Skeletal muscle, one of the most dynamic and plastic tissues in the human body, accounts for approximately 40 % of the total body weight in humans and is fundamental to movement, energy homoeostasis and overall quality of life(Reference Frontera and Ochala1–Reference Bear, Parry and Puthucheary3). However, skeletal muscle mass begins to decline in middle-aged and older adults, and adults between the ages of 40 and 80 years have already lost approximately 20 % of their skeletal muscle mass during their lifetime(Reference Marzetti, Hwang and Tosato4,Reference Alcazar, Aagaard and Haddock5) . Muscle mass decline makes middle-aged and older adults vulnerable to bone fractures and chronic metabolic diseases, such as type 2 diabetes and obesity, leading to a significant increase in healthcare costs(Reference Al-Ozairi, Alsaeed and Alroudhan6,Reference Kaji7) . Apart from that, muscle loss has even been reported as an independent risk factor for high mortality in older individuals(Reference Metter, Talbot and Schrager8,Reference Rantanen, Harris and Leveille9) . However, effective and strategic muscle-sparing intervention methods for older adults have not yet been revealed.
In recent years, researchers have found that the level of oxidative stress in skeletal muscle increases with age, and the imbalance between increased reactive oxygen species production and overall antioxidant defence is one of the leading causes of muscle damage(Reference Boengler, Kosiol and Mayr10,Reference Leitner, Wilson and Yan11) . At the same time, a series of studies have shown that dietary intake of antioxidant vitamins is associated with lower reactive oxygen species and better-preserved muscle mass(Reference Higgins, Izadi and Kaviani12–Reference Cruz-Jentoft, Dawson Hughes and Scott14). Additionally, exogenous supplementation of appropriate amounts of vitamins can protect against muscle loss during ageing(Reference Dekkers, van Doornen and Kemper15,Reference Nilsson, Mikhail and Lan16) . Total antioxidant capacity (TAC) is a term that reflects the antioxidant potential of dietary sources, which are mainly a combination of various vitamins(Reference Gupta, Finelli and Agarwal17–Reference Mazdak, Tolou Ghamari and Gholampour21). Researchers believe that TAC participates in the progression of several diseases, such as hypertension and cancer(Reference Fateh, Mirzaei and Gubari22,Reference Han, Chung and Park23) . However, the relationship between TAC and muscle loss has been scarcely studied. In patients with liver cirrhosis, researchers found that TAC was positively correlated with grip strength and arm muscle area(Reference de Freitas Lima, de Faria Ghetti and Hermsdorff24). Other animal experiments have confirmed that antioxidant supplementation can improve skeletal muscle quality(Reference Wen, Chen and Huang25,Reference Qin, Zhang and Cao26) . Given the higher risk of muscle mass loss in the middle-aged population than in the younger population, studies targeting TAC and muscle loss in this population are urgent and valuable.
Based on the National Health and Nutrition Screening Survey (NHANES) database, the purpose of this study was to investigate the association between dietary TAC of antioxidant vitamins and skeletal muscle mass in middle-aged individuals in the USA after adjusting for potential risk factors.
Methods
Study population
NHANES is a representative US population survey that uses complex multilevel probability sampling to provide information on the nutritional status and health status of the general US population. The NHANES research programmes were approved by the NCHS Research Ethics Review Committee and received written informed consent from the participants.
This study uses the US NHANES database for the rolling period 2011–2018 (n 39 156). After excluding patients with missing information on demographics, diet, examination and questionnaires, a total of 4009 subjects were included in the analysis. Figure 1 shows an example of a selection flow chart.
