Introduction
The gravel snail, Lithoglyphus naticoides (Pfeiffer, 1828), is native to the western part of the Black Sea, from where it colonized Europe in the second half of the 19th century (Bij de Vaate et al., Reference Bij de Vaate, Jazdzewski, Ketelaars, Gollasch and Van der Velde2002). Now, this species is a common component of various ecosystems of rivers, water reservoirs and lakes. In Lithuania, L. naticoides was mainly found in running waters, mostly in sites at the Nemunas River and also at some of its tributaries not far upstream from their confluences. The lentic sites where this species was detected are located in the Curonian Lagoon, and Kaunas and Elektrėnai water reservoirs (Butkus et al., Reference Butkus, Šidagytė, Rakauskas and Arbačiauskas2014). In Hungary, L. naticoides is widely distributed in the Danube River and its main tributaries (Bódis et al., Reference Bódis, Borza, Potyó, Puky, Weiperth and Guti2012).
Lithoglyphus naticoides is known to serve as first intermediate host for several trematode species (Zdun, Reference Zdun1961; Odening, Reference Odening1971; Chernogorenko, Reference Chernogorenko1983; Stanevičiūtė et al., Reference Stanevičiūtė, Petkevičiūtė and Kiselienė2008). Some parasite species, namely Nicolla skrjabini (Iwanitzky, 1928), Apophallus muehlingi (Jagerskiold, 1898) and Apophallus (=Rossicotrema) donicus (Skrjabin & Lindtrop, 1919), are highly specific to their first intermediate host, L. naticoides, and disperse together with snails (Zhokhov & Pugacheva, Reference Zhokhov and Pugacheva2001; Tyutin & Slynko, Reference Tyutin and Slynko2010). However, a number of cercariae developing in this snail are still not associated with adult stages of digeneans and their definitive hosts are unknown.
Morphologically different cercariae of cercariaeum type (without a tail) were found in L. naticoides snails by different authors (Zdun, Reference Zdun1961; Odening, Reference Odening1971; Chernogorenko, Reference Chernogorenko1983). They were described under different provisional names (see also the checklist provided by Cichy et al., Reference Cichy, Faltýnková and Żbikowska2011) and their identification and comparison based on morphological characters is very complicated due to the incomplete and sometimes erroneous descriptions. Frequent instances of repeatedly describing the same species have caused difficulties when attempting to compare data from various authors. The main consequence of using many synonyms is an unclear view of the digenean biodiversity in the European populations of molluscs.
While studying the larval trematodes associated with L. naticoides inhabiting different water bodies in Central and East Europe, we detected two morphologically different forms of lissorchiid cercariaeum developing in rediae. One of them, found in Kaunas reservoir and Elektrėnai reservoir, Lithuania, and in Lake Balaton, Hungary, was identified as Palaeorchis sp., and the other, obtained from snails from the Danube River, Hungary, conformed to the morphological characteristics of the genus Asymphylodora. The taxonomic status and actual diversity of the cercariaeum species developing in Lithoglyphus snails is not clear and deserves more comprehensive phylogenetic studies using molecular and/or karyological markers.
Herein, we provide new rDNA reference sequences for two different cercariaeum developing in L. naticoides and compare this information with the existing data for lissorchiid species. Sequences of adult Palaeorchis incognitus Szidat, 1943 obtained from the fish host, Rutilus rutilus(Linnaeus, 1758) and Asymphylodora progenetica (Sercova & Bychovsky, 1940) from the prosobranch snail Bithynia tentaculata (Linnaeus, 1758) were generated for the first time and used for comparative analysis. The chromosome set structure of larval Palaeorchis was studied. Our results provide new insights into the phylogenetic relationships of lissorchiid genera that have not been extensively characterized with respect to their position on molecular phylogenetic trees. The developmental stages of P. incognitus in the first intermediate host were verified with the aid of molecular evidence.
Material and methods
Specimens
Gravel snails were collected by hand in Kaunas (54°51′38″N, 24°09′07″E) and Elektrėnai (54°77′99″N, 24°65′87″E) water reservoirs, Lithuania, and in Lake Balaton (46°58′72″N, 17°57′44″E) and the Danube River (47°49′17″N, 19°00′43″E), Hungary. In the laboratory, snails were dissected and intramolluscan stages were examined alive unstained under slight coverslip pressure. Adult specimens of P. incognitus were recovered from the intestine of European roach, R. rutilus, caught in the Kaunas water reservoir, placed in saline solution, and identified in vivo. The morphology of the adult worms agreed well with the morphological characteristics of this species provided in the relevant identification keys to parasites of fish (Bykhovskaya-Pavlovskaya & Kulakova, Reference Bykhovskaya-Pavlovskaya, Kulakova and Bauer1987; Niewiadomska, Reference Niewiadomska2003). Photomicrographs of live adult and larval (redial and cercarial) isolates were taken with a digital camera mounted on an Olympus BX51 microscope (Olympus Corporation, Tokyo, Japan)(fig. 1a–c) and used for further identification. Drawing of the cercaria is based on digital photographs of live cercariae. Measurements are in micrometres and are presented as a range, followed by a mean in parentheses. Progenetic specimens of A. progenetica were obtained from B. tentaculata, collected in two nameless ponds (54°74′80″N, 25°27′77″E and 54°75′24″N, 25°29′22″E) in Vilnius, Lithuania. For molecular analyses of intramolluscan stages, cercariae and rediae were pooled from one single infected snail host and fixed in 96% ethanol.
