) is composed of distinct outer and inner layers, layers of exocuticle (Ex) composed of proteic-chitinous fibres and lamellae of the endocuticle (En) are arranged in a plywood pattern; scale bar: 4 µm. (C) An artichoke like structure is a tricorn sensillum (arrow), surrounded by lifted wedge-shaped scales; scale bar: 50 µm. (D) A tricorn-type sensillum with a pore forming structure and a socket. (E) Rounded epicuticular scales are arranged as shingles. (F) Wedge-shaped scales are surrounded by dense nanotubular network at their bases; scale bars D, E and F: 10 µm.
Cuticle ultrastructure and composition during moult cycle of Ligia pallasii
Cuticle is the primary barrier and communication surface between the crustacean body and the external environment. In its structural role the exoskeleton enables animal posture and movement, and its multi-functionality provides communication with the external environment through spines, sensory structures, colouration and epibionts. In terrestrial isopods of the genus Ligia which do not roll into a sphere like their Roly poly relatives, but run away to avoid danger, cuticle is thin and not intensely mineralized and has a low amorphous calcium carbonate/calcite ratio.
In premoult animals epidermal cells secrete new cuticle immediately after apolysis and long before the old cuticle is cast. Preecdysial cuticle is composed of epicuticle forming diversified scales and exocuticle with an extensive network of pore channels with cytoplasmic extensions of epithelial cells (Figure 3A, B, D). The very thin outer epicuticular layer is electron-lucent, the inner layer is perforated by numerous vertical channels (Figure 3C). A nanotubular network is very abundant at the scale bases and connected to the outer epicuticular layer (Figure 3E, F).
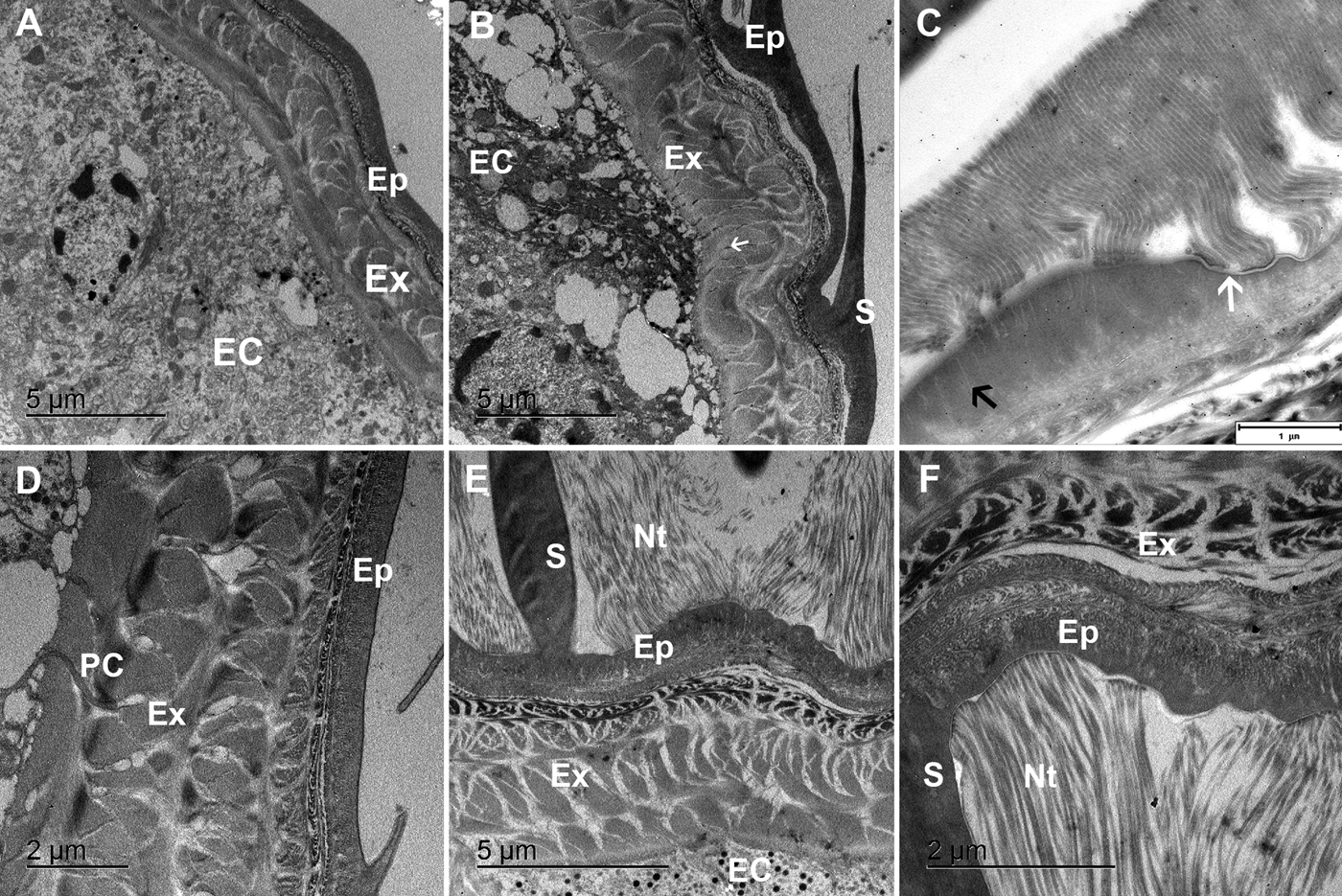