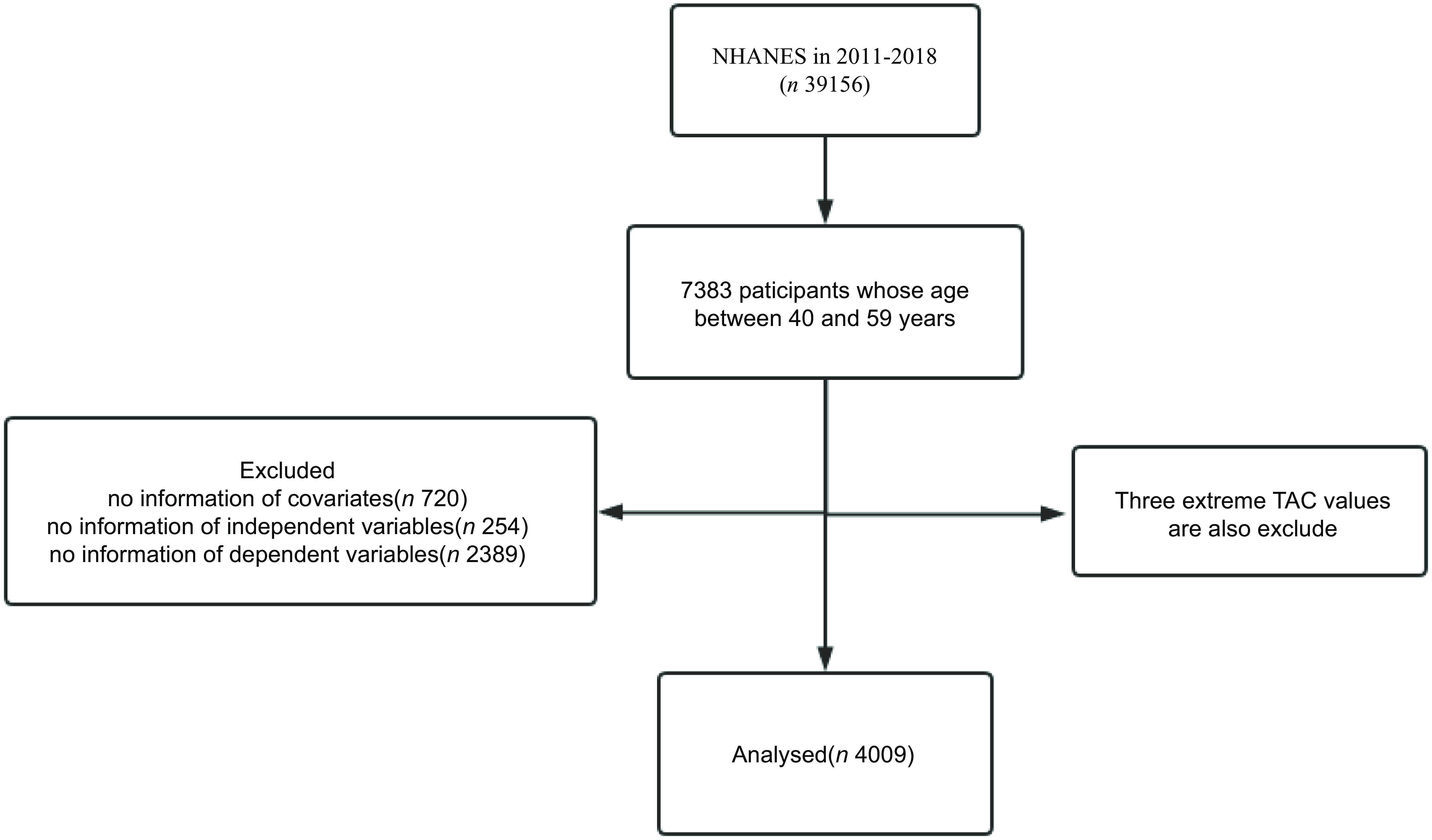
Fig. 1. Flow chart for participant inclusion and exclusion. NHANES, National Health and Nutrition Screening Survey; TAC, total antioxidant capacity.
