Introduction
The increase of agriculture and livestock production has made a great contribution to the expanding demands of crops and animal-based products. In 2014, 7.8% fewer people worldwide suffered from undernourishment than that of 25 years ago (FAO, 2015). Inevitably, agricultural and animal wastes are generated in large amounts on farms. As the world's largest animal producer, China alone generates ~4 billion tons of animal manure per year (Guo et al., Reference Guo, Peng, Gong and Xia2012). More than 40% of manure is not properly discharged (Ministry of Agriculture of China, 2016). The nitrogen (N), phosphorus (P) compounds and odors associated with animal manure are polluting the soil, water and air in the farm (Yang et al., Reference Yang, Li, Tang, Zhang, Yu, Shen and Zhao2017). However, the manure in large amounts from different animal species in farms has the potential to yield biogas (Shen et al., Reference Shen, Huang, Yang and Han2015). For crop waste in China, the annual total straw production was ~900 million tons (Ministry of Agriculture of China, 2016), more than 30% of straw was burned by farmers in fields as a traditional disposal way (Wang and Zhang, Reference Wang and Zhang2008), which causes the emissions of greenhouse gases, i.e., carbon dioxide (CO2), nitrous oxide (N2O), particulate matters (PM) and toxic gases, i.e., carbon monoxide (CO), ammonia (NH3), sulfur dioxide (SO2). All of them have increased by 500% from 1990 to 2013 (Li et al., Reference Li, Li, Bo and Xie2016), and they are believed to have negative effects on climate, human health and ecosystem. Environmental issues caused by large amounts of agricultural and animal waste are challenging the sustainability of agricultural and animal productions in China and all over the world.
Without effective management of those kinds of wastes, pollutants released from animal manure and straw burning would result in unprecedented environmental challenges, such as water, soil and air contamination and related human health threats. However, agricultural and livestock organic waste biomass are considered as valuable resources of biogas to generate electricity and reduce the greenhouse gas emissions (Li et al., Reference Li, Li, Bo and Xie2016). They can also be used as feedstock for the production of organic fertilizers, which are potential alternatives to chemical ones (Molina et al., Reference Molina, Soriano, Ingelmo and Llinares2013). It is also reported that anaerobic digestion (AD) will become a practical necessity to widespread water quality problems due to its cost effectiveness and energy saving aspects (Speece, Reference Speece1996).
Organic wastes can be converted into renewable energy methane (CH4) and organic fertilizers under the treatment of AD (Lin and Li, Reference Lin and Li2017). In China, it was estimated that ~27,000 medium- and large-scale biogas plants were constructed in 2010 (Song et al., Reference Song, Zhang, Yang, Feng, Ren and Han2014). However, a considerable volume of biogas digestate was produced from anaerobic biogas plants, which resulted in serious environmental problems (Li et al., Reference Li, Zhang, He, Zhang, Liu, Chen and Liu2014). Excessive land application of liquid digestate or uncontrolled decomposition could lead to environment challenges such as water, soil and air contaminations.
Previous research indicated that liquid anaerobic digestion (LAD) digestate is one of the best sources of inoculums, because it was enriched in actively acclimated methanogens (Yang et al., Reference Yang, Xu, Ge and Li2015). Inoculated with LAD digestate, biogas production was improved from lignocellulosic biomass wastes at solid state anaerobic digestion (SSAD) (Shi et al., Reference Shi, Wang, Stiverson, Yu and Li2013). SSAD has an advantage of reducing wastewater production compared with LAD, and it produces a manageable fertilizer for land application (Ahn et al., Reference Ahn, Smith, Kondrad and White2010).
Anaerobic co-digestion (AcoD) is a process which operated with at least two substrates and it has been considered as a promising alterative to improve the bioreactors performance in comparison with mono-digestion systems (Hagos et al., Reference Hagos, Zong, Li, Liu and Lu2017). SSAD of agricultural residues involves the hydrolysis of cellulosic biomass to fermentable small organic compounds such as sugars and volatile fatty acids (VFA) which are further fermented to CH4 and CO2. Although recent research on AcoD of digestate and agricultural biomass in SSAD is conducted and the results are shown in Table 1 (Lin and Li, Reference Lin and Li2017; Li et al., Reference Li, Xu, Li, Lu, Shah, Zhang, Zhang, Gong and Li2018, Reference Li, Zhou, Lu and Nges2019; Wang et al., Reference Wang, Li, Chi, Sun, Zhang, Jiang and Cui2018), few studies have focused on reuse of liquid digestate of digested swine wastewater and corn stover through thermophilic co-digestion with switchgrass, which is an interesting model of lignocellulosic plant for CH4 yield (Frigon et al., Reference Frigon, Mehta and Guiot2012). The objectives of this study are: (1) exploit new environment beneficial strategy to reuse liquid digestate by co-digestion with agricultural biomass and (2) study the operation to learn the performance of thermal SSAD, in terms of reaction time, biogas production, stability of the bioprocess and chemical properties of the digested switchgrass.
