Introduction
A fallow phase is common in Australian cropping systems, especially in the eastern cropping region. Depending on soil moisture conditions, a fallow phase may last for more than a year (Thomas et al. Reference Thomas, Felton, Radford, Clarke and Wylie1997; Webb et al. Reference Webb, Grundy, Powell, Littleboy, Clarke and Wylie1997). Although the purpose of the fallow phase is to help soils to conserve nutrients and moisture, weeds growing during the fallow phase can consume a significant amount of these resources (Chauhan and Jha Reference Chauhan and Jha2020), resulting in yield reductions of crops growing in subsequent seasons (Widderick et al. Reference Widderick, Sindel and Walker1999). In grain-cropping systems, about A$500 million is spent annually on fallow weed control, emphasizing the importance of weeds during the fallow phase (Llewellyn et al. Reference Llewellyn, Ronning, Ouzman, Walker, Mayfield and Clarke2016).
Conyza species, mainly hairy fleabane [Conyza bonariensis (L.) Cronquist] and tall fleabane [Conyza sumatrensis (Retz.) Walker], are very common in summer and winter fallows and crops, infesting an area of 600,000 ha annually in grain cropping systems (Llewellyn et al. Reference Llewellyn, Ronning, Ouzman, Walker, Mayfield and Clarke2016). Hairy fleabane has been a problematic weed in Australia since the 1980s (Felton et al. Reference Felton, Wicks and Welsby1994; Wicks et al. Reference Wicks, Felton, Murison and Martin2000), but tall fleabane has recently emerged as a problematic weed in grain and cropping systems (Asaduzzaman et al. Reference Asaduzzaman, Koetz, Wu and Shephard2022). In Australia, tall fleabane is present in all the states and territories, except the Northern Territory (AVH 2022). In addition to infesting fallow fields, it is a problematic weed in cotton (Gossypium hirsutum L.) and horticultural fields (Diez de Ulzurrun et al. Reference Diez De Ulzurrun, Acedo, Garavano, Gianelli and Ispizua2020; Everett Reference Everett and Harden1990; Thebaud and Abbott Reference Thebaud and Abbott1995) in other countries.
No information is available on the impact of tall fleabane on crop production in Australia. A recent study in Brazil showed a 100% reduction in soybean [Glycine max (L.) Merr.] grain yield at a density of 34 plants m−2 of tall fleabane (Cantu et al. Reference Cantu, Albrecht, Albrecht, Silva, Danilussi and Lorenzetti2021). Horseweed [Conyza canadensis (L.) Cronquist], a closely related species of tall fleabane, can cause >80% yield reductions in soybean (Bruce and Kells Reference Bruce and Kells1990). Hairy fleabane was found to reduce sorghum [Sorghum bicolor (L.) Moench] yield by up to 98% if not controlled (Wu et al. Reference Wu, Walker, Robinson and Coombes2010), suggesting the high economic impact of Conyza species in crop production. Tall fleabane can produce up to 60,000 seeds per plant (Hao et al. Reference Hao, Qiang, Liu and Cao2009) and the seeds can remain viable for 2 to 3 yr in the soil (Hayashi Reference Hayashi1979). Seeds of Conyza species are known to have a low settling velocity in the air, suggesting that seeds settle on the ground away from the parent plant (Andersen Reference Andersen1992).
Seeds of tall fleabane can germinate at temperatures ranging from 15/5 to 35/25 C [alternating day/night (12-h/12-h) temperatures; Mahajan et al. Reference Mahajan, Prasad and Chauhan2021], suggesting the potential for this weed to germinate throughout the year in Australian cropping systems. The greatest seed germination of tall fleabane has been observed on the soil surface, and no emergence occurs from seeds buried at 2 cm (Mahajan et al. Reference Mahajan, Prasad and Chauhan2021). The ability to produce a high number of seeds with wind-dispersal traits, the greatest germination for the surface seeds, and the ability to germinate at a wide range of temperatures suggest that the spread of tall fleabane across no-till farming systems could be very rapid.