Estimation of total antioxidant capacity from diet
On the first day of the interview, participants were asked to report in detail all food and beverages consumed in the past 24 h. Subsequently, after 3–7 d, the researcher collected dietary intake for the past 24 h again by telephone. The researchers then converted this information into nutrient intakes based on the USDA’s Food and Nutritional Database (FNDDS). The antioxidant vitamins recorded in the NHANES dietary interview consisted of vitamin A, vitamin C, vitamin E, α-carotene, β-carotene, β-cryptoxanthin, lycopene and lutein-zeaxanthin. According to Floegel et al.(Reference Floegel, Kim and Chung27), the individual antioxidant capacity of participants was determined by multiplying the individual amount of antioxidant compounds (antioxidant vitamins) by their antioxidant capacities:
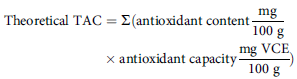
Antioxidant capacity was measured in the laboratory by chemical combustion, and the antioxidant capacity of vitamin C was used as a benchmark to assess the antioxidant capacity of other vitamins. In our study, we averaged the antioxidant nutrient intakes from the two surveys. TAC was divided into Q1 (0·236 to 22·188 mg VCE/100 g), Q2 (22·188 to 53·255 mg VCE/100 g), Q3 (53·255 to 112·933 mg VCE/100 g) and Q4 (112·933 to 779·247 mg VCE/100 g) according to the survey-weighted quartile.
Covariates
The demographic factors included age, sex (Men and Women), race (Mexican American, Other Hispanic, Non-Hispanic White, Non-Hispanic Black and Other Race) and socio-economic status (Low, PIR < 1·3; Middle, 1·3 ≤ PIR ≤ 3·5; High, PIR > 3·5). Lifestyle factors consisted of alcohol consumption (yes and no), smoking status (never, former and now), physical activity (none, moderate and heavy) and sedentary activity (online Supplementary Table S1). Other factors reported in the study that may influence body mass were obtained from the interview diet data and included protein, dietary fibre, Ca and phosphorus intake(Reference van Dronkelaar, van Velzen and Abdelrazek28).
Dependent variables
There are six dependent variables in this study, including appendicular relative lean mass (relative to body weight, g/kg), trunk relative lean mass (relative to body weight, g/kg), total relative lean mass (relative to body weight, g/kg), total percent fat (percent of body weight, %), trunk percent fat (percent of body weight, %) and BMI (kg/m2).
Through dual-energy X-ray absorptiometry, the lean body mass (excluding bone mineral content) and fat content of participants’ left and right legs, left and right arms, and trunk were measured separately. The appendicular relative lean mass is calculated by summing the lean body mass (excluding bone mineral content) of the left and right legs and arms. In addition, to account for the effects of body weight on these results, all dependent variables are relative to body weight (all lean body mass is per kilogram of body weight g; all fat is per kilogram of body weight kg).
Statistical analysis
The statistical analysis was conducted by using the statistical computing and graphics software R (version 4.2.1) and EmpowerStats (version 5.0). Continuous variables were compared for between-group differences using t tests or one-way ANOVA, expressed as mean (standard error) (se), and categorical variables were compared for between-group differences using non-parametric tests, as well as expressed as frequencies (percentages). After satisfying the linear regression assumptions, we determined the β and 95 % CI by analysing a multivariate linear regression between the TAC and all outcomes. The multivariate linear regression was built using three models: Model 1: not adjusted; Model 2: adjusted for sex, age, race and socio-economic status; Model 3: adjusted for all covariates. In addition, considering the non-normality of the TAC distribution, we again performed multivariate linear regression analyses by log-transforming the TAC. Smoothed curve fits were carried out concurrently with the variable adjustments. We used a threshold effects analysis model to examine the relationship and saturation effect between TAC and body mass. Finally, subgroup analysis was used to determine the population who experienced the most benefit. We used dietary day one sample weight to analyse all the results, and P < 0·05 was considered statistically significant.
