Introduction
There are more than 200 000 flowering plant species, and at least 500 000 insect species interact with them (Metcalf and Kogan Reference Metcalf and Kogan1987). Over their 400-million-year coexistence, insects and plants have developed extremely diverse and complex interactions (Samways Reference Samways1993). More specifically, they have developed nutrition, reproduction, protection, and defence relationships (Qi et al. Reference Qi, Duan, Ren and Wu2020).
A gall is any enlargement of plant cells, tissues, or organs that was induced by the stimulus of a parasitic organism as a regular incident in the life history of the parasite (Cosens Reference Cosens1913). Galls can develop on any plant tissue, but the majority occur on leaves (Dreger-Jauffret and Shorthouse Reference Dreger-Jauffret, Shorthouse, Shorthouse and Rohfritsch1992). Although plant galls can be formed by bacteria, fungi, mites, nematodes, parasitic plants, viruses, and insects, gall induction is mainly perpetrated by insects. Worldwide, the number of gall-producing insect species is estimated to be between 21 000 and 211 000 species, many of which are yet to be described (William Reference William2011).
Caragana liouana Zhao Y. Chang and Yakovlev, a leguminous shrub, is distributed in Ningxia, Inner Mongolia, Shanxi, and Shaanxi in China. It occurs naturally in semifixed and fixed sandy areas and loess hills in grasslands and desert grasslands, and it forms building blocks on fixed sand dunes or flat sandy areas. In this way, it plays an important role in revegetating fragile ecosystems in the arid and semiarid regions of China (Luo et al. Reference Luo, Liu and Li2007). In recent years and with widespread planting of C. liouana, gall tumour damage to the branches and trunks of plants in the middle of the C. liouana desert forest has broken out, and the damage is spreading (Song et al. Reference Song, Liu and Mo2010).
Trigonorhinus sp. is a newly discovered stem-boring pest that we discovered through indoor dissection of galls on the main and lateral branch stems of C. liouana (Fig. 1). The pest was first discovered in Wuqi County, Shaanxi Province, China (Song et al. Reference Song, Liu and Mo2010) but has neither been scientifically named nor been recorded in Inner Mongolia, China. We have no information about the pest’s damage characteristics, growth, and development. It was identified by Russian weevil expert Boris A. Korotyaev, who identified the insect as Coleoptera, family Anthribidae, genus Trigonorhinus. Further identification work is in progress. A similar species, Trigonorhinus dolgovi, was described by Korotyaev (Reference Korotyaev1977). Before that, Trigonorhinus alternatus and Trigonorhinus tomentosus tomentosus were discovered and reported by Say in 1826, and Trigonorhinus areolatus was discovered by Boheman in 1845 (Hume et al. Reference Hume, Patrice, Robert, Pierre, Robert and Reginald2013), with its description published by Antonio et al. (Reference Antonio, José and Antonio2013).

Fig. 1. Typical hazards of Trigonorhinus sp.
Anthribid beetles are a rare and ancient group with peculiar habits. Larval habits vary, with some living in woody fungi (Legalov et al. Reference Legalov, Nazarenko and Perkovsky2018), others feeding on seeds (Legalov et al. Reference Legalov, Nazarenko and Perkovsky2018) or the eggs of their hosts (Dervišević et al. Reference Dervišević, Stojanović, Simonović and Graora2019), and some preying on the eggs of scale insects (Dervisevic and Graora Reference Dervisevic and Graora2019). Although a few records report Anthribids causing and inhabiting plant galls, there are no descriptions of how the insects actually form the galls (Valentine Reference Valentine1998). For instance, the weevil Urodontus scholtzi Louw (Anthribidae) induces oval–cylindrical galls on the stems of host plant Galenia africana Linnaeus (Aizoaceae) (Price and Louw Reference Price and Louw1996), and Oberprieler and Scholtz (Reference Oberprieler and Scholtz2018) described the unusual gall-forming habit and life history of Urodontidius enigmaticus Louw (Anthribidae) larvae, which form galls on the leaves of Ruschia versicolor Linnaeus Bolus and then feed on the gelatinous cells inside the galls.