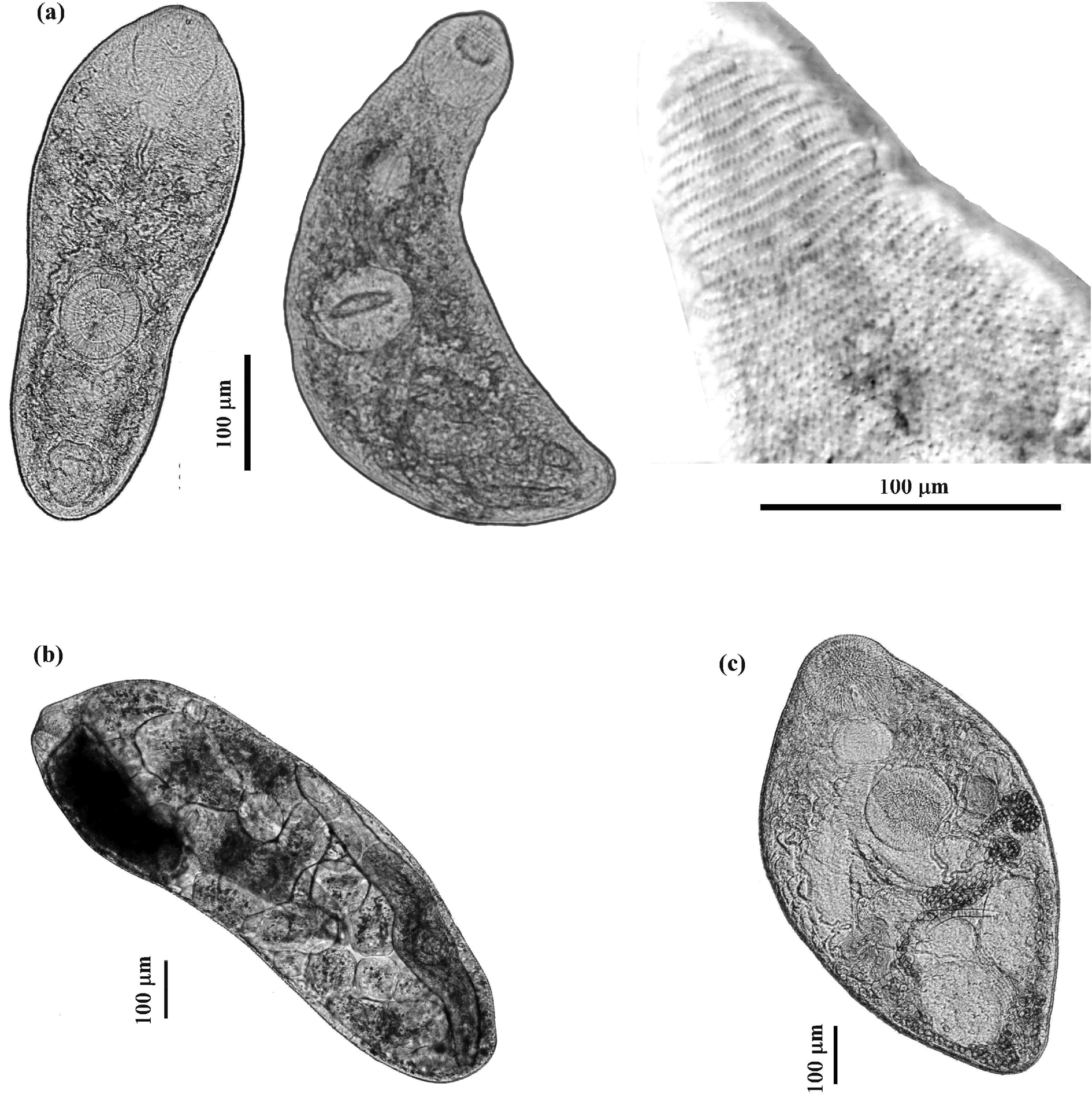
Fig. 1. Photomicrographs of live developmental stages of Palaeorchis incognitus: (a) two specimens of cercariae and tegumental spines of the anterior part of cercarial body; (b) rediae; (c) adult.
Karyological analysis
Samples of Palaeorchis sp., used for karyological analysis, were obtained from gravel snails collected in the Kaunas reservoir. Living snails were incubated in 0.01% colchicine in well water for 3–5 h at room temperature. Tissues containing parthenitae (rediae and cercariaeum) were dissected and treated in distilled water for hypotony for 40–50 min. The material was then fixed in fixative (ethanol:glacial acetic acid, 3:1) with three changes, 20 min each. Slide preparations of mitotic chromosomes were made using an air-drying technique (Petkevičiūtė et al., Reference Petkevičiūtė, Kudlai, Stunžėnas and Stanevičiūtė2015). Slides were stained with 4% Giemsa solution (pH 6.8) for 30–40 min and examined with an Olympus BX51 microscope using a 100× oil immersion objective. Chromosome measurements were made of the seven best mitotic spreads out of 36 analysed cells chosen from parthenitae from three infected snails. Measurements (absolute length in micrometres, relative length in percentage and centromeric indices) are given as mean values and standard deviations. The terminology used to describe the centromere position follows that of Levan et al. (Reference Levan, Fredga and Sandberg1964).
DNA isolation, polymerase chain reaction (PCR) amplification and DNA sequencing
Genomic DNA for molecular analysis was extracted from ethanol-fixed helminths following the protocol of Stunžėnas et al. (Reference Stunžėnas, Petkevičiūtė and Stanevičiūtė2011), with a slight modification described in Petkevičiūtė et al. (Reference Petkevičiūtė, Stunžėnas and Stanevičiūtė2014). DNA fragments spanning the 3′ end of the 5.8S rRNA gene, the complete internal transcribed spacer 2 region (ITS2) and a small section at the 5′ end of the 28S gene were amplified using universal primers for flatworms, the forward primer 3S (5′-CGG TGG ATC ACT CGG CTC GTG-3′) (Bowles et al., Reference Bowles, Blair and McManus1995) and the reverse primer ITS2.2 (5′-CCT GGT TAG TTT CTT TTC CTC CGC-3′) (Cribb et al., Reference Cribb, Anderson, Adlard and Bray1998). Primer pair AlJe-F (5′-GTC TGG CTT GGC AGT TCT A-3′) and AlJe-R (5′-CTG CCC AAT TTG ACC AAG C-3′), designed for species of the Allocreadiidae (Petkevičiūtė et al., Reference Petkevičiūtė, Stunžėnas, Zhokhov, Poddubnaya and Stanevičiūtė2018b)), was suitable to amplify the end of the internal transcribed spacer 1 (ITS1), the complete 5.8S rDNA and ITS2, and also a small section at the 5′ end of the 28S gene of A. progenetica. A fragment at the 5′ end of the 28S rRNA gene was amplified using the forward primers Digl2 (5′-AAG CAT ATC ACT AAG CGG-3′) (Tkach et al., Reference Tkach, Littlewood, Olson, Kinsella and Swiderski2003) or ZX-1 (5′-ACC CGC TGA ATT TAA GCA TAT-3′) (Bray et al., Reference Bray, Waeschenbach, Cribb, Weedall, Dyal and Littlewood2009) and the reverse primers L0 (5′-GCT ATC CTG AG (AG) GAA ACT TCG-3′) (Tkach et al., Reference Tkach, Grabda-Kazubska, Pawlowski and Swiderski1999) or 1500R (5′-GCT ATC CTG AGG GAA ACT TCG-3′) (Olson et al., Reference Olson, Cribb, Tkach, Bray and Littlewood2003; Tkach et al., Reference Tkach, Littlewood, Olson, Kinsella and Swiderski2003). Amplification protocols are as described in Petkevičiūtė et al. (Reference Petkevičiūtė, Stunžėnas and Stanevičiūtė2014). PCR products were purified and sequenced in both directions at BaseClear B.V. (Leiden, Netherlands) using the PCR primers. Contiguous sequences were assembled using Sequencher 4.10.1 software (Gene Codes Corporation, Ann Arbor, Michigan, USA). Sequences generated in this study have been deposited in the GenBank database (see accession numbers in table 1).