Results
Descriptions of participants
The characteristics of weighted demographics, dietary data and lifestyle of the participants are shown in Table 1. A total of 4009 participants were included in this study. Of these participants, the average age was 49·69, and 50·18 % were man. Among different groups of TAC (quartiles, Q1–Q4), age, sex, race, socio-economic status, smoking, physical activity, appendicular relative lean mass, trunk relative lean mass, total relative lean mass, total percent fat, trunk percent fat, protein, dietary fibre, Ca and phosphorus were all significantly different (P < 0·05). The relationships between the dependent variables and the covariates can also be seen in online Supplementary Table S2.
Table 1. Baseline characteristics of the participants grouped by TAC quartiles
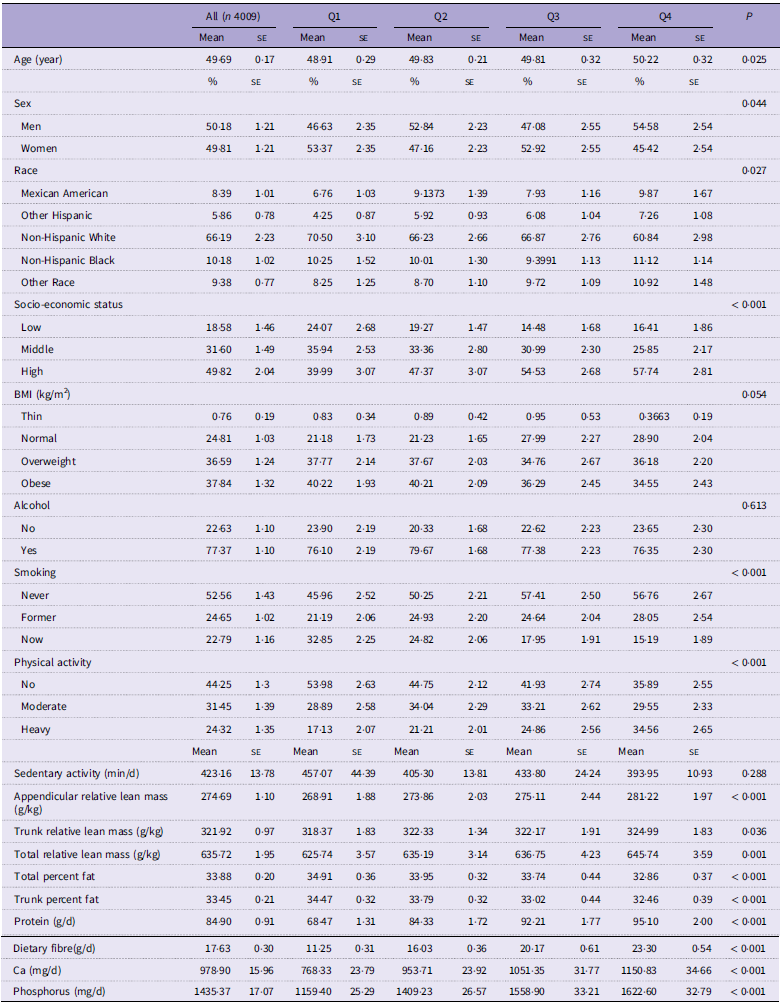
TAC, total antioxidant capacity.
Data are presented by % (se) for categorical variables or mean (se) for continuous variables.
Relationship between total antioxidant capacity and skeletal muscle mass
There was a significant positive association between dietary TAC and lean body mass in three weighted univariate and multivariate linear regression models (Table 2). In the fully adjusted model, each 1-unit increase in dietary TAC was associated with an increase of 0·018 g/kg appendicular lean mass (95 % CI 0·007, 0·029), 0·014 g/kg trunk lean mass (95 % CI 0·004, 0·024) and 0·035 g/kg total lean mass (95 % CI 0·014, 0·055).