Table 1. The comparison of the working conditions in solid state AcoD of agricultural biomass in recent 3 years.

AcoD, anaerobic co-digestion; LAD, liquid anaerobic digestion; VS, volatile solids; TS, total solids.
Materials and methods
Substrate and inoculum preparation
The switchgrass was obtained from the fields in Clayton, a town in Johnston County, North Carolina, USA, and dried in air, chopped to the size of 3 cm, and stored in sealed plastic bags at room temperature. Liquid digestate of digested swine wastewater and corn stover (2 mm) was taken from a 12 L continuously stirred tank reactor (CSTR, model number: MGF-214, New Brunswick Scientific Company, Enfield, Connecticut, USA) operated with an organic loading rate of 1.04 ± 0.02 kg volatile solid (VS) per m3 per day, and stored at 4°C in a refrigerator as the inoculums for SSAD. The CSTR which had an average biogas production of 7.6 ± 0.9 L day−1, had been operated for months at the working temperature of 50°C, and the hydraulic retention time was 10 days (Liu, Reference Liu2017). The characteristics of switchgrass and LAD digestate are given in Table 2.
Table 2. The properties of switchgrass, and liquid digestate as the inoculums (mean ± s.d.).
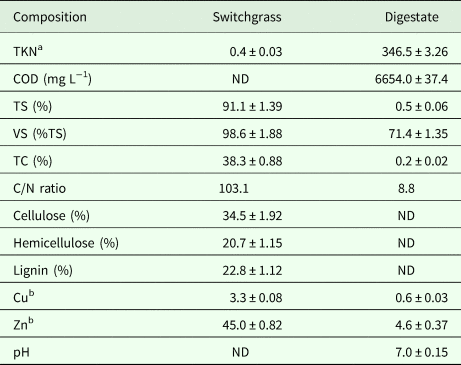
s.d., standard deviation (calculated from triplicate values of the same determined sample); TKN, total kjeldahl nitrogen; COD, chemical oxygen demand; TS, total solids; VS, volatile solids; TC, total carbon; C/N, carbon to nitrogen; Cu, copper, Zn, zinc; ND, not determined.
a % and mg L−1 for switchgrass based on dry weight and biogas digestate, respectively.
b mg kg−1 and mg L−1 for switchgrass (dry weight) and the digestate, respectively.
Bioreactor setup and operation
Two bioreactors with a working volume of ~15 L were used. Each reactor was equipped with a wet-tip gas meter (Archae Press, Nashville, Tennessee, USA) and calibrated to measure the volume of biogas production. A device at the bottom of the digester was used to collect and recirculate the leachate to the top of reactor using peristaltic pumps (Cole Palmer, Vernon Hills, IL, USA) for improving efficiency of the digestion. The temperature of the water in the hot water jacket was maintained at 50°C using a hot water thermostat (VWR International, Brooklyn, NY, USA) which circulated the hot water in jackets around the reactors (Fig. 1). The chopped switchgrass (1.4 kg) was loaded into each reactor and 4 L pre-mixed LAD digestate was poured from the top of each reactor as inoculum. Nitrogen gas (N2) was aerated into the reactors to create anaerobic conditions from the bottom after the reactors were sealed. The inoculums/feedstock ratio was 3/1 based on weight and the initial total solid (TS) content was 24%.