Trigonorhinus sp. females lay eggs in the central stem of C. liouana and, after hatching, the larvae feed on stem tissue, which then induces gall production. Unfortunately, the swelling and tuberculation of C. liouana branches caused by Trigonorhinus sp. result in severely decreased fruit set, and multiple gall tumours lead to conductive tissue damage and death, thereby posing a serious threat to plant growth. Few studies have been undertaken to investigate the frequency and kind of impacts caused by galling insects on their host plants (Sacchi et al. Reference Sacchi, Price, Craig and Itami1988). Because of this, we investigated both the growth of infected C. liouana during the Trigonorhinus sp. gall-forming process and the relationship between gall size and Trigonorhinus sp. population density. Our aim was to examine these questions: (1) What are the specific growth dynamics of the galls? (2) What are the changes in C. liouana growth potential after it has been affected? and (3) What factors are associated with gall size?
Methods
Study site description
The study site, which covered an area of about 3000 m2 in the C. liouana Forest in Usutu National Forest Park (60° 26' 30" N, 111° 9' 20" E) in Hohhot, China, was located on a semifixed sandy area halfway up a mountain slope. The forest’s average plant height was 112.87 cm, average annual temperature was 10 °C, average annual rainfall was 770 mm, annual sunshine was 2077 hours, and sunshine rate was 46%.
Gall and plant growth
Thirty current-year galls were randomly marked, and their lengths and widths, as well as the width of each infested branch and the ground diameters and heights of the infested plants, were measured using Vernier calipers every 7 days from late March to early November 2019. The galls were obtained from 30 different host plants, and the host plants received different degrees of damage. In the same plot, five healthy plants were randomly selected as controls, and their heights and ground diameters were measured at 7-day intervals.
Relationships between galls and each insect development stage
In 2019, we used branch cutters to randomly collect 20–30 galls every 7 days and every 30 days from 2020 to 2021. We then measured the length and width of each gall and the width of the affected branch, and then we dissected the collected galls to determine the development stages of the insects. To facilitate identification, insect developmental stages were divided into eggs, larvae, prepupae, pupae, and adult. The dissected galls and the different stages of the Trigonorhinus sp. individuals found within the galls were observed and photographed using a DVM6 digital microscope (Leica Microsystems, Wetzlar, Germany). In this experiment, each emergence hole in a gall was considered an individual because only one adult will emerge from each hole.
Statistical analyses
Because the galls are approximately cylindrical, their volumes (V) and relative volumes (RV) were calculated as:

and

where GD represents gall width, GL represents gall length, and HD is the width of ungalled tissue (Qi et al. Reference Qi, Duan, Ren and Wu2020).
To test whether the gall volume formula provided a statistically reliable estimate of gall size, we randomly resampled 50 galls by immersing each gall in a graduated tube filled with water and recording the volume of displaced water. Because the relationship between the two measurements was linear (y = 1.0328x + 0.1047, R 2 = 1), we used the gall volume formula to estimate gall size throughout the study.
The differences among the effects of growth period and branch size on gall length, width, relative width, volume, and relative volume were tested using one-way analysis of variance, as were the effect of gall size on branch dieback and the effects of the number of larvae in the galls on gall length, width, volume, relative volume, and whether the branches died. Statistical analyses were performed using SPSS 25.0 (IBM, Chicago, Illinois, United States of America).