Table 1. Species subjected to molecular phylogenetic analysis with information for hosts, localities and GenBank accession numbers.
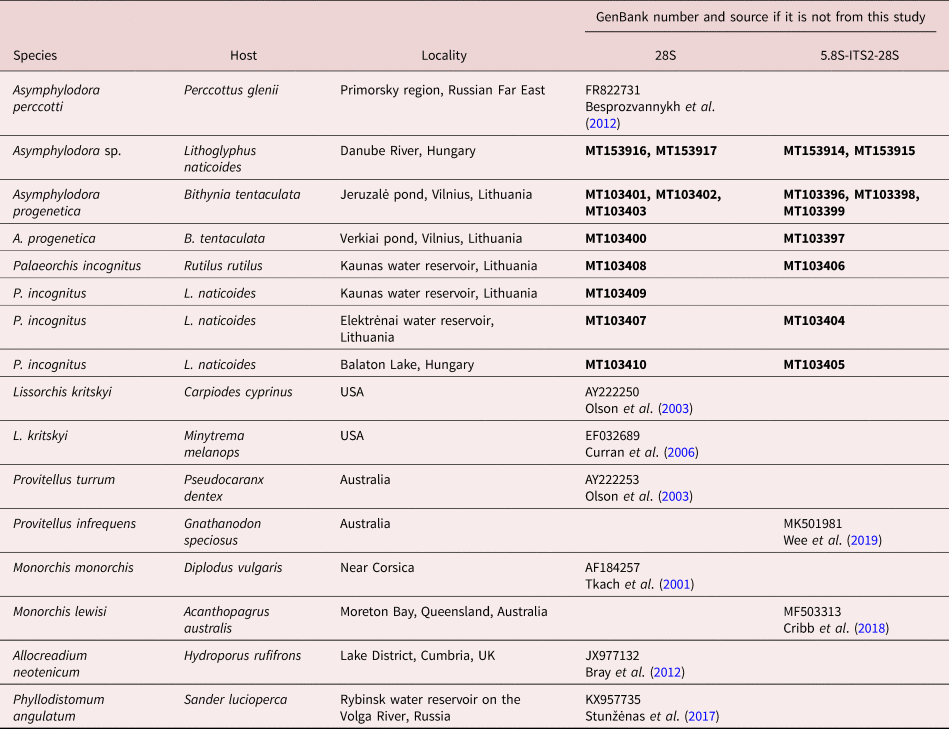
Sequences generated in the present study are indicated in bold.
Molecular phylogenetic analyses
For the phylogenetic analyses, both the ITS2 and 28S datasets were aligned independently using ClustalW (Thompson et al., Reference Thompson, Higgins and Gibson1994) with an open gap penalty of 15 and gap extension penalty of 6.66. The best-fit model of sequence evolution for phylogenetic analysis was estimated using jModeltest version 0.1.1 software (Posada, Reference Posada2008). Maximum likelihood (ML) phylogenetic trees were obtained and analysed using MEGA version 6 (Tamura et al., Reference Tamura, Peterson, Peterson, Stecher, Nei and Kumar2011). Branch support was estimated by bootstrap analyses with 1000 pseudoreplicates. The ML trees were obtained using the general time reversible model with a gamma distribution rate and a proportion of invariant sites (GTR + G + I) for both the ITS2 and the 28S gene datasets. The value for gamma and the number of invariant sites were estimated from the data. Parsimony analysis based on subtree pruning and regrafting was used with default parsimony settings. If two or more sequences belonged to one species, they were collapsed into one branch, except those newly obtained in this study. Estimates of mean evolutionary divergence over sequence pairs within and between groups were calculated using MEGA version 6.
Results
Morphology of cercaria
Palaeorchis incognitus Szidat, 1943 cercaria (figs 1a and 2)
Measurements based on nine specimens. Cercariae developing in elongate-saccular rediae without locomotor appendages (fig. 1b), containing several germ balls and few (mostly three or four) fully developed cercariae. Live cercariae are mobile and contractile. Cercarial body tailless, elongate, oval, with maximum width at level of acetabulum, and measured 390–535 × 160–193 (474 × 174). The body surface is armed with transversal rows of scale-like tegumental spines, covering the forebody, becoming smaller and scarcely distributed posteriorly (up to the post-acetabulum level). Oral sucker round, subterminal, 67–78 × 70–81 (73 × 76), with tiny stylet embedded in the anterior part of the rim. Prepharynx conspicuous; pharynx globular, muscular, 33–37 × 35–41 (35 × 38). Oesophagus long and sinuous, bifurcating at level of anterior border of acetabulum; intestinal caeca terminating blindly in the third quarter of the body. Ventral sucker almost equal in size to oral sucker, round, 68–77 × 70–75 (72 × 71), situated somewhat behind the middle of body. The excretory vesicle oval, thick-walled, with primary collecting ducts extending up above ventral sucker where they form loops and divide into anterior and posterior collecting ducts. Flame cell formula difficult to establish. Subtegumental gland cells large, with large nuclei scattered beneath tegument on both sides, especially numerous at level of acetabulum. Reproductive system poorly developed, anteriorly to excretory vesicle. In some specimens, the cirrus pouch and metraterm could be seen posterolateral to the acetabulum.