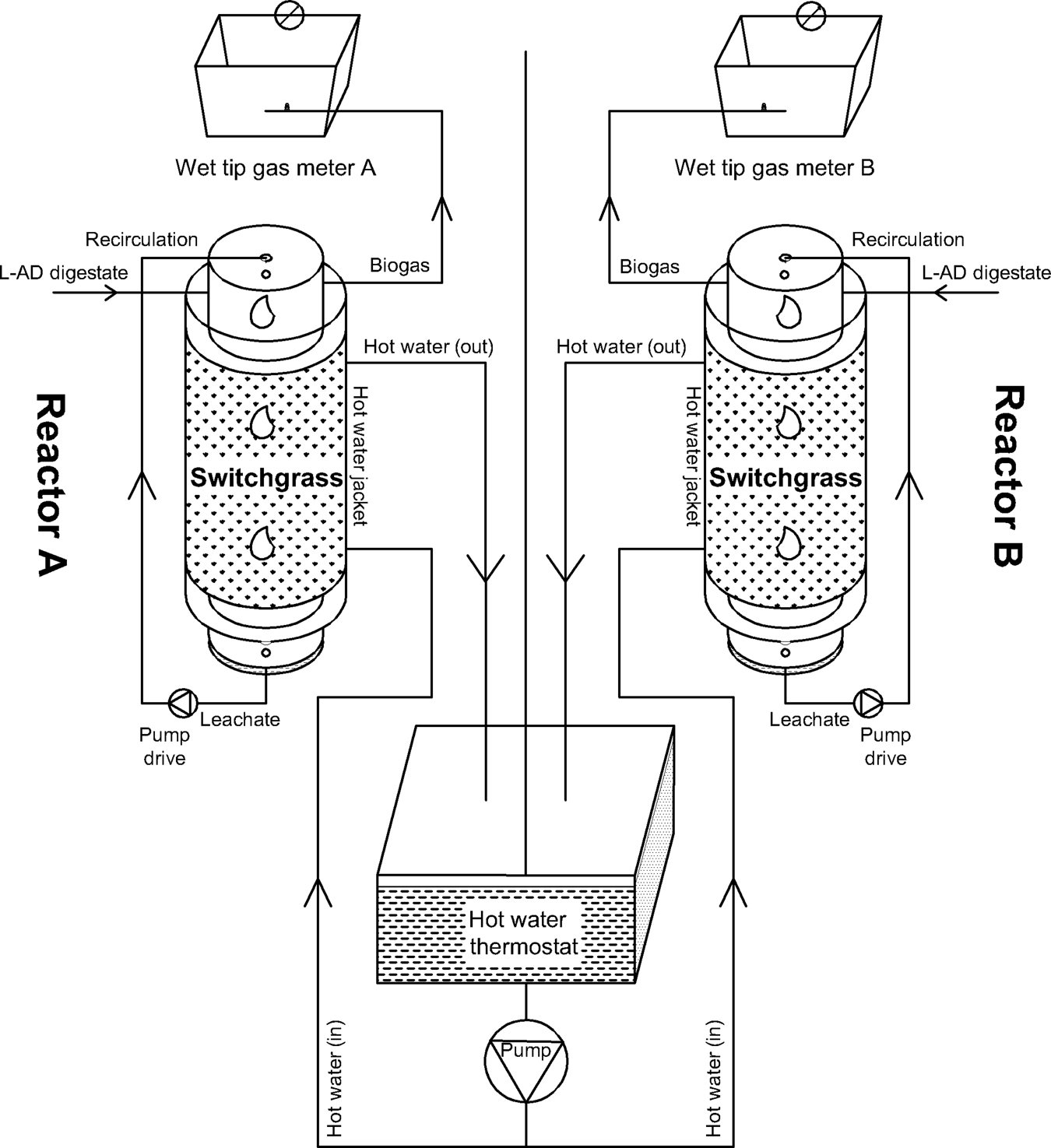
Fig. 1. Schematic of the solid state AcoD set-up.
Analytical procedure and methods
Total kjeldahl nitrogen (TKN), total phosphorus, TS and VS, total organic carbon (TOC) and total carbon (TC), carbon/nitrogen (C/N) ratio, chemical oxygen demand (COD) of liquid digestate, raw switchgrass or digested residues, and the pH values were determined by standard methods (APHA, 1998); organic matter (OM) was calculated based on TOC multiplied by 1.724, and total nutrients (TN) contents were determined according to the contents of N, phosphorus pentoxide (P2O5) and potassium oxide (K2O) (Ministry of Agriculture of China, 2012). The pH value of liquid digestate, final leachate and digested residues was determined using a pH meter (Mettler-Toledo FG2, Switzerland). Copper (Cu), zinc (Zn), cadmium (Cd), lead (Pb) and chromium (Cr) of digested switchgrass, digestate and leachate were analyzed by atomic absorption spectroscopy (Atomic absorption spectrometer 3100, PerkinElmer, German) after digestion with concentrated hydrochloric acid and nitric acid (HCl-HNO3). Potassium (K) of digested residues was analyzed using atomic emission spectroscopy (Atomic absorption spectrometer 3100, PerkinElmer, German). Biogas production was recorded daily using a wet-tip gas meter and biogas composition was determined by gas chromatograph (GC-17A, Shimadzu Corporation, Kyoto, Japan) equipped with a thermal conductivity detector, and the 100/120 Carbosieve SII packing was used as the column. The temperature of column was set at 50°C, and it was 250 °C for the detector. Helium (He) was used as the carrier gas at a flow rate of 30 mL min−1, and the injection volume was 3 mL. Cellulose, hemicellulose and lignin contents of pre- and post-digested switchgrass were determined according to the National Renewable Laboratory Analytical Procedure (Sluiter et al., Reference Sluiter, Hames, Ruiz, Scarlata, Sluiter, Templeton and Crocker2012). The concentrations of acetic acid and butyric acid of leachate were analyzed by high performance liquid chromatography (Prominence Series HPLC with a refractive index (RI) detector, model RID-10A, Shimadzu Corporation, Kyoto, Japan) using an Aminex HPX-87H column (300 × 7.8 mm, Bio-Rad, CA, USA) with a column temperature of 80°C. And 0.005 N sulfuric acid (H2SO4) was used as the mobile phase at a flow rate of 0.6 mL min−1. Detection was accomplished with the RI detector at the oven temperature of 40°C.