Fig. 3. TEM micrographs of the preecdysial cuticle in Ligia pallasii. (A) Epithelial cells (EC) secrete a new cuticle, composed of a layered epicuticle (Ep) and exocuticle (Ex) in early premoult. (B) Epicuticular scales (S) are formed and cuticle is perforated with numerous pore channels (arrow). (C) Nanotubules attached to the outer epicuticular layer (white arrow), are connected to thin vertical channels (black arrow) in the inner epicuticle. (D) Sublayers of the epicuticle (Ep) and exocuticle (Ex) are formed in intramoult animals and mineralization progresses by transport of components through pore channels (PC). (E) and (F) An extensive network of nanotubules (Nt) is attached to the outer epicuticle (Ep) around scales (S).
Bacteria are often present close to the scale armpits of epicuticular surface in intramoult animals and communicate with the network of nanotubules (Figure 4A, C). In late premoult and intramoult animals the ecdysial space between the old and new cuticle is filled with spherules, formed by the dissolution of the old cuticular layers and resorption of their components. Spherules and nanotubules are interconnected and attached to the epicuticular surface (Figure 4B). Nanotubules are extensive layers of spirally woven, rope-like structures which are distributed around epicuticular scales and tricorn sensillae in moulting animals (Figure 4D). Nanotubular network was visualized with TEM in (1) resin sections of tissue post-fixed with osmium tetroxide, contrasted with uranyl acetate and lead citrate and (2) in UA-contrasted thawed cryosections. Longitudinal and cross sections of the nanotubules with electron-dense outer and inner walls show that they have a diameter of 25–30 nm and contain electron dense material mostly at the contact sites with the epicuticle (Figure 4E, F).