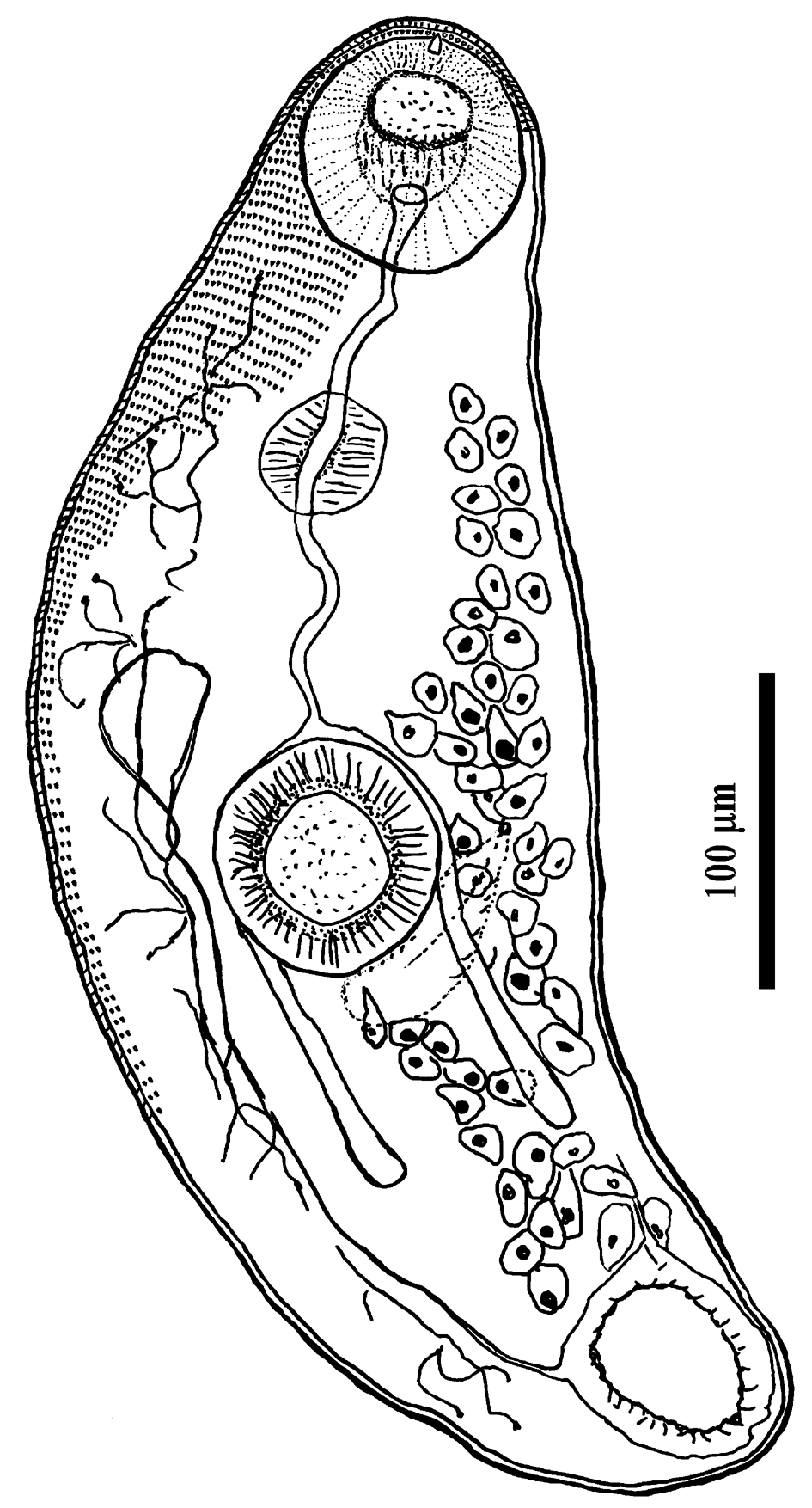
Fig. 2. Cercaria of Palaeorchis incognitus.
Taxonomic summary
First intermediate host. Lithoglyphus naticoides (Pfeiffer, 1828) (Gastropoda: Hydrobiidae).
Site of infection: Digestive gland, gonads and intestinal surface.
Prevalence: 0.47% (May–September 2019, n = 635).
Locality: Kaunas water reservoir, Lithuania (54°51′38″N, 24°09′07″E).
GenBank accession numbers: MT103409 (28S), MT103404 (5.8-ITS2-28S).
Karyotype structure: 2n = 20 = 8st + 4st-sm + 8sm.
Karyotype of P. incognitus
Most dividing mitotic cells (35 from 36 examined) of larval P. incognitus had 20 chromosomes in the diploid complements (fig. 3). One cell was aneuploid, with 19 chromosomes. The chromosomes are comparatively small and gradually decrease in length from 4.86 to 1.49 μm (table 2). The mean total length of the haploid complement is 26.7 μm. Chromosomes with subterminally located centromeres prevail in the karyotype. According to centromere position, only the last four pairs of small chromosomes – 7, 8, 9 and 10 – have submedially located centromeres and are classified as submetacentric. Secondary constriction in pericentromeric region of short arms of the chromosomes constituting pair 9 was observed in most metaphase plates; this constriction is most likely physically associated with the nucleolar organizer and contains rRNA genes.