Glyphosate, a 5-enolpyruvylshikimate-3-phosphate synthase (EPSPS) inhibitor, is commonly used to control weeds in no-till fallow conditions in Australia, as tillage is not used due to the need to conserve soil moisture and nutrients. However, overreliance on glyphosate has led to the evolution of 21 glyphosate-resistant weeds in Australia (Heap Reference Heap2022). For example, the first case of glyphosate-resistant hairy fleabane was reported in 2010 in New South Wales, and since then, several populations have evolved resistance to glyphosate (Heap Reference Heap2022; Walker et al. Reference Walker, Bell, Robinson and Widderick2011). In 2018, the first case of glyphosate-resistant tall fleabane was reported from a fallow field in Queensland (Heap Reference Heap2022). In addition to Australia, glyphosate-resistant tall fleabane is also present in Brazil, Greece, Spain, and Turkey. In other countries, this weed has also evolved resistance to paraquat, saflufenacil, 2,4-D, and penoxsulam (Heap Reference Heap2022).
During late summer 2018, inconsistent control of a tall fleabane population following glyphosate application was observed in a chemical fallow field in Dalby, Queensland. Seeds from the surviving tall fleabane plants were collected from the field and evaluated for resistance to glyphosate. No information is available on the performance of alternative herbicides to control glyphosate-resistant tall fleabane in fallow situations. Therefore, a study was conducted to 1) confirm the level of glyphosate resistance in the putative glyphosate-resistant tall fleabane population, and 2) evaluate the efficacy of alternative postemergence (POST) herbicides for use in fallow conditions to control glyphosate-resistant tall fleabane.
Materials and Methods
Seed Collection
Seeds of a putative glyphosate-resistant (GR) tall fleabane population were collected in January 2018 from a no-till fallow field near Dalby, Queensland. Seeds from about 15 mature plants were collected that had survived the field rate of glyphosate (740 g ha−1). Seeds from all the plants were mixed and stored at room temperature (25 ± 2 C) until used. Seeds of a known glyphosate-susceptible (GS) population were collected from the fenceline of a fallow field at the University of Queensland, Gatton, in September 2018. This fenceline population used to be well controlled with the field rate of glyphosate.
Experiment 1. Glyphosate Dose Response
Seeds of both populations (GR and GS) were sown on the soil surface in 20-cm-diameter pots filled with a commercial potting mix (Centenary Landscape, Brisbane). Pots were placed on benches in an open environment at the weed science research facility of the University of Queensland, Gatton, Queensland. An automated sprinkler system was used to irrigate pots every day. Immediately after emergence, seedlings were thinned, and five plants per pot were maintained. At the 4- to 5-leaf stage of each population, glyphosate was applied at 185, 370, 740, 1,480, and 2,960 g ha−1 using a research track sprayer equipped with Teejet XR110015 (Sprayshop; Toowoomba, Queensland, Australia) flat-fan nozzles that were calibrated to deliver 108 L ha−1 of spray solution at 200 kPa. There was also a control treatment, in which no herbicide was applied. The experiment was arranged in a randomized complete block design with four replications. The experiment was conducted in November 2018 and repeated in November 2019. The average maximum and minimum temperatures were 34 to 36 C and 15 to 16 C, respectively. At 3 wk after glyphosate application, plants were evaluated for survival and harvested at the base to determine their aboveground shoot dry biomass. The criterion for survival was the appearance of at least one new leaf after glyphosate application. Harvested plant samples were placed in an oven at 70 C for 72 h, and their biomass was determined. The biomass of only plants that survived was measured and expressed as a percentage of the nontreated control treatment.
Experiment 2. EPSPS Gene Sequencing
Seeds of both populations (GR and GS) were planted in pots as described above. There were only two treatments: nonsprayed (control) and sprayed with glyphosate at 740 g ha−1. Each treatment was replicated three times (five plants per pot). At 3 wk after spray, survived plants were sampled for the GR population, and the nonsprayed plants for the GS population (because glyphosate killed all GS plants). Fresh leaf samples (three plants of each population) were harvested, and genomic DNA was extracted from leaf tissues as described by Desai et al. (Reference Desai, Thompson and Chauhan2020). A polymerase chain reaction (PCR) was set up to amplify the conserved area of the EPSPS gene. Primer sequences (forward: AACAGTGAGGAYGTYCACTACATGCT; reverse: CGAACAGGAGGGCAMTCAGTGCCAAG) and subsequent DNA sequencing were adapted from a previous study (Ngo et al. Reference Ngo, Krishnan, Boutsalis, Gill and Preston2018). Each PCR was run as described by Desai et al. (Reference Desai, Thompson and Chauhan2020), and PCR products were sent to the Australian Genome Research Facility, Brisbane, Queensland, for Sanger sequencing using the same primers to detect any nucleotide changes in the amplified EPSPS DNA sequences. Alignment of sequences was conducted using Molecular Evolutionary Genetics Analysis software (version 11; proprietary freeware; Pennsylvania State University). The experiment was conducted two times, in February 2021 and October 2021.