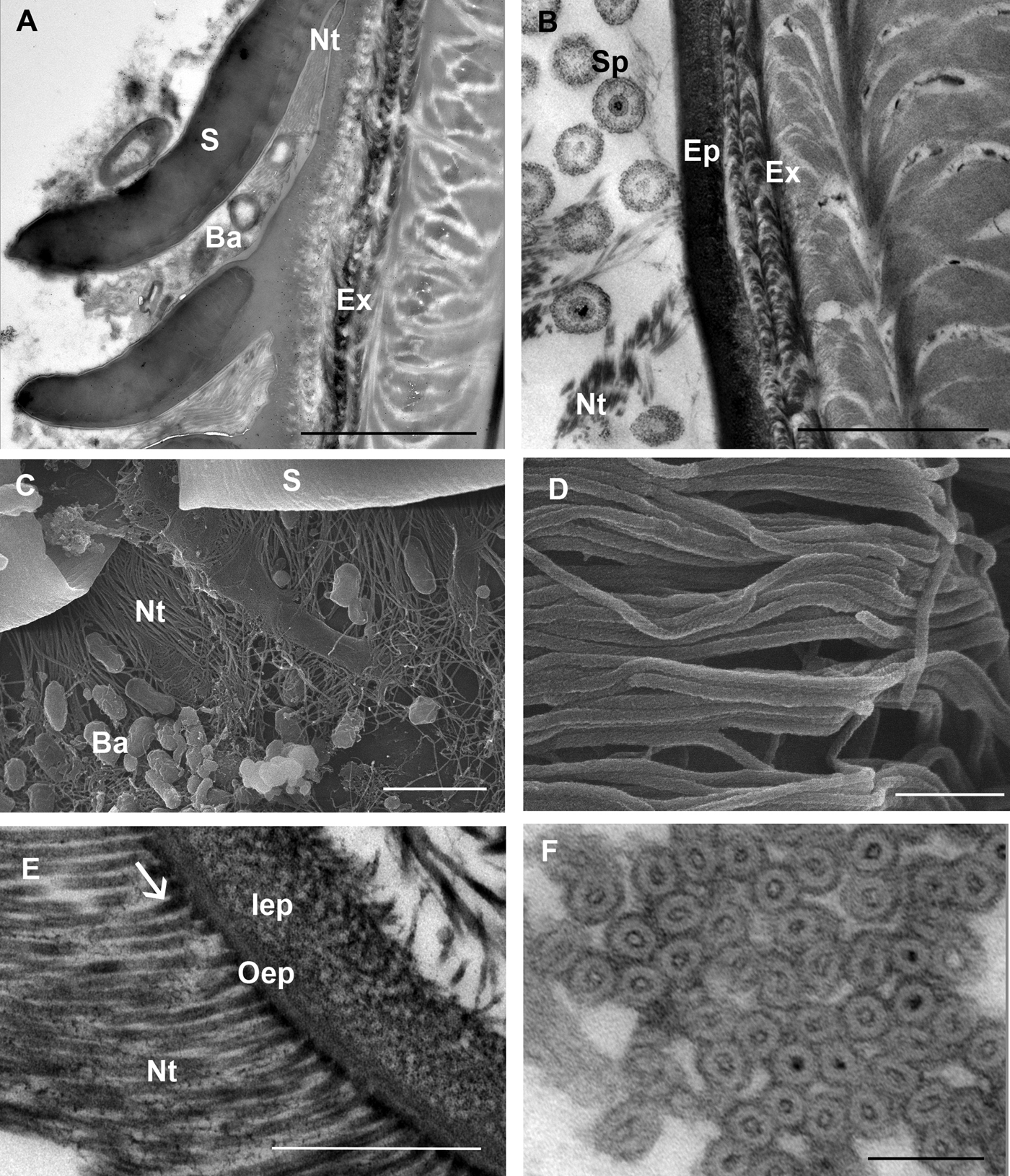
Fig. 4. SEM and TEM micrographs of a nanotubular network in the ecdysial space and at the cuticle surface in premoult and intramoult animals. (A) Bacteria (Ba) present in the matrix between epicuticular scales (S) and in the scale armpits are connected to nanotubules (Nt); scale bar: 1 µm. (B) Network of nanotubules (Nt) interconnects spherules (Sp) and epicuticle (Ep); exocuticle (Ex); scale bar: 2 µm. (C) Bacteria (Ba) and nanotubules (Nt) in the scale (S) armpits; scale bar: 2 µm. (D) SEM micrograph of rope-like nanotubules; scale bar: 250 nm. (E) Nanotubules with electron-dense material (arrow) at the cuticle surface; epicuticle is composed of outer (oEp) and inner epicuticular (iEp) layers; scale bar: 500 nm. (F) cross section of nanotubular bundles with electron-dense walls and material in the central part; scale bar: 100 nm. Sample preparation: (B, E) TEM of resin sections of tissue post-fixed with osmium tetroxide, contrasted with uranyl acetate and lead citrate (A, F) TEM of UA-contrasted thawed cryosections; (C, D) SEM of platinum coated tergites.
Postecdysial endocuticle is secreted in the posterior part of intramoult animals and in postmoult animals after exuviation of the anterior exoskeleton. In postmoult animals cuticle is further elaborated by secretion of additional endocuticular layers and mineralization. Secretory epithelial cells produce chitinous microfibrils at their microvillar tips and form lamellae of the new endocuticule (Figure 5A, B). Exocytosis of large secretory vesicles produced in the Golgi region provides proteins of the matrix including proteins that nucleate minerals (Figure 5C). Endocuticle is perforated by numerous pore channels with cytoplasmic projections of epithelial cells extending up to the outer cuticular layers (Figure 5D).