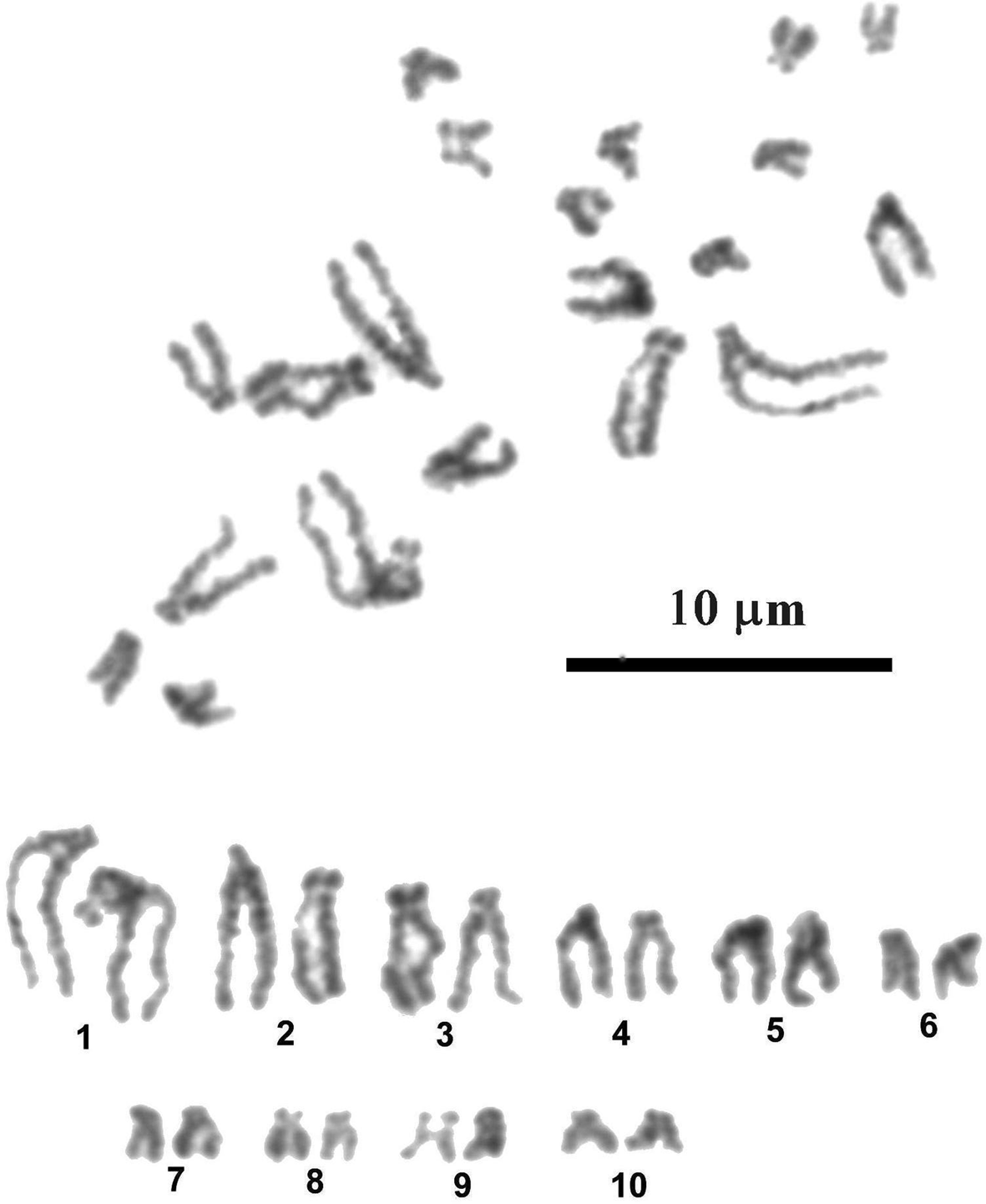
Fig. 3. Mitotic metaphase and karyotype of Palaeorchis incognitus.
Table 2. Measurements (means ± standard deviation) and classification of chromosomes of Palaeorchis incognitus.

sm, submetacentric chromosomes; st, subtelocentric chromosomes.
Molecular analysis and phylogeny
The newly obtained sequences of two different regions of rDNA (ITS2 region and partial 28S gene) of lissorchiid and additional relevant rDNA sequences of lissorchiid and monorchiid trematode species and outgroup taxa were downloaded from GenBank and included in pairwise sequence comparisons and phylogenetic analyses (table 1). An alignment of the ITS2 and partial 28S data sets yielded 654 and 1113 characters for analysis, respectively; a couple sequence of the Allocreadiidae and the Gorgoderidea were used as outgroup. The phylogenetic trees made with the new sequences and the sequences deposited in GenBank are shown in figs 4 and 5. Two genetically different lissorchiid cercariaeum were revealed from L. naticoides. The cercariaeum from Hungary (Balaton Lake) and Lithuania (Elektrėnai and Kaunas water reservoirs) matched exactly the sequences of adult P. incognitus ex European roach, R. rutilus. The other cercariaeum isolate from L. naticoides was detected only in one location, in the Danube River, Hungary. The species of this cercariaeum was identified as Asymphylodora sp. based on the results of molecular data. Differences between two lissorchiids from L. naticoides are 8% (38 nucleotides of 472) and 6.8% (73 of 1159), in ITS2 and partial 28S sequences, respectively. Asymphylodora sp. ex L. naticoides is similar to A. progenetica ex B. tentaculata, collected in Lithuania; diverging 7.8% (39 of 500) and 5.9% (68 of 1159) in ITS2 and partial 28S datasets, respectively. However, it appeared as sister clade to A. progenetica and Asymphylodora perccotti Besprozvannykh, Ermolenko & Atopkin, 2012 in the 28S tree (fig. 4), while the sequences of P. incognitus form distinct robustly supported lineage. In this tree, Lissorchis kritskyi Barnhart & Powell, 1979 forms a separate branch, which clustered with other lissorchiids in one well-supported higher clade. The topography of the ITS2 tree (fig. 5) is identical; however, it contains less species.