Statistical analysis
The means of acetic acid and butyric acid concentration within each bioreactor, were statistically evaluated by one-way analysis of variance using the GLM procedure of SAS (SAS 8.01 Institute, Cary, NC), and the average differences among different operation times were compared by Duncan's multiple range tests. A P-value of <0.05 was considered significant.
Results and discussion
Bioreactors’ start-up, biogas production, methane content and variations of pH and acids
Switchgrass and liquid digestate of swine waste water, and corn stover were loaded to each of the two bioreactors (reactors A and B) as illustrated in Figure 1. Both reactors and their recycled leachate were kept at 50°C. The total liquid digestate was completely soaked by switchgrass in 2 days after setup, and then 1 L digestate was poured into each reactor. The overall results showed that the start-up times were more than 2 weeks for both reactors, and it seemed that the SSAD bioreactors were unstable during the first phase of start-up. When the main substrates are energy crops, SSAD tends to fail, because higher organic load rate may likely lead to VFA and ammonia accumulation (Sawatdeenarunat et al., Reference Sawatdeenarunat, Surendra, Takara, Oechsner and Khanal2015). There was a long biomass retention time for both reactors, because the microorganisms were inhibited in digestion due to the recalcitrant structure of lignocellulosic biomass (Budzianowski, Reference Budzianowski2016). Thus the cellulose and hemicellulose are unavailable for fermentation directly (Li et al., Reference Li, Park and Zhu2011). The start-up periods lasted for 16 and 47 days for reactors A and B, respectively. This was in accordance with research suggestion that it took 1 week to months to start-up the SSAD reactor at a high feedstock/effluent (F/E) ratio of 40–50 by buffer addition, and inoculation was the main effect in the start-up period of SSAD (Motte et al., Reference Motte, Escudie, Bernet, Delgenes, Steyer and Dumas2013). The digestate from LAD established desirable digestion microbial community to feedstock, which is beneficial for the start-up of SSAD, because it was key to reactor performance that a quantity of microbes should be maintained (Wilson et al., Reference Wilson, Sharvelle and DeLong2016). It was found that failed SSAD could be recovered by adding inoculum (Zhu et al., Reference Zhu, Wan and Li2010; Yang et al., Reference Yang, Ge and Li2016).
The results showed that the highest peak of biogas production was lower (Fig. 2a, b) than that of 3.8 L day−1, and 20.3 N mL−1 g−1-VS from AcoD of switchgrass and swine manure at 55°C (Ahn et al., Reference Ahn, Smith, Kondrad and White2010), and LAD of switchgrass at 35°C (Niu et al., Reference Niu, Kong, Li, Sun, Yuan and Zhou2015), respectively. The lower biogas production could be largely due to the high C/N ratio of 90 for switchgrass in the current study (Table 2) in addition to its complexity of the biomass structure compared with the optimal C/N ratio of 29.6 for the highest biogas production from rice straw in SSAD (Yan et al., Reference Yan, Song, Li, Yuan, Liu and Zheng2015). On the one hand, the C/N ratio was a principal parameter for biogas production potential of biomass in AcoD, and the reported optimal range of C/N is between 16/1 and 30/1 (Estevez et al., Reference Estevez, Linjordet and Morken2012). The facts of the high C content in switchgrass and low N concentration of the digestate resulted in a high C/N ratio of 90, which means inadequate nitrogen for microbial digestion. Biogas reduction, instability and failure of AcoD process can be originated from higher or lower C/N ratio beyond the optimum range of substrates (Hagos et al., Reference Hagos, Zong, Li, Liu and Lu2017). On the other hand, the inoculum size is a critical factor for SSAD performance (Liew et al., Reference Liew, Shi and Li2011), and lignocellulosic biomass degradation (Divya et al., Reference Divya, Gopinath and Merlin-Christy2015). Previous research indicated that the cumulative biogas production was elevated from 85.4 L kg−1-VS at the inoculums/corn stover ratio of 5:1 (w/w) to 182.0 L kg−1-VS at that of 10:1 for SSAD (Zhang et al., Reference Zhang, Mao, Zhai, Wang and Yang2015). It was indicated that there was an inverse correlation between the F/E ratio with CH4 yield in SSAD (Xu et al., Reference Xu, Wang and Li2014). CH4 production decreased with increasing substrates/inoculums ratio of SSAD (Liew et al., Reference Liew, Shi and Li2012). The small inoculum size of 3/1 (inoculum to switchgrass, w/w) provided less microbes, nutrients and buffering capacity to SSAD, thus relatively low biogas yields were obtained in the current study. The poor biogas production can also be attributed to high TS at 24%. It was revealed that TS had a main effect on CH4 production during growth phase of SSAD, the TS interacted with organic solid particle size, and the increase of TS decreased CH4 production at the same particle size (Motte et al., Reference Motte, Escudie, Bernet, Delgenes, Steyer and Dumas2013). Similar results showed that higher VS might influence the alkalinity of reactor; therefore the CH4 production was lower at higher TS of rice straw (Darwin et al., Reference Darwin, Cheng, Liu, Gontupil and Kwon2014). In addition, relatively large lignocellulosic biomass particle size of 3 cm in current research may also lead to low biogas production. It was reported that biogas production was improved by reducing particles size as the specific surface of substrate in digestion was increased (Mata-Alvarez et al., Reference Mata-Alvarez, Macé and Llabrés2000). Conversion rate is also affected by the lignin content of biomass in SSAD (Li et al., Reference Li, Park and Zhu2011). The lignin content was 22.8% for switchgrass by dry weight in the current study (Table 2).