Experiment 3. Response of Tall Fleabane at Two Growth Stages to Herbicides
As described above, seeds of both populations were sown in 20-cm-diameter pots. After emergence, 8 plants pot−1 were maintained. Herbicides at the field rate (Table 1) were sprayed at the 4-leaf and 12- to 14-leaf stages of tall fleabane. Seedling survival and biomass were evaluated as described above for the glyphosate dose experiment. The experiment was conducted in a factorial randomized block design with three replications. The first factor was the leaf stage (4 leaves and 12 to 14 leaves), and the second factor was herbicide treatments. This experiment was conducted in December 2018 and repeated in December 2019.
Table 1. Herbicides, their doses, and adjuvants used in Experiments 3 and 4.

a A commercial mixture of two herbicides.
b Adjuvent suppliers: BS1000 and Cando: Nufarm, Australia Ltd, Laverton North, Victoria, Australia; Hasten: BASF Australia Ltd, Southbank, Victoria, Australia; Uptake: Corteva Agriscience Australia Pty Ltd, Chatswood, New South Wales, Australia.
Experiment 4. Response of Tall Fleabane to Herbicide Mixtures
As described above, seeds of both populations were plants in 20-cm-diameter pots. In this experiment, four plants per pot were maintained and sprayed at the 12- to 14-leaf stage with herbicides listed in Table 1. Seedling survival and their biomass were determined as described above for the glyphosate dose experiment. The experiment was arranged in a randomized complete block design with six replications. The experiment was conducted in two runs (July to October 2021 and January to April 2022).
Statistical Analyses
Data from the two runs were subjected to ANOVA to determine interactions between treatment and experimental run (Genstat 2021). The interactions were nonsignificant; therefore, the data over the two experimental runs were pooled for further analysis. Data were also validated to meet the assumption of normality and variance before analysis. In the glyphosate dose-response experiment, seedling survival, and biomass (percent of nontreatment control) data were regressed against herbicide doses using a three-parameter logistic model using SigmaPlot 14.5 (Systat Software, Inc.; Point Richmond, CA, USA). The model was as follows:

where y is the seedling survival (%) or seedling biomass (% of nontreated control) at glyphosate dose x, a is the maximum value of survival or biomass, x 50 is the glyphosate dose (in grams per hectare) required to cause a 50% reduction in seedling survival (LD50) or biomass (GR50), and b is the slope. The fitness of the selected model was determined using R 2 values.
In Experiment 3, data were subjected to ANOVA to test for interactions between populations, leaf stages, and herbicide treatments. In Experiment 4, data were subjected to test for interactions between populations and herbicide treatments. Means were separated at P ≤ 0.05 using Fisher’s protected LSD test.
Results and Discussion
Experiment 1. Glyphosate Dose Response
The glyphosate dose-response experiment revealed that the LD50 value for the GS population of tall fleabane was 402 g ha−1 (Figure 1A; Table 2). The LD50 value for the GR population was 1,604 g ha−1, indicating 4-fold resistance to glyphosate in this population. The LD90 values for the GR and GS populations were 520 and 1,703 g ha−1, respectively. Based on aboveground biomass, the GR50 values for the GR and GS populations were 600 and 170 g ha−1 (Figure 1B; Table 2). Based on the GR50 values, the GR population exhibited 3.5-fold resistance to glyphosate.