Table 2. Multivariate linear regression analysis of TAC and lean mass
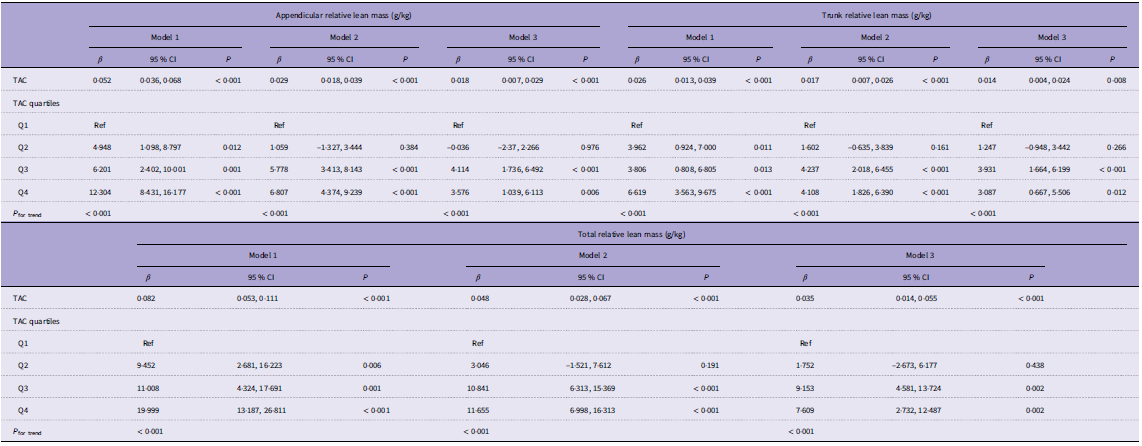
TAC, total antioxidant capacity.
Model 1: without adjustment.
Model 2: age, sex, race and socio-economic status were adjusted.
Model 3: Model 2 plus smoking, alcohol, physical activity, sedentary activity, protein, dietary fibre, Ca and phosphorus were adjusted.
β, 95 % CI, and P values are presented.
Dietary TAC also showed a significant negative association with total percent fat, trunk percent fat and BMI (Table 3). Assuming linearity, each 1-unit increase in dietary TAC was associated with −0·004 kg/kg total percent fat (95 % CI −0·006, −0·002), −0·005 kg/kg trunk percent fat (95 % CI −0·007, −0·002) and −0·003 kg/m2 BMI (95 % CI −0·006, −0·001). Furthermore, after log-transforming TAC, a significant association between TAC and skeletal muscle mass was still found (online Supplementary Table S3).
Table 3. Multivariate linear regression analysis of TAC and fat/BMI
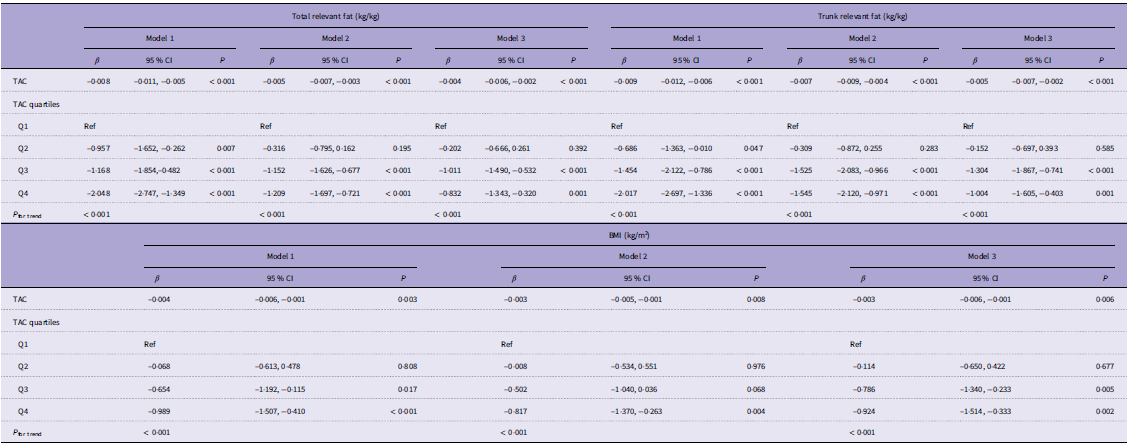
TAC, total antioxidant capacity.