Fig. 2. Biogas production in solid state AcoD of switchgrass and liquid digestate: (a) daily biogas output from set-up, (b) cumulative biogas yield after start-up and (c) the content of methane after start-up.
Figure 2 shows the daily biogas production, cumulative biogas production and CH4 percentage in two reactors. These results were consistent with previous result that the start-up and performance of SSAD depends on inoculation largely (Shi et al., Reference Shi, Xu, Wang, Stiverson, Yu and Li2014). The bioreactor started up from the second day after feeding, and the biogas produced quickly in the first 2 days, and reached the climax of 2.7 L kg−1-VS. The sharp increase in biogas production on days 1 and 2 was related to favorable digestion of preferably biodegradable carbohydrates (Ahn et al., Reference Ahn, Smith, Kondrad and White2010). However, biogas production may be limited by slow mass transfer for top-addition of inoculums (Yang et al., Reference Yang, Ge and Li2016). The biogas production dropped from 2.7 L kg−1-VS on day 3 to zero on day 7 (Fig. 2a), with a cumulative biogas production of 5.3 L kg−1-VS (Fig. 2b). The reactor was fed with 1 L LAD digestate on day 15, and the biogas was produced on day 16 and attained the second peak of 1.2 L kg−1-VS on day 22, but dropped to the lowest biogas yield of 0.3 L kg−1-VS on day 35. The second peak could be attributed to the easily hydrolyzed and soluble fraction of materials (Motte et al., Reference Motte, Escudie, Bernet, Delgenes, Steyer and Dumas2013). Then the biogas production was increased constantly and reached the maximum of 2.5 L kg−1-VS on day 40 at the third peak, and dropped gradually thereafter.
Different from reactor A, bioreactor B failed to start-up during the first week of loading (Fig. 2a). The possible reason for the difference of biogas production pattern between reactors A and B is the different distribution of inoculums in switchgrass for two high-solid systems. The liquid digestate may be more homogenized within switchgrass in reactor A than that in reactor B, as co-digestion is limited by unevenly distributed biomass (Hagos et al., Reference Hagos, Zong, Li, Liu and Lu2017). Uneven distribution of the digestate in bioreactor B may result from uneven compaction of switchgrass loaded by hand in this research during set-up. Acids accumulated in the hydrolysis could likely inhibit the methanogenesis process, which subsequently inhibited the biogas production and the low pH of 6.0 was measured in leachate on day 35 (Fig. 3). The optimal pH was found to range from ~6.5 to 8.2 for a successful AD process (Speece, Reference Speece1996).

Fig. 3. pH value of leachate in two solid state anaerobic reactors from set-up.
Acidification is likely to appear during thermophilic AD (TAD) (Mao et al., Reference Mao, Feng, Wang and Ren2015), and it is the most probable trigger of TAD failure (Yang et al., Reference Yang, Xu, Ge and Li2015). Acetic and butyric acids could inhibit the methanogenesis. The organic acids show acute toxicity toward the anaerobic microbes by adsorption onto the cell wall/membrane interfering with the transporter protective function (Rinzema et al., Reference Rinzema, Boone, van Knippenberg and Lettinga1994). The pH was 5.1 (Fig. 3), and acetic acid and butyric acid contents was detected (Table 3) on day 7 in reactor B, and the bioreactor often became acidified during SSAD of lignocellulosic biomass (Shi et al., Reference Shi, Xu, Wang, Stiverson, Yu and Li2014), therefore it was speculated that the ‘sour’ contributed to the failure of reactor B start-up. Reactor B was restarted on day 7 by adding 1 L of LAD digestate, the biogas production reached the peak level of 2.0 L kg−1-VS on the second day after LAD digestate was added, and ceased subsequently on day 17, producing about 10 L biogas in 10 days (Fig. 2a). The results indicated that the reactor was acidified again, as the pH value dropped (Fig. 3), and the concentrations of acetic acid and butyric acid increased (Table 3) from day 14 to day 35 significantly (P < 0.05). It is consistent with a previous report that the biogas yield was stopped from day 9 due to acidification in SSAD (Lin and Li, Reference Lin and Li2017). Acidification is a necessary step in the AD. To avoid inhibition from the fatty acids, acclimated anaerobic microorganism population has to be established and stabilized in the digester to maintain a pH of 6–8.