Results
The growth of insects, galls, and host plants
Trigonorhinus sp. life cycle
Trigonorhinus sp. produce one generation a year in Inner Mongolia. Each Trigonorhinus sp. larva is hidden in a single chamber, and there were 3–15 larvae per gall. While overwintering, the larvae feed in the gall and then stop feeding to pupate (Fig. 2C, D, H) in April. The grey–brown adults (Fig. 2E, I, J) emerge in early May and seek mating opportunities. After mating, females lay eggs in mid to late May on new branches of the year or on branches without galls (usually located in the middle or at the bottom of the branch; Fig. 2A, F). Their eggs hatch in late May, and the larvae (Fig. 2B, G) feed inside galls they form on the branches. In late July, adults mate for the second time and lay eggs on new branches of the year or on branches without galls. The larvae from those eggs feed, go dormant, and overwinter (August–March) inside galls on branches, and then the cycle begins again.

Fig. 2. Trigonorhinus sp. morphology: A and F, egg; B and G, larva; C and H, prepupa; D, pupa; and E, I, and J, adult.
Gall development dynamics
When galls begin to swell in March and April, the overwintered Trigonorhinus sp. larvae are now active and gnawing on branches. After the adult females lays eggs on new branches in mid to late May, the subsequent newly hatched larvae form new, tender, green, juicy, oval–cylindrical galls with much fibrous and watery internal tissue. In July, the galls are 1.8–5.5 times the diameter of a normal branch. Meanwhile, as galls develop and mature by larval feeding, they enlarge and their tissues become dry, woody, and brown-coloured. Gall stages include (1) winter dormancy (from August of year 1 to March of year 2), (2) maturity (from April to July in year 2), (3) winter dormancy (from August in year 2 to March of year 3), and (4) maturity (from April to July of year 3), followed by death (Fig. 3). Galls vary in size from 5.2 to 205.4 mm (n = 876) long, 0.8 to 37.6 mm (n = 876) wide, and 0.003 to 106.5 cm3 (n = 876) in volume (Fig. 3).

Fig. 3. Dynamic diagram of Trigonorhinus sp. gall development: I, spawning; II, formation; III, initial expansion; IV, expansion; V, dormancy; VI, maturity; VII, dormancy; VIII, maturity; and IX, death.
The average monthly length, width, relative width, volume, and relative volume of galls during the growth period (April–July) differed significantly among the months (length: F (8,22) = 136.553, P = 0.000; width: F (8,22) = 129.171, P = 0.000; relative width: F (8,22) = 34.384, P = 0.000; volume: F (8,22) = 183.613, P = 0.000; relative volume: F (8,22) = 157.421, P = 0.000; Table 1), thus indicating that the galls increased in size as the plants did.
Table 1. Average gall measurements during the Trigonorhinus sp. gall growth period, April–July 2019.

† Relative gall width = width of galled tissues − width of ungalled tissue.
Caragana liouana growth
Beginning in April, the average monthly diameter at ground level of C. liouana with galls was considerably less than that of healthy C. liouana (Fig. 4). Although there was some error in the shrub height measurements, the average monthly growth rates of plants with galls were generally lower than those of healthy plants (Fig. 5), indicating that galls can inhibit C. liouana growth and development to some extent.

Fig. 4. Monthly growth rates, measured as diameter at ground level, of Caragana liouana Zhao Y. Chang and Yakovlev. Solid squares indicate healthy C. liouana, and solid circles indicate C. liouana with galls. Data are means; bars represent the standard error of the mean.

Fig. 5. Monthly growth rates, measured as shrub height, of Caragana liouana. Solid squares indicate healthy C. liouana, and solid circles indicate C. liouana with galls. Data are means; bars represent the standard error of the mean.
Relationships between the number of larvae and galls
Quantitative characteristics of Trigonorhinus sp. larvae in galls
Usually, we found 1–4 galls per C. liouana branch, and each gall contained an average 6.84 ± 3.227 Trigonorhinus sp. larvae, with a maximum of 18 and a minimum of 1 (Fig. 6). The usual number of Trigonorhinus sp. in a gall was 6–9, which accounted for 44.86% of all galls.