Model: without adjustment.
Model 2: age, sex, race and socio-economic status were adjusted.
Model 3: Model 2 plus smoking, alcohol, physical activity, sedentary activity, protein, dietary fibre, Ca and phosphorus were adjusted.
β, 95 % CI, and P values are presented.
Dose–response relationships and their saturation effect
Figure 2 shows the dose–response relationship between dietary intake and TAC for all outcomes. Combining the smoothing curve and TAC quartile, a saturation effect was found between TAC and all outcomes. Then, a saturation effect analysis explored these turning points, and the saturation effect value was 67·433 mg VCE/100 g in the appendicular relative lean mass, 64·072 mg VCE/100 g in the trunk relative lean mass, 64·809 mg VCE/100 g in the total relative lean mass, 67·433 mg VCE/100 g in the total percent fat, 65·955 mg VCE/100 g in the trunk percent fat and 71·167 mg VCE/100 g in BMI (Table 4).
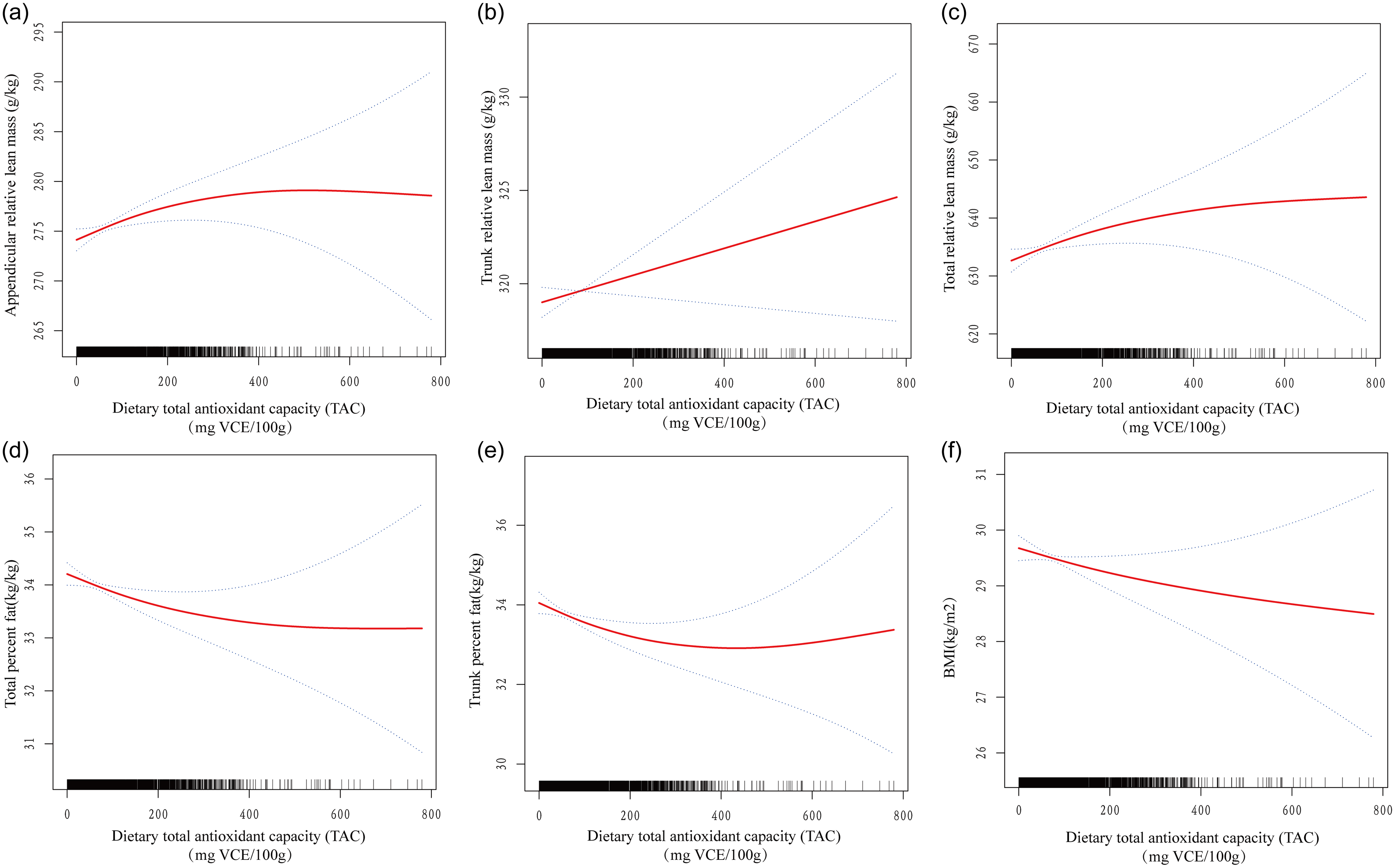
Fig. 2. Dose–response relationship between dietary TAC and skeletal muscle mass and body fat. (a) Appendicular relative lean mass (g/kg), (b) trunk relative lean mass (g/kg), (c) total relative lean mass (g/kg), (d) total percent fat (kg/kg), (e) trunk percent fat (kg/kg) and (f) BMI (kg/m2). TAC, total antioxidant capacity.
Table 4. Saturation effect analysis of TAC on all outcomes
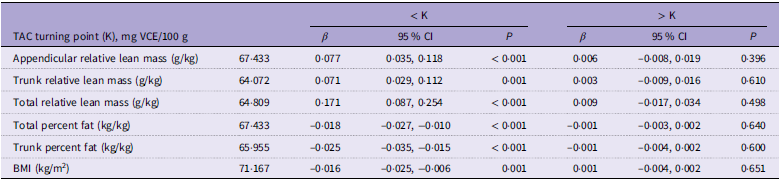
TAC, total antioxidant capacity.
Age, sex, race, socio-economic status, smoking, alcohol, physical activity, sedentary activity, protein, dietary fibre, Ca and phosphorus were adjusted.
β, 95 % CI, and P values are presented.
Subgroup analysis of the association between dietary total antioxidant capacity and skeletal muscle mass
Our study population contained participants aged 40–59 years with a mix of both men and women participants, so we also explored how age and sex influenced the aforementioned associations (Table 5, and online Supplementary Fig. S1–S2). When stratifying by age, the associations were significant in patients aged 40–50 years rather than in those aged 50–59 years. In the subgroup analysis of sex, women participants had significant associations between dietary TAC and skeletal muscle mass. Therefore, women younger than 50 years may experience the best benefits from dietary TAC.