Fig. 5. TEM micrographs of a postecdysial cuticle in postmoult animals. (A) Epithelial cells (EC) with distinct microvillar border deposit new lamellae of endocuticle (En). Abundant granular endoplasmic reticulum (GER) and Golgi stacks provide proteins for final cuticle elaboration; scale bar: 2 µm. (B) Microvillar border (arrow) of epithelial cell secreting chitinous microfibrils of a new endocuticular lamella. Cellular processes extend through the pore channels (PC); scale bar: 1 µm. (C) Golgi aparatus (GA) with secretory vesicles (SV); scale bar: 1 µm. (D) Secreted materials are transported to outer cuticular layers through numerous pore channels (PC) extending through endocuticle (En); scale bar: 2.5 µm.
Distribution of chitin and related polysaccharides in exo- and endocuticular layers was visualized by gold labelling on Tokuyasu thawed cryosections of exoskeleton with WGA lectins. Degrading endocuticular lamellae of the old cuticle are intensely labelled with WGA lectins in intramoult animals. Numerous spherules which detach from the old cuticle are connected to the nanotubular network in the ecdysial space. They are composed of concentric matrix layers with an electron-dense core and are not labelled with WGA lectins (Figure 6A, B). Exocuticle showed much weaker WGA lectin labelling than the endocuticle (Figure 6C), however it appears to be specific as control sections labelled with biotin antibody and protein A gold, but not with WGA, did not show any labelling (Figure 6D). Electron-dense epicuticular layer and the outermost exocuticular layer are mostly composed of glycoproteins as shown by histochemical staining with Alcian Blue and Coomassie Brilliant Blue. Histochemical staining with Oil Red O and Sudan Black shows presence of lipid components in the epicuticle. Epicuticular scales in intramoult animals exhibit different composition of an outer homogenous part staining intensely with Alcian Blue and a lighter-staining inner part (Supplementary Figures). As shown by TEM the inner ribbed part of the scale is weakly labelled with gold-conjugated WGA lectins, while the outer electron-dense part of the scale is not labelled (Figure 6E). Thin electron lucent vertical channels are prominent in the epicuticle. Nanotubules are attached to the epicuticle and show close connections with vertical channels. The outer epicuticle does not show any labelling with gold-conjugated WGA lectins, while a weak labelling is present in the inner epicuticle (Figure 6F).
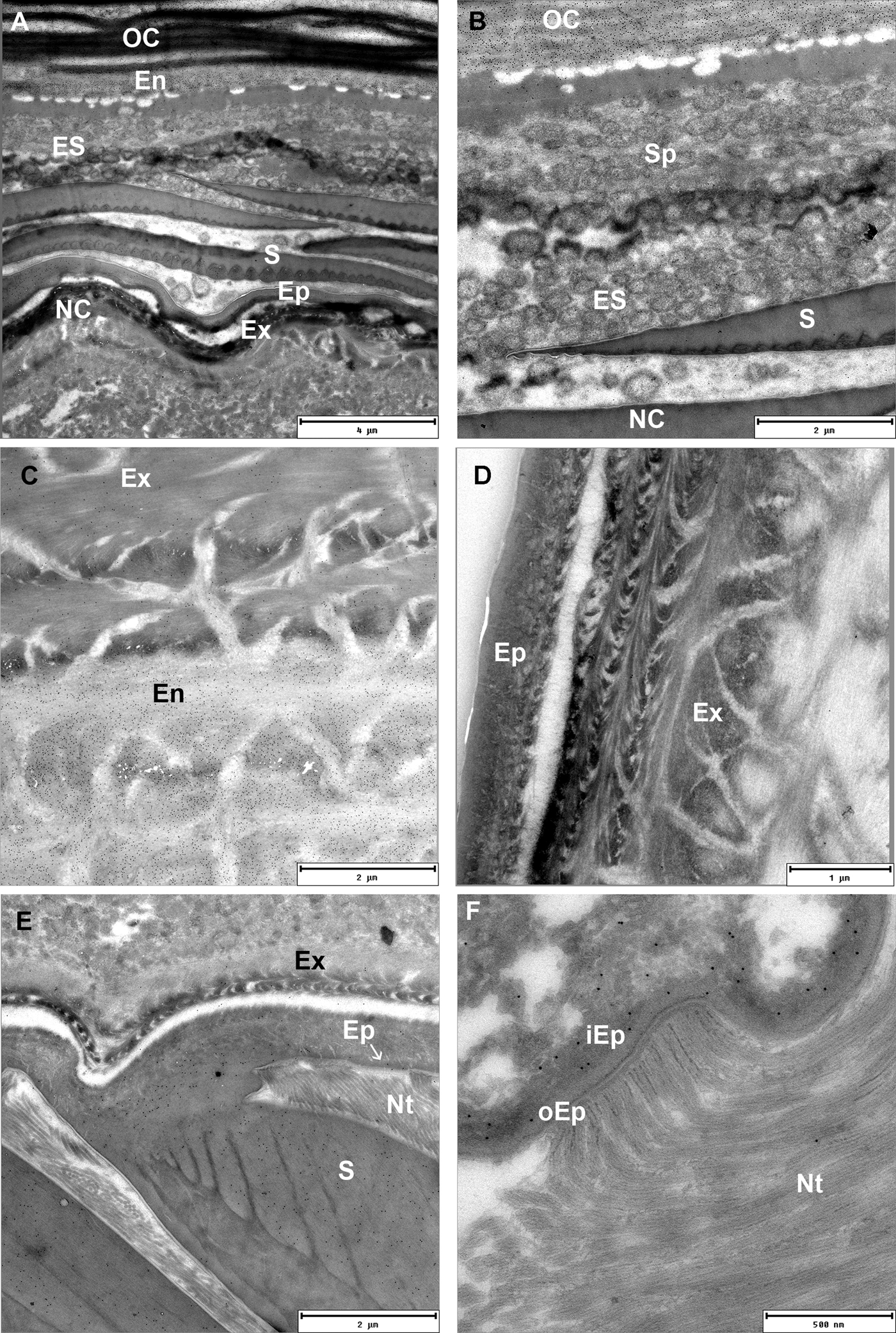