Fig. 6. Frequency distribution of the number of Trigonorhinus sp. larvae in individual Caragana liouana galls (n = 867).
Relationship between the number of Trigonorhinus sp. and gall size
The number of larvae within a gall had no significant effect on gall length, width, volume, or relative volume (n = 876; length: F (17,858) = 0.860, P = 0.622; width: F (17,858) = 0.921, P = 0.548; volume: F (17,858) = 0.512, P = 0.947; relative gall volume: F (17,858) = 0.532, P = 0.938). This indicates that the effects of gall formation are the same regardless of the number of larvae infesting a C. liouana shrub.
Relationships between galls and host plants
Number and volume of Trigonorhinus sp. galls in relation to C. liouana branch dieback
Of the 876 galls collected, 293 died, accounting for 33.45% of the total, and the branches on which the dead galls were located also died. Analysis of variance showed that, although the number of Trigonorhinus sp. within the galls did not significantly affect C. liouana branch dieback (F (1,874) = 0.428, P = 0.513), gall volume was significantly related to branch dieback (F (1,874) = 279.836, P = 0.000). Therefore, the number of Trigonorhinus sp. larvae does not cause branch dieback, but the size of the galls does: the larger the galls are, the more likely C. liouana branches will die.
Relationships between the branch on which galls were located and the gall
The diameter of the C. liouana branches on which galls were located differed significantly with respect to the length, width, relative width, volume, and relative volume of the galls (gall length: F (434,441) = 1.487, P = 0.000; width: F (434,441) = 2.461, P = 0.000; relative width: F (434,441) = 2.232, P = 0.000; volume: F (434,441) = 1.773, P = 0.000; relative volume: F (434,441) = 1.567, P = 0.000). These results show that larger-diameter infested branches had larger galls.
Other insects in the gall