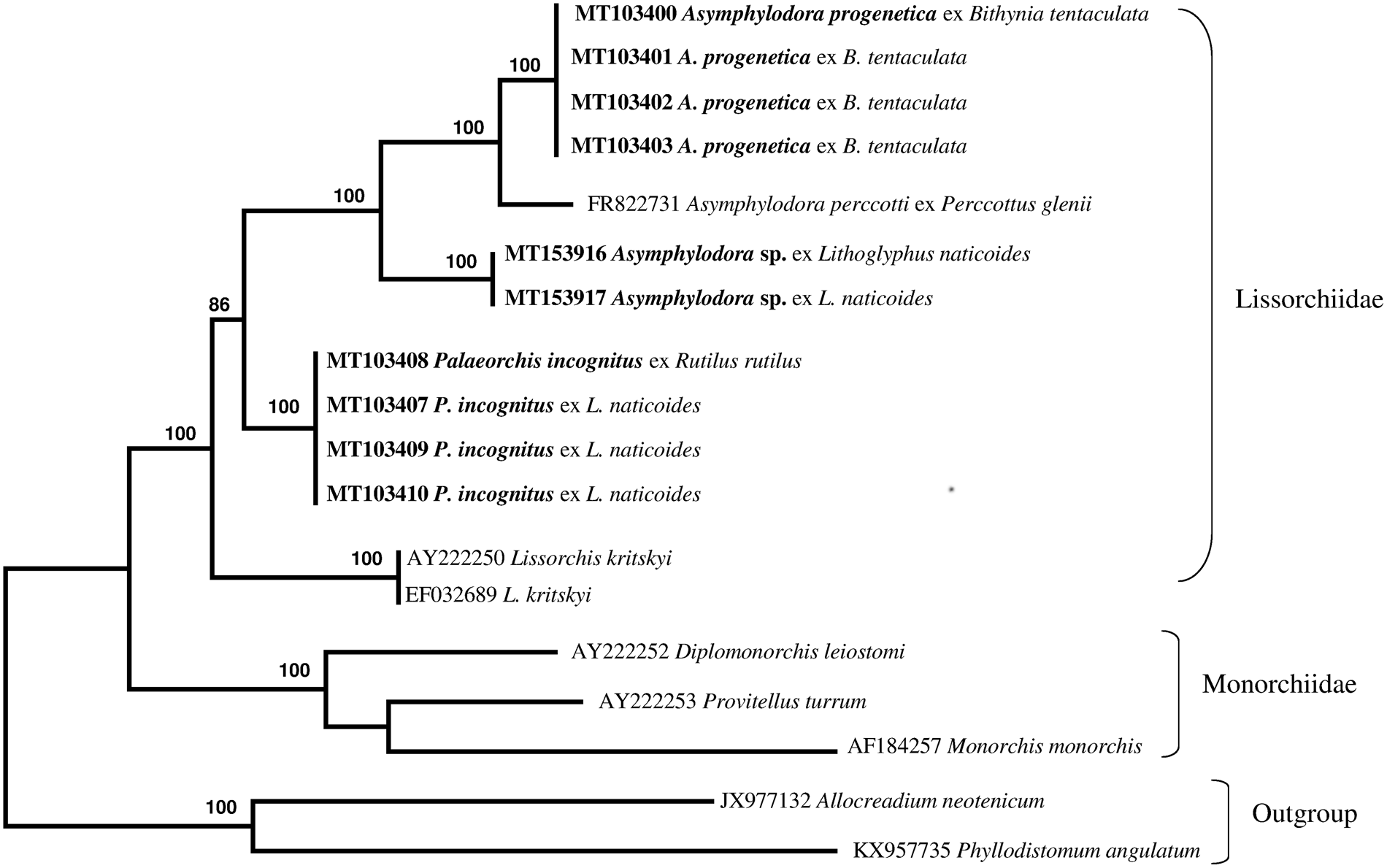
Fig. 4. Phylogenetic tree based on maximum likelihood analysis of partial sequences of the 28S nuclear rDNA gene. Bootstrap support values lower than 70% are not shown. The species sequenced in this study are indicated in bold.
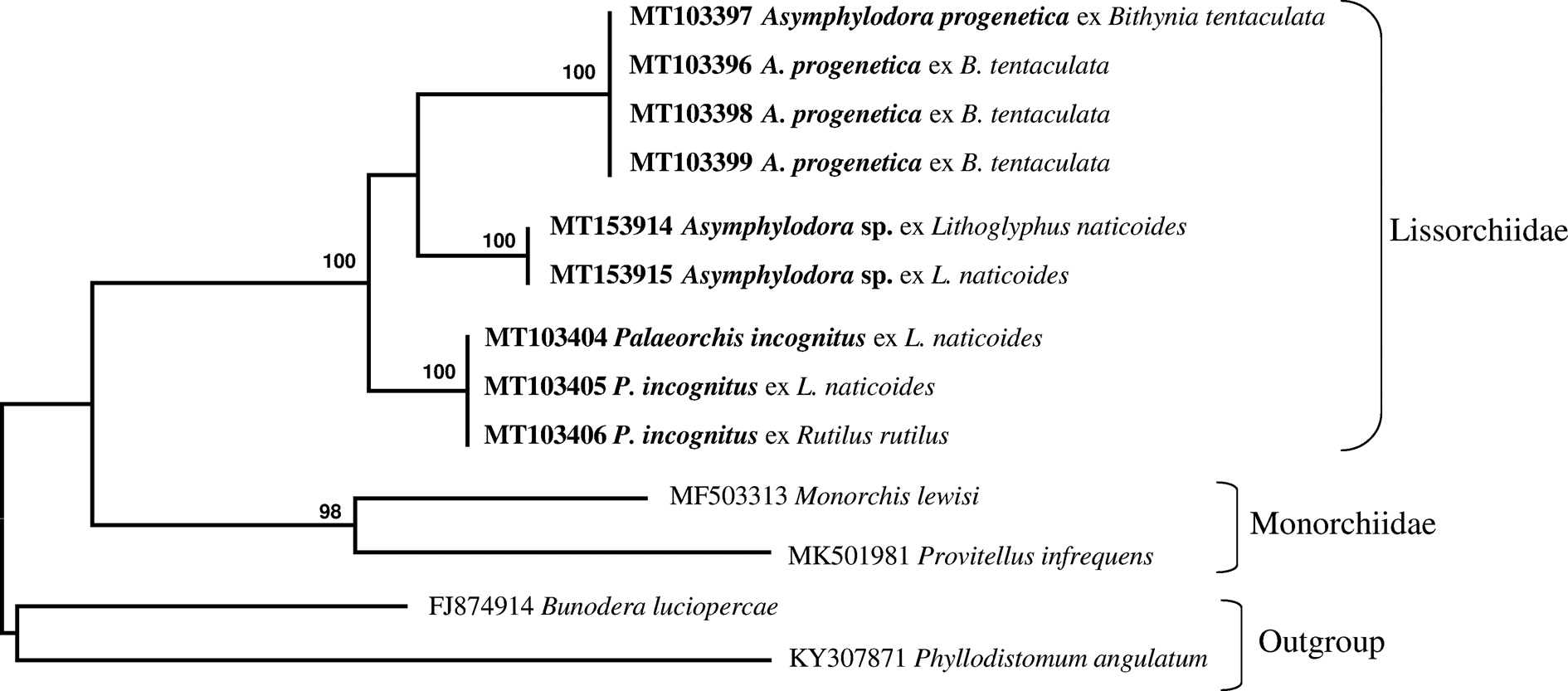
Fig. 5. Phylogenetic tree based on maximum likelihood analysis of the ITS nuclear rDNA region. Bootstrap support values lower than 70% are not shown. The species sequenced in this study are indicated in bold.