Fig. 6. TEM micrographs of Tokuyasu thawed cryosections of the anterior part of the exoskeleton in an intramoult animal gold labelled with WGA lectins. (A) and (B) Resorption of materials from the detached old cuticle (OC) which is connected to the new cuticle (NC) through ecdysial space (ES) filled with spherules (Sp). (C) Labelling with WGA lectins shows a difference in chitin content between the exocuticle (Ex) and endocuticle (En). (D) Control section of cuticle labelled with biotin antibody and protein A gold, but not with WGA. (E) The inner ribbed part of the scale (S) facing the epicuticle (Ep) is labelled with WGA lectins; nanotubules (Nt) are attached to the epicuticular surface; arrow points to the channels in the inner epicuticular layer. (F) In intramoult animals nanotubules (Nt) are attached to the non-labelled outer electron lucent epicuticle (oEp), inner epicuticle (iEp) is weakly labelled with WGA lectins.
Discussion
In this paper, we aimed to show that a combination of different microscopy methods could help explain the processes of cuticle formation and remodelling during different phases of the moult cycle and contribute to the knowledge on cuticle structure and composition in Ligia pallasii, Brandt 1833. Sea slaters of the genus Ligia are amphibious members of the woodlice family and are well adapted to a lifestyle in the upper intertidal rocky zone. They can tolerate broad ranges of light, temperature, and salinity fluctuations but are intolerant against desiccation and limited availability of algal detritus (Hurtado et al., Reference Hurtado, Mateos and Santamaria2010; Eberl, Reference Eberl2012). Their body surface is kept moist constantly by capillarity of the open water conducting system and direct absorption of water vapour. They move abruptly along rocky crevices and must prevent the materials from sticking to their cuticle surface. They can regenerate missing body parts very quickly, generally after several moults. They are quick runners and can avoid predators by flattening their body to the substrate and changing their colour. They are very sensitive to light and temperature changes and move vertically with changing tidal waves. They have complex compound eyes covered by cuticular cornea and numerous protuberances on the cuticle surface. Wedge-shaped scales are very prominent around tricorn sensory sensilla and on the lateral surface of tergites (epimeres). The tricorn sensilla are a general feature of cuticular surface in terrestrial isopods with mechano-, chemo- and hygroreceptive functions (Schmalfuss, Reference Schmalfuss1978; Powell & Halcrow, Reference Powell and Halcrow1985; Ziegler & Altner, Reference Ziegler and Altner1995) as was also described in Tylos europeaus (Seidl et al., Reference Seidl, Huemer, Neues, Hild, Epple and Ziegler2011).