Table 5. Association of dietary TAC with all outcomes stratified by age and sex

TAC, total antioxidant capacity.
Race, socio-economic status, smoking, alcohol, physical activity, sedentary activity, protein, dietary fibre, Ca and phosphorus were adjusted.
β, 95 % CI, and P values are presented.
Discussion
The present analysis was conducted to determine the relationship between dietary TAC intake and body mass components in adults over 40 years old. The US population data were extracted from the NHANES database. The results showed that for adults who had an increased risk of skeletal muscle mass loss, higher dietary TAC is related to greater preservation of appendicular lean mass, trunk lean mass and total lean mass. Also, higher dietary TAC intake is associated with lower total percent fat, trunk percent fat and BMI.
To the best of our knowledge, the association between dietary TAC and skeletal muscle mass has not yet been investigated in a cohort with this size and scope(Reference Ratajczak, Skrypnik and Bogdański29,Reference Lim, Fan and Say30) . Consistent with a previous cross-sectional study in cirrhotic outpatients, dietary TAC was positively associated with arm muscle area(Reference Al-Ozairi, Alsaeed and Alroudhan6). In a three-year-long cohort study, higher dietary antioxidant intake had positive effects on BMI and abdominal fat(Reference Boengler, Kosiol and Mayr10). Another study of children and adolescents showed that dietary antioxidant intake had an inverse association with total body fat in obese subjects(Reference Leitner, Wilson and Yan11). Above all, dietary TAC intake has an inspiring effect on lean body mass, fat and BMI(Reference Besagil, Çalapkorur and Şahin31,Reference Amani, Parohan and Jomehzadeh32) .