Table 3. The concentration of acetic acid and butyric acid in the leachate of two bioreactors (mean ± s.d.).
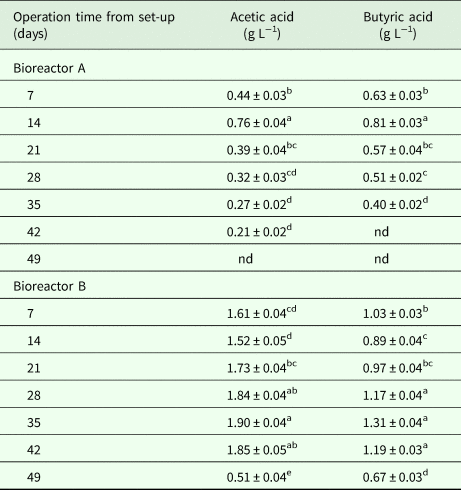
s.d., standard deviation (calculated from triplicate datasets of the different samples); nd, not detected.
Significant (P < 0.05) differences between operation times within each bioreactor are indicated by different letters.
In order to reduce the lag phase of SSAD in reactor B, 5 mL of 5 M sodium hydroxide (NaOH) was injected into the leachate once every 2 days from day 35 to 45. Although it took a long time to recover reactor B, the biogas production resumed on day 47, reached a peak level on day 48, then declined gradually and finally stopped on day 148. In contrast to bioreactor A, higher daily and cumulative biogas yield was observed for bioreactor B during the same normal operation time of 102 days, even though reactor B had a longer lag phase of biogas production. The NaOH, which was injected into leachate of reactor B before start-up, seems to be the reason why higher biogas yield was observed in reactor B. The supplemental NaOH not only prevented the pH from dropping, but also acted as a pretreatment reagent in lignocellulosic biomass during recirculation. Similar research reported that not only the total CH4 yield was increased but also failure risk can be mitigated by simultaneous NaOH treatment of leaves in SSAD (Liew et al., Reference Liew, Shi and Li2011). Alkaline pretreatment can decrystallize the cellulose and increase the accessible area of biomass to enzymes by swelling of fibers, therefore more biogas yield was obtained (Mao et al., Reference Mao, Feng, Wang and Ren2015; Patinvoh et al., Reference Patinvoh, Osadolor, Chandolias, Sárvári-Horváth and Taherzadeh2017).
By contrast, the percentage of CH4 was the lowest (data not shown) for bioreactor A (12.4%) on day 2 and for bioreactor B (3.9%) on day 8 from set-up, respectively. The biogas production was peaked at the time (Fig. 2a). CH4 contents of biogas increased gradually and stabilized at 50% for both reactors after start-up, indicating a normal operation of AD lasted for at least 100 days. It was observed that CH4 contents increased from 40.6 to 51.9% for reactor A, and increased from 37.5 to 51.9% for reactor B from day 7 to day 84. The CH4 contents reduced to 44.3 and 48.5% on day 98 from start-up time, respectively, and no more CH4 was produced after day 98 in both reactors (Fig. 2c). The slow increase of CH4 content was probably attributed to the slow infiltration of inoculums (Yang et al., Reference Yang, Ge and Li2016).
The pH values, acetic acid and butyric acid contents in the leachate from SSAD are shown in Figure 3 and Table 3, respectively. As a general result, changes of pH were consistent with the trend of biogas production, and the concentration of acetic acid and butyric acid, two types of VFA, for two bioreactors. The pH is a major factor for proper AD operation (Zhu et al., Reference Zhu, Wan and Li2010). Without enough buffer capacity, low pH would likely result in the bioreactor failure and low CH4 yield, because the drop in pH inhibited methanogenic archaea (Brown and Li, Reference Brown and Li2013). The high C/N ratio of 90 may result the VFA accumulation during start-up phase of two reactors. VFA, which was produced during fermentation stage of AD, can usually accumulate under unsuitable C/N ratio beyond the range of 20–30 (Li et al., Reference Li, Park and Zhu2011).