We found several other insect species within the dissected galls (n = 876). First, Pyemotes spp. (Fig. 7A, B) parasitises Trigonorhinus sp. larvae in galls and causes larval mortality, but that species was found only on one larva. The larvae of Buprestidae species (Fig. 7C) were found in the gall, accounting for only 3% of larvae of other species. Larvae of Xylotrechus spp. (Fig. 7D) were also found; they accounted for 4.5% of the total number of insects. How these insects are related to Trigonorhinus sp. and how they are related to each other warrant further study.
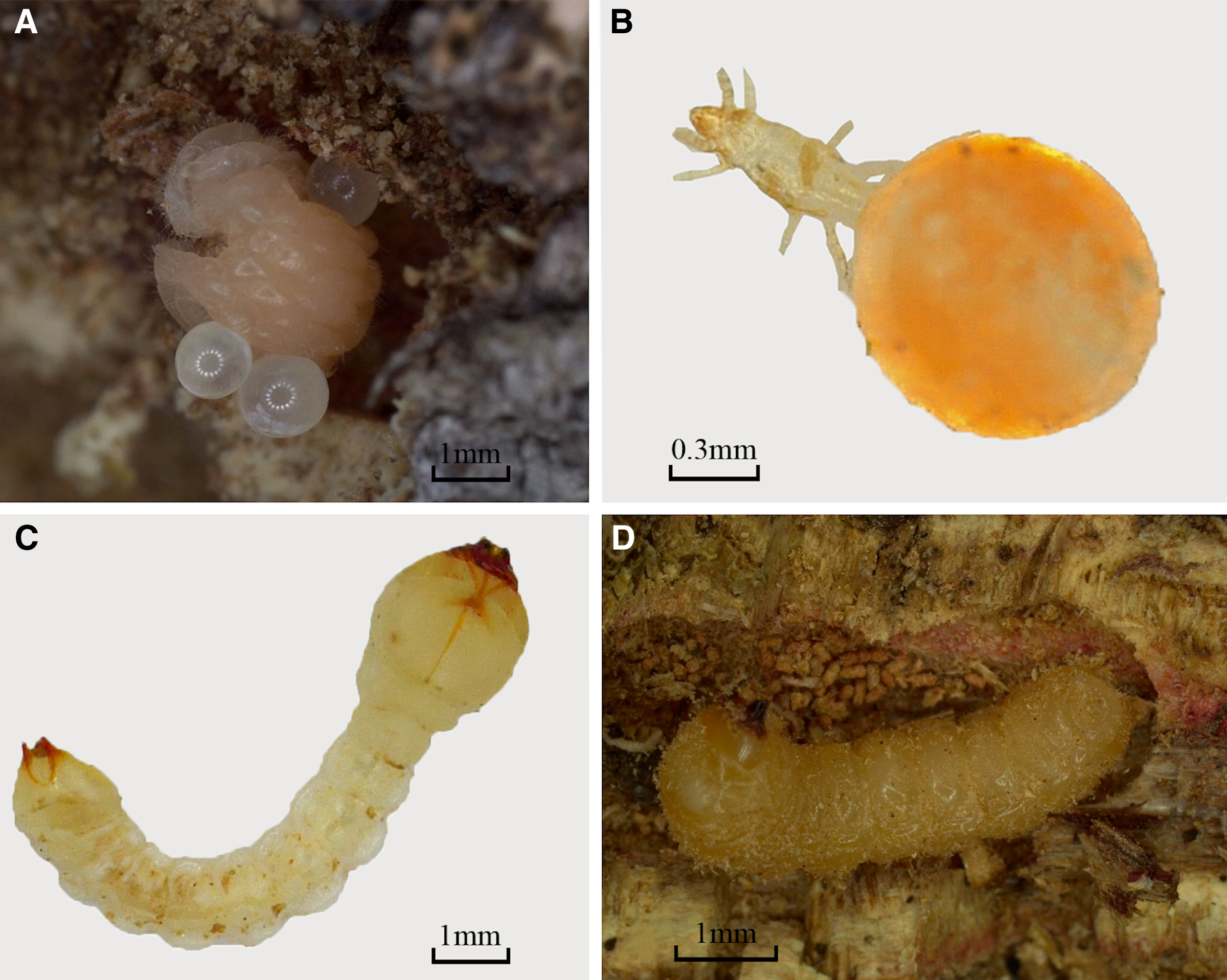
Fig. 7. Other insects found within the galls: A and B, Pyemotes spp.; C, the larvae of Buprestidae species; and D, the larvae of Xylotrechus spp.
Discussion
Trigonorhinus sp. damage to Caragana liouana
The growth of C. liouana infested with galls was obviously worse than that of healthy C. liouana, especially when Trigonorhinus sp. are active (May–July). These results are not unexpected: gall-inducing insects develop and complete their life cycles either within or on plants, deriving nutrition from the plant as they do so (Stone and Schönrogge Reference Stone and Schönrogge2003; Raman Reference Raman2011). Fay et al. (Reference Fay, Hartnett and Knapp1993) showed that the formation of galls can prevent photosynthesis, inhibit growth, and divert sufficient energy to gall tissue, thereby ensuring the growth and development of both the galls and the gall-causing insects. According to Miller and Raman (Reference Miller and Raman2018), the type of symbiosis (exploitative, mutual, and commensal) between two interacting organisms is based on the net gains or losses to the interactants. In our study, the affected host plants (C. liouana) obviously suffered net energy drains, as shown by stunting and death, while the gall-inducing insect (Trigonorhinus sp.) gained and usually successfully reproduced. This demonstrates an exploitative (parasitic) symbiotic relationship.
Gall response to damage by Trigonorhinus sp.