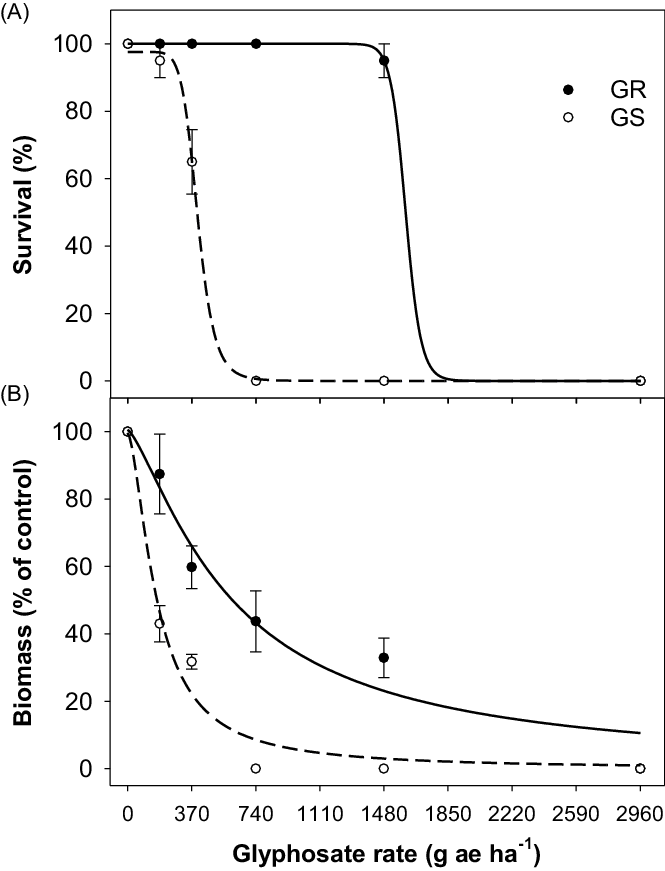
Figure 1. Effect of glyphosate dose on (A) survival and (B) biomass (percent of nontreated control) of glyphosate-resistant (GR) and glyphosate-susceptible (GS) populations of tall fleabane. A three-parameter log-logistic model was fitted to the data. Plants were sprayed at the 4-leaf stage of each population.
Table 2. Estimated glyphosate dose required to kill 50% of the plants (LD50) and reduce biomass by 50% (GR50) of glyphosate-resistant and glyphosate-susceptible populations of tall fleabane. a,b

a Values in parentheses are ± standard errors (SE).
b Plants were sprayed at the 4- to 5-leaf stage.
In a recent study in Turkey, tall fleabane populations from peach [Prunus persica (L.) Batsch] orchards exhibited 3.6-fold to 6.6-fold resistance to glyphosate on the basis of LD50 values, and 1.4-fold to 1.7-fold resistance based on GR50 values compared to susceptible populations (İnci et al. Reference İnci, Galvin, Al-Khatib and Uludağ2019). In another study conducted in Spain, a susceptible population of tall fleabane had a GR50 value of 33 g ha−1, and the GR50 values for resistant populations were 177 to 229 g ha−1, indicating 5.4-fold to 6.9-fold resistance in the resistant populations (Palma-Bautista et al. Reference Palma-Bautista, Tataridas, Kanatas, Travlos, Bastida, Domínguez-Valenzuela and De Prado2020). Similar to our study, the first GR population of tall fleabane in Brazil exhibited a 2.9-fold level of resistance (Santos et al. Reference Santos, Oliveira, Constantin, Constantin Francischini, Machado, Mangolin and Nakajima2014). Another population of tall fleabane collected from a vineyard in France, however, exhibited 20-fold resistance compared to that of a susceptible population (Tahmasebi et al. Reference Tahmasebi, Alebrahim, Roldán-Gómez, Silveira, Carvalho, Alcántara-De La Cruz and De Prado2018). Glyphosate resistance has also been reported in horseweed. For example, a horseweed population from Montana in the United States, was found to exhibit 2.5-fold resistance to glyphosate on the basis of GR50 values (Kumar et al. Reference Kumar, Jha and Jhala2017). Similarly, another population of horseweed from the Central Valley of California, also in the United States, exhibited a 4.8-fold level of resistance (Hanson et al. Reference Hanson, Shrestha and Shaner2009).
Results suggest that glyphosate used at the maximum recommended field rate (i.e., 740 g ha−1) for most broadleaf weeds may no longer be an effective option for controlling this GR population. Tall fleabane can grow throughout the year in eastern Australia, and its seeds have no or a low level of dormancy (Mahajan et al. Reference Mahajan, Prasad and Chauhan2021). These traits suggest that the sole reliance on glyphosate for tall fleabane control in fallows and glyphosate-resistant cotton crops in Australia may result in a rapid increase in resistant cases. Tall fleabane is a self-pollinated species, and wind-mediated dispersal of seeds could help in the spread of resistance from one field to another (Andersen Reference Andersen1993; Diez de Ulzurrun et al. Reference Diez De Ulzurrun, Acedo, Garavano, Gianelli and Ispizua2020; Hao et al. Reference Hao, Qiang, Liu and Cao2009). A study in China concluded that autonomous seed production contributed significantly to the invasiveness of tall fleabane (Hao et al. Reference Hao, Qiang, Liu and Cao2009).