For bioreactor A, the pH of leachate is lower than 6 on days 7 and 14 after setup, when the reactor was failed to produce the biogas. The pH increased to 6.68 on day 42, when daily biogas production was increased to the peak value, and the pH decreased gradually and maintained around 6.2 thereafter (Fig. 3), when reactor A was demonstrated as a steady operation. The concentrations of acetic acid and butyric acid increased from day 7 to day 14 significantly (P < 0.05), then decreased to 0 on day 49. Butyric acid was not detected on days 42 and 49 (Table 3). It was likely that the acids were consumed to produce CH4 from day 14, since biogas continuously produced from this time and the maximum biogas production was obtained on day 40 (Fig. 2a).
For bioreactor B, the reactor failed to start-up during the first week, and the pH value, acetic acid and butyric acid contents in leachate was 5.1, 1.61 g L−1, 1.03 g L−1 on day 7, respectively (Fig. 3 and Table 3). The detected acids could explain the facts of low pH value, which in turn inhibited the AD process. A similar study indicated that the VFA accumulation resulted in low pH (5.0–5.5) and irreversible acidification in dry AcoD of switchgrass and animal manure (Ahn et al., Reference Ahn, Smith, Kondrad and White2010). Environmental pH is the most sensitive factor to AD under various physical and chemical conditions (Dhar et al., Reference Dhar, Kumar, Kumar, Mukherjee and Vaidya2016). Consistent with the biogas production pattern (Fig. 1a), the pH value increased from day 7 to day 14, then decreased gradually and reached lower than 5 on day 35. The pH variation tendency is also in agreement with the change of the acid contents in the reactor. The acetic acid and butyric acid concentrations in leachate of bioreactor B lowered significantly (P < 0.05) from day 7 to day 14, and increased significantly (P < 0.05) from day 14 to day 35 (Table 3). VFA can be produced from carbohydrate-rich feedstock, and excessive VFA could decrease pH value, consequently inhibits methanogen in SSAD (Yang et al., Reference Yang, Xu, Ge and Li2015). By injecting 5 mL NaOH (5 M) into the leachate one time every 2 days from day 35 to 45, the contents of acetic acid and butyric acid were decreased to 1.85 and 1.19 g L−1 on day 42, in contrast to that of 1.90 and 1.31 g L−1 on day 35, respectively. The pH was increased to 6.4 and the concentrations of acetic and butyric acids were decreased to the lowest level on day 49 after reactor B restarted on day 47. Accordingly, pH was increased and remained at favorable digestion condition of 6.5–7.0, and biogas production was increased continuously. Unfavorable conditions (pH < 6.5) were observed, and acetic acid and butyric acid were detected in both reactors before start-up.
Degradation of feedstock and the characteristics of digested residues and final leachate
The TS, VS, cellulose, hemicelluloses and lignin contents in bioreactor A reduced by 15.6, 16.8, 18.4, 8 and 0.5%, respectively (Table 4). For bioreactor B, higher degradation rates of 29.5, 31.6, 40.6, 15 and 1.2% were observed in those parameters, respectively (Table 4). This was correlated with the result that the cumulative biogas yield of bioreactor B was 46.2% higher than that of bioreactor A (Fig. 2b). The addition of NaOH likely improves the conversion efficiency of lignocellulosic biomass in bioreactor B. The TS and VS reduction were elevated by 1–5% through NaOH pretreatment of corn stover in SSAD, and the highest cellulose and hemicelluloses degradation were obtained at 5% NaOH loading (Zhu et al., Reference Zhu, Wan and Li2010). The more cellulose and hemicelluloses were degraded, the more CH4 yields were obtained during SSAD (Liew et al., Reference Liew, Shi and Li2011).
Table 4. The characteristics (mean ± s.d.), and reduction efficiency of digested residues in two bioreactors, and the requirements of China organic fertilizer standard (2012).

s.d., standard deviation (calculated from triplicate values of the same determined sample); TKN, total kjeldahl nitrogen; TOC, total organic carbon; OM, organic matter; C/N, carbon to nitrogen; Cu, copper; Zn, zinc; Cd, cadmium; Pb, lead; Cr, chromium; TS, total solids; VS, volatile solids.
a China organic fertilizer standard (2012).
Except for the moisture and TN contents, the determined characteristics of digested residues (Table 4) met the requirements of China organic fertilizer standard (NY 525-2012) (Ministry of Agriculture of China, 2012), suggesting that the digested residue could be used as an organic fertilizer for soil OM improvement. Compared with the requirements, the contents of OM was more than 45%, the concentrations of heavy metals such as Cd, Pb and Cr are much less than 3, 50 and 150 mg kg−1, respectively, and the pH values are in the range of 5.5–8.5. It is speculated that heavy metals are less enriched in agricultural soils through the use of the organic fertilizers of digested residues in the present research. The digested residues are environmentally friendly, easier to handle and more water can be applied to land and crops along with fertilizer application for soil amendment, though the water contents are higher than the requirement of 30%.