Although some studies have been deployed to detect the association between antioxidant intake and body components in particular populations, including children and adolescents, women, and healthy young adults, they not only primarily focused on the effects of single antioxidant intake, which might not fully explain the synergistic effects of all antioxidant vitamins in the diet(Reference Higgins, Izadi and Kaviani12), but also provide less knowledge of the middle-aged population who suffer a higher risk of skeletal muscle mass loss(Reference Bloom, Shand and Cooper33). In this study, we paid attention to the comprehensive TAC values rather than considering the effects of single compounds, and we focused on the people who may experience greater benefits from the above results.
Although the underlying mechanisms between TAC and body composition were not elaborated in our study, by reviewing previously reported studies, we hypothesised that oxidative stress plays an integral role. Oxidative stress levels in skeletal muscle increase with age, which may lead to impaired muscle protein synthesis and muscle fibre damage(Reference Luo, Mills and le Cessie34,Reference Yoshiko, Shiozawa and Niwa35) . Whereas, increased dietary TAC may protect muscles from damage by neutralising free radicals and reducing oxidative stress. In addition, antioxidants have anti-inflammatory effects and can reduce inflammatory responses in muscle tissue(Reference Kobayashi, Murakami and Sasaki36). Inflammation is a known contributor to muscle atrophy; therefore, by reducing inflammation, a high TAC diet may help maintain muscle mass(Reference Sumi, Ashida and Nakazato37).
Dose–response curves suggest that all outcomes displayed a close correlation with dietary TAC. However, there also displayed a saturation effect of correlation between dietary TAC and skeletal muscle mass. All these results indicated that higher dietary TAC would likely improve lean body mass and decrease body fat and BMI. The saturation effect revealed that there was a threshold effect between dietary TAC and all outcomes. A subsequent subgroup analysis indicated that women and individuals aged 40–50 years will experience maximum benefits from higher dietary TAC on skeletal muscle mass. Our findings not only provide possible nutritional interventions for slowing or preventing the decline of muscle mass and function in middle-aged and older adults but also provide detailed recommendations for dietary intake in relation to the challenges of ageing regarding muscle loss and fat gain.
However, there are still some limitations in our study. First, this study was a cross-sectional design, which means that the causal relationship between dietary TAC and skeletal muscle mass could not be clearly determined owing to its original survey. Second, vitamin supplementation, such as vitamin C supplementation, is not taken into consideration while only focusing on dietary TAC intake in this design(Reference Mansournia, Ostadmohammadi and Doosti-Irani38). Finally, the bioavailability of dietary vitamins in participants was not included in this study because of the defect value in the NHANES dataset(Reference Platel and Srinivasan39). Furthermore, more work should be done to investigate the relationship between serum TAC levels and skeletal muscle mass both clinically and experimentally in the future to figure out their causal effect and potential mechanism.
In summary, our results found not only a simple linear positive association between TAC and lean body mass and a negative association between body fat but also a saturation threshold. This result is encouraging for enhancing health management of muscle loss and fat gain in middle-aged populations.
Supplementary material
For supplementary material/s referred to in this article, please visit https://doi.org/10.1017/S0007114524002575
Acknowledgements
The authors gratefully acknowledge the contributions of all staffs who work on the National Health and Nutrition Examination Surveys.
None.
Conceptualisation: L. W. and S. C.; Methodology: J. Z. and W. F.; Software: J. Z. and W. F.; Formal Analysis: J. Z. and W. F.; Writing – Original Draft Preparation: J. Z. and W. F.; Writing – Review and Editing: L. W., J. Z. and W. F.; Supervision: L. W. All authors have read and approved the final manuscript.
The authors declare no conflicts of interest.