Experiment 2. EPSPS Gene Sequencing
Comparisons of the sequences for each population showed that all plants of the GR population shared the same sequence as the plants of the GS population (Table 3). Both populations consisted of a missense mutation at Pro-106, in which threonine is substituted for proline; a nucleotide change at the codon, from CCA to ACA. A DNA sequencing chromatogram showed that samples from both populations carried homozygous and heterozygous mutation at the position Pro-106 (Table 3), suggesting that the Pro-106 mutation to Thr may not be sufficient to confer target-site resistance in tall fleabane. Therefore, the resistance mechanism in the GR population of tall fleabane is likely to be a nontarget site.
Table 3. Aligned nucleotide sequences of the conserved region of EPSPS of a GS and a GR population of tall fleabane. a,b

a Abbreviations: EPSPS, 5-enolpyruvylshikimate-3-phosphate synthase; GR, glyphosate resistant; GS, glyphosate susceptible.
b Plants were sprayed at the 4- to 5-leaf stage.
c Missense mutation.
d Heterozygous.
In a similar study in Spain, one resistant population of tall fleabane had threonine substituted for proline at Pro-106, but another resistant and the susceptible population did not have any amino acid substitution (Palma-Bautista et al. Reference Palma-Bautista, Alcántara-De La Cruz, Rojano-Delgado, Dellaferrera, Domínguez-Martínez and De Prado2019). In an earlier study also conducted in Spain, however, both target-site and nontarget site resistant (NTSR) mechanisms contributed to glyphosate resistance in tall fleabane (Amaro-Blanco et al. Reference Amaro-Blanco, Fernández-Moreno, Osuna-Ruiz, Bastida and De Prado2018). The NTSR mechanisms involved restricted uptake and translocation of glyphosate. The NTSR mechanisms were not studied in the current study.
Experiment 3. Response of Tall Fleabane at Two Stages to Herbicides
Interactions were significant between population, leaf stage, and herbicide treatment for seedling survival (Table 4) and biomass (Table 5). All herbicide treatments provided 100% mortality of the GS population when applied at the 4-leaf stage. Except for glyphosate, all other herbicides also provided complete control of the GR population at this leaf stage (Table 4). About 96% of seedlings survived the field rate of glyphosate, and the plants that survived produced 26% biomass of the nontreated control treatment. At the 12- to 14-leaf stage, none of the herbicide treatments provided more than 60% mortality, irrespective of the population. At this stage, 100% of seedlings of the GR population and 75% of seedlings of the GS population survived the field rate of glyphosate. Plants that survived produced similar biomass to that of the control plants for the GR population, whereas biomass for the GS population was reduced by 76%. Compared with the biomass of nontreated control plants, other herbicide treatments significantly reduced biomass for both GR and GS populations (Table 5). Among all treatments, saflufenacil was the most effective herbicide. Although 40% to 50% of seedlings survived the field rate of saflufenacil (12 g ha−1), these seedlings produced only 15% to 25% biomass of the nontreated control treatment.
Table 4. Interaction effect of population, herbicide, and leaf stage on the survival of GR and GS populations of tall fleabane. a,b

a Abbreviations: GR, glyphosate resistant; GS, glyphosate susceptible; LSD, least significant difference.
b Plants were sprayed at the 4 and 12- to 14-leaf stages.
Table 5. Interaction effect of population, herbicide, and leaf stage on aboveground biomass of GR and GS populations of tall fleabane. a– c

a Abbreviations: GR, glyphosate resistant; GS, glyphosate susceptible; LSD, least significant difference.
b Plants were sprayed at the 4-leaf and 12- to 14-leaf stages.
c Values in parentheses represent the percent reduction in biomass compared with their respective nontreated control biomass.