Discussion
In Europe, the Lissorchiidae includes the genera Asymphylodora Looss, 1899 and Palaeorchis Szidat, 1943. These trematodes are common and widely distributed parasites of freshwater fish in the Palaearctic region (Bray, Reference Bray, Jones, Gibson and Bray2008), and are well known from fish hosts in Lithuania and neighbouring countries (Rauckis, Reference Rauckis1988; Niewiadomska, Reference Niewiadomska2003; Kirjušina & Vismanis, Reference Kirjušina and Vismanis2007). In older publications, the species of these genera are assigned to the family Monorchiidae Odhner, 1911. Later, Shimazu (Reference Shimazu1992) gave a clear summary of features distinguishing members of Lissorchiidae from monorchiids. Molecular evidence in Tkach et al. (Reference Tkach, Pavlowski, Mariaux, Swiderski, Littlewood and Bray2001) confirmed the independent status of the Lissorchiidae.
Despite the fact that our current knowledge of life cycles is relatively good for trematodes parasitic in European freshwater fish (Faltýnková et al., Reference Faltýnková, Sures and Kostadinova2016), the vast majority of helminth species are known only from their adult stage (Blasco-Costa & Poulin, Reference Blasco-Costa and Poulin2017). Life cycles are known for some freshwater lissorchiid species, which infect pulmonate (heterobranch) and prosobranch gastropods as first intermediate hosts (Stunkard, Reference Stunkard1959; Lambert, Reference Lambert1976; van den Broek & de Jong, Reference van den Broek and de Jong1979; Našincová & Scholz, Reference Našincová and Scholz1994; Besprozvannykh, Reference Besprozvannykh2005; Besprozvannykh et al., Reference Besprozvannykh, Ermolenko and Atopkin2012). Numerous other putative but unidentified freshwater lissorchiid cercariae (non-oculate cercariae of cercariaeum type in rediae in snails) are known from different gastropods.
Similarly sized (morphometric) parameters and the absence of contrasting characters in rediae and cercariae of closely related species are sometimes compounded by contradicting descriptions by different authors. In many cases, it is impossible to correlate the morphological data on intramolluscan stages with those of their adults; moreover, sometimes it was even difficult to establish the attribution of particular larvae to any trematode family. Thus, the widespread enigmatic species Cercariaeum crassum Wesenberg-Lund, 1934, developing in rediae in the sphaeriid clam Pisidium amnicum (Müller, 1774), was incorrectly assigned to the lissorchiid genus Palaeorchis as Palaeorchis sp. or Palaeorchis crassus in some publications (see Zhokhov & Pugacheva, Reference Zhokhov and Pugacheva1995; Holopainen et al., Reference Holopainen, Lamberg, Valtonen and Rantanen1997; Rantanen et al., Reference Rantanen, Valtonen and Holopainen1998). Subsequent comprehensive morphological and then karyological and molecular analysis corroborate the allocation of C. crassum to Allocreadium Looss, 1900 in the Allocreadiidae (Niewiadomska & Valtonen, Reference Niewiadomska and Valtonen2007; Petkevičiūtė et al., Reference Petkevičiūtė, Stunžėnas and Stanevičiūtė2012).
Recent molecular tools in the form of DNA sequence data provide reliable markers for species identification and are fast dominating in the studies of digenean life cycles. However, not a single life cycle of European Asymphylodora or Palaeorchis species has been proven by molecular methods, and there are many controversies in the data on lissorchiid life cycles known from experimental infections.
Two species of the genus Palaeorchis are known from fish hosts (mainly cyprinids) in Lithuania and neighbouring countries: P. incognitus and Palaeorchis unicus Szidat, 1943 (Rauckis, Reference Rauckis1988; Niewiadomska, Reference Niewiadomska2003; Kirjušina & Vismanis, Reference Kirjušina and Vismanis2007). Their life cycles are not elucidated. Szidat (Reference Szidat1943) assumed that Cercariaeum paludinae impurae f. armatum de Filippi, 1854 from B. tentaculata is the larval stage of P. incognitus. This assumption was based on morphological similarity and was not confirmed under experimental conditions or other evidence. Szidat (Reference Szidat1943) declared that the larvae of Palaeorchis certainly belong in the ‘Helveticum’ group of Dubois (Reference Dubois1929) (these larvae develop in rediae; they have two testes; the excretory vesicle is tubular and flexible). In his study of trematodes using the snail L. naticoides as first intermediate host in Lake Müggelsee (Berlin), Odening (Reference Odening1971) described cercariaeum developing in rediae identified as ‘? Cercariaeum papillosum Mödlinger, 1934’, which closely resembles our cercariaeum of P. incognitus in having the tailless body and two suckers of equal size. The oral sucker bears a tiny stylet. The anterior part of the body is armed with tegumental spines that are inconspicuous posteriorly. The prepharynx is conspicuous, the pharynx is almost globular and the oesophagus is long, flexuous, bifurcating in front of the acetabulum. The excretory vesicle is thick-walled, elongate-oval, flexible. Cystogenic gland cells numerous. The genitalia are not clearly visible. Daughter rediae with only a few developing cercariae and several germ balls (in older specimens).
Tyutin & Slynko (Reference Tyutin and Slynko2010) found rediae and cercariae morphologically similar to the local species Parasymphylodora (=Asymphylodora) markewitschi (Kulakowskaja, 1947) in 15.5% of examined L. naticoides, which usually had a thin-walled shell, as compared to uninfected individuals.