The contents of TKN, COD, TS, VS, TC, TOC, Cu, Zn, Cd, Pb, Cr, C/N ratio and pH value of the final leachate are shown in Table 5. It was observed that TKN (41.5, 21 mg L−1) and COD (692, 442 mg L−1) contents of final leachate in bioreactors A and B were much lower than that of liquid digestate (Table 2) of 346.5 and 6654 mg L−1, respectively. In addition, TKN and COD in the final leachate of bioreactor B are lower than those in bioreactor A, which illustrated that the biomass conversion efficiency was higher in bioreactor B. The concentrations of Cu, Zn, Cd, Pb and Cr are lower than 0.5 mg L−1 in the final leachate in both reactors. Cu and Zn contents in the final leachate are much lower than that in the initial digestate (Table 2), and Cd, Pb and Cr concentrations are <0.2 mg L−1 in the final leachate in both reactors. TS and TC contents in the final leachate are similar in both reactors A and B. The C/N ratios of final leachate in reactors A and B were about 2–3 times of that in the initial digestate. The pH value of ~6 was observed in the final leachate.
Table 5. The characteristics of the final leachate in two bioreactors (mean ± s.d.).

s.d., standard deviation (calculated from triplicate values of the same determined sample); TKN, total kjeldahl nitrogen; COD, chemical oxygen demand; TS, total solids; VS, volatile solids; TC, total carbon; TOC, total organic carbon; C/N, carbon to nitrogen; Cu, copper; Zn, zinc; Cd, cadmium; Pb, lead; Cr, chromium.
The carbon balance of two bioreactors
The carbon (C) balance of switchgrass and liquid digestate co-digestion in SSAD is shown in Figure 4. For both bioreactors A and B, there is a very small difference (0.2–0.5%) between C input and output. Compared with bioreactor A, the C content of digested residues in bioreactor B was less, but C originated from CH4 and CO2 was more for bioreactor B. This is consistent with the cumulative biogas production differences in two bioreactors. Approximately 90% of C was presented in the final digested switchgrass, which may be due to the high C/N ratio (90) and high TS (24%) of the substrates in two reactors. The optimum C/N ratio needs to be obtained by supplementing N sources such as solid swine feces to reuse liquid digestate in SSAD of agricultural biomass in future perspective.

Fig. 4. The carbon balance in two solid state anaerobic reactors.
Conclusions and implications
Though a long start-up time and relatively low biogas production were observed, it is feasible to reuse primary liquid digestate as inoculums for co-digestion with agricultural residues in SSAD for biogas and fertilizer production. Therefore, it is promising that the finding could be applied in two-stage (primary and secondary) TAD for the management of animal and crop wastes on farms. However, energy cost will probably be a major limiting factor for two-stage TAD in cold weather. The biogas produced from the two-stage TAD, which can be used to supply heat to the primary LAD and secondary TAD, can balance the energy consumption in turn.
Higher biogas production and biomass consumption in reactor B were observed, which is likely attributed to the addition of NaOH into the recycling leachate for pH optimization and simultaneous cellulose biomass pretreatment before start-up. This implied that controlling pH is critical to normal operation in full scale systems, and adjusting pH of leachate of recirculation is feasible in practice. The properties of digested residues met the China organic fertilizer standard (NY 525-2012) with the exception of high moisture and low TN. Compared with initial digestate, the contents of TKN, COD and heavy metals in the final leachate are rather low, which is beneficial to liquid digestate reuse. Further investigation on proper designs for stable and optimal operation (e.g., C/N ratio, biomass pretreatment, substrate loading approach and pH management) of the solid state AcoD system for agricultural and animal waste reuse is necessary for practical and economical application.
Author ORCIDs
Jianjun Zang, 0000-0002-1613-6119
Acknowledgements
This work was supported by the National Key Research and Development Program of China (grant number 2016YFD0500506); the DaBeiNong Group (Beijing, China) program (grant number C2016029) and US Department of Agriculture through NC Agricultural Research Service (Project No. 02390). This work was collaborated and conducted in Dr Jay J. Cheng's laboratory at North Carolina State University, Raleigh, NC, USA. The authors are grateful to Dr Cong Tu and Dr Quan Zhou for sample analysis, greatly indebted to Dr Matthew Campbell for proof reading the manuscript, and we would like to acknowledge the United States Department of Agriculture-National Institute of Food and Agriculture and Holistic Farming, Inc. for their support of the study, thank Miss Kaylie Loyd, Mrs. Yumei Fu and Dr Xiuguo Zou for their contributions to the research.