There are three hypotheses as to what factors influence gall growth and development. The first hypothesis states that the relationship between gall size and the number of pests in a gall is positively correlated – that is, the larger the number of pests in the gall, the larger the gall (Wool and Ben Reference Wool and Ben1998; Zhang et al. Reference Zhang, Duan, Duan, Li and Feng2013). Leggo and Shorthouse (Reference Leggo and Shorthouse2006) showed that the overall size of mature galls is determined by the number of larval chambers. The second hypothesis views galls as phenotypic entities that develop under the influences of both plant and insect genotypes (Weis et al. Reference Weis, Walton and Crego1988), and it considers gall size to be the result of natural selection that depends on a balance between the causes of mortality for a given population. Thus, the net selective pressure may favour either an increase or a decrease in gall size (Qi et al. Reference Qi, Duan, Ren and Wu2020). The third hypothesis states that gall development is independent of the number of pests in the galls and that the gall-causing insects serve only to initiate gall formation (Wool and Manheim Reference Wool and Manheim1986; Wool and Burstein Reference Wool and Burstein1991; Wool and Bar-el Reference Wool and Bar-el1995; Stone et al. Reference Stone, Schönrogge, Atkinson, Bellido and Pujade2002; Qi et al. Reference Qi, Duan, Ren and Wu2020). Our observations support the third view: the galls formed by Trigonorhinus sp. in the present study grew constantly during the C. liouana growing season, regardless of whether they were occupied, and we found no significant correlation between gall size and the number of insects in individual galls.
Harmful characteristics of Trigonorhinus sp.
Although most Trigonorhinus sp. galls contained multiple individuals, each larva within a gall occupied its own separate chamber. When the larvae were dissected from the galls and placed together, they killed each other. The presence of multiple insects within a gall may be due to the female laying multiple eggs at close intervals on the same branch, possibly making the most of available resources. Trigonorhinus sp. females select egg-laying sites in attempts to maximise their productivity and their offspring’s survival (Thompson Reference Thompson1988; Craig et al. Reference Craig, Itami and Price1989; Price Reference Price1991). Stone and Schönrogge (Reference Stone and Schönrogge2003) showed that insect egg-laying behaviour occurs before gall formation and that the physical or chemical action of the host plant influences the gall-causing insect’s egg-laying, which in turn determines the insect’s development and survival. Because gall-causing insect larvae obtain nutrients from the host plant, females may select more vigorous host plants on which to lay their eggs (Tooker and Helms Reference Tooker and Helms2014). Gall-forming coleopteran larvae employ many mechanisms during their life cycles. For instance, P. affinis (Coleoptera), a major pest of Gossypium spp., has larvae that usually survive by first forming galls at the base of the stem near the soil and then tunnelling around the stem, creating spiral channels that destroy vascular tissue and cause plant death (Dharajothi et al. Reference Dharajothi, Rajendran and Surulivelu2004). After hatching, H. truncatulus larvae gnaw on host stem tissues to induce galls and begin feeding on plant tissues by cutting longitudinal feeding channels (Agarwal Reference Agarwal1985). Alcides bubo females lay eggs on the stem, and the hatching larvae bore into the stem, inducing thickening (Govindan Reference Govindan1975; Jalaluddin Reference Jalaluddin1999). Likewise, larval Trigonorhinus sp. feeding on stem tissue induce gall production. These coleopteran larvae all feed on stem tissue, which induces galls. The present study enriches this understanding.
Acknowledgements
This research was supported by the National Natural Science Foundation of China (32160372), the Inner Mongolia Autonomous Region Natural Science Foundation (2020BS03014), the Inner Mongolia Autonomous Region Higher Education Research Project (NJZZ18047), the Inner Mongolia Autonomous Region Linxue “Double First-class” Construction Project (DC2100001171), the Inner Mongolia Autonomous Region Science and Technology Plan Project (2019GG007), the Inner Mongolia Agricultural University experimental teaching equipment development and specimen making project (YZ2019007), and the Inner Mongolia Agricultural University High-level Talent Research Startup Plan (203206038). Yanru Zhang and Yaru Hu should be considered joint first authors of this paper.
Competing interests
The authors declare they have no competing interest.