The described physiological and behavioural patterns can be achieved due to structural and functional properties of their exoskeleton, a lightweight, flexible, anti-adhesive and renewable cuticle, housing different receptors for sensing the environment. Our results show that cuticle of amphibious sea slaters is a dynamic extracellular matrix which is constantly renewed by frequent moults during development and growth.
Structural and functional properties of epicuticle
A shingle-like epicuticular surface of anterior pereonites of premoult and postmoult animals is covered with rounded scales oriented posteriorly and ‘artichoke-like’ sensory fields of tricorn sensilla surrounded by lifted wedge-shaped scales. The functional aspects of tricorn sensilla in terrestrial isopods were discussed by several authors and chemo- and mechanoreceptive functions were proposed for dorsal carapace sensilla in Ligia exotica (Hatanaka, Reference Hatanaka1989). As hygroreceptors represent an important part of the sensory system in terrestrial isopods, a thermo-hygro-sensitivity similar to that in insects was proposed for tricorn sensilla in Porcellio scaber (Ziegler & Altner, Reference Ziegler and Altner1995). Epicuticle is the first layer secreted in early premoult animals and consists of an outer epicuticle, and a thin electron lucent sublayer, similar to the envelope in insects (Moussian et al., Reference Moussian, Seifarth, Müller, Berger and Schwarz2006) and a thicker and electron-dense inner epicuticle with thin vertical channels. Epicuticular channels or cavities with external pores and communicating with pore channels in the exocuticle were also described in amphipod crustaceans (Halcrow & Powell, Reference Halcrow and Powell1992). The outer epicuticle is composed of lipids and waxes (Compere, Reference Compere, Juchault and Mocquard1991) which could be secreted through channels present in the inner epicuticle and/or through the nanotubular network attached to the outer epicuticle in premoult and intramoult animals. A remarkably complex epicuticle structure composed of trilaminar outer epicuticle and thicker inner epicuticle comprising three sublayers with special reinforcing central zone was described in the marine isopod Idotea baltica (Powell & Halcrow, Reference Powell and Halcrow1985). It is generally accepted that the epicuticle of arthropods contains no chitin and is composed of lipoproteins, and functions in draining water and materials over the surface, and prevents them from sticking to the surface (Compere, Reference Compere, Juchault and Mocquard1991; Locke, Reference Locke2001). Lipoproteic composition of insect epicuticle was described as an important adaptation to terrestrial lifestyle (Moussian, Reference Moussian2010). The composition of epicuticular layers in terrestrial isopods was investigated by several authors (Price & Holdich, Reference Price and Holdich1980a, Reference Price and Holdich1980b; Compere, Reference Compere, Juchault and Mocquard1991) and it was shown that epicuticle does not contain chitin. Histochemical staining of cuticle in premoult and intramoult terrestrial isopods (see Supplementary Figures) confirms lipoproteic composition of epicuticle. In terrestrial isopods a glyco- and lipoproteic composition of cuticular scales would be advantageous to keep clean surfaces and prevent excessive water loss. It is possible that vertical channels present in the inner epicuticle deliver wax-like materials to the outer cuticular layer during post-ecdysial cuticle modification and thus affect cuticle permeability. The extensive nanotubular network associated with spherules in the ecdysial space and attached to the outer epicuticle is present in premoult and intramoult animals. It was shown that spherules contain and transport organic components and minerals from the degrading old cuticle to the new cuticle (Ziegler, Reference Ziegler1997; Žnidaršič et al., Reference Žnidaršič, Tušek-Žnidarič, Strus, Matsko, Letofsky-Papst, Grogger and Hofer2010). Our results of different fixation procedures of exoskeleton show that the nanotubules fixed with osmium tetroxide and contrasted with metal salts contain electron-dense material especially at the epicuticle attachment site. In Tokuyasu sections contrasted with uranyl acetate, the walls and the lumen of nanotubules appear electron dense, presumably due to material of proteinaceous origin. The role of the nanotubular network in cuticle elaboration may be the delivery of resorbed cuticular components, including minerals and water, to the new epicuticular layers in premoult and intramoult animals.