The results of this trial suggest that there are several alternate herbicide options for the control of the GR population of tall fleabane when sprayed at the 4-leaf stage. However, due to environmental constraints and the tendency to wait for a greater number of seedlings to emerge to save costs on fuel and herbicides, growers may not be able to target tall fleabane at a young seedling stage (e.g., 4-leaf). Results indicate that herbicides, including 2,4-D, bentazone, fluroxypyr, metsulfuron, and saflufenacil, may not provide effective control of tall fleabane when those herbicides are applied alone at the 12- to 14-leaf stage. In a study conducted in Brazil, younger plants (5 to 6 leaves) of tall fleabane tended to be controlled better than older plants (12 to 15 leaves) by glyphosate even at rates lower than the recommended rate (Santos et al. Reference Santos, Oliveira, Constantin, Constantin Francischini, Machado, Mangolin and Nakajima2014). The study suggested that herbicide translocation is favored by young plants, and with the development of plants, the size exclusion limit of plasmodesmata reduces, which might explain the lower susceptibility of plants to herbicides when they develop. Similar results were reported in horseweed also. The LD50 values for a GS and GR population of horseweed were 620 and 1,940 g ha−1, respectively, when glyphosate was applied at an early-rosette stage (5- to 8-cm-diameter; Kumar et al. Reference Kumar, Jha and Jhala2017). The LD50 values increased to 980 and 7,800 g ha−1, respectively, when glyphosate was sprayed at a late-rosette stage (12 to 15 cm diameter).
Similar to the results reported here, fluroxypyr (200 g ha−1) and 2,4-D (720 g ha−1) alone provided 50% to 80% control levels in both GR and GS populations of tall fleabane in a study conducted in Europe (Tahmasebi et al. Reference Tahmasebi, Alebrahim, Roldán-Gómez, Silveira, Carvalho, Alcántara-De La Cruz and De Prado2018). In Brazil, poor control of tall fleabane was obtained with 2,4-D, glyphosate, paraquat, and saflufenacil when these herbicides were applied to 12- to 15-cm-tall plants (de Pinho et al. Reference De Pinho, Leal, Souza, De Oliveira, De Oliveira, Langaro, Machado, Christoffoleti and Zobiole2019). Previous studies and the current study suggest that tall fleabane control is difficult to achieve when herbicides are applied alone on large plants.
Experiment 4. Response of Tall Fleabane to Herbicide Mixtures
As described above, the sole application of single herbicides was not effective on tall fleabane when applied at the 12- to 14-leaf stage. Therefore, this experiment evaluated a range of herbicide mixtures for the control of GR and GS populations of tall fleabane at the 12- to 14-leaf stage. The sole application of glufosinate, glyphosate, paraquat, saflufenacil, and tiafenacil failed to provide complete control of the GR and GS populations of tall fleabane (Table 6). Although 17% to 21% of tall fleabane seedlings survived the field rate of glufosinate, the survived plants produced only 4% to 8% biomass of their nontreated control treatments. The sole application of the commercial mixture of saflufenacil + trifludimoxazin provided 100% mortality in the GS population but about 17% of seedlings of the GR population survived this herbicide treatment. The plants of the GR population that survived, however, produced only 5% biomass of the nontreated control treatment. The results of this trial suggest that although the sole application of glufosinate and saflufenacil + trifludimoxazin did not provide complete control of tall fleabane, these were the best sole treatment, providing >90% reduction in biomass.
Table 6. Interaction effect of population and herbicide on survival and aboveground biomass of GR and GS populations of tall fleabane. a–c

a Abbreviations: GR, glyphosate resistant; GS, glyphosate susceptible; LSD, least significant difference.
b Plants were sprayed at the 4-leaf and 12- to 14-leaf stages.
c Values in parentheses represent the percent reduction in biomass compared with their respective nontreated control biomass.
In general, herbicide mixtures worked better on the GS population compared with their effect on the GR population (Table 6). For both populations, seedling survival and biomass were similar across mixtures of saflufenacil with glyphosate, glufosinate, or paraquat. Saflufenacil when mixed with these individual herbicides provided 79% to 100% mortality of the GS population and reduced seedling biomass by 96% to 100%. These herbicide treatments provided only 62% to 81% mortality of the GR population, but the biomass of seedlings that survived was reduced by 80% to 98% compared with that of the nontreated control treatments. As a mixture partner with either of the nonselective herbicides (glufosinate, glyphosate, or paraquat), saflufenacil + trifludimoxazin was the best herbicide treatment, providing 100% mortality of both populations of tall fleabane. Tiafenacil mixture with glyphosate was not effective, but with glufosinate, it provided complete control of both populations.