Comparative ITS2 and 28S sequence analysis carried out by us confidently confirmed the link between redial and cercarial isolates ex L. naticoides from different populations in Lithuania and Hungary, and the adult stages of P. incognitus parasitizing R. rutilus from Kaunas water reservoir, supporting their conspecificity. No intraspecific variation was detected between these isolates. In our analysis, representatives of three genera of Lissorchiidae produced strongly supported clades. Palaeorchis is represented in the analysis by just one species, which forms a distinct genetic lineage. Cercariaeum obtained from snails collected in the Danube River clusters together with representatives of the genus Asymphylodora. The species identification of this cercariaeum is not presently possible, but the collection locality, host record and sequence data will all be valuable reference information for the future collection of adults. The number of DNA sequences deposited in GenBank is rapidly increasing and molecular analysis represents a straightforward method to approach questions related to the species identity of larval stages. Redial and sexually mature stages of A. progenetica were originally described from B. tentaculata collected near Leningrad, Russia (Serkova & Bychovsky, Reference Serkova and Bychovsky1940). The life cycle of this species is truncated to one host, and the mollusc is used as a definitive host as well, or another pathway exists in which trematodes mature in fish (Kulakova, Reference Kulakova1982). Despite the altered life cycle, this species shows close affinities with A. perccotti from the Russian southern Far East, which lacks the capacity for progenesis (Besprozvannykh et al., Reference Besprozvannykh, Ermolenko and Atopkin2012).
It should be noted that adult P. incognitus was detected only in those Lithuanian water bodies where the L. naticoides was also found – that is, the Nemunas River, Curonian lagoon and Kaunas water reservoir (Rauckis, Reference Rauckis1988). Palaeorchis incognitus was also recorded from fish hosts from the four localities under investigation in the Danube River system in Central Europe (Moravec et al., Reference Moravec, Konecny, Baska, Rydlo, Scholz, Molnár and Schiemer1997), where L. naticoides is widely distributed. On this basis, it can be inferred that P. incognitus is specific to its first intermediate host. A direct consequence of species specificity of parthenitae in many species of trematodes is almost a complete coincidence of their ranges with the ranges of molluscan hosts.
Prior to the present study, sequence data were available for only two lissorchiid species from the Russian southern Far East and from the south-eastern region of the US: A. perccotti and L. kritskyi, respectively (Olson et al., Reference Olson, Cribb, Tkach, Bray and Littlewood2003; Besprozvannykh et al., Reference Besprozvannykh, Ermolenko and Atopkin2012). This study contributed novel molecular data from three additional lissorchiid species in two genera. Molecular data from P. incognitus represent the first such available data for the genus. Here, the generation of ITS2 rDNA sequences for three lissorchiids represents all available data for the family. In the main, the new sequences reported here serve to improve the dataset for future analyses of diversity, life cycles and phylogenetic relations of lissorchiid species.
Karyological features, as well as molecular data, may indicate the evolutionary distance between species, which may not be obvious at the morphological level, and provide helpful markers that can serve as species-specific characteristics (White, Reference White1978; Petkevičiūtė et al.,Reference Petkevičiūtė, Stunžėnas and Stanevičiūtė2014, Reference Petkevičiūtė, Stunžėnas and Stanevičiūtė2018a). In general, comparative data based on different levels of organization provide a more comprehensive understanding of the evolutionary processes of species divergence and phylogenetic relations. Unfortunately, chromosomal analysis is rarely explored in taxonomic and phylogenetic studies of parasitic flatworms.
The chromosome number and morphology of only five lissorchiid species have been analysed. Diploid chromosome sets of three unidentified species of larval Asymphylodora, developing in B. tentaculata, comprise 20 or 22 elements with two or one pair of large metacentric elements, respectively (Baršienė, Reference Baršienė1993). The karyotype with 2n = 20 and with similar chromosome morphology was described for unidentified larval stages of lissorchiid from the snails Melanopsis dufouri (Férussac, 1823) collected in Spain (Baršienė et al., Reference Baršienė, Roca, Tapia and Martin1995). Apparently, Asymphylodora spp. form a group of karyologically closely related species. The karyotype with 2n = 20 presumably arose through the centromeric fusion of two acrocentric non-homologous chromosomes (Robertsonian translocation). The only karyotype of Palaeorchis sp. known so far from B. tentaculata includes 14 chromosomes and does not show any similarity to the chromosome sets of Asymphylodora species (Baršienė, Reference Baršienė1993). The chromosome set revealed for P. incognitus in this study, with 2n = 20, clearly differs from the karyotype of its congener with 2n = 14. It resembles the chromosome sets of Asymphylodora spp. in diploid number and shows very close relative length values compared to Asymphylodora sp. 1 described by Baršienė (Reference Baršienė1993), but differs in centromere position of corresponding chromosome pairs. Such variation in centromere position of corresponding chromosomes of related species is most easily explained by pericentric inversions. Cytogenetic theory leads to the assumption that the more derived species usually evolve a karyotype consisting of biarmed elements, while a ‘primitive’ karyotype within a given phyletic group consists, typically, of acrocentric chromosomes (White, Reference White1978; Birstein, Reference Birstein1987). In view of this, the karyotype of P. incognitus, consisting of predominantly subtelocentric chromosomes, should be regarded as quite ‘primitive’ in comparison with karyotypes of Asymphylodora spp. composed predominantly of meta- and submetacentric elements. Hence, the karyotypic structure of P. incognitus is consistent with its position in the 28S-based phylogenetic tree, where it occupies a basal position in relation to Asymphylodora spp. It is notable that trematodes are fairly conservative karyologically, and species related from a morphological point of view most often show close karyological affinities. The existence of radically different karyotypes in the congeneric species of digenean trematodes is an unusual and intriguing phenomenon. It is likely that if karyotype features are plotted over a phylogenetic tree based on molecular data, the processes involving chromosome evolution might be clarified.
The results of the present study have shown that the analysis of larval trematodes, including karyological studies and, in particular, molecular sequencing, can provide markers to identify species and to elucidate life cycles and, finally, determine the true biodiversity of the digeneans in the European populations of molluscs.
Acknowledgements
We are grateful to Dr Erica Bódis and Bence Tóth (Hungarian Danube Research Station of the Hungarian Academy of Sciences) for their help with the collection of molluscs in the Danube River.
Financial support
This research was funded by the Research Council of Lithuania (grant numbers LEK-10/2010 and MIP-43/2015).
Conflicts of interest
None.
Ethical standards
All applicable institutional, national and international guidelines for the care and use of animals were followed. All procedures performed in studies involving animals were in accordance with the ethical standards of the institution or practice at which the studies were conducted.