A prominent feature of the cuticular surface in Ligia pallasii is extensive epicuticular scales. Epicuticular scales in moulting Ligia pallasii exhibit two distinct parts with different components. Ultrathin Tokuyasu thawed cryosections of the anterior tergite cuticle labelled with WGA revealed different structure and composition of the outer and inner surfaces of the epicuticular scales, namely the outer homogenous electron-dense surface and ribbed inner surface. Lectin WGA binds highly specifically to N-acetyl-D-glucosamine and has a strong affinity to oligomers and polymers of N-acetyl-glucosamine, especially chitin with the combining site complementary to a sequence of three ß-(1→4)-linked N-acetyl-glucosamine residues. The existence of eight simultaneously functional sugar binding sites on the WGA dimer indicates a complexity of WGA interactions with cell surface glycoconjugates, thus, a careful interpretation of WGA binding is requested in case of chitin localization (Peters & Latka, Reference Peters and Latka1986; Iskratsch et al., Reference Iskratsch, Braun, Paschinger and Wilson2009; Schwefel et al., Reference Schwefel, Maierhofer, Beck, Seeberger, Diederichs, Möller, Welte and Wittmann2010). Based on WGA gold labelling on Tokuyasu sections, we confirmed that the outer electron-dense and homogenous part of the scale, which is not labelled with WGA lectins, does not contain chitin-like material. The results of combined histochemical staining and WGA lectin labelling, demonstrate that acidic carbohydrates/glycoconjugates and lipoproteins are abundant in the outer part of the scales of intramoult animals. We suppose that the inner part of the scale, which labels weakly with gold-conjugated WGA lectins, is composed mostly of glycoproteins and acts as a hydrophilic surface to keep the cuticle moistened by retaining water. The inner surface of the scale is connected to a nanotubular network which might function in water retention (Glötzner & Ziegler, Reference Glötzner and Ziegler2000) or as snorkels for transport of water and hydrophilic substances across ecdysial space in moulting animals. It is well known that crustaceans absorb large amounts of water during ecdysis, so the formation of a spacious new cuticle enables tissue growth. It was shown that an increase in the haemolymph volume in intramoult Ligia pallasii was a result of a sudden uptake of seawater to increase the volume of the body immediately after posterior moult (Ziegler et al., Reference Ziegler, Grospietsch, Carefoot, Danko, Zimmer, Zerbst-Boroffka and Pennings2000). Very often numerous bacteria are found close to the inner surface of the scale and are connected to nanotubules at the new epicuticle surface of intramoult animals. It is probable that bacteria are involved in processes of cuticle remodelling during exuviation. Recently an extensive review was presented on the microbiome in terrestrial isopods with relevance to ecosystem functioning (Bouchon et al., Reference Bouchon, Zimmer and Dittmer2016). A few studies exposed the presence of symbiotic bacteria in the digestive system of semi-terrestrial isopods showing their role in oxidation of phenols and digestion of cellulose which is a likely preadaptation of isopods to a terrestrial existence (Zimmer et al., Reference Zimmer, Danko, Pennings, Danford, Carefoot, Ziegler and Uglow2001, Reference Zimmer, Danko, Pennings, Danford, Carefoot, Ziegler and Uglow2002; Eberl, Reference Eberl2012). Rod-shaped bacteria from the novel lineage of Mollicutes, attached to the gut cuticle were described in moulting terrestrial isopod Porcellio scaber (Kostanjšek et al., Reference Kostanjšek, Štrus and Avguštin2007).