Saflufenacil, saflufenacil + trifludimxoxazin, and tiafenacil are relatively new herbicides in Australia. These three herbicides are an inhibitor of protoporphyrinogen oxidase (PPO), which exhibit foliar and residual activity on broadleaf weeds, including tall fleabane (Park et al. Reference Park, Ahn, Nam, Hong, Song, Kim, Yu and Sung2018; Soltani et al. Reference Soltani, Shropshire and Sikkema2021; Waggoner et al. Reference Waggoner, Mueller, Bond and Steckel2011). Information on the effect of the PPO inhibitors and their mixtures with other herbicides is very limited on tall fleabane, especially in Australia. However, results are available on other Conyza species. Similar to the results of current study, GR horseweed control was similar across the paraquat + saflufenacil, glyphosate + saflufenacil, and glufosinate + saflufenacil mixtures in a field study conducted in the United States (Waggoner et al. Reference Waggoner, Mueller, Bond and Steckel2011). The previous study also suggested that saflufenacil at 25 g ha−1 (vs. 12.5 and 50 g ha−1) was the most optimal rate for mixtures with the three nonselective herbicides. In the current study, however, saflufenacil at 12 g ha−1 was used. In a study in Canada, GR horseweed control was only 68% when tiafenacil at 25 g ha−1 was mixed with glyphosate at 900 g ha−1 (Soltani et al. Reference Soltani, Shropshire and Sikkema2021). These results are similar to the results of the current study, in which tiafenacil at 28 g ha−1 plus glyphosate at 740 g ha−1 provided a 73% reduction in biomass of the GR tall fleabane. The commercial mixture of saflufenacil + trifludimoxazin alone or in a mixture with glyphosate, glufosinate, or paraquat were the best herbicide treatments to control GR and GS populations of tall fleabane. Limited information is available to compare the response of tall fleabane to saflufenacil + trifludimoxazin-based herbicide mixtures. Trifludimoxazin is the newest herbicide among these PPO inhibitors, and it is being evaluated for possible use as a soil-residual herbicide treatment in cotton (Asher et al. Reference Asher, Dotray, Liebl, Keeling, Ritchie, Udeigwe, Reed, Keller, Bowe, Aldridge and Simon2021).
The results of this study confirm the first report of GR tall fleabane in Australia. Along with GR hairy fleabane and other GR summer weeds (Heap Reference Heap2022), the evolution of GR tall fleabane will be an additional challenge for Australian cotton and grain growers. Because tall fleabane is a prolific seed producer (Hao et al. Reference Hao, Qiang, Liu and Cao2009) and its seeds spread via wind, there is a great chance for the fast spread of GR populations of tall fleabane. Tall fleabane can germinate at temperatures occurring throughout the year in Australia, especially in the eastern cropping region (Mahajan et al. Reference Mahajan, Prasad and Chauhan2021), suggesting that the spread of GR tall fleabane will not be restricted to one season. Both summer and winter fallows are common in Australia and weed control during fallow periods relies primarily on the spraying of glyphosate. Results suggest that overreliance on glyphosate will further increase the cases of GR tall fleabane. This study also found several effective alternate herbicides for the control of small plants (4-leaf) of GR tall fleabane; however, these herbicides were not effective on large plants (12 to 14 leaf). For large plants, saflufenacil + trifludimoxazin alone or in mixtures with glyphosate, glufosinate, and paraquat were the best herbicide treatments. Mixing herbicides with different sites of action may slow down the evolution of resistance in tall fleabane. In addition to this, integrated management options need to be used to reduce the evolution of resistance, and such options should take into account nonchemical means, such as tillage and crop competition. For example, burying seeds below their maximum depth of emergence (i.e., >2 cm) could help manage GR tall fleabane (Mahajan et al. Reference Mahajan, Prasad and Chauhan2021).
Future research should evaluate the impact of tall fleabane on crop production. There is a need to screen tall fleabane populations across Australia for understanding the level of resistance to glyphosate. There is also a need to evaluate the performance of sequential herbicides (i.e., commonly known as the double-knock technique in Australia) on GR tall fleabane populations. In addition, in-crop herbicide options also need to be evaluated, especially in glyphosate-resistant cotton crops.
Acknowledgments
Thanks to the Grains Research and Development Corporations for funding this research. No conflicts of interest have been declared. The author thanks Albert Wong for helping in the gene sequencing experiment.