Structural and functional properties of exocuticle and endocuticle
Exocuticular layers of preecdysial cuticle are secreted during premoult when the materials of the detached old cuticle are resorbed. Apical membrane of the epidermal cells forms short microvilli, where chitinous microfibrils are formed and incorporated into subsequent cuticular layers. Exocytosis at the apical cell membrane provides proteins and glycoproteins which are synthesized in abundant rough endoplasmic reticulum and packed into secretory vesicles in Golgi apparatus of the epidermal cells. Extensive network of pore channels with cell processes reaching the outermost cuticular layers, transports minerals and other constituents to outer cuticular layers. On the other side constituents resorbed from the old detached cuticle are organized into spherules, which fill the ecdysial gap. Spherules are composed of concentric layers of electron dense material and loose net-like matrix. Connections between spherules and nanotubules, which are regularly observed at the epicuticular surface, confirm a transporting role of nanotubules. As we have shown in previous publications spherules contain calcium minerals and possibly function as transporting elements of organic material and minerals resorbed from the old cuticle in premoult and intramoult animals (Strus & Compere, Reference Strus and Compere1996; Strus & Blejec, Reference Strus, Blejec, Kensley and Brusca2001). In postmoult animals, after ecdysis of the anterior half of the cuticle, endocuticular lamellae are formed and pore channels with extending cytoplasmic cellular processes connect the epidermal cells with the outermost cuticular layers. It was shown that in decapod crustaceans permeability properties of postmoult cuticle change significantly due to secretion of epicuticular components (Dillaman et al., Reference Dillaman, Roer, Modla and Williams2009). Calcification of the cuticle starts already in intramoult animals and continues during the postmoult period. Pore channels are the sites of transport of mineral and mineral nucleating proteins to the distal cuticular layers. This was also shown in terrestrial isopods with highly mineralized cuticles (Neues et al., Reference Neues, Ziegler and Epple2007; Seidl et al., Reference Seidl, Huemer, Neues, Hild, Epple and Ziegler2011). Distribution of the mineral component in an intramoult animal with CT scanning showed progressive resorption of mineral from the old cuticle in a posterior-anterior direction. The method is very promising for studies of mineral translocation in the exoskeleton during moulting.
Cuticle of Ligia pallasii is a unique extracellular matrix which is constantly remodelled during growth and regeneration. It is a porous and vital extracellular matrix with an extensive network of pore channels that deliver materials synthesized in epidermal cells to the outermost cuticular layers. Even after detachment of the old cuticle during early premoult both the newly formed and the old cuticle are connected by filaments extending through the ecdysial space at special myotendinous junctions which enables movement and feeding of premoult animals (Žnidaršič et al., Reference Žnidaršič, Mrak, Tušek-Žnidarič and Štrus2012). With a combination of different microscopy approaches, we described structural features of exoskeletal cuticle at different resolution levels and exposed properties of different cuticular layers as possible functional and behavioural adaptations of moulting sea slaters Ligia pallasii to a terrestrial lifestyle. Members of the genus Ligia are very convenient for studies of structural and functional adaptations during transition from marine to land habitats and the light, flexible, waterproofing and anti-adhesive exoskeleton is a great advantage to these quickly moving animals of the upper intertidal rocky shores.
Supplementary Material
The supplementary material for this article can be found at https://doi.org/10.1017/S0025315418001017.
Acknowledgements
We thank the Electron Microscopy Laboratory at the University of Oslo, Department of Biosciences led by Norbert Roos. We are extremely grateful to Gareth Griffiths, University of Oslo and Heinz Schwarz, Max Planck Institute for Developmental Biology in Tuebingen, for discussions and guidance. Thanks to Professor Billie Swalla, the director of Friday Harbor Laboratories for support and special thanks to Ms Kathy Cowell for arranging the stay of JS and AB at Whiteley Centre at FHL. Elaine Humphrey from Advanced Microscopy facility at the University of Victoria, Canada helped with proofreading of the manuscript and editing micrographs. Thanks to Polona Mrak from Department of Biology, University of Ljubljana for histochemical analysis of cuticle. This paper was presented at the 52nd European Marine Biology Symposium in Piran, Slovenia.
Financial support
Research work at microscopy facility at the Department of Biosciences, University of Oslo and Department of Biology, University of Ljubljana was funded by Erasmus staff mobility grant in 2016, and the research programme P1-0184 of the Slovenian Research Agency. Research work performed at Friday Harbor Laboratories was funded from the European Union, European Social Fund, Ministry of Education, Science and Sports, project Internationalization of the University of Ljubljana, Staff mobility, contract No. 3330-17-539031.